Open Access
ARTICLE
Chemical Composition, Antioxidant and Anticancer Activities of Thymus capitatus Essential Oil: Experimental and Computational Approaches
1 Faculty of Sciences, Arab American University, Ramallah, P600, Palestine
2 Faculty of Medicine, Arab American University, Jenin, P.O. Box 240, Palestine
* Corresponding Author: Hamada Imtara. Email:
(This article belongs to the Special Issue: Innovative Approaches in Experimental Botany: Essential Oils as Natural Therapeutics)
Phyton-International Journal of Experimental Botany 2025, 94(3), 723-737. https://doi.org/10.32604/phyton.2025.063403
Received 14 January 2025; Accepted 21 February 2025; Issue published 31 March 2025
Abstract
Traditional Palestinian medicine uses Thymus capitatus (T. capitatus), a plant recognized for its therapeutic properties due to its high concentration of essential oils such as thymol and carvacrol, to treat skin diseases, gastrointestinal disorders, and respiratory infections. The present study was conducted to evaluate the antioxidant and anticancer activities of T. capitatus essential oil (EO). Moreover, this study employed computational methods including ADMET analysis and molecular docking. Using Gas chromatography-mass spectrometry (GC-MS) analysis, the phytochemical composition of T. capitatus essential oil was identified. The DPPH scavenging method was used to assess antioxidant activity. The Michigan Cancer Foundation-7 (MCF-7) and human colorectal carcinoma (HCT-116) cell lines were used to test for cytotoxic and cytostatic effects. The results of GC/MS analysis revealed 21 chemicals, accounting for 95.82% of their content, with carvacrol (61.23%), p-Cymene (9.49%) and γ-Terpinene (9.4%) being the most abundant. With an IC50 value of 0.27 ± 0.009 mg/mL, the DPPH assay demonstrated a robust scavenging capacity when compared to the IC50 value of butylated hydroxytoluene (BHT), which was 0.37 ± 0.007 mg/mL. T. capitatus EO showed potent anticancer activity on HCT-116 and MCF cell lines. The ADMET in-silico investigations revealed satisfying physicochemical and pharmacokinetics profiles, justified by good human intestinal absorption (HIA exceeding 93%), good permeabilities to the blood-brain barrier (BBB) and central nervous system (CNS), without any inhibition effect on 1A2, 2C9, 2C19, 2D6, and 3A4 cytochromes. Furthermore, all ligands were determined to be non-toxic, with no Ames mutagenicity detected based on toxicity predictions, making them ideal candidates for further drug development.Keywords
Medicinal and aromatic plants (MAPs) have emerged as a major source of natural chemicals, exhibiting a wide range of biologically validated effects in recent decades. The potential of these plants to provide natural remedies in fields including antibacterial, anticancer, anti-inflammatory, and antioxidant therapy has attracted a lot of attention [1,2]. The increasing demand for safer, more cost-effective, and environmentally friendly alternatives for synthetic medications in the treatment of contemporary health issues is reflected in the rising reliance on MAPs. In fact, the development of civilizations is linked with the history of medicinal and aromatic plants [3,4].
The history of various cultures worldwide demonstrates that these plants have always played a significant role in healing, the creation of fragrances, and food preparation [5]. In addition, the use of plants for medicinal purposes is ingrained in all civilizations’ traditions. Natural products are an important source of novel and chemically diverse molecules for drug discovery [6]. Nevertheless, the necessity of developing new natural substances with therapeutic properties persists [7]. These substances consistently offer novel remedies as alternatives to existing pharmaceuticals since they have the advantage of being less harmful than their counterparts made of synthetic materials. Furthermore, for many thousands of years, essential oils (EOs) have been a source of bioactive compounds and are being extensively researched for their potential use as a synthetic product alternative for treating infectious illnesses and numerous pathological ailments linked to oxidative stress [5,8]. Oxidative stress has been linked to many diseases such as cancer, diabetes, cardiovascular, and neurological [9,10]. As a result, antioxidants are recommended, with synthetic ones being the most commonly used. Since synthesized antioxidants are currently being questioned for use because of their potential health risks and toxicity, the investigation of plant antioxidant activity is crucial [11]. Today, the investigation of plant antioxidant activity is becoming crucial. The potent antioxidant properties of medicinal plants are due to their richness of polyphenols, such as phenolic acids, flavonoids, carotenoids, and vitamins (E, C, and A) [12].
The Lamiaceae family, which includes Thymus capitatus L., represents one of the most botanically and pharmacologically significant plant groups due to its rich diversity of secondary metabolites and widespread medicinal applications. Thymus capitatus, an endemic Mediterranean plant, is distributed across regions such as Albania, Algeria, Morocco, Palestine, and Türkiye, among others, reflecting its ecological adaptability and cultural significance in traditional medicine [13,14]. This plant’s therapeutic potential lies in its bioactive compounds, particularly phenolic acids, flavonoids, and essential oils, which are responsible for its potent antioxidant, antiviral, and antibacterial properties [15,16]. These properties have made it a cornerstone in the treatment of various ailments, including diabetes, cancer, heart disease, and gastrointestinal disorders, in regions where it is naturally found. The high concentrations of carvacrol, thymol, and p-Cymene in its essential oil not only contribute to its biological activities but also enhance its pharmacological relevance, bridging its historical use in traditional medicine with its potential in modern pharmaceutical and nutraceutical development [17–19]. This convergence of geographical distribution, biochemical composition, and therapeutic applications underscores the holistic importance of T. capitatus.
The aim of the present work was to use gas chromatography-mass spectrometry (GC-MS) to thoroughly analyzes the chemical composition of T. capitatus essential oil and quantify their antioxidant capacity, via 2,2′-diphenyl-1-picrylhydrazyl (DPPH) assay, to evaluate their biological activities. Additionally, the essential oil of T. capitatus was tested on HCT-116 and MCF-7 to examine its anti-cancer properties. Finally, in silico receptor-ligand docking experiments were used to further examine the antioxidant and anticancer properties of T. capitatus essential oil. By fanticipating the interactions between the oil’s bioactive components and certain molecular targets, these computer analyses attempted to offer comprehensive insights into the mechanisms of action that underlie its therapeutic effects.
2,2′-diphenyl-1-picrylhydrazyl (DPPH), butylated hydroxytoluene (BHT), acidic isopropanol, ethanol, and hexane were purchased from Sigma-Aldrich, Germany. Human colorectal carcinoma (HCT-116, ATCC® CCL-247™) and breast cancer (MCF-7, ATCC® HTB-22™) were obtained from the American Type Culture Collection (Manassas, VA, USA). 3-[4,5-dimethylthiazol-2-yl]-2,5 diphenyl tetrazolium bromide (MTT) DMEM-5671, fetal calf serum, glutamine, penicillin, formazan crystals, Streptomycin, formazan crystals and were purchased from Sigma-Aldrich, St. Louis, MO, USA.
Thymus capitatus aerial parts, including the leaves and flowers, were gathered from the Hebron region of Palestine to extract the plant’s essential oil. The integrity of the plant material and its medicinal ingredients were preserved by careful harvesting. In order to minimize the loss of volatile chemicals during the drying process, the aerial parts were allowed to air dry naturally at room temperature after collecting.
100 g of the plant’s aerial parts were weighed and utilized immediately for the distillation process. 1000 mL of distilled water and the plant material were added to a distillation flask. A Clevenger-type device was then used to hydrodistillate the mixture, which is a common and dependable technique for extracting essential oils. Steam and volatile oils were co-distilled, condensed, and collected over the course of the four-hour distillation process. This time frame guarantees the oil’s complete extraction while maintaining its chemical makeup. Following the distillation process, the essential oil was meticulously separated and dried over anhydrous sodium sulphate to eliminate any remaining moisture that would have risked its stability and purity. After that, the dried essential oil was placed in an airtight container and kept at a regulated temperature of 2 to 4°C in a dark atmosphere to preserve its chemical integrity and stop oxidative deterioration [20].
2.4 2,2-Diphenyl-1-Picryl-Hydrazyl-Hydrate (DPPH) Assay
The DPPH reagent, which was made by dissolving 0.005 g of DPPH in 200 mL of 96% ethanol, was combined with a 50 μL aliquot of the T. capitatus essential oil (EO) dilution series, which had initial concentrations ranging from 15.62 to 500 mg/mL and 0.84 to 104 mg/mL, respectively [20]. The sample and the DPPH solution were added to the total reaction volume, which was set at 1 mL. A spectrophotometer was used to measure the absorbance at 517 nm after the reaction mixture had been incubated for an hour.
The scavenging activity of the DPPH radical was calculated as a percentage of inhibition using the following formula:
%Inhibition=((controlabsorbance−sampleabsorbance)/controlabsorbance)×100
A positive control, which contained 25 μL of Butylated Hydroxytoluene (BHT), a common antioxidant, and a negative control, in which water was used in place of the sample, were compared to the absorbance readings. Furthermore, using the graph of the inhibition percentage plotted against the oil concentrations, the concentration of the essential oil needed to attain 50% radical inhibition (IC50) was determined.
Cell lines from breast cancer (MCF-7, ATCC® HTB-22™) and human colorectal carcinoma (HCT-116, ATCC® CCL-247™) were cultivated in DMEM-5671 medium with 4.5 g/L of glucose. The medium was supplemented with 10% (vol/vol) heat-inactivated fetal calf serum (FCS), 1% glutamine, 1% nonessential amino acids, 100 U/mL penicillin, and 10 μg/mL streptomycin. A humidified environment containing 5% CO2 and a medium pH of 7.4 were used to incubate the cells at 37°C.
2.5.2 Cytotoxic and Cytostatic Assays
For cytotoxic and cytostatic assays, cells were placed into 96-well microtiter plates at densities of 20,000 and 5000 cells/100 μL, respectively. After one day, the cells were exposed to increasing concentrations of essential oil solutions for a 24-h cytotoxic assay and a 72-h cytostatic assay. The MTT test was used to determine cell viability, which was then expressed as a percentage of treated cells’ absorbance in comparison to untreated control cells.
2.5.3 3-[4,5-Dimethylthiazol-2-yl]-2,5 Diphenyl Tetrazolium Bromide (MTT) Assay
The MTT assay was performed according to the protocol described by [21,22]. After being incubated with essential oil solutions, the media were taken out of each well, and the cells were then rinsed with phosphate-buffered saline (PBS). Following that, the cells were cultured for four hours in the dark in serum-free media containing MTT (0.5 mg/mL). Following incubation, the cells were rinsed with PBS, and 100 μL of acidic isopropanol (0.08 N HCl) was added to each well to dissolve the formazan crystals that had developed in the mitochondria. With an ELISA reader, the absorbance of the dissolved MTT formazan was determined at 570 nm. The absorbance of treated cells divided by that of untreated control cells, given as a percentage, was used to determine cell viability.
2.6 Gas Chromatography–Mass Spectrometry (GC-MS) Analysis
The GC-MS system (GC ULTRA S/N 20062969; Polaris QS/N 210729) with a nonpolar fused silica HP-5MS column (60 m × 0.32 mm, 0.25 μm film thickness) was used to analyze the volatile components of the sample. A 1:10 dilution of the sample was made in hexane, and 1 μL of the diluted oil was introduced into the apparatus. The column temperature was planned to rise from 40°C to 260°C at a rate of 2°C per minute, while the injector temperature was kept at 250°C. With a steady flow rate of 1 mL/min, helium was employed as the carrier gas. The identification of the volatile components was performed by determining their Kovats retention indices (Ki), which were calculated relative to a series of n-alkanes (C8–C20). The obtained retention indices were compared with those reported in the literature. Additionally, the mass spectra of the components were matched against those in the NIST MS Library to confirm their identity [23,24].
Physicochemical and pharmacokinetics features of absorption, distribution, metabolism, excretion, and toxicity (ADMET) were properly predicted for three major compounds of the thymus plant using Swiss ADME and pKCSM servers [25]. With the help of Protein Data Bank (PDB), four targeted proteins coded 6GUE, 2CDU, 1OG5, and 4JK4 have been extracted using the X-ray diffraction method with good resolutions of 1.99, 1.8, 2.55, and 2.65 Å, respectively. After that, we used AutoDock 4.2 software to start the docking calculation. We organized the grid box using the AUTOGRID method, setting the sizes 100, 100, and 100 in its three-dimensional structure with a 0.375 Å spacing between them. Ten adaptive algorithms were then run, a total of 25 million evals. Ultimately, we obtained the strongest complex of the fifty conformations that were acquired and then used Discovery Studio 2021 to illustrate the protein-ligand interactions in both two and three dimensions.
The identification of antioxidant activity in vitro of the EO of T. capitatus was determined through the method of the scavenger effect against DPPH radical. Results of the antioxidant activity of T. capitatus EO are summarized in Fig. 1. The purple DPPH radical transforms into a stable yellow molecule. The level of discoloration can be a sign of free radical scavenging capacity. According to the IC50 values, the IC50 (mg/mL) of T. capitatus EO by DPPH radical scavenging assay was found to be 0.27 ± 0.009 mg/mL, while the reference BHT was 0.37 ± 0.007 mg/mL. Our results showed an agreement with previous reports on the oil of T. capitatus [5]. A study conducted by Amarti et al. showed that T. capitatus EO exhibited remarkable antioxidant activity [26]. In the same context, Bounatirou et al. and Zaïri et al. reported that T. capitatus EO demonstrated a high antioxidant power [27,28]. Furthermore, our results were in agreement with studies in which the phenolic compounds were responsible for the antioxidant activity [29,30]. The high concentration of phenolic compounds, such as carvacrol, in the examined thyme species, is associated with the plants’ antioxidant capacities.
Figure 1: Antioxidant results of T. capitatus essential oil by DPPH assay. The data are shown as mean ±SD, and the experiment was conducted at three repetitions
The cytotoxic potential of T. capitatus EO was performed against colorectal carcinoma cells HCT and MCF human breast cancer cell lines. Results of the cytostatic and cytotoxic activity of tested essential oil are summarized in Fig. 2 and Table 1. As can be seen, EO presented a higher anti-proliferative activity/cytotoxicity on HCT-116 and MCF cell lines. Comparing cell survival in the presence of different concentrations of EO indicated higher sensitivity of carcinogenic cell lines in all tested tumor cell lines, although each culture showed different sensitivity, in accordance with determined IC50. It is depicted in this table that T. capitatus EO exhibited a potent cytostatic and cytotoxic activity on HCT-116 with IC50 values equal to 178.85 and 301.05 μg/mL, respectively. For the MCF cell lines, the EO exhibited a cytotoxicity effect with an IC50 value of 464.0.4 μg/mL. Consistent with our results are numerous literature data concerning the chemotherapeutic potential of different Thymus species EO [31,32].
Figure 2: Effects of T. capitatus essential oil on HCT-116 and MCF-7 cells, both cytostatic and cytotoxic, at concentrations ranging from 0 to 2000 μg/mL. The data are presented in the form of mean ± standard deviation (SD)
Volatile compounds contained in T. capitatus such as carvacrol and thymol demonstrated anticancer properties on several cancer cell lines. The mechanisms underlying these effects involve the arrest of cell cycle progression in the S phase and G0/G1 transition, and inducing of apoptosis [14]. Importantly, our findings support the hypothesis that a mixture of phytochemicals with a plethora of biological effects found in EO may have synergistic effects against cancer cell lines and may be more potent against cancer than an isolated compound. Within this context, numerous pre-clinical and clinical studies have suggested lower effectiveness of single phytochemicals compared to whole plant foods against cancer [33].
3.3 Phytochemical Profile of T. capitatus Essential Oil
The gas chromatography–mass spectrometry (GC-MS) method was used to determine the chemical makeup of T. capitatus’s essential oil. The chemical components of T. capitatus essential oil are detailed in Table 2. GC/MS identified 21 chemicals in total, which accounted for 95.82% of the total content. The most abundant components found were carvacrol (61.23%), which was followed by p-Cymene (9.49%) and γ-Terpinene (9.4%). It should be mentioned that, in comparison to the other essential oils, carvacrol was the one with the highest content. α-Thujene (3.81%), Caryophyllene (1.51%), Camphene (1.23%), Tricyclene (4.23%), and α-terpinene (1.09%) were the minor constituents. For the same plant, El Ouariachi et al. confirmed the same composition in their work [15]. It was found that the main constituents in T. capitatus EO from Tunisia were carvacrol (61.6%–83%), p-Cymene (5%–17%), γ-Terpinene (2%–14%), and caryophyllene (1%–4%), but this composition changes depending on the location and the growing season [28]. Similarly, T. capitatus EO from Greece, Portugal [34], Sicily [35,36], and Albania [37] are characterized by a high percentage of carvacrol. However, Italian researchers detected that thymol (29.3%) was considered one of the primary molecules present in T. capitatus while carvacrol represents only (10.8%) [38]. Furthermore, in another recent study from the south of Tunisia, thymol (89%) was found to be one of the major compounds in the EO of T. capitatus. The oil from Türkiye showed carvacrol (35.6%), p-Cymene (26.4%), and thymol (18.6%) as the principal components [18]. In general, carvacrol and p-Cymene are the main chemicals that can be identified in T. capitatus based on our findings and these earlier investigations. The origin of the plant, various environmental factors (such as sunlight, salinity, crop effects, harvest time, and seasonal and climatic conditions), genetic background, and the extraction technique of the plants could all be responsible for the variation observed in the percentages of the main components of the essential oils examined in this and other studies [24,39]. Consequently, the impact of these factors on biosynthesis pathways results in variations in the qualitative and quantitative characteristics of the majority of compounds, leading to the existence of many chemotypes that differentiate essential oils from different sources [40,41].
3.4 ADMET Properties Prediction
In the present study, the major compounds, namely p-Cymene (C6), γ-Terpinene (C8), and Carvacrol (C16) were chosen for ADMET in-silico investigations. Based on important rules like Lipinski’s Rule of Five, Veber, Egan, Muegge, and Ghose guidelines, the physicochemical characteristics of compounds C6, C8, and C16 were assessed. They looked at variables like molecular weight, molar refractive index, rotatable bonds, logP, and hydrogen bond donors and acceptors. All compounds showed molar refractive indices (45.99–48.01) within Ghose’s permissible range (40–130) and complied with molecular weight limits (<500 g/mol). Their favorable membrane permeability and bioavailability were shown by their meeting the requirements for rotatable bonds (<10) and logP (<5). C16 met Lipinski’s requirements for increased cell permeability since it possessed one hydrogen bond donor and one acceptor, but C6 and C8 lacked both (Table 3).
To predict their pharmacokinetic and toxicological profiles, the ADMET (Absorption, Distribution, Metabolism, Excretion, and Toxicity) properties of the main compounds were assessed. The findings from these evaluations are detailed in Table 4. All of the ligands showed high intestinal absorption rates, with C6 at 95.52%, C8 at 95.716%, and C16 at 93.712%, indicating excellent bioavailability potential. Blood-brain barrier (BBB) permeability values suggested limited CNS penetration for all of the compounds, with numeric values below 0.6. Metabolic analysis revealed no interaction with key cytochrome P450 enzymes (CYP1A2, CYP2C19, CYP2C9, and CYP2D6), indicating a low likelihood of metabolic complications. Concerns of drug resistance linked to efflux were also allayed because none of the ligands were P-glycoprotein substrates. Additionally, all ligands were found to be non-toxic, with no Ames mutagenicity found, according to toxicity predictions, which made them excellent candidates for additional drug development.
3.5 Molecular Docking Simulation
A powerful computer method that is widely used to gain a crucial understanding of the molecular mechanisms underlying pharmacologically active medications is molecular docking. In this section, the major compound namely carvacrol was chosen for molecular docking toward four targeted proteins to explore the inhibition mechanisms responsible for antioxidant and anticancer activities.
Due to the overexpression of CDK2 and cyclins A and E in patients with ovarian, breast, colorectal, breast, prostate, and lung cancer, [42–44], protein 6GUE.pdb was selected to assess the anticancer mechanism of carvacrol in T. capitatus essential oil. The crystal structure of human Cyclin-Dependent Kinase 2 (CDK2) in association with Cyclin A2 and the inhibitor AZD5438 is represented by the protein 6GUE. A member of the cyclin-dependent kinase family, CDK2, plays a critical role in regulating the cell cycle, particularly the passage through the S phase and the change from the G1 phase to the S phase. Since dysregulation of CDK2 activity can lead to unregulated cell proliferation and genetic instability, it is commonly associated with cancer. Because of this, CDK2 is a prospective therapeutic target for the treatment of cancer. Inhibitors like AZD5438 are being researched for their ability to reduce tumor growth by stopping CDK2 activity [45]. The obtained results demonstrate that the carvacrol compound was docked to 6GUE.pdb with a binding energy of −5.34 kcal/mol, revealing various intermolecular interactions including two Pi-Sigma bonds detected with Leu134 and Phe80 amino acids residues, more than one Hydrogen bond created towards Leu83 amino acid residue and several Alkyl and Pi-Alkyl bonds, as presented in Fig. 3A.
Figure 3: The intermolecular interactions between carvacrol and its target proteins, 6GUE (A), 2CDU (B), 1OG5 (C), and 4JK4 (D), in both two and three dimensions
The mechanism by which carvacrol suppresses free radicals was examined using the remaining three proteins (2CDU, IOG5, and 4JK4). A water-forming NAD(P)H oxidase from Lactobacillus sanfranciscensis, an enzyme that is a member of the NAD(P)H oxidase family, has a crystal structure represented by the 2CDU. By catalyzing the oxidation of NADH or NADPH, which reduces oxygen to water and controls the amounts of reactive oxygen species (ROS), this enzyme is essential for maintaining cellular redox equilibrium. 2CDU helps the cellular antioxidant defense system by regulating ROS concentrations, which stops oxidative stress and shields cellular constituents from harm [46,47]. As shown in Fig. 3B, carvacrol also complexed to the active sites of the NADPH oxidase protein encoded 2CDU.pdb, exhibiting three hydrogen bonds fixed to Ser115, Lys134, and Asp282 amino residues, more than the Alkyl and Pi-Alkyl bonds found towards Ala11 and Ala303 active sites. Second, 1OG5, a human cytochrome P450 2C9 (CYP2C9) complexed with warfarin, an anticoagulant medication, is shown. A member of the cytochrome P450 superfamily, CYP2C9 is an enzyme that is essential to the oxidative metabolism of several endogenous and exogenous substances, such as steroids, fatty acids, and medications. The liver is the primary site of expression for this enzyme, which metabolizes 15% of pharmaceuticals used in clinical settings. The 1OG5 structure offers comprehensive information about the binding interactions between warfarin and CYP2C9, exposing a new binding pocket and pointing to possible allosteric mechanisms during substrate metabolism [47–49]. As shown in Fig. 3C, the candidate molecule was once more docked to the structure of human cytochrome P450 CYP2C9 encoded as 1OG5.pdb, with a binding energy of −5.09 kcal/mol. This revealed multiple Alkyl and Pi-Alkyl bonds, two hydrogen bonds fixed to the residues of the amino acids Arg124 and Arg433, and one Pi-Pi Stacked bond with the residue of Ile434. Ultimately, the main compound was docked to the crystal structure of bovine serum albumin (4JK4.pdb), which is coded as 4Jk4.pdb. The lowest binding affinity, which was −5.54 kcal/mol, revealed various intermolecular interactions, including those found towards the Tyr149 hydrogen bond, the Arg256 anion bond, and a number of Alkyl and Pi-Alkyl bonds, as shown in Fig. 3D. The 4JK4.pdb of bovine serum albumin (BSA) is important for antioxidant defense because it may bind free radicals and scavenge reactive oxygen species (ROS). By trapping dangerous reactive molecules and halting cell and tissue damage, BSA functions as a molecular buffer in the bloodstream, lowering oxidative stress. Furthermore, BSA can neutralize ROS through thiol-based redox processes because of its high cysteine concentration, especially the free thiol group in Cys-34. Because of its antioxidant properties, BSA is essential for preserving redox equilibrium and shielding biological systems from oxidative stress [46,47,49].
The inhibition mechanisms produced by molecular docking simulations were successfully examined using the validation protocol, in which the resulting intermolecular contacts were compared to the experimental interactions in complex the native ligand bound to each protein target. Thereafter, the superposition process of docked and co-crystallized ligands was done with the root mean square deviations (RMSDs) not exceeding the 2 Å threshold, as presented in Fig. 4.
Figure 4: Results of docking validation protocol (Re-docking methodology). 6GUE (A), 2CDU (B), 1OG5 (C), and 4JK4 (D)
The study highlights the significant antioxidant and anticancer properties of Thymus capitatus essential oil, showing its medicinal potential. Carvacrol, p-Cymene, and γ-Terpinene were the main constituents of the 21 compounds that were discovered by GC-MS analysis. Compared to the synthetic antioxidant BHT, the EO showed a stronger antioxidant capacity. Additionally, T. capitatus EO has strong anticancer effects on MCF-7 and HCT-116 cell lines. Advantageous physicochemical and pharmacokinetic profiles were validated by computational ADMET calculations. These profiles include high intestine absorption in humans, good blood-brain barriers and central nervous system permeability, and non-toxicity with no Ames mutagenicity or inhibition of important cytochrome enzymes. These results make T. capitatus EO a viable option for medication development, especially for uses that target cancer and oxidative stress.
Acknowledgement: Not applicable.
Funding Statement: The authors received no specific funding for this study.
Author Contributions: The authors confirm their contribution to the paper as follows: Conceptualization: Hamada Imtara and Feras Abujaber; methodology: Hamada Imtara, Feras Abujaber and Faady Siouri; software: Aziz Tumeh; validation: Aziz Tumeh and Bashar Saad; formal analysis: Hamada Imtara, Feras Abujaber, Faady Siouri, Aziz Tumeh and Bashar Saad; data curation: Hamada Imtara and Aziz Tumeh; writing—original draft preparation: Hamada Imtara; writing—review and editing: Feras Abujaber, Faady Siouri, Aziz Tumeh and Bashar Saad. All authors reviewed the results and approved the final version of the manuscript.
Availability of Data and Materials: The data that support the findings of this study are available from the corresponding author, upon reasonable request.
Ethics Approval: Not applicable.
Conflicts of Interest: The authors declare no conflicts of interest to report regarding the present study.
References
1. Maleš I, Pedisić S, Zorić Z, Elez-Garofulić I, Repajić M, You L, et al. The medicinal and aromatic plants as ingredients in functional beverage production. J Funct Foods. 2022;96:105210. doi:10.1016/j.jff.2022.105210. [Google Scholar] [CrossRef]
2. Vaou N, Stavropoulou E, Voidarou C, Tsigalou C, Bezirtzoglou E. Towards advances in medicinal plant antimicrobial activity: a review study on challenges and future perspectives. Microorganisms. 2021;9(10):2041. doi:10.3390/microorganisms9102041. [Google Scholar] [PubMed] [CrossRef]
3. Ekor M. The growing use of herbal medicines: issues relating to adverse reactions and challenges in monitoring safety. Front Pharmacol. 2014;4:177. doi:10.3389/fphar.2013.00177. [Google Scholar] [PubMed] [CrossRef]
4. Kala CP. Medicinal and aromatic plants: boon for enterprise development. J Appl Res Med Aromat Plants. 2015;2(4):134–9. doi:10.1016/j.jarmap.2015.05.002. [Google Scholar] [CrossRef]
5. Goudjil MB, Zighmi S, Hamada D, Mahcene Z, Bencheikh SE, Ladjel S. Biological activities of essential oils extracted from Thymus capitatus (Lamiaceae). S Afr N J Bot. 2020;128:274–82. doi:10.1016/j.sajb.2019.11.020. [Google Scholar] [CrossRef]
6. Pye CR, Bertin MJ, Scott Lokey R, Gerwick WH, Linington RG. Retrospective analysis of natural products provides insights for future discovery trends. Proc Natl Acad Sci U S A. 2017;114(22):5601–6. doi:10.1073/pnas.1614680114. [Google Scholar] [PubMed] [CrossRef]
7. Verkaik MJ, Busscher HJ, Jager D, Slomp AM, Abbas F, van der Mei HC. Efficacy of natural antimicrobials in toothpaste formulations against oral biofilms in vitro. J Dent. 2011;39(3):218–24. doi:10.1016/j.jdent.2010.12.007. [Google Scholar] [PubMed] [CrossRef]
8. Sharifi-Rad J, Sureda A, Tenore GC, Daglia M, Sharifi-Rad M, Valussi M, et al. Biological activities of essential oils: from plant chemoecology to traditional healing systems. Molecules. 2017;22(1):70. doi:10.3390/molecules22010070. [Google Scholar] [PubMed] [CrossRef]
9. Elshamy AI, Abd-ElGawad AM, El Gendy AEG, Assaeed AM. Chemical characterization of Euphorbia heterophylla L. essential oils and their antioxidant activity and allelopathic potential on Cenchrus echinatus L. Chem Biodivers. 2019;16(5):e1900051. doi:10.1002/cbdv.201900051. [Google Scholar] [PubMed] [CrossRef]
10. Smilin Bell Aseervatham G, Sivasudha T, Jeyadevi R, Arul Ananth D. Environmental factors and unhealthy lifestyle influence oxidative stress in humans—an overview. Environ Sci Pollut Res Int. 2013;20(7):4356–69. doi:10.1007/s11356-013-1748-0. [Google Scholar] [PubMed] [CrossRef]
11. Tabti L, Dib ME, Gaouar N, Samira B, Tabti B. Antioxidant and antifungal activity of extracts of the aerial parts of Thymus capitatus (L.) hoffmanns against four phytopathogenic fungi of Citrus sinensis. Jundishapur J Nat Pharm Prod. 2014;9(1):49–54. doi:10.17795/jjnpp-13972. [Google Scholar] [PubMed] [CrossRef]
12. Çakir A, Mavi A, Kazaz C, Yildirim A, Küfrevioğlu Ö. Antioxidant activities of the extracts and components of teucrium orientale L. var. orientale. Turk J Chem. 2006;30:483–94. [Google Scholar]
13. Benoutman A, Erbiai EH, Edderdaki FZ, Cherif EK, Saidi R, Lamrani Z, et al. Phytochemical composition, antioxidant and antifungal activity of Thymus capitatus, a medicinal plant collected from northern Morocco. Antibiotics. 2022;11(5):681. doi:10.3390/antibiotics11050681. [Google Scholar] [PubMed] [CrossRef]
14. Bouyahya A, Belmehdi O, Benjouad A, El Hassani RA, Amzazi S, Dakka N, et al. Pharmacological properties and mechanism insights of Moroccan anticancer medicinal plants: what are the next steps? Ind Crops Prod. 2020;147:112198. doi:10.1016/j.indcrop.2020.112198. [Google Scholar] [CrossRef]
15. Ouariachi EE, Paolini J, Bouyanzer A, Tomi P, Hammouti B, Salghi R, et al. Chemical composition and antioxidant activity of essential oils and solvent extracts of Thymus capitatus (L.) Hoffmanns and link from Morocco. J Med Plants Res. 2011;5:5773–8. [Google Scholar]
16. Khaled-Khodja N, Boulekbache-Makhlouf L, Madani K. Phytochemical screening of antioxidant and antibacterial activities of methanolic extracts of some Lamiaceae. Ind Crops Prod. 2014;61:41–8. doi:10.1016/j.indcrop.2014.06.037. [Google Scholar] [CrossRef]
17. El-Mokasabi FM, Al-Sanousi MF, El-Mabrouk RM. Taxonomy and ethnobotany of medicinal plants in eastern region of Libya. [cited 2025 Jan 10]. Available from: https://www.semanticscholar.org/paper/Taxonomy-and-Ethnobotany-of-Medicinal-Plants-in-of-El-Mokasabi-Al-Sanousi/6e8c85c0c856217f05deeaaf3a6853528540fbc9. [Google Scholar]
18. Mkaddem MG, Romdhane M, Ibrahim H, Ennajar M, Lebrihi A, Mathieu F, et al. Essential oil of Thymus capitatus Hoff. et link. from matmata, Tunisia: gas chromatography-mass spectrometry analysis and antimicrobial and antioxidant activities. J Med Food. 2010;13(6):1500–4. doi:10.1089/jmf.2009.0117. [Google Scholar] [PubMed] [CrossRef]
19. Msaada K, Tammar S, Salem N, Bachrouch O, Sriti J, Hammami M, et al. Chemical composition and antioxidant activities of Tunisian Thymus capitatus L. methanolic extract. Int J Food Prop. 2016;19(6):1381–90. doi:10.1080/10942912.2015.1082138. [Google Scholar] [CrossRef]
20. Imtara H, Elamine Y, Lyoussi B. Honey antibacterial effect boosting using Origanum vulgare L. essential oil. Evid Based Complement Alternat Med. 2018;2018:7842583. doi:10.1155/2018/7842583. [Google Scholar] [PubMed] [CrossRef]
21. Imtara H, Kmail A, Touzani S, Khader M, Hamarshi H, Saad B, et al. Chemical analysis and cytotoxic and cytostatic effects of twelve honey samples collected from different regions in Morocco and Palestine. Evid Based Complement Alternat Med. 2019;2019:8768210. doi:10.1155/2019/8768210. [Google Scholar] [PubMed] [CrossRef]
22. Kadan S, Saad B, Sasson Y, Zaid H. In vitro evaluations of cytotoxicity of eight antidiabetic medicinal plants and their effect on GLUT4 translocation. Evid Based Complement Alternat Med. 2013;2013:549345. doi:10.1155/2013/549345. [Google Scholar] [PubMed] [CrossRef]
23. Rached S, Imtara H, Habsaoui A, Mzioud K, Haida S, Saleh A, et al. Characterization, chemical compounds and biological activities of Marrubium vulgare L. essential oil. Processes. 2022;10(10):2110. doi:10.3390/pr10102110. [Google Scholar] [CrossRef]
24. Imtara H, Al-Waili N, Aboulghazi A, Abdellaoui A, Al-Waili T, Lyoussi B. Chemical composition and antioxidant content of Thymus vulgaris honey and Origanum vulgare essential oil; their effect on carbon tetrachloride-induced toxicity. Vet World. 2021;14(1):292–301. doi:10.1016/j.ecoenv.2021.112672. [Google Scholar] [PubMed] [CrossRef]
25. Zarougui S, Er-rajy M, Faris A, Imtara H, El fadili M, Al kamaly O, et al. QSAR, DFT studies, Docking Molecular and simulation dynamic molecular of 2-styrylquinoline derivatives through their anticancer activity. J Saudi Chem Soc. 2023;27:101728. doi:10.1016/j.jscs.2023.101728. [Google Scholar] [CrossRef]
26. Amarti F, Satrani B, Ghanmi M, Aafi A, Farah A, Aarab L, et al. Activité antioxydante et composition chimique des huiles essentielles de quatre espèces de thym du Maroc. Acta Bot Gallica. 2011;158(4):513–23. doi:10.1080/12538078.2011.10516292. [Google Scholar] [CrossRef]
27. Zaïri A, Nouir S, Zarrouk A, Haddad H, Khélifa A, Achour L, et al. Chemical composition, Fatty acids profile and Biological properties of Thymus capitatus (L.) Hoffmanns, essential Oil. Sci Rep. 2019;9(1):20134. doi:10.1038/s41598-019-56580-y. [Google Scholar] [PubMed] [CrossRef]
28. Bounatirou S, Smiti S, Miguel MG, Faleiro L, Rejeb MN, Neffati M, et al. Chemical composition, antioxidant and antibacterial activities of the essential oils isolated from Tunisian Thymus capitatus Hoff. et Link. Food Chem. 2007;105(1):146–55. doi:10.1016/j.foodchem.2007.03.059. [Google Scholar] [CrossRef]
29. César LJ, Javier H, Fernando AJ, Carlos V, Enrique RR, Efrain A, et al. Identification of the main phenolic compounds responsible for the antioxidant activity of Litsea glaucescens Kunth. S Afr N J Bot. 2022;147:208–14. doi:10.1016/j.sajb.2022.01.012. [Google Scholar] [CrossRef]
30. Ouamnina A, Alahyane A, Elateri I, Boutasknit A, Abderrazik M. Relationship between phenolic compounds and antioxidant activity of some Moroccan date palm fruit varieties (Phoenix dactylifera L.a two-year study. Plants (Basel). 2024;13(8):1119. doi:10.3390/plants13081119. [Google Scholar] [PubMed] [CrossRef]
31. Akalin Ciftci G, Incesu Z. The effect of carvacrol on apoptosis of H-RAS and N-RAS transformed cell lines. Turk J Pharm Sci. 2011;8:105–16. [Google Scholar]
32. Sertel S, Eichhorn T, Plinkert PK, Efferth T. Cytotoxicity of Thymus vulgaris essential oil towards human oral cavity squamous cell carcinoma. Anticancer Res. 2011;31(1):81–7. [Google Scholar] [PubMed]
33. Kapinova A, Stefanicka P, Kubatka P, Zubor P, Uramova S, Kello M, et al. Are plant-based functional foods better choice against cancer than single phytochemicals? A critical review of current breast cancer research. Biomed Pharmacother. 2017;96:1465–77. doi:10.1016/j.biopha.2017.11.134. [Google Scholar] [PubMed] [CrossRef]
34. Miguel MG, Gago C, Antunes MD, Megías C, Cortés-Giraldo I, Vioque J, et al. Antioxidant and antiproliferative activities of the essential oils from Thymbra capitata and Thymus species grown in Portugal. Evid Based Complement Alternat Med. 2015;2015:851721. [Google Scholar] [PubMed]
35. Casiglia S, Bruno M, Scandolera E, Senatore F, Senatore F. Influence of harvesting time on composition of the essential oil of Thymus capitatus (L.) Hoffmanns. & Link. growing wild in northern Sicily and its activity on microorganisms affecting historical art Crafts. Arab J Chem. 2019;12(8):2704–12. doi:10.1016/j.arabjc.2015.05.017. [Google Scholar] [CrossRef]
36. Napoli EM, Curcuruto G, Ruberto G. Screening of the essential oil composition of wild Sicilian thyme. Biochem Syst Ecol. 2010;38(4):816–22. doi:10.1016/j.bse.2010.08.008. [Google Scholar] [CrossRef]
37. Ibraliu A, Mi X, Risti MS, Stefanović Z, Shehu J, Pani J. Analysis of essential oils of three wild medicinal plants in Albania. J Med Plants Res. 2011;5:58–62. [Google Scholar]
38. Cosentino S, Tuberoso CI, Pisano B, Satta M, Mascia V, Arzedi E, et al. In-vitro antimicrobial activity and chemical composition of Sardinian Thymus essential oils. Lett Appl Microbiol. 1999;29(2):130–5. doi:10.1046/j.1472-765x.1999.00605.x. [Google Scholar] [PubMed] [CrossRef]
39. Yuan Y, Huang M, Pang YX, Yu FL, Chen C, Liu LW, et al. Variations in essential oil yield, composition, and antioxidant activity of different plant organs from Blumea balsamifera (L.) DC. at different growth times. Molecules. 2016;21(8):1024. doi:10.3390/molecules21081024. [Google Scholar] [PubMed] [CrossRef]
40. Ballester-Costa C, Sendra E, Fernández-López J, Pérez-Álvarez JA, Viuda-Martos M. Assessment of antioxidant and antibacterial properties on meat homogenates of essential oils obtained from four Thymus species achieved from organic growth. Foods. 2017;6(8):59. doi:10.3390/foods6080059. [Google Scholar] [PubMed] [CrossRef]
41. Pérez-Izquierdo C, Serrano-Pérez P, del Rodríguez-Molina MC. Chemical composition, antifungal and phytotoxic activities of Cistus ladanifer L. essential oil and hydrolate. Biocatal Agric Biotechnol. 2022;45:102527. doi:10.1016/j.bcab.2022.102527. [Google Scholar] [CrossRef]
42. Pandiyan S, Wang L. A comparative study of Bazedoxifene, Exemestane, Fulvestrant, Raloxifene, Tryprostatin A, and Vorinostat compounds as potential inhibitors against breast cancer through molecular docking, and molecular dynamics simulation. Chin J Anal Chem. 2023;51(10):100315. doi:10.1016/j.cjac.2023.100315. [Google Scholar] [CrossRef]
43. Ikwu FA, Isyaku Y, Obadawo BS, Lawal HA, Ajibowu SA. In silico design and molecular docking study of CDK2 inhibitors with potent cytotoxic activity against HCT116 colorectal cancer cell line. J Genet Eng Biotechnol. 2020;18(1):51. doi:10.1186/s43141-020-00066-2. [Google Scholar] [PubMed] [CrossRef]
44. Govindarasu M, Ganeshan S, Ansari MA, Alomary MN, AlYahya S, Alghamdi S, et al. In silico modeling and molecular docking insights of kaempferitrin for colon cancer-related molecular targets. J Saudi Chem Soc. 2021;25(9):101319. doi:10.1016/j.jscs.2021.101319. [Google Scholar] [CrossRef]
45. Bank RPD. RCSB PDB-6GUE: CDK2/CyclinA in complex with AZD5438. [cited 2025 Jan 10]. Available from: https://www.rcsb.org/structure/6GUE?utm_source=chatgpt.com. [Google Scholar]
46. Bouzammit R, Lakkab I, fadili El M, Kanzouai Y, Chalkha M, Nakkabi A, et al. Synthesis, crystal structure, antioxidant activity and molecular docking studies of 2-(1-(3-methyl-1-oxo-1,2,3,4-tetrahydronaphthalen-2-yl)ethyl)malononitrile. J Mol Struct. 2024;1312:138582. doi:10.1016/j.molstruc.2024.138582. [Google Scholar] [CrossRef]
47. Kandsi F, Elbouzidi A, Lafdil FZ, Meskali N, Azghar A, Addi M, et al. Antibacterial and antioxidant activity of Dysphania ambrosioides (L.) mosyakin and clemants essential oils: experimental and computational approaches. Antibiotics. 2022;11(4):482. doi:10.3390/antibiotics11040482. [Google Scholar] [PubMed] [CrossRef]
48. Bank RPD. RCSB PDB-1OG5: structure of human cytochrome P450 CYP2C9. [cited 2025 Jan 10]. Available from: https://www.rcsb.org/structure/1og5?utm_source=chatgpt.com. [Google Scholar]
49. Louet M, Labbé CM, Fagnen C, Aono CM, Homem-de-Mello P, Villoutreix BO, et al. Insights into molecular mechanisms of drug metabolism dysfunction of human CYP2C9*30. PLoS One. 2018;13(5):e0197249. doi:10.1371/journal.pone.0197249. [Google Scholar] [PubMed] [CrossRef]
Cite This Article
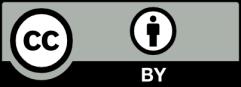
This work is licensed under a Creative Commons Attribution 4.0 International License , which permits unrestricted use, distribution, and reproduction in any medium, provided the original work is properly cited.