Open Access
ARTICLE
Effect of Proline Pretreatment on the Water Stress Response in “Siete Caldos” Pepper Plants
1 Tecnológico Nacional de México Campus Tuxtla Gutiérrez, Carretera Panamericana Km 1080, Tuxtla Gutiérrez, 29050, Chiapas, México
2 SECIHTI-Tecnológico Nacional de México Campus Tuxtla, Carretera Panamericana Km 1080, Tuxtla Gutiérrez, 29050, Chiapas, México
3 SECIHTI-Centro de Investigaciones Biológicas del Noroeste S.C., Instituto Politécnico Nacional 195, Playa Palo de Santa Rita Sur, La Paz, 23096, Baja California Sur, México
4 Tecnológico Nacional de México-Instituto Tecnológico Superior de Cintalapa, Cintalapa de Figueroa, 30400, Chiapas, México
* Corresponding Author: Nancy Ruiz-Lau. Email:
(This article belongs to the Special Issue: Abiotic Stress in Agricultural Crops)
Phyton-International Journal of Experimental Botany 2025, 94(3), 861-873. https://doi.org/10.32604/phyton.2025.062410
Received 18 December 2024; Accepted 12 February 2025; Issue published 31 March 2025
Abstract
Exogenous proline is an effective agent for increasing plant tolerance to abiotic stress in plants. In this study, we evaluated its effect on seedlings of Siete Caldos chili pepper (Capsicum frutescens), a semi-domesticated variety. The Capsicum genus is known for its sensitivity to water stress. We pretreated the seedlings’ roots by immersing them in proline solutions (0, 2.5, 5, 7.5, and 10 mM) for 48 h. Then, we exposed them to water stress using a Hoagland nutrient solution supplemented with 10% polyethylene glycol (PEG-8000) for nine days. We analyzed key physiological and biochemical parameters, including relative water content, cell membrane stability index, electrolyte leakage, chlorophyll, and proline content. The results indicated that proline concentrations of 2.5 and 5 mM significantly increased tolerance to water stress, with 100% survival. These seedlings maintained greater hydration and cell membrane stability compared to non-pretreated seedlings. In contrast, at the highest concentrations (7.5 and 10 mM Pro), survival was 63.63% and 54.54%, respectively. This study demonstrated that exogenous proline enhances water stress tolerance in Capsicum frutescens seedlings by mitigating the negative impact on physiological and biochemical processes vital for survival. This theoretical foundation can be applied to improve chili seedling performance in controlled production environments.Keywords
The chili pepper (Capsicum spp.) is a horticultural crop of importance for both gastronomy and the economy since its fruits are rich in various dietary and nutritional compounds, such as capsaicinoids, vitamins, and minerals [1,2]. In 2022, its global production reached ~37 million tons [3]. However, various environmental factors, such as salinity, drought, and extreme temperatures, can affect chili productivity [4,5].
Chili pepper cultivation is particularly vulnerable to water deficit in three critical stages: vegetative, flowering, and fruit development [6]. Among the morphological changes observed are the curling of the leaves, a delay in growth, and a reduction in the number of leaves [7]. Physically, there is a decrease in water content in shoots and roots, as well as photosynthetic pigments [8]. In biochemical terms, studies show that antioxidant and osmoprotective enzymes, such as proline (Pro), increase [7,9].
The search for strategies to improve tolerance to abiotic stress conditions and increase crop yields has focused on applying compounds such as growth regulators, hormones, polyamines, antioxidants, and exogenously [10]. Researchers have highlighted proline as an amino acid known for its efficacy as an osmoprotectant and signaling molecule, essential in primary metabolism in leaf and root tissues [11]. Several investigations have demonstrated that increasing proline accumulation positively correlates with regulating cell osmosis, stabilizing proteins and enzymes, and eliminating reactive oxygen species (ROS), all contributing to stress tolerance mechanisms [12–14].
Applying exogenous Pro through seed priming, foliar spraying, and rooting/root immersion increases tolerance to abiotic stress [15]. However, its efficiency depends on the development stage, species variety, application time, and concentration [16]. Researchers have reported that applying proline under drought conditions induces an increase in the activity of antioxidant enzymes such as catalase (CAT), superoxide dismutase (SOD), and peroxidases (POD), as well as improvements in fresh weight yield, shoot height, sugar content, photosynthetic pigments, phenolic compounds, and a reduction in electrolyte leakage. In addition to promoting the absorption and accumulation of nitrogen (N), phosphorus (P) and potassium (K+) [17–19].
Researchers have reported that applying exogenous Pro in Capsicum annuum L. culture increases tolerance to salinity and temperature stress, affecting the activity of CAT, SOD, as well as photosynthetic and transpiration rate. They have also found that it improves growth and increases the relative water content (RWC), proline concentration, and proteins [20–22]. Given the need to search for strategies to enhance the natural tolerance of plants to abiotic factors and the limited research on the application of Pro in chili pepper crops under water stress conditions, this study aimed to determine how applying proline affects tolerance to water stress in a semi-domesticated species of Capsicum frutescens (Siete Caldos).
The seeds of Capsicum frutescens L., variety “Siete Caldos”, were obtained from mature fruits collected in a shade house located in the ejido El Porvenir Agrarista (16°10′02′′ N, 91°50′59′′ W; 1488 m a.s.l.) in the municipality of La Trinitaria, Chiapas, Mexico. This semi-domesticated chili variety is cultivated for local consumption.
2.2 Germination and Acclimatization of Seedling
The seedling of Siete Caldos chili pepper (Capsicum frutescens L.) germinated in polystyrene seedbeds using a mixture of peat and perlite (3:1 v/v). Thirty days after emergence, they transferred the seedlings to a hydroponic system with Hoagland nutrient (0.2 mM MgSO4·7H2O, 0.2 mM KH2PO4, 0.8 mM de Ca(NO3)2·4H2O, 1.2 mM KNO3, 10 µM Fe-EDTA, 0.1 µM NiCl·6H2O, 0.1 µM (NH4)6Mo3O24·2H2O, 0.5 µM CuSO4, 1 µM ZnSO4·7H2O, 1 µM MnSO4·H2O, 12.5 µM H3BO3 and 50 µM CaCl2 (Sigma-Aldrich®, Merck KGaA, Darmstadt, Germany) in distilled water) at 1/5 of its ionic strength (i.s.) [23] for acclimatization over three days. The development and growth of the seedlings took place in a growth chamber with a photoperiod of 16 h of light and 8 h of darkness, maintaining an average temperature of 25 ± 2°C and a relative humidity of 52% [24].
2.3 Application of Priming with Proline
We applied proline through root immersion [25]. We placed the seedling 33 days after emergence in Hoagland nutrient solution (1/5 i.s.), supplemented with 0, 2.5, 5, 7.5, and 10 mM of proline (Sigma-Aldrich®, HPLC grade), for 48 h.
We added polyethylene glycol (PEG 8000 Sigma-Aldrich®, reactive grade) to Hoagland’s nutrient solution at 0% and 10% concentrations to induce water stress. We conducted the trial using a completely randomized factorial design with three replications. Each treatment included 15 plants housed in 250 mL glass containers. We maintained the ten treatments for nine days (216 h) with constant aeration, a photoperiod of 16/8 h light/dark, a temperature of 25 ± 2°C, and a relative humidity of 52%.
At the end of exposure to water deficit, we determined the shoot’s height, the root system’s length, the fresh and dry weight, and the presence of flower buds.
2.6 Survival Rate and Relative Water Content
To calculate the percentage of survival, we used Eq. (1) [26] based on the number of plants living (Pl) and dead (Pd).
Survival (\% )=PlPl+PdSurvival (\% )=PlPl+Pd (1)
The relative water content (%RWC) of both the aerial and root systems was determined using Eq. (2), based on the protocol described by Jothimani et al. [27].
%RWC=(fresh weight−dry weightfresh weight)×100%RWC=(fresh weight−dry weightfresh weight)×100 (2)
2.7 Cell Membrane Stability Index and Electrolyte Leakage
We strained three discs of fresh leaf tissue with a 1 cm diameter and 25 mg of root tissue in test tubes containing 15 mL of tri-distilled water (MEYER®, Mexico). We measured the initial electrical conductivity (EC1) after two hours of incubation at room temperature. Then, we subjected the samples to a water bath at 120°C for 20 min to measure the final conductivity (EC2). We measured electrical conductivity using the CON-BTA Vernier® conductivity probe. We used Eq. (3), described by Semida et al. [28], to determine the cell membrane stability index (MSI) and Eq. (4) to calculate electrolyte leakage Restrepo et al. [29].
%MSI=(1−EC1EC2)×100 (3)
%electrolyte leakage =(EC1EC2)×100 (4)
We determined the total chlorophyll photosynthetic pigment following the protocol Inskeep et al. [30] described, with some modifications. We ground 50 mg of fresh leaf in 1.5 mL of 80% acetone (MEYER®, Mexico) and incubated at 4°C in the dark for 60 min. Next, we centrifuged the samples at 10,000 rpm for 5 min. We measured the absorbance at wavelengths (λ) of 664 and 647 nm using a HACH® DR 5000 spectrophotometer. Finally, we calculated the total chlorophyll concentration (μg·mL−1) using Eq. (5).
Chl a=12.634A664−2.52A647 (5)
To measure the proline content in roots and leaves, the methodology of Bates et al. [31] was followed, with modifications by Escalante-Magaña [32]. We transferred the supernatant obtained from fresh leaf and root tissue into test tubes containing a mixture of glacial acetic acid (MEYER®, Mexico) and acidic ninhydrin (Sigma-Aldrich®, Merck KGaA, Darmstadt, Germany). We incubated the samples in a water bath at 96°C for 60 min. Afterward, we added toluene (MEYER®, Mexico) and measured the absorbance of the organic phase at λ 520 nm. We used Eq. (6) to calculate the proline concentration.
Pro (μmoles×g−1)=[(µgproline×mL−1)(mL toluene)115.5µ×µmol−1gsample5] (6)
To perform the statistical analysis, we used a complete factorial design. We analyzed the obtained data through an analysis of variance (ANOVA) and applied the LSD test with 95% reliability using the Statgraphics Centurion XIX software (Statgraphics Technologies, Inc., Madrid, Spain).
3.1 Effect of Water Stress on Growth Parameters
The water stress induced by exposure to PEG (10%) negatively affected the growth of chili pepper plants. In Table 1, shoot height in plants without proline pretreatment [0 mM] and stress exposure decreased by ~16%. However, in the pretreated plants, no statistically significant difference appeared compared to the non-stressed plants. Regarding root length, under stress conditions without pretreatment, we observed a reduction of ~30%, while those pretreated with 2.5 mM of proline increased their length by ~20%, indicating a protective effect. The application of proline (5, 7.5, and 10 mM) under non-stress conditions decreased root length, but under stress conditions, we observed an improvement compared to plants without pretreatment. Additionally, water stress induced early flowering in plants without pretreatment and those pretreated with 7.5 and 10 mM Pro (Table 1).
Fresh and dry shoot and root biomass parameters were reduced by ~50% in stressed plants without pretreatment [0 mM] compared to plants without stress (Table 2). Pretreatment with proline mitigated the adverse effects of osmotic stress in chili pepper plants at concentrations of 2.5 and 5 mM, showing a significant protective effect on fresh weight under stress conditions.
3.2 Effect of Water Stress on Physiological and Biochemical Parameters
Pretreatments with a 2.5 and 5 mM proline positively affected the survival rate by maintaining 100% in plants exposed to water stress (Table 3). For their part, all proline pretreatments [2.5, 5, 7.5, and 10 mM] increased the chlorophyll concentration compared to untreated plants [0 mM Pro] and exposed to stress (10% PEG); under these stress conditions, untreated plants [0 mM] exhibited a drastic decrease of ~37% compared to the control plants.
Table 4 presents the relative water content (RWC) in the shoot and root of chili pepper plants under stress conditions. The values obtained show that the plants pretreated with 2.5 and 5 mM maintained an RWC similar to that of the non-stressed plants, both in the shoot (86.23% and 86.31%) and in the root (78.40% and 79.34%), indicating more excellent resistance to PEG-induced dehydration. Pretreatments with 7.5 and 10 mM reduced the RWC in both the shoot and root, but the reduction was less severe than in plants without pretreatment.
Table 5 shows electrolyte leakage values are higher in root tissue than in shoot. Under stress-free conditions, electrolyte leakage in the shoots and roots of chili pepper plants ranged from ~24% to 35%, respectively. However, treatment with 5 mM Pro showed the lowest electrolyte leakage in both tissues compared to non-stress plants. Under stress conditions, plants without pretreatment experienced an increase of ~105% compared to control plants, indicating more significant cell damage. The shoots of the plants treated with 7.5 and 10 mM Pro showed an increase of ~59.66% and 88.86%, respectively, while in the roots, it was ~75.24% and 81.66% compared to the non-stressed plants. In contrast, shoots and roots pretreated with 2.5 and 5 mM Pro exhibited values similar to control plants.
Since the cell membrane stability index (MSI) inversely correlates with electrolyte leakage, an increase in leakage means a decrease in membrane stability. Under stress-free conditions, plants exhibited an MSI greater than 74% in shoots and 64% in roots. Under stress, the lowest MSI values appeared in plants without pretreatment. In contrast, plants pretreated with 2.5 mM of Pro maintained MSI values comparable to those without stress in both tissues, suggesting that this concentration of Pro effectively preserves membrane stability during water stress (Table 6).
The concentration of proline in leaves and roots increased markedly with pretreatment and with water stress (Table 7). Under stress-free conditions, the 2.5 and 5 mM doses of Pro markedly increased Pro levels by ~4.15 (leaves) and ~3.26 (roots) times compared to non-stressed plants. In contrast, at 7.5 and 10 mM, the increase was ~2 times the basal content in both tissues. In the presence of PEG, the data analysis shows a statistically significant difference in the endogenous proline content between the pretreatments, being more lavish with 2.5 and 5 mM Pro in both tissues.
During water stress, physiological and biochemical processes are affected, severely affecting crop growth and development [33]. Researchers have identified proline as one of the metabolites contributing to plant stress tolerance mechanisms. Exogenous proline can alleviate the damage caused by abiotic stress and improve growth [15,34,35]. Our results showed that the Siete Caldos chili pepper plants (Capsicum frutescens L.) presented a remarkable susceptibility to the water deficit induced by PEG-8000. At the same time, the pretreatment with proline significantly improved their tolerance, evidencing its efficacy in mitigating the adverse effects of stress.
Previous studies have shown that water stress hurts the growth of various crops [36–38]. A typical response to water deficit is the reduction of fresh biomass due to the inhibition of cell expansion that limits the growth of leaves, stems, and roots [39]. In this study, applying different proline concentrations to non-stressed plants did not result in significant height or root length changes, possibly because we assessed the plants nine days after the treatments were applied. However, exposure to 10% PEG-8000 affected shoot height and root length to some degree, a ~50% decrease in fresh biomass of the aerial part compared to control plants (Tables 1 and 2). These results are consistent with those described by Pino et al. [39], who observed a ~82% and 92% decrease in total weight in two wild potato species (Solanum tuberosum and Solanum commersonni), induced in vitro drought with PEG-4000 at 4% and 8%. Other studies have reported similar reductions in fresh and dry weight in different tomato varieties (Solanum lycopersicum) exposed to PEG-6000 at 2%, 4%, 6%, 8%, and 10%, highlighting that the magnitude of the growth reduction depends on the tolerance of each variety [40,41]. Xu et al. [42] attributed a ~16% reduction in fresh and dry biomass in tobacco plants (Nicotiana tabacum L. K326) exposed to 20% PEG-6000 due to cell damage from increased reactive oxygen species. We observed that parameters related to dehydration escape mechanisms were activated nine days after the onset of stress. These results are consistent with the evidence observed in crops such as rice, corn, barley, wheat, chili, and tomato that show that drought accelerates flowering in some plants to complete their life cycle before conditions become more adverse [43–45].
Studies have shown that treatment with appropriate concentrations of exogenous proline can effectively reduce the adverse effects of abiotic stress [25]. High doses negatively impact plant growth by significantly reducing the effect of the root’s total soluble protein or causing toxic effects [46–48]. In our study, proline pretreatment indicated an optimal dose effect on tolerance to water stress tolerance and an optimal dose effect on tolerance to water stress tolerance in this Capsicum variety. The effects of exogenous Pro application vary depending on the species, growth stage, and concentration. Proline pretreatment at 2.5 and 5 mM significantly improved the parameters related to the stress response. In contrast, though beneficial, the positive effects of 7.5 and 10 mM treatments were not drastic. Our results are consistent with previous reports. Alkahtani et al. [19] observed that foliar application of 10 mM Pro significantly increased the fresh and dry weight of the shoot and root in sugar beet plants under drought conditions. Similarly, Elewa et al. [17] found that doses of 12.5 and 25 mM Pro mitigated drought effects in quinoa (Chenopodium quinoa), improving shoot weight, particularly with the 25 mM dose, due to enhanced tissue water status. In wild tobacco (Nicotiana tabacum), cultivating Petit Havana SR1 [49] reported three foliar applications of 10 mM Pro before a water deficit increased shoots and roots biomass. This effect resulted from increased ATP production generated by Pro metabolism, which promotes growth and stress tolerance.
A plant’s survival rate closely depends on its relative water content (RWC), which reflects its water status [50]. In this study, we observed a decrease in survival when the RWC dropped in the tissues of chili plants exposed to PEG. Proline application at doses below 5 mM significantly improved both parameters, likely by maintaining turgor and osmotic balance, as Ibrahim et al. [16] reported for maize with 2 and 4 mM Pro application. Abdelaal et al. [51] reported that 10 mM of proline mitigated RWC reduction in barley under stress conditions. Similar results have been reported in sugar beet (Beta vulgaris L. cv. Samba) and two wheat varieties (Triticum aestivum L.) [18,19].
Although higher doses were less effective than lower ones, they positively influenced chlorophyll content in chili plants, even without stress. Water stress damages photosystems and triggers the overproduction of reactive oxygen species (ROS), reducing chlorophyll content and photosynthetic rate [27,52,53]. Exogenous proline regulates chlorophyll synthesis by enhancing enzymatic activity and adjusting genetic and hormonal regulation, thereby contributing to photosynthetic stability [54]. Demiralay et al. [25] found that in maize plants, a low dose of proline [1 mM] was more effective than a high dose [10 mM] in mitigating photosystem damage. This improvement resulted from enhanced gas exchange, transpiration rate, substomatal CO2 levels, and stomatal conductance [17].
The exogenous application of proline induced an increase in endogenous proline, as observed in this study, where the endogenous proline content in chili plants increased across all treatments except in the control plants (without pretreatment and stress). Exogenous proline stimulates metabolic regulation by activating genes responsible for its synthesis, enhancing osmoregulation, and maintaining osmotic balance [55,56]. These coordinated actions not only provide a direct supply of proline but also modulate the plant’s physiology to increase endogenous proline content, even in the absence of external stress [15,54,57]. Under stress conditions, we observed an increase in endogenous proline (Table 7), which aligns with the findings of Landi et al. [52] in tomato plants (Solanum lycopersicum L., cultivar Red Setter 1753) subjected to 15% PEG-8000 for 48 h. These authors reported endogenous proline concentrations 27 times higher (~6 mg·g−1 FW) than in control plants. They found that proline synthesis correlated with the overexpression of the P5CS gene, thereby enhancing drought tolerance. Similarly, Cacefo et al. [58] observed an increase in proline concentrations in the leaves and roots of wild tobacco pretreated with 10 mM proline and exposed to drought. Elewa et al. [17] also reported increased proline and free amino acid content in quinoa plants under water deficit, improving osmotic adjustment. Farooq et al. [18] highlighted that proline accumulation could result from increased precursors such as ornithine, glutamic acid, and arginine. This response helps mitigate drought effects and sustain growth under adverse conditions [59], as observed in this study’s pretreated chili plants under stress conditions.
Pretreatment with proline is an effective strategy to increase the resilience of Siete Caldos (Capsicum frutescens L.) chili pepper plants against water stress; concentrations of 2.5 and 5 mM of proline proved to be particularly effective in this semi-domesticated species, significantly improving cell membrane stability, water retention, biomass and reducing electrolyte leakage under conditions of water deficit. Because higher proline concentration did not improve the system, it reaffirms the importance of establishing accurate dosing to optimize osmoprotective effects.
Acknowledgement: We gratefully acknowledgement la Secretaría de Ciencia, Humanidades, Tecnología e Innovación (SECIHTI) for awarding the scholarship (801648) to B.O.T.-P.
Funding Statement: Not applicable.
Author Contributions: Conceptualization, Nancy Ruiz-Lau; Formal analysis, Blanca Olivia Trejo-Paniagua; Investigation, Blanca Olivia Trejo-Paniagua and Nancy Ruiz-Lau; Methodology, Blanca Olivia Trejo-Paniagua, María Goretty Caamal-Chan and Nancy Ruiz-Lau; Resources, Rosa Isela Cruz-Rodríguez and Nancy Ruiz-Lau; Supervision, María Goretty Caamal-Chan, Nancy Ruiz-Lau, Rosa Isela Cruz-Rodríguez, Anayancy Lam-Gutiérrez and Víctor Manuel Ruíz-Valdiviezo; Writing—original draft, Blanca Olivia Trejo-Paniagua; Writing—review & editing, María Goretty Caamal-Chan and Nancy Ruiz-Lau; Rosa Isela Cruz-Rodríguez, Anayancy Lam-Gutiérrez and Víctor Manuel Ruíz-Valdiviezo. All authors reviewed the results and approved the final version of the manuscript.
Availability of Data and Materials: The authors confirm that the data supporting the findings of this study are available within the article.
Ethics Approval: Not applicable.
Conflicts of Interest: The authors declare no conflicts of interest to report regarding the present study.
References
1. Kalita R, Bhorali P, Gogoi MB, Gogoi B. Advances in chilli pepper (Capsicum spp.) improvement using modern genetic tools. In: Genetic engineering of crop plants for food and health security. Berlin/Heidelberg, Germany: Springer; 2024. p. 151–68. [cited 2024 Nov 2]. Available from: https://link.springer.com/chapter/10.1007/978-981-99-5034-8_7. [Google Scholar]
2. Rajeswari V, Vijayalakshmi D, Srinivasan S, Swarnapriya R, Varanavasiappan S, Jeyakumar P. Physiological and reproductive abrasions in chilli under combined high temperature and water-deficit stress condition. Plant Physiol Rep. 2024;29(1):88–104. [cited 2024 Aug 6]. Available from: https://link.springer.com/article/10.1007/s40502-023-00764-2. doi:10.1007/s40502-023-00764-2. [Google Scholar]
3. FAOSTAT. [cited 2024 Aug 5]. Available from: https://www.fao.org/faostat/en/#data/QCL/visualize. [Google Scholar]
4. Malika LY, Deshabandu KSHT, De Costa WAJM, Ekanayake S, Herath S, Wijayasiri Weerakoon WM. Physiological traits determining tolerance to intermittent drought in the Capsicum annuum complex. Sci Hortic. 2019;246:21–33. doi:10.1016/j.scienta.2018.10.047. [Google Scholar] [CrossRef]
5. Lim CW, Bae Y, Lee SC. Differential role of Capsicum annuum FANTASTIC FOUR-like gene CaFAF1 on drought and salt stress responses. Environ Exp Bot. 2022;199:104887. doi:10.1016/j.envexpbot.2022.104887. [Google Scholar] [CrossRef]
6. Okunlola GO, Olatunji OA, Akinwale RO, Tariq A, Adelusi AA. Physiological response of the three most cultivated pepper species (Capsicum spp.) in Africa to drought stress imposed at three stages of growth and development. Sci Hortic. 2017;224:198–205. doi:10.1016/j.scienta.2017.06.020. [Google Scholar] [CrossRef]
7. Macias-Bobadilla I, Vargas-Hernandez M, Guevara-Gonzalez RG, Rico-Garcia E, Ocampo-Velazquez RV, Torres-Pacheco I. Differential response to water deficit in chili pepper (Capsicum annuum L.) growing in two types of soil under different irrigation regimes. Agriculture. 2020;10(9):381. [cited 2024 Aug 6]. Available from: https://www.mdpi.com/2077-0472/10/9/381/htm. doi:10.3390/agriculture10090381. [Google Scholar] [CrossRef]
8. Widuri LI, Lakitan B, Sakagami J, Yabuta S, Kartika K, Siaga E. Short-term drought exposure decelerated growth and photosynthetic activities in chili pepper (Capsicum annuum L.). Ann Agric Sci. 2020;65(2):149–58. doi:10.1016/j.aoas.2020.09.002. [Google Scholar] [CrossRef]
9. Mardani S, Tabatabaei SH, Pessarakli M, Zareabyaneh H. Physiological responses of pepper plant (Capsicum annuum L.) to drought stress. J Plant Nutr. 2017;40(10):1453–64. doi:10.1080/01904167.2016.1269342. [Google Scholar] [CrossRef]
10. Zhang H, Sun X, Dai M. Improving crop drought resistance with plant growth regulators and rhizobacteria: Mechanisms, applications, and perspectives. Plant Commun. 2021;3(1):100228. doi:10.1016/j.xplc.2021.100228. [Google Scholar] [PubMed] [CrossRef]
11. Alagoz SM, Lajayer BA, Ghorbanpour M. Proline and soluble carbohydrates biosynthesis and their roles in plants under abiotic stresses. In: Plant stress mitigators. Cambridge, MA, USA: Academic Press; 2023. p. 169–85. [Google Scholar]
12. Qirat M, Shahbaz M, Perveen S. Beneficial role of foliar-applied proline on carrot (Daucus carota L.) under saline conditions. Pak J Bot. 2018;50(5):1735–44. [Google Scholar]
13. Qamar R, Noreen S, Safdar M, Babar ME. Influence of exogenous application of proline on some physio-biochemical parameters of maize (Zea mays L.) under drought stress. Int J Sci Res Publ IJSRP. 2019;9(8):p92117. doi:10.29322/IJSRP.9.08.2019.p92117. [Google Scholar] [CrossRef]
14. Arteaga S, Yabor L, Díez MJ, Prohens J, Boscaiu M, Vicente O. The use of proline in screening for tolerance to drought and salinity in common bean (Phaseolus vulgaris L.) genotypes. Agronomy. 2020;10(6):817. [cited 2024 Aug 7]. Available from: https://www.mdpi.com/2073-4395/10/6/817/htm. doi:10.3390/agronomy10060817. [Google Scholar] [CrossRef]
15. Hosseinifard M, Stefaniak S, Javid MG, Soltani E, Wojtyla Ł, Garnczarska M. Contribution of exogenous proline to abiotic stresses tolerance in plants: a review. Int J Mol Sci. 2022;23(9):5186. [cited 2025 Jan 1]. Available from: https://www.mdpi.com/1422-0067/23/9/5186/htm. doi:10.3390/ijms23095186. [Google Scholar] [PubMed] [CrossRef]
16. Ibrahim AE, El Mageed TA, Abohamid Y, Abdallah H, El-Saadony M, AbuQamar S, et al. Exogenously applied proline enhances morph-physiological responses and yield of drought-stressed maize plants grown under different irrigation systems. Front Plant Sci. 2022;13:897027. doi:10.3389/fpls.2022.897027. [Google Scholar] [PubMed] [CrossRef]
17. Elewa T, Sadak M, Saad A. Proline treatment improves physiological responses in quinoa plants under drought stress. Biosci Res. 2017;14(1):21–33. [Google Scholar]
18. Farooq M, Nawaz A, Chaudhry MAM, Indrasti R, Rehman A. Improving resistance against terminal drought in bread wheat by exogenous application of proline and gamma-aminobutyric acid. J Agron Crop Sci. 2017;203(6):464–72. [cited 2024 Jan 30]. Available from: https://onlinelibrary.wiley.com/doi/full/10.1111/jac.12222. doi:10.1111/jac.12222. [Google Scholar] [CrossRef]
19. Alkahtani MDF, Hafez YM, Attia K, Rashwan E, Al Husnain L, Algwaiz HIM, et al. Evaluation of silicon and proline application on the oxidative machinery in drought-stressed sugar beet. Antioxidants. 2021;10(3):398. [cited 2024 Aug 26]. Available from: https://www.mdpi.com/2076-3921/10/3/398/htm. doi:10.3390/antiox10030398. [Google Scholar] [PubMed] [CrossRef]
20. da Silva Sá Francisco V, de Lima Geovani S, dos Santos João B, Hans RG, dos Anjos Soares Lauriane A, Lourival FC, et al. Growth and physiological aspects of bell pepper (Capsicum annuum) under saline stress and exogenous application of proline. Afr J Biotechnol. 2016;15(36):1970–6. doi:10.5897/AJB2016.15441. [Google Scholar] [CrossRef]
21. Jamil M, Kharal MA, Ahmad M, Abbasi G, Nazli F, Hussain A, et al. Inducing salinity tolerance in red pepper (Capsicum annuum L.) through exogenous application of proline and L-tryptophan. Soil Env. 2018;37(2):160–8. doi:10.25252/SE/18/31052. [Google Scholar] [CrossRef]
22. Almeida dos Anjos Soares L, de Lima GS, dos Santos JB, Gheyi HR, Nobre RG, Soares da Silva S, et al. Growth and physical characterization of fruits of bell pepper (Capsicum annuum L.) cv. ‘All Big’ subjected to saline stress and exogenous application of proline. Aust J Crop Sci. 2018;12(9):1528–35. doi:10.21475/ajcs.18.12.09.PNE1306. [Google Scholar] [CrossRef]
23. de Freitas PAF, de Carvalho HH, Costa JH, de Souza Miranda R, da Cruz Saraiva KD, de Oliveira FDB, et al. Salt acclimation in Sorghum plants by exogenous proline: physiological and biochemical changes and regulation of proline metabolism. Plant Cell Rep. 2019;38(3):403–16. doi:10.1007/s00299-019-02382-5. [Google Scholar] [PubMed] [CrossRef]
24. Trejo-Paniagua BO, Caamal-Chan MG, Cruz-Rodríguez RI, Lam-Gutiérrez A, Ruiz-Lau N. Physiological, biochemical, and molecular response in siete caldos chili pepper plants (Capsicum frutescens) exposed to water deficit. Horticulturae. 2024;10(6):558. [cited 2024 Jun 23]. Available from: https://www.mdpi.com/2311-7524/10/6/558/htm. doi:10.3390/horticulturae10060558. [Google Scholar] [CrossRef]
25. Demiralay M, Altuntaş C, Sezgin A, Terzi R, Kadioğlu A. Application of proline to root medium is more effective for amelioration of photosynthetic damages as compared to foliar spraying or seed soaking in maize seedlings under short-term drought. Turk J Biol. 2017;41(4):649–60. [cited 2024 Jan 30]. Available from: https://journals.tubitak.gov.tr/biology/vol41/iss4/9. doi:10.3906/biy-1702-19. [Google Scholar] [CrossRef]
26. Jiménez Cueva TP, Palacios Herrera, BG. Establecimiento de una plantación de nueve especies forestales con fines de rehabilitación de suelos degradados en la hacienda la Florencia en el Cantón y provincia de Loja. Cienc Lat Rev Cient Multidiscip. 2023;7(4):2036–51. doi:10.37811/CL_RCM.V7I4.7031. [Google Scholar] [CrossRef]
27. Jothimani K, Arulbalachandran D. Physiological and biochemical studies of black gram (Vigna mungo (L.) Hepper) under polyethylene glycol induced drought stress. Biocatal Agric Biotechnol. 2020;29:101777. doi:10.1016/j.bcab.2020. [Google Scholar] [CrossRef]
28. Semida WM, Abdelkhalik A, Rady MOA, Marey RA, Abd El-Mageed TA. Exogenously applied proline enhances growth and productivity of drought stressed onion by improving photosynthetic efficiency, water use efficiency and up-regulating osmoprotectants. Sci Hortic. 2020;272:109580. doi:10.1016/j.scienta.2020.109580. [Google Scholar] [CrossRef]
29. Restrepo H, Gómez MI, Garzón A, Manrique L, Alzate F, López J, et al. Respuesta bioquímica de plántulas de maíz (Zea mays L.) a diferentes condiciones de temperaturas nocturnas. Rev Colomb Cienc Hortic. 2014;7(2):252–62. [Google Scholar]
30. Inskeep WP, Bloom PR. Extinction coefficients of chlorophyll a and B in n, n-dimethylformamide and 80% acetone. Plant Physiol. 1985;77(2):483–5. doi:10.1104/pp.77.2.483. [Google Scholar] [PubMed] [CrossRef]
31. Bates LS, Waldren RP, Teare ID. Short communication rapid determination of free proline for water-stress studies. Plant Soil. 1973;39(1):205–7. doi:10.1007/BF00018060. [Google Scholar] [CrossRef]
32. Escalante-Magaña CA. Tesis que presenta Camilo Andres Escalante Magaña En opción al título de DOCTORADO EN CIENCIAS (Ciencias Biológicas: Opción Bioquímica y Biología Molecular). Mérida, México: Centro de Investigación Científica de Yucatán; 2020. [Google Scholar]
33. Yu B, Chao DY, Zhao Y. How plants sense and respond to osmotic stress. J Integr Plant Biol. 2024;66(3):394–423. [cited 2024 Nov 18]. Available from: https://pubmed.ncbi.nlm.nih.gov/38329193/. doi:10.1111/jipb.13622. [Google Scholar] [PubMed] [CrossRef]
34. Ghosh UK, Islam MN, Siddiqui MN, Cao X, Khan MAR. Proline, a multifaceted signalling molecule in plant responses to abiotic stress: understanding the physiological mechanisms. Plant Biol. 2022;24(2):227–39. [cited 2024 Nov 18]. Available from: https://pubmed.ncbi.nlm.nih.gov/34796604/. doi:10.1111/plb.13363. [Google Scholar] [PubMed] [CrossRef]
35. Zulfiqar F, Ashraf M. Proline alleviates abiotic stress induced oxidative stress in plants. J Plant Growth Regul. 2023;42(8):4629–51. [cited 2024 Nov 18]. Available from: https://link.springer.com/article/10.1007/s00344-022-10839-3. doi:10.1007/s00344-022-10839-3. [Google Scholar] [CrossRef]
36. Ahluwalia O, Singh PC, Bhatia R. A review on drought stress in plants: implications, mitigation and the role of plant growth promoting rhizobacteria. Resour Environ Sustain. 2021;5(16):100032. doi:10.1016/j.resenv.2021.100032. [Google Scholar] [CrossRef]
37. Kompas T, Che TN, Grafton RQ. Global impacts of heat and water stress on food production and severe food insecurity. Sci Rep. 2024;14(1):1–9. doi:10.1038/s41598-024-65274-z. [Google Scholar] [PubMed] [CrossRef]
38. Vadez V, Grondin A, Chenu K, Henry A, Laplaze L, Millet EJ, et al. Crop traits and production under drought. Nat Rev Earth Env. 2024;5(3):211–25. [cited 2024 Nov 18]. Available from: https://www.nature.com/articles/s43017-023-00514-w. doi:10.1038/s43017-023-00514-w. [Google Scholar] [CrossRef]
39. Pino MT, Ávila A, Molina A, Jeknic Z, Chen THH. Enhanced in vitro drought tolerance of Solanum tuberosum and Solanum commersonii plants overexpressing the ScCBF1 gene. Cienc Investig Agrar. 2013;40(1):171–84. doi:10.4067/S0718-16202013000100015. [Google Scholar] [CrossRef]
40. Ayaz M, Ahmad R, Shahzad M, Khan N, Shah MM, Khan SA. Drought stress stunt tomato plant growth and up-regulate expression of SlAREB, SlNCED3, and SlERF024 genes. Sci Hortic. 2015;195:48–55. doi:10.1016/j.scienta.2015.08.025. [Google Scholar] [CrossRef]
41. Akram S, Saleem Y, Khan AR, Wadood A, Hameed A, Sajjad S. Identification of drought-tolerant tomato genotypes using multi-trait index at early growth stage. J Soil Sci Plant Nutr. 2024;24(2):2456–68. [cited 2024 Aug 12]. Available from: https://link.springer.com/article/10.1007/s42729-024-01665-5. doi:10.1007/s42729-024-01665-5. [Google Scholar] [CrossRef]
42. Xu J, Zhou Y, Xu Z, Chen Z, Duan L. Combining physiological and metabolomic analysis to unravel the regulations of coronatine alleviating water stress in tobacco (Nicotiana tabacum L.). Biomolecules. 2020;10(1):99. [cited 2024 Aug 12]. Available from: https://www.mdpi.com/2218-273X/10/1/99/htm. doi:10.3390/biom10010099. [Google Scholar] [PubMed] [CrossRef]
43. Shavrukov Y, Kurishbayev A, Jatayev S, Shvidchenko V, Zotova L, Koekemoer F, et al. Early flowering as a drought escape mechanism in plants: how can it aid wheat production? Front Plant Sci. 2017;8:1950. doi:10.3389/fpls.2017.01950. [Google Scholar]
44. Bürger M. Escaping the drought: the OST1-VOZ1 module regulates early flowering in tomato. Plant Cell. 2022;34(5):1886–7. doi:10.1093/plcell/koac042. [Google Scholar] [PubMed] [CrossRef]
45. Chen M, Zhang TL, Hu CG, Zhang JZ. The role of drought and temperature stress in the regulation of flowering time in annuals and perennials. Agronomy. 2023;13(12):3034. [cited 2024 Aug 25]. Available from: https://www.mdpi.com/2073-4395/13/12/3034/htm. doi:10.3390/agronomy13123034. [Google Scholar] [CrossRef]
46. Nanjo T, Fujita M, Seki M, Kato T, Tabata S, Shinozaki K. Toxicity of free proline revealed in an Arabidopsis T-DNA-tagged mutant deficient in proline dehydrogenase. Plant Cell Physiol. 2003;44(5):541–8. doi:10.1093/pcp/pcg066. [Google Scholar] [PubMed] [CrossRef]
47. Dawood MG, Taie HAA, Nassar RMA, Abdelhamid MT, Schmidhalter U. The changes induced in the physiological, biochemical and anatomical characteristics of Vicia faba by the exogenous application of proline under seawater stress. S Afr J Bot. 2014;93:54–63. doi:10.1016/j.sajb.2014.03.002. [Google Scholar] [CrossRef]
48. Suekawa M, Fujikawa Y, Esaka M. Exogenous proline has favorable effects on growth and browning suppression in rice but not in tobacco. Plant Physiol Biochem. 2019;142:1–7. doi:10.1016/j.plaphy.2019.06.032. [Google Scholar] [PubMed] [CrossRef]
49. Kahlaoui B, Hachicha M, Misle E, Fidalgo F, Teixeira J. Physiological and biochemical responses to the exogenous application of proline of tomato plants irrigated with saline water. J Saudi Soc Agric Sci. 2018;17(1):17–23. doi:10.1016/j.jssas.2015.12.002. [Google Scholar] [CrossRef]
50. Soltys-Kalina D, Plich J, Strzelczyk-Żyta D, Śliwka J, Marczewski W. The effect of drought stress on the leaf relative water content and Tuber yield of a half-sib family of ‘Katahdin’-derived potato cultivars. Breed Sci. 2016;66(2):328–31. [cited 2024 Aug 26]. Available from: https://pmc.ncbi.nlm.nih.gov/articles/PMC4785010/. doi:10.1270/jsbbs.66.328. [Google Scholar] [CrossRef]
51. Abdelaal KAA, Attia KA, Alamery SF, El-Afry MM, Ghazy AI, Tantawy DS, et al. Exogenous application of proline and salicylic acid can mitigate the injurious impacts of drought stress on barley plants associated with physiological and histological characters. Sustainability. 2020;12(5):1736. [cited 2024 Aug 26]. Available from: https://www.mdpi.com/2071-1050/12/5/1736/htm. doi:10.3390/su12051736. [Google Scholar] [CrossRef]
52. Landi S, Nurcato R, De Lillo A, Lentini M, Grillo S, Esposito S. Glucose-6-phosphate dehydrogenase plays a central role in the response of tomato (Solanum lycopersicum) plants to short and long-term drought. Plant Physiol Biochem. 2016;105:79–89. doi:10.1016/j.plaphy.2016.04.013. [Google Scholar] [PubMed] [CrossRef]
53. Suminar E, Budiarto R, Nuraini A, Mubarok S, Ezura H. Morpho-physiological responses of iaa9 tomato mutants to different levels of PEG simulated drought stress. Biodiversitas. 2022;23(6):3115. [Google Scholar]
54. Hossain A, Garai S, Mondal M, Hamed Abdel Latef AA. The key roles of proline against heat, drought and salinity-induced oxidative stress in wheat (Triticum aestivum L.). In: Organic solutes, oxidative stress, and antioxidant enzymes under abiotic stressors. Boca Raton, FL, USA: CRC Press; 2021. p. 171–90. [cited 2023 May 14]. Available from: https://www.taylorfrancis.com/chapters/edit/10.1201/9781003022879-9/key-roles-proline-heat-drought-salinity-induced-oxidative-stress-wheat-triticum-aestivum-akbar-hossain-sourav-garai-mousumi-mondal-arafat-abdel-hamed-abdel-latef. [Google Scholar]
55. Szepesi Á, Szőllősi R. Mechanism of proline biosynthesis and role of proline metabolism enzymes under environmental stress in plants. In: Plant metabolites and regulation under environmental stress. Cambridge, MA, USA: Academic Press; 2018. p. 337–53. [Google Scholar]
56. El Moukhtari A, Cabassa-Hourton C, Farissi M, Savouré A. How does proline treatment promote salt stress tolerance during crop plant development? Front Plant Sci. 2020;11:1127. [cited 2024 Aug 7]. Available from: https://pubmed.ncbi.nlm.nih.gov/32793273/. doi:10.3389/fpls.2020.01127. [Google Scholar] [PubMed] [CrossRef]
57. Tabatabai SMT, Goshasbi F, Bakhshi B. Evaluation of the effect of polyethylene glycol (PEG) on germination and morphological characteristics of bread wheat. Cereal Res Commun. 2022;50(4):1009–15. [cited 2024 Aug 11]. Available from: https://link.springer.com/article/10.1007/s42976-022-00242-9. doi:10.1007/s42976-022-00242-9. [Google Scholar] [CrossRef]
58. Cacefo V, Ribas AF, Guidorizi KA, Vieira LGE. Exogenous proline alters the leaf ionomic profiles of transgenic and wild-type tobacco plants under water deficit. Ind Crops Prod. 2021;170:113830. doi:10.1016/j.indcrop.2021.113830. [Google Scholar] [CrossRef]
59. Forlani G, Trovato M, Funck D, Signorelli S. Regulation of proline accumulation and its molecular and physiological functions in stress defence. In: Osmoprotectant-mediated abiotic stress tolerance in plants: recent advances and future perspectives. Berlin/Heidelberg, Germany: Springer; 2019. p. 73–97. [cited 2023 May 14]. Available from: https://link.springer.com/chapter/10.1007/978-3-030-27423-8_3. [Google Scholar]
Cite This Article
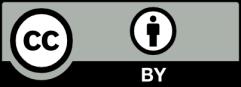
This work is licensed under a Creative Commons Attribution 4.0 International License , which permits unrestricted use, distribution, and reproduction in any medium, provided the original work is properly cited.