Open Access
ARTICLE
Nitrogen/Phosphorus Ratio Affected the Growth of an Invasive Plant Alternanthera philoxeroides under Cadmium Stress
1 School of Life Science and Engineering, Handan University, Handan, 056005, China
2 College of Life Sciences, Hebei University, Baoding, 071002, China
* Corresponding Author: Chao Si. Email:
(This article belongs to the Special Issue: Plant and Environments)
Phyton-International Journal of Experimental Botany 2025, 94(3), 709-721. https://doi.org/10.32604/phyton.2025.062281
Received 14 December 2024; Accepted 10 February 2025; Issue published 31 March 2025
Abstract
Plant invasion poses a serious environmental challenge to freshwater ecosystems. Heavy metal pollution and nutrient enrichment, particularly nitrogen (N) and phosphorus (P), are two additional critical environmental issues known to influence the growth of invasive plants. Notably, the N/P ratio in the environments is often inconstant; however, whether these variations can cause differential responses in invasive plants remains underexplored, despite its crucial importance for understanding plant invasiveness. In this study, we examined the effects of different N/P ratios (4:1, 16:1, 32:1, 64:1) on the growth of a widespread invasive plant Alternanthera philoxeroides in cadmium (Cd) polluted water, while maintaining either a constant N concentration (2 mmol L−1) or a constant P concentration (0.5 mmol L−1). Two control treatments were also included: one without Cd, N, or P, and another with Cd but without N or P. The addition of N and P did not mitigate the inhibitory effects of Cd on A. philoxeroides growth nor did it enhance its tolerance. However, under conditions of constant N concentration, the N/P ratio of 4:1 significantly increased both the main stem length and the number of nodes in A. philoxeroides under Cd stress, potentially enhancing its expansion and clonal growth. Our results suggest that A. philoxeroides exhibits greater sensitivity to variations in the N/P ratio when N concentration is held constant, compared to when P concentration is constant in Cd polluted water. This study investigated the effects of varying N/P ratios on invasive plants under Cd pollution, which has been underexplored in previous research. The findings provide an experimental basis for controlling and preventing the spread of invasive plants in freshwater systems polluted with Cd and experiencing eutrophication.Keywords
Heavy metal pollution and eutrophication are both serious environmental issues in freshwater habitats, primarily caused by anthropogenic activities [1–4]. Industrial processes generate wastewater, waste gas, and waste residues that are discharged into the environment, thereby introducing heavy metals [5,6]. Notably, some heavy metals such as cadmium (Cd), mercury (Hg), and lead (Pb) are non-essential elements and even with toxic effects on living organisms [7,8]. Additionally, inefficient fertilizer use in agricultural practices can contribute to elevated levels of nutrients like nitrogen (N) and phosphorus (P) in the soil [9–12]. Furthermore, daily human activities including sewage discharge and waste disposal also release heavy metals as well as excessive amounts of N and P into the environment [5,12]. Ultimately, these pollutants enter freshwater ecosystems through mechanisms such as direct discharge, atmospheric deposition, and runoff causing significant harm to the ecosystem health [13–16].
The invasion of introduced plant species poses a formidable challenge to freshwater ecosystems [17–19]. Once established in an area, these invasive species can rapidly exploit the ecological niches of native species, leading to their displacement and eventual dominance [20,21]. In habitats already compromised by pollution and eutrophication, the behavior of invasive plants may exacerbate existing issues [22]. Previous studies have investigated the combined effects of heavy metals and nutrients, suggesting that elevated nutrient levels within a certain range could alleviate the inhibitory impacts of heavy metals on invasive plants [23–25]. Furthermore, due to the intricate and variable nature of heavy metals and nutrients in freshwater systems, some research has explored not only nutrient concentrations but also the interactions between different nitrogen sources and heavy metals [26–28]. The N/P ratio is also a crucial factor in nutrient dynamics that may influence the effects of heavy metals; however, its impact on heavy metal effects has received limited attention thus far.
An appropriate N/P ratio not only promotes plant growth and development, but also enhances photosynthesis efficiency, nutrient utilization efficiency, and adaptability to environmental changes and stresses [29–32]. Previous studies have indicated that certain plant species, such as alpine grasses, achieve optimal growth at an N/P ratio of 135:1 (through either nitrogen addition or phosphorus reduction) [33]. Other plant species may thrive at different N/P ratios, such as Acer mono Maxim. demonstrated superior growth performance at a N/P ratio of 5:4 [34]. Increasing N and P levels can enhance root neck diameter, chlorophyll content, and root development to improve the aboveground performance of plants [35,36]. For instance, N/P ratio of 5:4 can effectively stimulate root growth and development, contributing to overall plant health and productivity of A. mono [34]. Conversely, an imbalance in the N/P ratio can lead to suboptimal leaf growth with reduced photosynthetic efficiency ultimately inhibiting aboveground structure growth and development [33]. The importance of N/P ratios is particularly evident in helping plants adapt to environmental stresses such as flooding [37] and environmental pollution (including Cd, aluminum and ammonia) [38–40]. Currently, limited research has explored the responses of invasive plants to Cd pollution under varying N/P ratios in freshwater systems. Based on previous studies, it is hypothesized that different N:P ratios influence the growth and physiological responses of invasive plants exposed to Cd. Clarifying these impacts is crucial for assessing the invasion potential of specific species, predicting their spread rates, and establishing a robust theoretical foundation for managing and controlling invasive plants in freshwater ecosystems. Consequently, further research in this area is essential.
To investigate the potential impact of N/P ratio on the growth response of invasive plants under Cd stress, we conducted a greenhouse experiment using Alternanthera philoxeroides, a common invasive species. We cultivated single fragments of A. philoxeroides in containers filled with a cadmium solution, maintaining either a constant nitrogen concentration or a constant phosphorus concentration while varying the N/P ratios. As controls, we also grew single fragments in containers without Cd and NP, as well as in containers with Cd but without N and P. Specifically, we aimed to test the following hypotheses: (1) the response of A. philoxeroides to Cd is affected by N/P ratio; (2) the effect of N/P ratio on A. philoxeroides depends on whether N or P concentration remains constant.
Alternanthera philoxeroides (Mart.) Griseb., is a perennial clonal plant that was introduced into China as a forage crop from South America in the 1930s [41,42]. The stolon of A. philoxeroides exhibits the ability to produce opposite leaves, side branches, and roots at each node, thereby functioning as ramets capable of establishing and clonal growth [25]. Due to its quickly asexual reproduction, high phenotypic plasticity, and strong stress resistance, this species has emerged as one of the most problematic invasive plants in both aquatic and terrestrial habitats in China [43–47].
The plant materials used in this study were collected by cutting from naturally growing A. philoxeroides plants on the Handan University campus, located in Hebei province, China. Prior to the experiment, the plant materials were cultivated in plastic boxes filled with tap water in a greenhouse (36°34′N, 114°29′E) at Handan University.
On 15 August 2023, more than one hundred fragments were cut from the aforementioned greenhouse-cultivated stock plant. Each fragment consisted of one node, two fully expanded opposite leaves, and a nascent bud in each axil on both sides. The fragments were subsequently placed in plastic containers filled with tap water for hydroponic cultivation in the greenhouse at Handan University, as part of this experimental procedure (as shown in Fig. 1).
Figure 1: Schematic representation of the experimental design
To test the effect of N/P ratio, we conducted an experimental design involving two constant concentration treatments (maintaining either N or P concentration at a constant level) that were fully crossed with four N/P ratio treatments (4:1, 16:1, 32:1, and 64:1). Additionally, two control treatments were included, one without any N and P nutrients or Cd presence and another with Cd presence but lacking both N and P nutrients.
When maintaining a constant N concentration, it was set at 2 mmol L−1, while for a constant P concentration, it was set at 0.1 mmol L−1. N and P were supplied by Ca (NO3)2·4H2O (Calcium nitrate, the purity is more than 99.0 percent, Tianjin Yongda Chemical Reagent Co., Ltd., Tianjin, China) and KH2PO4 (Potassium dihydrogen phosphate, the purity is more than 99.5 %, Xilong Scientific Co., Ltd., Shantou, China), respectively. Cd was provided by 3CdSO4·8H2O (Cadmium sulfate, the purity is more than 99.0%, Shanghai Chemical Reagent Co., Shanghai, China) at a concentration of 1 mg L−1 Cd2+. Each treatment was replicated ten times, resulting in a total of 100 containers.
On 21 August 2023, a total of 100 fragments with the similar initial size (each measuring 13.2 cm in length and containing five pairs of leaves, n = 20) were selected from the cultured materials for experimental purposes. These fragments were individually inserted into designated apertures on floating plates within a container (with a diameter of 10 cm and height of 9 cm), which was filled with an appropriate treatment solution volume of 800 mL. The roots were fully submerged while the leaves and stems grew above the water surface level. All containers were randomly positioned on a bench within the same greenhouse for material cultivation.
The experiment ran for a total of 20 days, concluding on 09 September 2023. By the end of the experiment, the heights of plants in many containers had reached levels comparable to those typically observed in the natural habitat of this species. Throughout the experimental period, the average temperature within the greenhouse was 25.0°C, while the average humidity was 58.2%. To compensate for water loss resulting from transpiration and evaporation processes, tap water was periodically replenished every 1–2 days during the course of the experiment. The solution in the container will be replaced on 03 September 2023.
2.3 Measurements and Data Analysis
At harvest, we measured the length of main stem and counted the number of nodes of A. philoxeroides in each container. The plants were divided into shoot (leaf and stem) and root parts, and subsequently dried at 70°C until a constant weight was achieved. The samples were weighed multiple times using an electronic balance (JT3003D, precision: 0.001 g, Lichen Technology Co., Changsha, China) to ensure that the measured values stabilized and no further changes were observed during the drying process. Final weights were then recorded. Additionally, we calculated the root-shoot ratio [root mass/(leaf mass + stem mass)] as well as tolerance index (TI) of both shoots and roots (which was calculated by dividing the shoot or root biomass of plants in each Cd treatment by that in treatments without Cd and NP) [48].
We employed two-way analysis of variance (ANOVAs) to investigate the effects of constant concentration treatment, N/P ratio, and their interactions on total mass, shoot mass, root mass, main stem length, number of nodes, root-shoot ratio and TI of shoot and root of A. philoxeroides. Differences among all treatments were assessed using the Duncan test. Prior to analysis, data were checked for normality and homoscedasticity and transformed as necessary to enhance homoscedasticity. All analyses were performed using SPSS 22.0 (IBM, Inc., Armonk, NY, USA).
3.1 Effects of Constant Concentration Treatment and N/P Ratios on the Plant Growth
Based on the results of the two-way ANOVA, constant concentration treatment significantly affected biomass (total biomass, shoot biomass, and root biomass) (Table 1). Both N/P ratio and the interaction between constant concentration treatment and N/P ratio significantly affected root biomass (Table 1). In comparison to maintaining the constant P concentration, the greater biomass was observed when maintaining the constant N concentration (Fig. 2). Under conditions of constant N concentration, root mass decreased with increasing N/P ratio (Fig. 2C). However, no significant differences in root mass were observed among the four different N/P ratios when maintaining the constant P concentration (Fig. 2C).
Figure 2: Effects of different constant concentration treatments and N/P ratios on total mass (A), shoot mass (B), and root mass (C) of Alternanthera philoxeroides. Bars and vertical lines are means and SE. Different small letters above the bars indicate significant differences among the treatments
Based on the Duncan test results, biomass in all treatments with both Cd, and N and P was significantly reduced compared to the treatment without both Cd and N and P, irrespective of constant N concentration or constant P concentration, as well as the N/P ratio (Fig. 2). Addition of nutrients did not have a significant impact on total mass or shoot mass when compared to the treatment with Cd but without N and P (Fig. 2A,B). However, under Cd stress, A. philoxeroides exhibited a significant reduction in root mass at an N/P ratio of 16:1 to 64:1 when N concentration was constant and at all N/P ratios when P concentration was constant (Fig. 2C).
3.2 Effects of Constant Concentration Treatment and N/P Ratios on the Plant Morphology
Based on the results of the ANOVA, constant concentration treatment, N/P ratio and their interaction significantly affected main stem length, number of nodes and root-shoot ratio (Table 2). Main stem length, number of nodes and root-shoot ratio were overall greater when concentration was constant than that when P concentration was constant (Fig. 3). When N concentration was maintained constant, main stem length, number of nodes and root-shoot ratio reached their highest values at a N/P ratio of 4:1 (Fig. 3). However, when P concentration was constant, these measurements did not differ significantly among the four N/P ratios (Fig. 3).
Figure 3: Effects of different constant concentration treatments and N/P ratios on main stem length (A), number of nodes (B), and root-shoot ratio (C) of Alternanthera philoxeroides. Bars and vertical lines are means and SE. Different small letters above the bars indicate significant differences among the treatments
According to the Duncan test, compared to the treatment without Cd, N and P, the treatments with Cd showed significant reductions in main stem length, number of nodes, and root-shoot ratio (Fig. 3), except for the N/P ratio of 4:1 when N concentration was constant which did not significantly affect the number of nodes (Fig. 3B). In comparison to the treatment with Cd but lacking N and P, an increase in main stem length and number of nodes was observed at a 4:1 N/P ratio when N concentration was constant; however, no significant changes were observed at ratios of 16:1, 32:1, and 64:1 when N was constant or any N/P ratios when P was constant (Fig. 3A,B). In addition, root-shoot ratio in all treatments with Cd and NP, except for the 4:1 N/P ratio under constant N conditions, exhibited lower values compared to the treatment with Cd alone (Fig. 3C).
3.3 Effects of Constant Concentration Treatment and N/P Ratios on the Tolerance Index
The results of the two-way ANOVA showed that constant concentration treatment and N/P ratio significantly affected the both shoot TI and root TI (Table 3). When N concentration was constant, both shoot TI and root TI were higher compared to when P concentration was constant (Fig. 4). The interaction between constant concentration treatment and N/P ratio significantly affected root TI (Table 3). Specifically, when N concentration was constant, both shoot TI and root TI were tended to decrease with increasing N/P ratio (Fig. 4). However, no differences in shoot TI or root TI were observed among the four N/P ratios when P concentration was maintained constant (Fig. 4).
Figure 4: Effects of different constant concentration treatments and N/P ratios on shoot tolerance index (A) and root tolerance index (B) of Alternanthera philoxeroides. Bars and vertical lines are means and SE. Different small letters above the bars indicate significant differences among the treatments
The results of the Duncan test revealed no significant difference in shoot TI between treatments with Cd and various N/P ratios compared to the treatment with Cd alone (Fig. 4A). However, the root TI was significantly lower in treatments containing N and P (except for the treatment at 4:1 N/P ratio when N concentration was constant) compared to the treatment without N and P (Fig. 4B).
4.1 Response of Plants Growth to Constant Concentration Treatment and N/P Ratios
The presence of Cd inhibited the overall growth of A. philoxeroides due to reduced absorption and transport of nutrients and water, increased oxidative damage, disrupted plant metabolism, and impaired plant morphology and physiology [49–51]. Previous studies have reported inconsistent effects of nutrients on plant growth under Cd stress [23–26,52]. For instance, some research has shown that higher nutrient levels alleviate the inhibitory effect of Cd on plant growth [23–24,52]. However, Zhang et al. [25] found no significant effect on plant growth under Cd stress with high nutrient levels. Furthermore, Li et al. [26] reported that increased nutrient availability exacerbated the inhibitory effects of Cd on plant growth. In this study, the addition of N and P did not significantly alleviate the inhibitory effects of Cd, regardless of whether the concentration of N or P was constant or their ratios. Although total nutrient concentrations were similar in these studies, variations in specific experimental conditions suggest potential explanations for divergent results. Additionally, the complexity of environmental changes faced by natural aquatic ecosystems poses further challenges in estimating these effects. This serves as a reminder that although the results of existing greenhouse simulation experiments can offer some guidance for controlling and managing invasive plants in heavy metal polluted and eutrophic water bodies, additional experimental findings with diverse designs are still necessary as a basis.
4.2 Response of Plants Morphology to Constant Concentration Treatment and N/P Ratios
The presence of Cd significantly reduced the main stem length and number of nodes, consistent with previous studies indicating the inhibitory effect of Cd on A. philoxeroides elongation and asexual reproductive capacity [23–26]. The root-shoot ratio was significantly decreased in the presence of Cd, suggesting an escape strategy to alleviate its toxicity [24,25]. Under Cd stress, the morphological performance was related to either N concentration or P concentration was constant. Compared to the treatment of P concentration constant, the morphology of A. philoxeroides exhibits greater sensitivity to different N/P ratios when N concentration was constant. Specifically, within the concentration range set in this experiment, the morphology of A. philoxeroides was more susceptible to variations in P concentration compared to N concentration under Cd stress. Additionally, in contrast to the Cd treatment without the addition of N and P, the main stem length and number of nodes significantly increased under Cd stress with N and P addition, particularly when maintaining a constant N concentration at an N/P ratio of 4:1. Moreover, the root-shoot ratio remained consistent with that observed under conditions of N and P addition with presence of Cd exposure. Thus, this specific combination of N and P concentrations appears to promote clonal offspring expansion and generation in A. philoxeroides inhabiting Cd polluted water while simultaneously mitigating Cd induced inhibition on root growth.
4.3 Response of Plants Tolerance Index to Constant Concentration Treatment and N/P Ratios
The shoot TI and root TI exhibited similar responses to constant concentration and N/P ratio, with both measurements showing relatively higher values at a constant N concentration of 2 mmol L−1 and tending to decrease as the N/P ratio increased under constant N concentration. Compared to a constant phosphorus concentration, the root TI displayed a stronger response to the N/P ratio when the N concentration was remained constant. Furthermore, there were no significant changes observed in shoot TI to Cd when comparing treatments with or without N and P under constant N concentration, constant P concentration, or varying N/P ratios. However, for root TI, significant negative effects were observed at N/P ratios of 16:1–64:1 under constant N concentration and all tested N/P ratios under constant P concentration. Root TI just matched that of the treatment without N and P when using an N/P ratio of 4:1 under constant N conditions; thus, adding N and P could not enhance A. philoxeroides’ tolerance to Cd. In fact, providing nutrients at specific N/P ratios may actually diminish root tolerance [53]. This phenomenon could be attributed to exceeding a certain threshold of the N/P ratio becoming harmful for plants [31,54]. It is worth to note that this study represents a preliminary investigation into the effect of the N/P ratios. We focused on examining the effects within the range of 4:1 to 64:1 and under certain concentration conditions. However, the natural environment is considerably more complex, which may introduce certain limitations in the generalizability of our findings. Nevertheless, this study demonstrates the significant influence of the N/P ratio on the growth of A. philoxeroides in Cd polluted water. This provides a valuable scientific basis for managing and predicting the spread of this invasive species in similar environments.
Although the effects of N and P nutrients on invasive plants in polluted freshwater have been well studied, whether their effects are influenced by the N/P ratio remains underexplored. This study addresses this research gap, and the results showed that the addition of N and P in any treatment did not alleviate the inhibitory effect of Cd on the growth of A. philoxeroides. Moreover, neither shoot TI nor root TI improves with the addition of N and P; instead, the root TI even decreases (except for the N/P ratio of 4:1 under N concentration constant condition). However, it is worth noting that compared to Cd treatment without N and P, the N/P ratio of 4:1 under a constant N concentration condition significantly enhances both the main stem length and the number of nodes in A. philoxeroides under Cd stress, potentially facilitating its expansion and clonal growth. These findings establish a theoretical foundation for the management and control of A. philoxeroides, an invasive plant species, in freshwater ecosystems characterized by Cd pollution and eutrophic water conditions. Furthermore, they highlight the complexity of nutrient impacts on invasive plant responses to Cd stress. Considering the limited range of nutrient concentrations and N/P ratios examined in this experiment, the application of the research findings may be constrained. Therefore, future experimental designs should include a broader spectrum of conditions and account for additional environmental factors to provide more accurate guidance for managing and controlling invasive plants in freshwater ecosystems.
Acknowledgement: Not applicable.
Funding Statement: This study was supported by the National Natural Science Foundation of China (Grant No. 32101264), the Science and Technology Research and Development Project of Handan (Grant 23422304086) and the National College Student Innovation Training Project of China (Grant No. 202410103013).
Author Contributions: Rui Zhang: Methodology, Formal Analysis, Writing—Original Draft, Writing—Review & Editing. Le Ren: Methodology, Formal Analysis, Writing—Original Draft, Writing—Review & Editing. Zhihang Liu: Methodology, Formal Analysis, Writing—Original Draft. Xinyue Gu: Investigation, Writing—Original Draft. Shanshan Du: Investigation, Writing—Review & Editing. Xiao Chen: Investigation, Writing—Review & Editing. Bing-Ran Xi: Investigation, Writing—Review & Editing. Bingnan Zhao: Investigation, Writing—Review & Editing. Chao Si: Conceptualization, Formal Analysis, Writing—Review & Editing, Supervision. All authors reviewed the results and approved the final version of the manuscript.
Availability of Data and Materials: The data that support the findings of this study are available from the corresponding author, Chao Si, upon reasonable request.
Ethics Approval: Not applicable.
Conflicts of Interest: The authors declare no conflicts of interests to report regarding the present study.
References
1. Mariyanto M, Amir MF, Utama W, Hamdan AM, Bijaksana S, Pratama A et al. Heavy metal contents and magnetic properties of surface sediments in volcanic and tropical environment from Brantas River, Jawa Timur Province, Indonesia. Sci Total Environ. 2019;675(17):632–41. doi:10.1016/j.scitotenv.2019.04.244. [Google Scholar] [PubMed] [CrossRef]
2. Hamdan AM, Kirana KH, Hakim F, Iksan M, Bijaksana S, Mariyanto M, et al. Magnetic susceptibilities of surface sediments from estuary rivers in volcanic regions. Environ Monit Assess. 2022;194(4):239. doi:10.1007/s10661-022-09891-z. [Google Scholar] [PubMed] [CrossRef]
3. Briffa J, Sinagra E, Blundell R. Heavy metal pollution in the environment and their toxicological effects on humans. Heliyon. 2020;6(9):e04691. doi:10.1016/j.heliyon.2020.e04691. [Google Scholar] [PubMed] [CrossRef]
4. Singh V, Ahmed G, Vedika S, Kumar P, Chaturvedi SK, Rai SN, et al. Toxic heavy metal ions contamination in water and their sustainable reduction by eco-friendly methods: isotherms, thermodynamics and kinetics study. Sci Rep. 2024;14(1):7595. doi:10.1038/s41598-024-58061-3. [Google Scholar] [PubMed] [CrossRef]
5. Mohammed AS, Kapri A, Goel R. Heavy metal pollution: source, impact, and remedies. In: Biomanagement of metal-contaminated soils. Dordrecht: Springer; 2011. p. 1–28. [Google Scholar]
6. Hu H, Jin Q, Kavan P. A study of heavy metal pollution in China: current status, pollution-control policies and countermeasures. Sustainability. 2014;6(9):5820–38. doi:10.3390/su6095820. [Google Scholar] [CrossRef]
7. Mansoor S, Kour N, Manhas S, Zahid S, Wani OA, Sharma V, et al. Biochar as a tool for effective management of drought and heavy metal toxicity. Chemosphere. 2021;271(4):129458. doi:10.1016/j.chemosphere.2020.129458. [Google Scholar] [PubMed] [CrossRef]
8. Ghuge SA, Nikalje GC, Kadam US, Suprasanna P, Hong JC. Comprehensive mechanisms of heavy metal toxicity in plants, detoxification, and remediation. J Hazard Mater. 2023;450(3):131039. doi:10.1016/j.jhazmat.2023.131039. [Google Scholar] [PubMed] [CrossRef]
9. Khan MN, Mohammad F. Eutrophication: challenges and solutions. In: Eutrophication: causes, consequences and control. Vol. 2. Dordrecht: Springer; 2014. p. 1–15. [Google Scholar]
10. Bonsdorff E. Eutrophication: early warning signals, ecosystem-level and societal responses, and ways forward. Ambio. 2021;50(4):753–8. doi:10.1007/s13280-020-01432-7. [Google Scholar] [PubMed] [CrossRef]
11. Maúre EDR, Terauchi G, Ishizaka J, Clinton N, DeWitt M. Globally consistent assessment of coastal eutrophication. Nat Commun. 2021;12(1):6142. doi:10.1038/s41467-021-26391-9. [Google Scholar] [PubMed] [CrossRef]
12. Akinnawo SO. Eutrophication: causes, consequences, physical, chemical and biological techniques for mitigation strategies. Environ Chall. 2023;12:100733. doi:10.1016/j.envc.2023.100733. [Google Scholar] [CrossRef]
13. Gagneten AM. Effects of contamination by heavy metals and eutrophication on zooplankton, and their possible effects on the trophic webs of freshwater aquatic ecosystems. In: Eutrophication: causes, consequences and control. Dordrecht: Springer; 2011. p. 211–23. [Google Scholar]
14. Guo C, Chen Y, Xia W, Qu X, Yuan H, Xie S, et al. Eutrophication and heavy metal pollution patterns in the water suppling lakes of China’s south-to-north water diversion project. Sci Total Environ. 2020;711(20):134543. doi:10.1016/j.scitotenv.2019.134543. [Google Scholar] [PubMed] [CrossRef]
15. Li CM, Wang HC, Liao X, Liao XL, Liu KH, Bai JH, et al. Heavy metal pollution in coastal wetlands: a systematic review of studies globally over the past three decades. J Hazard Mater. 2022;424:127312. doi:10.1016/j.jhazmat.2021.127312. [Google Scholar] [CrossRef]
16. Sharma M, Kant R, Sharma AK, Sharma AK. Exploring the impact of heavy metals toxicity in the aquatic ecosystem. Int J Energy Water Resour. 2024;168:1–14. doi:10.1007/s42108-024-00284-1. [Google Scholar] [CrossRef]
17. Thomaz SM, Kovalenko KE, Havel JE, Kats LB. Aquatic invasive species: general trends in the literature and introduction to the special issue. Hydrobiologia. 2015;746(1):1–12. doi:10.1007/s10750-014-2150-8. [Google Scholar] [CrossRef]
18. Petruzzella A, da Rodrigues TA, van Leeuwen CH, de Assis Esteves F, Figueiredo-Barros MP, Bakker ES. Species identity and diversity effects on invasion resistance of tropical freshwater plant communities. Sci Rep. 2020;10:5626. doi:10.1038/s41598-020-62660-1. [Google Scholar] [PubMed] [CrossRef]
19. Qi GP, Li LX, Li HY, Liu Y, Xie TW, Guo HQ, et al. Ecological effects of the huge invasive species removal project in coastal China. Environ Sci Technol. 2024;58:21229–41. doi:10.1021/acs.est.4c05253. [Google Scholar] [PubMed] [CrossRef]
20. MacDougall AS, Gilbert B, Levine JM. Plant invasions and the niche. J Ecol. 2009;97:609–15. doi:10.1111/j.1365-2745.2009.01514.x. [Google Scholar] [CrossRef]
21. Afreen T, Kumari S, Bhadouria R, Devi RS, Singh S, Tripathi S. Plant invasion and soil processes: a mechanistic understanding. In: Plant invasions and global climate change. Singapore: Springer Nature Singapore; 2023. p. 227–46. [Google Scholar]
22. Matzek V. Superior performance and nutrient-use efficiency of invasive plants over non-invasive congeners in a resource-limited environment. Biol Invasions. 2011;13:3005–14. doi:10.1007/s10530-011-9985-y. [Google Scholar] [CrossRef]
23. Wang XG, Zhang RXG, Qian ZH, Qiu SY, He XG, Wang SJ, et al. Effects of cadmium and nutrients on the growth of the invasive plant Alternanthera philoxeroides. Folia Geobot. 2022;57:259–67. doi:10.1007/s12224-022-09423-0. [Google Scholar] [CrossRef]
24. Zhang R, Chen ZH, Cui WT, Qiu SY, Qian ZH, He XG, et al. Cadmium stress interacts with nutrient availability and light condition to affect the growth of Hydrocotyle vulgaris. PLoS One. 2023a;18:e0280449. doi:10.1371/journal.pone.0280449. [Google Scholar] [PubMed] [CrossRef]
25. Zhang R, Chen ZH, Lyu WY, Hu YF, Yang JX, Xin JC, et al. Effects of fragmentation, cadmium, and nutrients on the growth of a wetland invasive plant Alternanthera philoxeroides. Plant Species Biol. 2023b;38:180–91. doi:10.1111/1442-1984.12405. [Google Scholar] [CrossRef]
26. Li D, Wang XG, Yang JX, Hu YF, Lyu WY, Xin JC, et al. Cadmium interacts with nitrogen level and nitrogen form to affect the growth of an invasive plant Alternanthera philoxeroides. Aquat Ecol. 2024;58:289–97. doi:10.1007/s10452-023-10065-3. [Google Scholar] [CrossRef]
27. Adomako MO, Alpert P, Du DL, Yu FH. Effects of clonal integration, nutrients and cadmium on growth of the aquatic macrophyte Pistia stratiotes. J Plant Ecol. 2020;13:765–72. [Google Scholar]
28. Wang KT, Wu YC, Qu C, Liu M, Liu XR, Li H, et al. Effects of the combined regulation of nitrogen, phosphorus, and potassium nutrients on the migration and transformation of arsenic species in paddy soil. Ecotoxicol Environ Saf. 2024;282:116745. doi:10.1016/j.ecoenv.2024.116745. [Google Scholar] [PubMed] [CrossRef]
29. Güsewell S. N: P ratios in terrestrial plants: variation and functional significance. New Phytol. 2004;164:243–66. doi:10.1111/j.1469-8137.2004.01192.x. [Google Scholar] [PubMed] [CrossRef]
30. Garrish V, Cernusak LA, Winter K, Turner BL. Nitrogen to phosphorus ratio of plant biomass versus soil solution in a tropical pioneer tree, Ficus insipida. J Exp Bot. 2010;61:3735–48. doi:10.1093/jxb/erq183. [Google Scholar] [PubMed] [CrossRef]
31. Guignard MS, Leitch AR, Acquisti C, Eizaguirre C, Elser JJ, Hessen DO, et al. Impacts of nitrogen and phosphorus: from genomes to natural ecosystems and agriculture. Front Ecol Evol. 2017;5:70. doi:10.3389/fevo.2017.00070. [Google Scholar] [CrossRef]
32. Gong H, Li Y, Yu T, Zhang S, Gao J, Zhang S, et al. Soil and climate effects on leaf nitrogen and phosphorus stoichiometry along elevational gradients. Global Ecol Conserv. 2020;23:e01138. doi:10.1016/j.gecco.2020.e01138. [Google Scholar] [CrossRef]
33. Luo X, Mazer SJ, Guo H, Zhang N, Weiner J, Hu SJ. Nitrogen: phosphorous supply ratio and allometry in five alpine plant species. Ecol Evol. 2016;6:8881–92. doi:10.1002/ece3.2587. [Google Scholar] [PubMed] [CrossRef]
34. Razaq M, Zhang P, Shen HL, Salahuddin. Influence of nitrogen and phosphorous on the growth and root morphology of Acer mono. PLoS One. 2017;12:e0171321. doi:10.1371/journal.pone.0171321. [Google Scholar] [PubMed] [CrossRef]
35. Grunes DL. Effect of nitrogen on the availability of soil and fertilizer phosphorus to plants. Adv Agron. 1959;11:369–96. [Google Scholar]
36. Liu JT, Wang DN, Yan XL, Jia LM, Chen N, Liu JJ, et al. Effect of nitrogen, phosphorus and potassium fertilization management on soil properties and leaf traits and yield of Sapindus mukorossi. Front Plant Sci. 2024;15:1300683. doi:10.3389/fpls.2024.1300683. [Google Scholar] [PubMed] [CrossRef]
37. Cao Y, Tong R, Tan Q, Mo S, Ma C, Chen G. Flooding influences on the C, N and P stoichiometry in terrestrial ecosystems: a meta-analysis. Catena. 2022;215:106287. doi:10.1016/j.catena.2022.106287. [Google Scholar] [CrossRef]
38. Sousa Junior GS, Hurtado AC, Souza Junior JP, Prado RM, Piccolo MC, Dos Santos DMM. Beneficial role of silicon on regulating C, N, and P stoichiometric homeostasis and the growth of sugarcane seedlings under aluminum toxicity. J Soil Sci Plant Nutr. 2022;22:4138–52. doi:10.1007/s42729-022-01013-5. [Google Scholar] [CrossRef]
39. Olivera-Viciedo D, Aguilar DS, Prado RM, Calzada KP, Hurtado AC, Piccolo MC, et al. Silicon-mediated adjustments in C: N: P ratios for improved beetroot yield under ammonium-induced stress. Agronomy. 2024;14:1104. doi:10.3390/agronomy14061104. [Google Scholar] [CrossRef]
40. Zhang J, Shoaib N, Lin KX, Mughal N, Wu XG, Sun XM, et al. Boosting cadmium tolerance in Phoebe zhennan: the synergistic effects of exogenous nitrogen and phosphorus treatments promoting antioxidant defense and root development. Front Plant Sci. 2024;15:1340287. doi:10.3389/fpls.2024.1340287. [Google Scholar] [PubMed] [CrossRef]
41. Tanveer A, Ali HH, Manalil S, Raza A, Chauhan BS. Eco-biology and management of alligator weed [Alternanthera philoxeroides (Mart.) Griseb.]: a review. Wetlands. 2018;38:1067–79. doi:10.1007/s13157-018-1062-1. [Google Scholar] [CrossRef]
42. Ye WH, Li J, Cao HL, Ge XJ. Genetic uniformity of Alternanthera philoxeroides in South China. Weed Res. 2003;43:297–302. doi:10.1046/j.1365-3180.2003.00346.x. [Google Scholar] [CrossRef]
43. Shi MZ, Fu JW. Alligator weed Alternanthera philoxeroides (Mart.) Griseb. In: Wan F, Jiang M, Zhan A, editors. Biological invasions and its management in China. invading nature-springer series in invasion ecology. Vol. 13. Singapore: Springer; 2017. [Google Scholar]
44. Yan HY, Feng L, Zhao YF, Feng L, Wu D, Zhu CP. Prediction of the spatial distribution of Alternanthera philoxeroides in China based on ArcGIS and MaxEnt. Global Ecol Conserv. 2020;21:e00856. doi:10.1016/j.gecco.2019.e00856. [Google Scholar] [CrossRef]
45. Julien MH, Skarratt B, Maywald GF. Potential geographical distribution of alligator weed and its biological control by Agasicles hygrophila. J Aquat Plant Manage. 1995;33:55–60. [Google Scholar]
46. Shen GJ, Xu ZH, Yu GS. Distribution and damage of alligator weed, Alternanthera philoxeroides and its control. Plant Prot. 2005;31:14–8. [Google Scholar]
47. Weber E, Sun SG, Li B. Invasive alien plants in China: diversity and ecological insights. Biol Invasions. 2008;10:1411–29. doi:10.1007/s10530-008-9216-3. [Google Scholar] [CrossRef]
48. Metwally A, Safronova VI, Belimov AA, Dietz KJ. Genotypic variation of the response to cadmium toxicity in Pisum sativum L. J Exp Bot. 2005;56:167–78. doi:10.1093/jxb/eri017. [Google Scholar] [PubMed] [CrossRef]
49. Haider FU, Liqun C, Coulter JA, Cheema SA, Wu J, Zhang R, et al. Cadmium toxicity in plants: impacts and remediation strategies. Ecotoxicol Environ Saf. 2021;211:111887. doi:10.1016/j.ecoenv.2020.111887. [Google Scholar] [PubMed] [CrossRef]
50. Aslam MM, Okal EJ, Waseem M. Cadmium toxicity impacts plant growth and plant remediation strategies. Plant Growth Regul. 2023;99:397–412. doi:10.1007/s10725-022-00917-7. [Google Scholar] [CrossRef]
51. Kumar R, Kumar V, Tandon V, Kumar S, Roohi. Effect and responses of cadmium in plants. In: Cadmium toxicity in water: challenges and solutions. Cham: Springer Nature Switzerland; 2024. p. 327–47. [Google Scholar]
52. Adomako MO, Zhang Q, Yu FH. Genotypic differences in response to different patterns of clonal fragmentation in the aquatic macrophyte Pistia stratiotes. J Plant Ecol. 2022;15:1199–212. doi:10.1093/jpe/rtac035. [Google Scholar] [CrossRef]
53. Zhang CY, Zhang ZK, Zhou JH, Wang Y, Ai YM, Li XP, et al. Responses of the root morphology and photosynthetic pigments of ryegrass to fertilizer application under combined petroleum-heavy metal stress. Environ Sci Pollut Res. 2022;29:87874–83. doi:10.1007/s11356-022-21924-1. [Google Scholar] [PubMed] [CrossRef]
54. Zhang L, Hoshika Y, Carrari E, Cotrozzi L, Pellegrini E, Paoletti E. Effects of nitrogen and phosphorus imbalance on photosynthetic traits of poplar Oxford clone under ozone pollution. J Plant Res. 2018;131:915–24. doi:10.1007/s10265-018-1071-4. [Google Scholar] [PubMed] [CrossRef]
Cite This Article
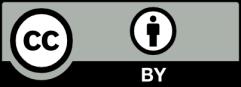
This work is licensed under a Creative Commons Attribution 4.0 International License , which permits unrestricted use, distribution, and reproduction in any medium, provided the original work is properly cited.