Open Access
ARTICLE
Research on the Cymbidium tortisepalum var. longibracteatum Growth and Non-Tube Rapid Propagation Based on Response Surface Methodology
College of Life Science and Engineering, Southwest University of Science and Technology, Mianyang, 621000, China
* Corresponding Author: Jie Li. Email:
(This article belongs to the Special Issue: Optimization of Propagation Techniques and Improvement of the Production and Quality of Red Fruit)
Phyton-International Journal of Experimental Botany 2025, 94(3), 953-971. https://doi.org/10.32604/phyton.2025.060634
Received 06 November 2024; Accepted 08 February 2025; Issue published 31 March 2025
Abstract
The objective of this study was to determine the optimal proportions of plant growth regulators for growth and non-tube rapid propagation of Cymbidium tortisepalum var. longibracteatum; seedlings were utilized as the material. The effects of various combinations and concentrations of 6-benzylaminopurine (6-BA), gibberellic acid (GA3), and naphthaleneacetic acid (NAA) on growth and non-tube rapid propagation were assessed through a single-factor testing and response surface methodology. The results indicated that 6-BA at 60 mg/L, GA3 at 150 mg/L, and NAA at 30 mg/L were the most effective concentrations for promoting leaf buds formation in the single-factor analysis. Response surface methodology clarified the sensitivity of the proliferation rate of lateral buds to the three factors, with 6-BA being the most influential, followed by GA3 and NAA. The increase in leaf area was most significantly influenced by NAA, then GA3, and least by 6-BA, while the increase in plant height was most responsive to GA3, followed by 6-BA, and then NAA. The ideal concentrations of plant growth regulators were established as 6-BA at 43 mg/L, GA3 at 169 mg/L, and NAA at 36 mg/L. Under these conditions, the lateral bud number per plant was 2.78, with a leaf area increment of 2.87 cm2 and a plant height increment of 2.67 cm.Keywords
The Orchidaceae is the most species-rich among flowering plants, with over 28,000 species exhibiting diverse breeding characteristics [1]. Cymbidium tortisepalum var. longibracteatum (C. tortisepalum var. longibracteatum) is a species of Cymbidium genus and an economically significant perennial herbaceous plant prized for its high ornamental value, which is derived from its foliage, flower color, shape, and fragrance. Historically, C. tortisepalum var. longibracteatum was abundant in rocky and scrubby slopes at altitudes between 1000 and 2000 m, but it has recently been subjected to threats from over-collection and habitat disturbance [1–5]. Consequently, it has been classified as an endangered (EN) species in China [2].
Traditional methods of reproduction, which rely on the division of multi-conjoined cluster seedlings, exhibit a low reproduction coefficient. Tissue culture techniques faced challenges such as high costs, contamination risks, and an inability to meet market demand. In contrast, non-tube rapid plant propagation methods provide a promising alternative for rapid propagation. It aims to stimulate the photosynthetic assimilation instinct of plants through environmental optimization techniques [6]. The approach endeavors to achieve autotrophic self-sufficiency and scientifically regulate the carbon sources, mineral nutrients, and hormones necessary for plant development, ultimately establishing a photoautotrophic rapid propagation system in an open environment [6]. This technique offers several advantages, including robust regenerated seedlings, stable phenotypes, shortened growth cycles, and high survival rates. By utilizing this method, growers can quickly produce a large number of propagates from a small number of elite plants in a short timeframe [6]. Rapid multiplication through non-tube rapid propagation could be an effective solution for mass-propagating plants. Despite the rich diversity of orchid species enriched in China, research on non-tube rapid propagation of Cymbidium is limited, with most studies focusing on Dendrobium [6].
Plant growth regulators are essential in various functional processes associated with plant growth and development. Research indicates that these regulators can significantly reduce production cycles and improve the tillering rate in Cymbidium goeringii, Cymbidium sinense, Cymbidium ensifolium, and Dendrobium nobile [7,8]. 6-Benzyl-adenine (6-BA), a synthetic cytokinin, is instrumental in cell division, bud formation, and callus induction during plant ontogeny [9–12]. Zheng’s research established that 6-BA within an optimal concentration range, stimulates plant proliferation and lateral bud differentiation in the hybrid protocorms of C. goeringii and Cymbidium hybridium [13]. Gibberellin (GA3) is integral to modulating various physiological processes in higher plants, encompassing stem elongation, leaf expansion, and the germination of dormant buds [14–17]. Chen discovered that the synergistic application of 6-BA, GA3, and KT at 60, 50, and 100 mg/L, respectively, can precipitate earlier bud sprouting in Cattleya hybrida [18]. Furthermore, 20–30 mg/L GA3 on C. ensifolium has been demonstrated to enhance the germination and growth of vegetative and floral buds [19]. Naphthalene-acetic acid (NAA) is pivotal for regulating cell elongation, division, proliferation, and root initiation, thereby playing a critical role in plant growth and development [20–22]. Li et al. demonstrated that the treatment of cuttings with 80–160 mg/L NAA significantly promoted shoot elongation and the rooting rate in Punica granatum while also markedly reducing the mortality rate [23].
The enduring market preference for traditional, high-quality seedling varieties, which command high prices, has rendered the rapid propagation of orchids a critical bottleneck in the development of the orchid industry. To address this issue, response surface methodology was employed to optimize the effects of plant growth regulators on the growth and non-tube rapid propagation of C. tortisepalum var. longibracteatum, establishing a foundation for their growth and non-tube rapid propagation and providing a reference methodology for research on other floral species [24].
The C. tortisepalum var. longibracteatum was planted in the Orchid Plant Resource Nursery of Southwest University of Science and Technology, Mianyang (31.5°N, 104.7°E). Uniformly sized, 2-year-old seedlings of C. tortisepalum var. longibracteatum, exhibiting robust growth and reaching a height of approximately 6–8 cm were selected for this experimental material. These seedlings were cultivated under natural conditions for 90 days before experimentation.
2.2.1 Single-Factor Experiment of Plant Growth Regulators on the Growth and Non-Tube Rapid Propagation of C. tortisepalum var. longibracteatum
The experimental treatment comprised varying concentrations of 6-BA (0, 20, 40, 60, and 80 mg/L), GA3 (0, 50, 100, 150, and 200 mg/L), and NAA (0, 10, 20, 30, and 40 mg/L), respectively. Each treatment was replicated three times. The seedlings’ pseudobulbs were irrigated with 10 mL of the respective treatment solution once weekly, resulting in a total of eight applications. The emergence of lateral buds was documented at 30, 60, and 90 d, while leaf length, leaf width, and plant height were measured at 90 d mark.
2.2.2 Response Surface Experiment of Plant Growth Regulators on the Growth and Non-Tube Rapid Propagation of C. tortisepalum var. longibracteatum
The experimental design employed was a response surface methodology with a Box-Benhken design, encompassing a total of 17 experimental runs, including four replicates at the center points. The variables selected for optimization were 6-BA, GA3, and NAA, coded as A, B, and C, respectively, with their range and levels detailed in Table 1. The sprouting was recorded at 30, 60, and 90 d, and leaf length, leaf width, and plant height were measured at 90 d.
Data analysis for the single-factor test was executed utilizing SPSS 26 software, and the corresponding data visualization was generated with Origin 2022. The analysis of the optimization experiment data was carried out employing Design-Expert 13.
Following a 60-day treatment with 6-BA, small green protrusions initiated emergence on a subset of pseudobulbs, with the peak incidence observed at a concentration of 60 mg/L (Fig. 1A). In contrast, no such protrusions were detected on pseudobulbs after a 60-day exposure to GA3 and NAA. These protrusions progressively matured into light green or white shoot tips (Fig. 1B–D). Within the treatment groups, lateral buds induced by GA3 exhibited a relatively slender morphology compared to those induced by 6-BA and NAA, despite their height surpassing that of the other treatment groups (Fig. 1C). In contrast, the lateral buds derived from NAA treatments were more robust than those from the other treatments (Fig. 1D).
Figure 1: Effects of 6-BA, GA3, and NAA on the growth of lateral bud sprouting in C. tortisepalum var. longibracteatum: 6-BA treatment (A, B); GA3 treatment (C); NAA treatment (D)
3.1.1 Effects of 6-BA on the Growth and Non-Tube Rapid Propagation of C. tortisepalum var. longibracteatum
6-BA is a typical cell division agent that predominantly stimulates stem and leaf development, bud proliferation, and cell division. After 90 d of experimental treatment, the data illustrated in Figs. 1A,B and 2. reveal that as the concentration of 6-BA increments, the number of lateral buds initially increases and subsequently decreases. When the 6-BA concentration was 60 mg/L, the number of lateral bud peaks at 3.29; conversely, at 80 mg/L, the number of lateral bud drops to a low of just 1.29. The trends in the line chart between 20 and 40 mg/L were relatively flat and not significantly different, but when the concentration of 6-BA concentration was 60 mg/L, there was a significant difference with other levels. Excessively high levels of 6-BA may inhibit lateral bud development while concurrently promoting overall plant growth. A concentration of 6-BA that is optimal and suitable for lateral bud development might suppress overall plant growth, while a concentration that favors plant growth could inhibit the formation of lateral buds.
Figure 2: Effect of 6-BA on C. tortisepalum var. longibracteatum growth and non-tube rapid propagation. A: the number of lateral bud, B: leaf area, C: plant height. Note: The data are expressed as mean ± standard deviation. The significance level is set to 0.05 (the same below)
The leaf area and plant height with the concentration of 6-BA increase. At a concentration of 6-BA which was 80 mg/L, significant differences in leaf area and plant height are observed compared to the 20, 60 mg/L, and the control group.
3.1.2 The Influence of GA3 on the Growth and Non-Tube Rapid Propagation of C. tortisepalum var. longibracteatum
GA3 is a widely utilized plant growth regulator that exerts a profound influence on various aspects of plant development, including stem and leaf growth, seedling development, and the formation of lateral buds. As shown in Figs. 1C and 3, GA3 significantly influences the formation of lateral buds. The highest number of lateral buds (1.92) was observed at a GA3 concentration of 150 mg/L, with the new buds exhibiting a slender morphology (Fig. 1C). In contrast, at the lowest concentration of 50 mg/L only 1.25 lateral buds were noted. This trend indicates that increasing GA3 concentration generally promotes lateral bud formation. However, it is important to recognize that excessively high concentrations of GA3 (>150 mg/L) may inhibit lateral bud formation.
Figure 3: Effect of GA3 on C. tortisepalum var. longibracteatum growth and non-tube rapid propagation. (A) The number of lateral bud, (B) leaf area, (C) plant height
The leaf area and plant height with the concentration of GA3 increases. When the GA3 concentration was 200 mg/L, it significantly differed from other levels, while the line chart from 0 to 150 mg/L displayed only minor changes. Overall, while GA3 promotes lateral bud formation, leaf area, and plant height up to a certain concentration, excessive levels may hinder these developments.
3.1.3 The Effect of NAA on the Growth and Non-Tube Rapid Propagation of C. tortisepalum var. longibracteatum
NAA is known to regulate plant elongation and promote cell division. As illustrated in Figs. 1D and 4, the highest number of lateral buds (2.2) was observed at an NAA concentration of 30 mg/L, while the lowest (1.33) was noted at 0 mg/L. This trend suggests that increasing NAA levels can effectively promote lateral bud germination. However, the germination of lateral buds was suppressed at concentrations higher than 30 mg/L.
Figure 4: Effect of NAA on C. tortisepalum var. longibracteatum growth and non-tube rapid propagation. (A) The number of lateral bud, (B) leaf area, (C) plant height
Regarding leaf area, NAA did not show significant differences across the tested concentrations. The exhibit shows a relatively gentle trend in the line graph of plant height between the NAA concentrations of 10 and 40 mg/L, with no significant differences observed. This suggests that while NAA positively influences lateral bud formation within a certain concentration range, its impact on leaf area and plant height may be limited.
The number of lateral buds (Y1), the relative growth rate of leaf area (Y2), and the relative growth rate of plant height (Y3) were the response variables, while 6-BA (A), GA3 (B), and NAA (C) served as the independent variables. The Design-Expert 13 software was employed to regress the response variables from Table 2 and the coded values for each component, resulting in the regression equation. As shown in Fig. 5A,B, a false bulb developed two white bud points at 30 d. These white bud points gradually changed color, and the young shoots grew to a height of 2 cm (Fig. 5C–F) at 60 d. Among all treatment groups, Group 1 exhibited the highest lateral bud sprouting rate, reaching 3.6, while Groups 3 and 13 recorded the lowest lateral bud sprouting rate, at only 1.67.
Figure 5: Effects of different combinations and concentrations of 6-BA, GA3 and NAA on bud proliferation of C. tortusisepalum var. longibracteatum. Germination of lateral buds in Group 1 at 30 days (A, B); germination of lateral buds in Group 1 at 60 days (C–E); and germination of lateral buds in Group 3 at 60 days (F)
3.2.1 Analysis of Response Surface for the Number of Lateral Bud
Y1 = 2.34 − 0.4625A + 0.2588B − 0.1788C − 0.300AB + 0.2750AC − 0.0675BC − 0.0218A2 − 0.1793B2 + 0.3758C2
The number of lateral buds ranged from 1.67 to 3.6. The three independent variables had important effects on the response (number of lateral buds) (Table 3). The value of the coefficient of determination (R2) was 0.9776; the proficiency of the model is demonstrated if R2 is equal to 0.75 or higher than this value [25]. The adjusted determination coefficient (adjusted R2) was found to be 0.9488. The regression equation model yielded a p value of less than 0.0001. Upon subjecting the equation to a lack of fit test, the results were F = 0.24 and p = 0.8621 > 0.05, indicating a non-significant lack of fit. This suggests that the regression equation aligns well with the actual experiment data, exhibiting minimal experimental errors, and can accurately describe the relationship between the various factors and response values. The quadratic coefficients of B2 and C2; A, B, and C were statistically the most significant factors. The cross-coefficient AB and AC were also significant. However, the regression coefficients of BC and A2 were not significant. The interaction between 6-BA and both GA3 and NAA significantly affected the quantity of lateral buds. In contrast, the interaction between GA3 and NAA did not significantly affect the quantity of lateral buds of the species.
The utilization of Design-Expert 13 software to generate contour plots and response surface plots (Fig. 6) provides a detailed analysis of the influence of various factors on the response values. The interaction among various factors and their impact on the number of lateral buds can be discerned through the morphology of the contour line and the curvature of the response surface. A steeper curvature of the response surface and a denser distribution of contour line indicate a more significant influence, while a shape that approaches an elliptical form suggests a stronger interaction between the two factors.
Figure 6: Response surface and contour map of the lateral buds under different culture conditions. (A) Response surface diagram of 6-BA and GA3 affecting the lateral buds. (B) Contour map of 6-BA and GA3 affecting the lateral buds. (C) Response surface diagram of 6-BA and NAA affecting the lateral buds. (D) Contour map of 6-BA and NAA affecting the lateral buds. (E) Response surface diagram of GA3 and NAA affecting the lateral buds. (F) Contour map of GA3 and NAA affecting the lateral buds
Fig. 6 shows a 2D contour plot and 3D response surface plots for the number of lateral buds. Fig. 6A and B demonstrates a significant interaction between 6-BA and GA3. When the concentration NAA was fixed at 30 mg/L and GA3 was maintained at a constant level, an increase in the concentration of 6-BA resulted in a corresponding increase in the number of lateral buds. At higher levels of GA3, the number of lateral buds increases rapidly with rising concentrations of 6-BA. In contrast, when GA3 was at lower levels, the number of lateral buds increased more gradually. Additionally, when lower concentrations of 6-BA were applied, the number of lateral bud increased slowly with rising concentrations of GA3. Conversely, at higher concentrations of 6-BA, the number of lateral buds rose rapidly as GA3 concentrations were increased.
As illustrated in Fig. 6C and D, the interaction between 6-BA and NAA was significant. When the concentration of GA3 was held constant at 150 mg/L and NAA was maintained at a constant level, the number of lateral buds increased with the concentration of 6-BA. However, at a higher level of NAA, the number of lateral buds roses rapidly with increasing concentrations of 6-BA. Conversely, when NAA was at a lower level, the growth of lateral buds was slower, and even with a constant 6-BA concentration, the number of lateral buds still increased with the concentration of NAA.
From Fig. 6E and F, the response surface curves for GA3 and NAA were relatively flat, suggesting that the interaction between GA3 and NAA was not significant, and their effects on the number of lateral buds were not significant.
In summary, the concentration of 6-BA had the most significant impact on the germination of lateral buds, followed by GA3, while NAA concentration had the least effect. Therefore, it can be inferred that the germination of lateral buds was relatively sensitive to variations in 6-BA concentration, with sensitivity levels ranked as 6-BA > GA3 > NAA.
3.2.2 Analysis of Response Surface Optimization for the Growth of Leaf Area
Y2 = 1.858 − 0.1725A + 0.1763B + 0.4738C + 0.0375AB – 0.1375AC + 0.0500BC + 0.0785A2 – 0.7140B2 + 0.0560C2
The three independent variables significantly influenced the response variable (leaf area). As indicated in Table 4, the p value of the regression equation model was less than 0.0001, suggesting a highly significant model fit. A lack-of-fit test was conducted on the equation, and the results revealed that the lack-of-fit term was not significant (F = 3.34, p = 0.1371 > 0.05), indicating that the model adequately describes the experimental data. The coefficient of determination (R2) was 0.9862, and the adjusted determination coefficient (adjusted R2) was 0.9688, both of which imply that the regression equation closely aligns with the actual experiment data, exhibiting minimal experimental errors and accurately capturing the relationship between the various factors and response values. The quadratic coefficients of B2, A, B, and C were statistically the most significant factors. Additionally, the cross-coefficient AC was also significant. Conversely, the following factors were statistically insignificant: the quadratic coefficient of (A2, C2) and the cross-coefficient (AB, BC). The results demonstrated that the interaction between 6-BA and NAA significantly affected the growth rate of leaf area. However, the interactions between GA3 and NAA, and between GA3 and 6-BA, did not exert a significant effect on the growth rate of the leaf area in this species.
The results of the 2D contour plot and 3D response surface plots are presented in Fig. 7. Fig. 7A,C demonstrates that the response surface for 6-BA was relatively flat. When the concentration of 6-BA exceeded 60 mg/L, it had minimal effect on the increase in leaf area. In contrast, the response surface curve for GA3 (Fig. 7A,E) was parabolic and steeper. When the NAA concentration was 30 mg/L and 6-BA concentration remained constant, with increasing GA3 concentration leaf area first increased and then decreased. When GA3 exceeds 175 mg/L, the curve flattens, and the leaf area decreases with increasing concentration. This pattern indicates that the leaf area dependent on a specific range of GA3 concentration.
Figure 7: Response surface and contour map of the leaf area increment under different culture conditions. (A) Response surface diagram of 6-BA and GA3 affecting the leaf area increment. (B) Contour map of 6-BA and GA3 affecting the leaf area increment. (C) Response surface diagram of 6-BA and NAA affecting the leaf area increment. (D) Contour map of 6-BA and NAA affecting the leaf area increment. (E) Response surface diagram of GA3 and NAA affecting the leaf area increment. (F) Contour map of GA3 and NAA affecting the leaf area increment
Fig. 7C and E highlights that the response curves for NAA were steep and linear, signifying a substantial influence on leaf area growth. In Fig. 7C and D, the interaction between 6-BA and NAA was pronounced, as evidenced by dense contour lines. When the GA3 concentration was 150 mg/L and the 6-BA concentration remained constant, the increase in leaf area corresponded with the concentration of NAA. However, at high levels of 6-BA, the increase in leaf area was slow. Conversely, when 6-BA was at a low level, the leaf area increment rapidly increased with rising NAA concentrations.
In summary, the concentration of NAA had the largest effect impact on the increase in leaf area, followed by GA3, while the influence of 6-BA concentration was the least. Therefore, it can be concluded that the leaf area growth was relatively sensitive to changes in NAA concentration, with sensitivity levels ranked as follows: NAA > GA3 > 6-BA.
3.2.3 Analysis of Response Surface Optimization for the Growth of Plant Height
Y3 = 2.98 + 0.1325A + 0.4338B − 0.0063C – 0.0400AB – 0.0200AC + 0.1875BC – 0.5225A2 – 1.0128B2 – 0.6528C2
The regression equation model (Table 5) had a p value of less than 0.0001, indicating significance and suggesting that the model was appropriate for this experiment. The lack-of-fit term test was used to analyze the equation, yielding F = 0.24, p = 0.8669 > 0.05, which indicates a non-significant lack-of-fit term, suggesting that the model fits the test well. Among them, the coefficient of determination (R2) was 0.9851, and the adjusted determination coefficient (adjusted R2) was found to be 0.9659. These values indicate that the regression closely aligns with the actual experiment data, exhibiting minimal experimental errors and accurately describing the relationship between the various factors and response values. The quadratic coefficients of A2, B2, and C2 were also significant. The cross-coefficient BC, A, and B displayed statistically significant factors. While C, AB, and AC had non-significant regression coefficients. The results demonstrated that the interaction of GA3 with NAA significantly affected the relative growth rate of plant height. However, the interaction between 6-BA and NAA, GA3, did not significantly affect the relative growth rate of plant height in this species.
The response surface plots and contour maps, which depict interactions among various factors through fitting equations, typically display a hilly curvature, indicating the presence of a maximum within the selected ranges of each factor. As illustrated in Fig. 8A,C, and E, the response curve of GA3 exhibits a parabolic trend and is relatively steep, while the response curves for 6-BA and NAA are comparatively flat. This indicates that the concentration of GA3 significantly influences the plant height, followed by 6-BA and NAA. When the NAA concentration was 30 mg/L and 6-BA remained constant, as GA3 concentration increased, plant height initially rose and then decreased (Fig. 8A). When GA3 was at 150 mg/L, the increase in plant height was the greatest. While concentrations exceeding 175 mg/L lead to a flattening of the response curve and a reduction in height, indicating that excessively high concentrations inhibit growth.
Figure 8: Response surface and contour map of the plant height increment under different culture conditions. (A) Response surface diagram of 6-BA and GA3 affecting the plant height increment. (B) Contour map of 6-BA and GA3 affecting the plant height increment. (C) Response surface diagram of 6-BA and NAA affecting the plant height increment. (D) Contour map of 6-BA and NAA affecting the plant height increment. (E) Response surface diagram of GA3 and NAA affecting the plant height increment. (F) Contour map of GA3 and NAA affecting the plant height increment
As shown in Fig. 8A–D, it is clearly shown that the interactions between 6-BA and GA3, and between 6-BA and NAA, were not significant. The projections of the contour maps predominantly appear circular or nearly circular, which aligns with the results of the variance analysis. In contrast, the projections in Fig. 8E and F exhibit an elliptical shape with a parabolic and steep response curve, indicating a significant interaction between GA3 and NAA, consistent with the variance analysis findings.
In summary, the concentration of GA3 had the most pronounced effect on plant height, followed by 6-BA and NAA. Therefore, it can be concluded that plant height growth is relatively sensitive to changes in GA3 concentration, with the sensitivity levels ranked as follows: GA3 > 6-BA > NAA.
3.2.4 Optimal Ratio of Plant Growth Regulators and Verification
The analysis conducted using Design-Expert 13 software determined that the optimal conditions for enhancing the number of lateral buds, leaf area growth, and plant height growth were as follows: 6-BA at 43.34 mg/L, GA3 at 168.55 mg/L, and NAA at 35.68 mg/L. The predicted optimal value for lateral buds was 2.75 buds per plant, and the leaf area growth was 2.38 cm2, while the plant height was 2.38 cm. For practical application, adjustments were made based on actual conditions, setting 6-BA to 43 mg/L, GA3 to 169 mg/L, and NAA to 36 mg/L for three parallel experiments. The results yielded an average of 2.78 lateral buds, 2.87 cm2 for leaf area growth, and 2.67 cm for plant height growth, which were generally consistent with the theoretical predictions. This indicates that the model is reliable and can be utilized for optimizing the growth of C. tortisepalum var. longibracteatum and its rapid propagation beyond tissue culture.
Response surface methodology (RSM) is collection of statistical techniques utilized for designing experiments, constructing models, evaluating variable effects, and determining optimal conditions for desired responses. It is widely employed for the development, enhancement, and optimization of various processes. RSM assesses the impact of independent variables, either individually or in combination, in relation to the process under investigation. In addition to analyzing the effects of independent variables, this methodology generates a mathematical model that accurately describes the overall process, requiring relatively few experiments [25]. The number of lateral buds, leaf area, and plant height were utilized as response values in this experiment, which employed the response surface analysis method to examine the effects of plant growth regulators on the growth and non-tube rapid propagation of C. tortisepalum var. longibracteatum.
Selecting a suitable propagation method is an effective measure for protecting endangered plants and conserving wild resources [26]. Plant growth regulators play a crucial role in various functional processes related to plant growth and development [22]. Among these, the type and concentration of plant growth regulators are the most significant factors [7,27]. The determination of the ideal concentration of the plant growth regulator is the main reason for the success of the multiplication and rooting stages [28]. The differentiation and proliferation of adventitious buds are vital for achieving rapid propagation.
Different plants require varying concentrations of 6-BA. Song et al. found that 6-BA had the most significant impact on C. goeringii protocorm proliferation [29]. This perspective aligns with the results of our study. Wang et al. found that 6-BA significantly influenced the germination ability of Phalaenopsis axillary buds, with increasing 6-BA concentration differentiation rate first increased and then decreased [30]. 6-BA plays a crucial role in facilitating the tissue culture process of Phalaenopsis [31]. A combination of cytokinins and auxins can effectively induce bulblet formation. 6-BA notably affects the differentiation of adventitious buds in explants, while NAA promotes the growth of these buds. The combined effect of 6-BA and NAA can more effectively enhance adventitious bud differentiation, consistent with the research findings of Zhou et al. [7] and Shalan et al. [28]. The investigation conducted in this experiment demonstrated that the number of lateral buds first increased and then decreased in response to increasing 6-BA concentrations. Low concentrations of 6-BA were beneficial for the germination of adventitious buds, while high concentrations could inhibit lateral bud growth [7], in agreement with the research findings of Zheng [13].
Auxin is instrumental in regulating meristem development and cell division, thereby facilitating the formation of adventitious roots. NAA can elevate turgor pressure in leaf cells, soften the cell walls, and stimulate cell elongation. The quantity and concentration of applied auxin applied determine its effects on plant development [32,33]. It has been found that low concentrations of NAA (100 mg/L) promote the growth of both the root system and aerial parts of Carya illinoensis [34]. Xu demonstrated that NAA significantly enhances plant height in alfalfa tube-seedlings growth [35]. Zhang et al. discovered that a specific concentration of NAA could notably increase the leaf area of Maranta arundinacea L. [36]. This perspective aligns with the findings of our study. Li found that applying NAA can enhance the leaf area of the top five tobacco leaves to varying degrees, with the most significant effect on leaf area, which generally aligns with previous research [37]. In our research, it was observed that NAA could stimulate plant growth at low concentrations but inhibits it at high concentrations. Previous studies have also indicated that cluster buds may proliferate more favorably with the addition of a certain dose of NAA. However, it had been found that the combination of a low concentration of 6-BA and a high concentration of NAA was more beneficial for the proliferation of Phalaenopsis cluster buds during tissue culture induction and proliferation [38]. A specific concentration of NAA was added to the study of bud proliferation in Chinese orchids, including Cymbidium kanran, C. goeringii, and C. tortisepalum var. longibracteatum [38]. This finding indicated that NAA has a promoting effect on the bud proliferation of Chinese orchids, consistent with the results of our study.
GA3 is a phytohormone that regulates growth and development. It also enhances plant height and the weight of both shoots and roots. GA3 is synthesized in plant cell plastids. It promotes plant height by enhancing cell division, increasing the frequency of cell divisions, and inducing the expression of expansin [39]. This effect is primarily due to the presence of gibberellin receptors in plants. GA3 initially binds to its receptor, then associates with the DELLA protein to form a complex, which subsequently binds to the SCF complex. Subsequently, the DELLA protein is ubiquitinated by the F-box component of SCF and degraded by proteases, leading to the activation of downstream gibberellin-related genes, which results in changes in plant height [40]. It has been found to be highly effective in increasing the sprouting percentage of corms, as well as enhancing cormel production and size in gladiolus [15]. The findings of this study indicate that GA3 influenced the growth and rapid non-tuber propagation of C. tortisepalum var. longibracteatum. These results align with Zhou’s research on Zantedeschia aethiopica [41] but differ from Cattleya hybrid. The growth of the Cattleya hybrid is significantly impacted by 200 mg/L of GA3 [18]. However, in the single-factor test investigation, it was shown that 200 mg/L GA3 inhibited the germination of lateral buds but did not affect the development, which exhibited the maximum increase in plant height and leaf area. This may be due to the varying responses of different types to GA3. Chen found that the application of a specific concentration of GA3 could enhance lateral bud germination [42]. The number of lateral buds initially increase and then decrease with increasing GA3 concentration, indicating that low concentrations promote lateral bud germination while high concentrations inhibit this process.
After four months, some seedlings exhibited yellowing leaves, and the leaf hearts of new buds developed brown, water-stained lesions that eventually began to wither and die, while the roots and pseudobulbs remained unaffected. The cause may stem from irrigation practices, which could lead to the accumulation of plant growth regulators to in the matrix rather than their absorption by the plants, with only partial volatilization occurring. Consequently, to better address these challenges, future studies can refine the application concentrations and techniques. Additionally, the timing and methods of applying plant growth regulators, the chemical residues left by these agents under various application scenarios, and the underlying mechanisms that regulate plant development.
The response surface and contour lines illustrate the varying effects of different concentrations of 6-BA, GA3, and NAA on the growth and non-tubular rapid propagation of C. tortisepalum var. longibracteatum. This species exhibited a specific sensitivity hierarchy: for lateral bud, the sensitivity order was 6-BA > GA3 > NAA; for leaf area increase, it was NAA > GA3 > 6-BA; and for plant height increase, the order was GA3 > 6-BA > NAA. The optimal concentrations of plant growth regulators for maximizing the number of lateral buds, leaf area increase, and plant height increase were determined to be 6-BA at 43 mg/L, GA3 at 169 mg/L, and NAA at 36 mg/L. Verification results indicated the actual outcomes as 2.78 lateral buds per plant, an increase in leaf area of 2.87 cm2, and a plant height increase of 2.67 cm.
Acknowledgement: None.
Funding Statement: This study was funded by the Sichuan Science and Technology Program “Research on Synergistic Relationship and Reintroduction Technology of C. tortisepalum var. longibracteatum and Endophytic Mycorrhizal Fungi in Eastern Sichuan” (2017JY0132); Longshan Academic Talent Research Supporting Program of SWUST (17LZX521, 18LZX522).
Author Contributions: Study conception and design: Guolan Wang and Jie Li; data collection: Guolan Wang and Ting Xie; analysis and interpretation of results: Guolan Wang and Siying Qu; draft manuscript preparation and review: Guolan Wang and Lijun Fu. All authors reviewed the results and approved the final version of the manuscript.
Availability of Data and Materials: All data included in this work are available upon request by contact with the corresponding author.
Ethics Approval: Not applicable.
Conflicts of Interest: The authors declare that the research was conducted in the absence of any commercial or financial relationships that could be construed as a potential conflict of interest.
References
1. Li C, Dong N, Zhao Y, Wu S, Liu Z, Zhai J. A review for the breeding of orchids: current achievements and prospects. Hortic Plant J. 2021;7(5):380–92. doi:10.1016/j.hpj.2021.02.006. [Google Scholar] [CrossRef]
2. Chen J. Orchid biochemistry. Int J Mol Sci. 2020;21(7):2338. doi:10.3390/ijms21072338. [Google Scholar] [PubMed] [CrossRef]
3. Kindlmann P, Kull T, McCormick M. The distribution and diversity of orchids. Diversity. 2023;15(7):810. doi:10.3390/d15070810. [Google Scholar] [CrossRef]
4. Barman D, Naik S. Effect of substrate, nutrition and growth regulator on productivity and mineral composition of leaf and pseudobulb of Cymbidium hybrid “Baltic Glacier Mint Ice”. J Plant Nutr. 2017;40(6):784–94. doi:10.1080/01904167.2016.1201496. [Google Scholar] [CrossRef]
5. Jing X, Hu F. Research progress of Orchidaceae. Mol Plant Breed. 2018;16(15):5080–92. [Google Scholar]
6. Fu C, Zhou X, Zhang Y, Wang D, Chen Q. Experimental study on plant non-tube efficient rapid propagation technology. Xinjiang Agr Sci Tech. 2025;2005(3):19–20. [Google Scholar]
7. Zhou J, Liu Y, Wu L, Zhao Y, Zhang W, Yang G, et al. Effects of plant growth regulators on the rapid propagation system of Broussonetia papyrifera L. Vent Explants. 2021;12(7):874. doi:10.3390/f12070874. [Google Scholar] [CrossRef]
8. Zheng S, Pan Y, Sun Z. Effects of different plant growth regulators on tillering and growth of Cymbidium hybridum. North Hort. 2007;(1):85–7. [Google Scholar]
9. Lu X, Meng C, Han S, Qian Y, Li F, Jiao F. Effects of different culture media on 6-BA absorption and proliferation of mulberry explants. Acta Sericologica Sin. 2021;47(4):301–9. [Google Scholar]
10. Song W, Song Y, Liu X, Zhang X, Xin R, Duan S, et al. Improvement of culture conditions and plant growth regulators for in vitro callus induction and plant regeneration in Paeonia lactiflora Pall. Plants. 2023;12(23):3968. doi:10.3390/plants12233968. [Google Scholar] [PubMed] [CrossRef]
11. Tian G. Study of plant growth regulators on the effects of the growth and flowering of Bougainwort. China: Fujian Agriculture and Forestry University; 2018. [Google Scholar]
12. Wang H, Yang M, Chen S. Effect of different concentrations of 6-BA on Cyclamen leaf bud differentiation. Anhui Agric Sci Bull. 2006;12(4):71–2. [Google Scholar]
13. Zheng L. The effects of 6-BA concentration on the protocorm propagation and differentiation of Cymbidium goeringii ‘Song Mei’ × Cymbidium Hybridium ‘Fen Ji’. J Zhejiang Educ Inst. 2008;6:68–72. [Google Scholar]
14. Olszewski N, Sun T, Gubler F. Gibberellin signaling, biosynthesis, catabolism, and response pathways. Plant Cell. 2002;14(Suppl_1):S61–80. doi:10.1105/tpc.010476. [Google Scholar] [PubMed] [CrossRef]
15. Singh A, Kumar S, Rehan, Jha D. Effect of plant growth regulators (GA3, NAA and BA) on growth and flowering of Gladiolus (Gladiolus hybridus Hort.) cv. White Prosper. Int J Curr Microbiol App Sci. 2021;10(2):2008–24. doi:10.20546/ijcmas.2021.1002.241. [Google Scholar] [CrossRef]
16. Ram R, Mukherjee D, Manuja S. Plant growth regulators affect the development of both corms and cormels in Gladiolus. Hort Sci. 2002;37(2):343–4. [Google Scholar]
17. Li M, Yang Y, Wang H, Liu J, Jin W. Effects of gibberellin on growth and development of rose ‘Carola’. Acta Hort Sin. 2019;46(4):749–60. [Google Scholar]
18. Chen X. Study on solution culture technology of Cattleya hybrida. China: Agricultural University of Hebei; 2011. [Google Scholar]
19. Qi X, Liu Y, Xiang L, Wang J. Effects of cultivation measures on seedling growth of mature Cymbidium Golden Elf ‘Sundust’. Guizhou Agric Sci. 2013;41(2):77–80,85. [Google Scholar]
20. Paek K, Yeung E. The effects of 1-naphthaleneacetic acid and N6-benzyladenine on the growth of Cymbidium forrestii rhizomes in vitro. Plant Cell Tiss. Organ Cult. 1991;24:65–71. doi:10.1007/BF00039732. [Google Scholar] [CrossRef]
21. Kang H, Kang K, Kim D, Sivanesan S. In vitro propagation of Gastrochilus matsuran (Makino) Schltr., an endangered epiphytic orchid. Plants. 2020;9(4):524. doi:10.3390/plants9040524. [Google Scholar] [PubMed] [CrossRef]
22. Ishtiaq H, Bhardwaj S, Ashraf A, Kapoor D. Versatile role of auxin and its crosstalk with other plant hormones to regulate plant growth and development. Plant Arch. 2021;21(1):1621–7. doi:10.51470/PLANTARCHIVES.2021.v21.no1.221. [Google Scholar] [CrossRef]
23. Li C, Li Z. The effect of NAA treatment on Punica granatum L. pomegranate cutting seedling. South China Fruits. 2019;48(1). [Google Scholar]
24. Akalın M, Tekin K, Akyüz M, Karagöz S. Sage oil extraction and optimization by response surface methodology. Ind Crops Prod. 2015;76:829–35. doi:10.1016/j.indcrop.2015.08.005. [Google Scholar] [CrossRef]
25. Gómez-Montes E, Oliver-Salvador C, Durán-Figueroa N, Badillo-Corona J, Salas C. Optimization of direct shoot regeneration using cotyledonary explants and true leaves from lettuce cv. Romaine (Lactuca sativa L.) by surface response methodology. Plant Growth Regul. 2015;77:327–34. doi:10.1007/s10725-015-0067-5. [Google Scholar] [CrossRef]
26. Tao Q, Han G, Ma B, Jia H, Zhao C, Li WS, et al. In vitro propagation and artificial seed production of Fritillaria cirrhosa D. Don, an endangered medicinal plant. Phyton-Int J Exp Bot. 2024;93(6):1297–310. doi:10.32604/phyton.2024.051923. [Google Scholar] [CrossRef]
27. Fan B, He R, Shang Y, Xu L, Wang N, Gao H, et al. System construction of virus-free and rapid-propagation technology of Baodi garlic (Allium sativum L.). Sci Hortic. 2017;225:498–504. doi:10.1016/j.scienta.2017.07.042. [Google Scholar] [CrossRef]
28. Shalan E, Soliman S, Mahmoud A, Al-Khayri J, ALshamrani S, Safhi A, et al. Micropropagation of daylily (Hemerocallis fulva) from crown-tip explants and assessment of somaclonal variation of in vitro-propagated plants using SCoT markers. Phyton-Int J Exp Bot. 2023;92(7):2183–96. doi:10.32604/phyton.2023.028537. [Google Scholar] [CrossRef]
29. Yan H, Gao Z, Chen T, Cao L, Wang P. Callus induction of hybrid Broussonetia papyrifera leaves. Henan Sci. 2020;38:1776–80. [Google Scholar]
30. Song L, Deng W, Li J, Yang G, Qian X, Wu L. Optimization of influential factors for multiplication culture of protocorm of wild Cymbidium goeringii in Qiannan. Seed. 2012;31(9):106–8. [Google Scholar]
31. Wang C, Li X, Zhang J, Wang J, Wang D. Study on tissue culture and rapid propagation system of Phalaenopsis. North Hort. 2013;(15):122–4. [Google Scholar]
32. Zhang H, He D, Li X, Dun B, Wu D, Huang G. The establishment of rapid propagation system of ‘RED SUN’ Phalaenopsis aphrodite. Sustainability. 2022;14(22):15305. doi:10.3390/su142215305. [Google Scholar] [CrossRef]
33. He H, Qin J, Cheng X, Xu K, Teng L, Zhang D. Effects of exogenous 6-BA and NAA on growth and contents of medicinal ingredient of Phellodendron chinense seedlings. Saudi J Biol Sci. 2018;25(6):1189–95. doi:10.1016/j.sjbs.2017.11.037. [Google Scholar] [PubMed] [CrossRef]
34. Qian Y, Yang L, Huang C, Wang Z, Yang H, Wu A. Effects of combined administration of naphthalene acetic acid with paclobutrazol on growth and biomass allocation of Carya illinoensis ‘Pawnee’ container seedling. J Northeast Univ. 2023;(6):64–9. [Google Scholar]
35. Xu S. The influence of naphthyl acetic acid nano-suspension to alfalfa tube-seedlings growth. China: Liaoning Normal University; 2012. [Google Scholar]
36. Zhang G, Lv Z, Lai Y, Liang Z, Wang Y, Wang Y. Effects of naphthyl acetic acid and indole butyric acid on the growth and development of tissue culture seedlings of Maranta arundinacea L. Agric Technol. 2023;43(19):10–3. [Google Scholar]
37. Li J, Xue L, Zhu J, Xu Y, Jin L, Hao H, et al. Interaction effect of gibberelin and NAA on growth, carbon and nitrogen metabolism and leaf quality in flue-cured tobacco. Plant Physiol. 2015;(9):1473–81. doi:10.13592/j.cnki.ppj.2015.0245. [Google Scholar] [CrossRef]
38. Ruan X. Breeding system of Cymbidium tortisepalum var. longibracteatum and Cymbidium goeringii and identification of F1 hybrid seed generation. China: Southwest University of Science and Technology; 2022. [Google Scholar]
39. Bai Y, Zhu X, Fan X, Wang C, Zhang W, Liu C, et al. Research progress on plant DELLA proteins and its response to gibberellin signal regulating plant growth and development. Fenzi Zhiwu Yuzhong Mol Plant Breed. 2019;17(8):2509–16. [Google Scholar]
40. Pu R, Ma Y, Chen M, Zhang Y, Zhou G, Yan Y, et al. Research progress on regulation mechanism of gibberellin on rice plant height. Mol Plant Breed. 2022;20(20):6861–8. [Google Scholar]
41. Zhou X. Effects GA3, 6-BA, NAA on growth of calla lily (Zantedeschia hybrida). China: Sichuan Agriculture University; 2012. [Google Scholar]
42. Chen C. Effects of different plant growth regulators on the growth of hybrid cymbidium. J Anhui Agric Sci. 2018;46(10):97–9,117. [Google Scholar]
Cite This Article
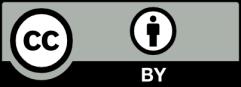
This work is licensed under a Creative Commons Attribution 4.0 International License , which permits unrestricted use, distribution, and reproduction in any medium, provided the original work is properly cited.