Open Access
ARTICLE
Assessing Stress Tolerance of SUBI and DRO1 Introgression Lines under Flooding and Drought Conditions at Different Growth Stages
1 The United Graduate School of Agricultural Sciences, Kagoshima University, Kagoshima, 890-8580, Japan
2 Sierra Leone Agricultural Research Institute, Freetown, PMB 1313, Sierra Leone
3 National Agricultural Research Organization, Abi Zonal Agricultural Research, and Development Institute, Entebbe, P.O. Box 295, Uganda
4 Directorate of Research and Training, Ministry of Agriculture and Food Security, Juba, P.O. Box 33, Republic of South Sudan
5 Institute of Agriculture, Tokyo University of Agriculture and Technology, Tokyo, 156-8502, Japan
6 International Rice Research Institute, Africa Office, Nairobi, P.O. Box 30709, Kenya
7 Faculty of Agriculture, Kagoshima University, Kagoshima, 890-0065, Japan
* Corresponding Author: Jun-Ichi Sakagami. Email: -u.ac.jp
(This article belongs to the Special Issue: Abiotic Stress in Agricultural Crops)
Phyton-International Journal of Experimental Botany 2025, 94(2), 303-316. https://doi.org/10.32604/phyton.2025.060016
Received 22 October 2024; Accepted 17 January 2025; Issue published 06 March 2025
Abstract
Rice varieties tolerant to submergence regulate shoot elongation during short-term submergence by expressing the SUB1A gene. In contrast, the deep-rooted DRO1 is effectively expressed under drought conditions to enhance water and nutrient uptake. This study investigates the growth and yield of rice with both SUB1A and DRO1 in the background of IR64, under early-season flooding, and mid-season drought. The study used a randomized complete design with two factors: soil moisture treatments (submergence, drought, and their combination) and genotypes. The genotypes included IR64, and three near-isogenic lines (NILs): NIL-SUB1DRO1, NIL-SUB1, and NIL-DRO1. Complete submergence was imposed for 7 days on 14-day-old seedlings, while drought was imposed on control and submerged plants following a 21-day recovery period from submergence, using 42-day-old plants. Variables were measured before and after treatments (submergence and drought), and at harvest or grain maturity. The stresses negatively affected the genotypes. At harvest, IR64 and NIL-SUB1DRO1 under both stresses showed a significant reduction in tiller numbers, shoot dry weights, and yields compared to their control plants. IR64 exhibited a significant delay in reaching flowering under all stresses. The rice introgression lines showed significant improvements in tolerance to the stresses. The study showed no negative consequences of combining drought and submergence tolerance in rice.Keywords
Drought and submergence are considered the predominant abiotic stresses impacting global rice production [1], affecting approximately 40 million hectares of rice-growing areas at various crop growth stages and negatively influencing plant growth, development, and yield [2]. Although typically expected to occur at different levels in a topographic sequence, submergence and drought can occur in the same area within a single growing season in rainfed rice ecosystems.
Typically cultivated under partially flooded conditions, rice is a semiaquatic species. However, flash flooding that completely submerges rice plants for extended periods can cause most rice cultivars to die within a few days [3]. Flooding severely restricts O2 and CO2 gas exchange between rice tissues and the atmosphere, inhibits aerobic respiration and photosynthesis, and accelerates the consumption of energy reserves, leading to stunted growth and death [4]. Some rice genotypes can overcome complete submergence through antithetical growth responses. For instance, the submergence-tolerant lowland rice cultivar FR13A limits its elongation growth, conserving carbohydrate reserves to support the development of new leaves after submergence [3]. This quiescence response is regulated by the submergence 1A (SUB1A) gene, which dampens ethylene production and enhances the mRNA and protein accumulation of two negative regulators of gibberellic acid signaling, resulting in the suppression of the energy-consuming escape response during submergence [4]. Regeneration ability after flooding is vital for plants to produce high yields. Rapid regeneration of growth following flooding is an important plant characteristic [3]. After flooding, tolerant varieties (SUB1A) show higher survival and faster regeneration ability by producing fresh leaves more rapidly and maintaining shoot growth compared to non SUB1A varieties, such as IR64 [4,5]. Recovery is also faster in tolerant varieties due to their efficient reactive oxygen species scavenging systems and reduced lipid peroxidation when exposed to air [6]. Compared with sensitive lines, SUB1A introgression lines maintain more sugar and starch concentration after submergence [7].
Drought, defined as the occurrence of a rainfall shortage relative to the expected (average) amount for a target region [8], is the most important source of climate-related risk in rainfed areas [1]. It is a major challenge for all agricultural crops, particularly for rice. Water unavailability in root zones is an important factor that reduces growth and yield of drought susceptible rice genotypes [9]. Alterations in agronomic traits under drought conditions alter the final crop yield, including growth parameters, such as plant height and biomass at harvest, and components of harvestable yield, such as the panicle number per unit area, grain number per panicle, 1000-grain weight, panicle length, and filled grain percentage/seed setting rate [10]. Drought tolerance is defined as the ability of plants to survive under a low tissue water content [11,12] and they show adaptive mechanisms such as higher leaf water potential and better osmotic adjustment, along with protective mechanisms such as leaf rolling and stomatal closure. Drought triggers the production or mobilization of the phytohormone abscisic acid, which is well known for inducing stomatal closure to conserve water [13]. Apart from abscisic acid, other factors that accumulate during drought and affect stomatal function include plant hormones (auxins, ethylene, brassinosteroids, and cytokinins), microbial elicitors (salicylic acid, harpin, and chitosan), and polyamines [12]. Leaf rolling, a common adaptive response to drought stress in plants, is caused by alterations in water potential within epidermal and bulliform cells. Leaf rolling slows down transpiration and enhances water retention. Among the various components of drought tolerance in rice, root architecture remains a promising trait for analyzing differences in rice genotypes in response to drought, although it has been less explored than the above-ground changes [9]. The four primary root traits related to drought tolerance are root length, volume, thickness, and root growth angle [9]. Root length is a key component of root traits for drought tolerance, as it determines root depth. Deeper and profuse root systems help plants survive drought stress by extracting water from deeper soil layers. In upland rice, these root systems enhance drought tolerance by improving the plant’s water uptake [14]. Three major quantitative trait loci (QTLs) associated with root growth angles have been reported in rice [9]. Among these three, DEEPER ROOTING 1 (DRO1) remains the major. Some tolerant rice cultivars, such as Chuanguyou208 and Deyou4727, have demonstrated higher root number, root weight, and greater root length, along with increased expression of important enzymatic antioxidants such as superoxide dismutase and peroxidase under drought stress conditions [15]. Plants’ recovery ability, during post-drought stress, is also considered important, with some researchers suggesting its priority over drought tolerance itself [16].
Despite the importance of submergence and drought to rice production in rainfed environments, the growth and yield of rice genotypes when subjected to both submergence and drought within the same season have not been fully investigated. Therefore, we evaluated the growth and yield of Near Isogenic Line (NIL)-SUB1DRO1 developed in IR64 genetic background, under early flood and mid-growth drought. NIL-SUB1DRO1 was obtained by crossing NIL-SUB1 (a cross between IR64 and the submergence-tolerant rice FR13A) with NIL-DRO1 (a cross between IR64 and the drought-tolerant rice Kinandang Patong).
This study was conducted from May to October 2023 in a greenhouse at Kagoshima University, Kagoshima, Japan with mean temperature and relative humidity of 27.9°C and 74.3%, respectively, during the experimental period.
The experiment comprised four environmental factors: control (optimum moisture), submergence, drought, and submergence + drought combination treatments. Rice genotypes used in this study were provided by various institutions, IR64 and NIL-SUB1, International Rice Research Institute (IRRI), Philippines; NIL-DRO1, National Agriculture and Food Research Organization, Japan; and NIL-SUB1DRO1, Tokyo University of Agriculture and Technology, Japan under collaboration with Kagoshima University, Japan. The seeds were placed in a petri dish containing filter paper moistened with distilled water and left to germinate at 30°C in an incubator under dark conditions for 48 h. After germinating, the seeds were carefully selected and directly sown into polyvinyl chloride (PVC) pipes with an inner diameter of 8 cm and height of 80 cm, filled with 5.6 kg soil and arranged in a completely randomized design with 5 replications and each replication contained 1 sample. To prevent nutrient deficiencies in the experimental soil, 10 g N, P, and K (8-8-8) compound fertilizer was mixed with the soil during preparation. At the panicle initiation stage, a mixture of 0.4 g of nitrogen and 4.6 g of ammonium sulfate fertilizers were added to each PVC pipe. For each treatment, three germinated seeds were sown per pipe, which were thinned to one seedling after five days.
Fourteen-day-old seedlings grown in 20 cm height PVC pipes were transferred to a transparent glass container (80 cm × 55 cm × 50 cm) and completely submerged with tap water for seven days. After the desubmergence, the 20 cm height PVC pipes were attached to 60 cm height PVC pipes. Drought treatment was imposed on plants that received both submergence and drought treatments after 21 days of recovery from submergence. For plants that received only drought treatment, the treatment was imposed after 41 days of seedling growth. Drought was induced by withholding irrigation for 14 days, while irrigation continued for the control plants and those that had previously received submergence treatment.
The greenhouse temperature and humidity were measured using the Long-Range Wireless Connection Logger Telemoni TML2101-A (AS ONE Corporation, Osaka, Japan). A soil moisture sensor placed at a depth of 10 cm was used to measure the soil water content of the pipes. Data was recorded using a ZL6 Basic Datalogger (Meter Group, Inc., Pullman, WA, USA) with a 60-min interval between each measurement throughout the drought period. Shoot or plant length was measured from the base of the stem to the highest shoot tip using a meter ruler, taken before and after each treatment and at harvest. A chlorophyll meter (SPAD-502, Konica Minolta Corporation, Tokyo, Japan) was used to measure the chlorophyll content or SPAD value present in leaves. The SPAD value was an average of three measurements taken on the upper part of a newly fully developed leaf. This parameter was taken before and after each treatment, and at harvest. Days to flowering were determined by counting the days from sowing to when half of the culms of the plant in the PVC pipe had flowered (heading). Tiller and panicle numbers were determined by physically counting culm numbers and culms bearing grain, respectively. Tillers were counted before and after each treatment and at harvest, while panicle numbers were only taken at harvest. To determine grain yield, the plants in each PVC pipe were harvested and threshed. After empty spikelets were removed, the filled spikelets were weighed. Following harvest, fresh shoots were separated from dry shoots and then oven-dried at 80°C for 72 h to determine the shoot dry weight after weighing. The root dry weight was determined after washing the roots with tap water, followed by oven-drying at 80°C for 72 h and weighing.
Data from 5 replications, was analyzed using a two-way analysis of variance. If significant differences were found, the least significant difference (LSD) test was conducted at p = 0.05 for mean separation.
Fig. 1 presents the soil moisture content of the rice genotypes during the 14-day drought period. IR64, NIL-SUB1DRO1, and NIL-DRO1 showed reductions of 70.2%, 69.2%, and 70.5% in soil moisture content relative to the control, respectively.
Figure 1: Soil moisture content of rice genotypes during the drought period
The plant length of IR64 increased significantly by 22.1% during submergence, while those of submerged NIL-SUB1, NIL-SUB1DRO1, and control plants showed no significant difference (Fig. 2a). At harvest, submerged IR64 showed a 17.7% decrease in plant length compared to the control. Under drought conditions, IR64 was significantly shorter than the control after 14 days of drought, while drought-treated NIL-DRO1 and NIL-SUB1DRO1 showed no significant difference in plant length compared to the control plants. At harvest, drought-treated IR64 and NIL-SUB1DRO1 showed respective 17.1% and 9.2% decreases in plant length compared to the control plants (Fig. 2b). Under the combined submergence and drought treatments, the plant length of IR64 increased significantly during submergence but was markedly reduced pre-drought, post-drought, and at harvest compared to the control. IR64 showed a 12.9% decrease relative to NIL-SUB1DRO1 at harvest under a combination of the two stresses (Fig. 2c). IR64, NIL-SUB1DRO1, and NIL-SUB1 showed respective decreases of 27.2%, 15.5%, and 16.5% in SPAD values after 7 days of complete submergence (Fig. 3a). At 14 days post-drought, no significant difference in SPAD values was observed between NIL-SUB1DRO1, NIL-DRO1, and the control plants, while IR64 showed a 14.6% decrease compared to the control plants (Fig. 3b). Under the combined treatment, the SPAD value of IR64 decreased significantly, by 27.3% after seven days of complete submergence and by 8.6% after 14 days of drought (Fig. 3c). Similarly, NIL-SUB1DRO1 showed significant decreases of 15.5% after submergence and 5.6% after drought compared to the control. No significant differences were found between the genotypes and control plants for SPAD values under the different treatments during harvest.
Figure 2: Plant length of submergence (a), drought (b), and submergence + drought (c) treatments. C = control, S = submergence, D = drought, S + D = submergence + drought, NIL-S1 = NIL-SUB1, NIL-D1 = NIL-DRO1 and NIL-S1D1 = NIL-SUB1DRO1. Different letters at the same time of data collection indicate a significant difference at p < 0.05 based on the least significant difference (LSD) test
Figure 3: SPAD values of submergence (a), drought (b), and submergence + drought (c) treatments. C = control, S = submergence, D = drought, S + D = submergence + drought, NIL-S1 = NIL-SUB1, NIL-D1 = NIL-DRO1 and NIL-S1D1 = NIL-SUB1DRO1. Different letters at the same time of data collection indicate a significant difference at p < 0.05 based on the LSD test
Before each of the three treatments, no significant differences were observed between the NILs in tiller number. However, IR64 showed 38.9% and 55.6% decreases under drought and combined treatment relative to the control. At harvest, submerged IR64 and NIL-SUB1DRO1 showed significant decreases in tiller numbers of 77.0% and 42.1%, respectively, relative to the control, with respective values of 66.3% and 37.5% under drought treatment and 71.9% and 51.1% under the combined treatment (Fig. 4a–c). The different stresses delayed the flowering of the genotypes compared to their controls. Submergence, drought, and their combination delayed the flowering of IR64 significantly, by 10.4, 11.0, and 19.2 days, respectively (Fig. 5). Significant differences were also observed between NIL-SUB1DRO1 and IR64 for days to flowering under submergence and drought, with IR64 showing significantly more days in both cases. No significant difference was found between the genotypes for days to flowering under control conditions.
Figure 4: Tiller number of submergence (a), drought (b), and submergence + drought (c) treatments. C = control, S = submergence, D = drought, S + D = submergence + drought, NIL-S1 = NIL-SUB1, NIL-D1 = NIL-DRO1 and NIL-S1D1 = NIL-SUB1DRO1. Different letters at the same time of data collection indicate a significant difference at p < 0.05 based on the LSD test
Figure 5: Days to the flowering of four rice genotypes under control, submergence, drought, and submergence + drought treatments. Different letters indicate a significant difference at p < 0.05 based on the LSD test
Submergence, drought, and their combination reduced the grain yield of IR64 by 77.1%, 57.5%, and 75.5%, respectively, as well as that of NIL-SUB1DRO1 by 46.1%, 21.0%, and 52.8%, respectively, relative to the control (Fig. 6). Drought significantly reduced the grain yield of NIL-DRO1 by 23.4%, and submergence markedly decreased the grain yield of NIL-SUB1 by 26.0%.
Figure 6: Grain yield of four rice genotypes under control, submergence, drought, and submergence + drought treatments. Different letters indicate a significant difference at p < 0.05 based on the LSD test
Submergence significantly reduced the panicle number, shoot dry weight, and root dry weight of IR64 by 70.2%, 57.2%, and 65.2%, respectively (Tables 1 and 2). The panicle number, shoot dry weight, and root dry weight of NIL-SUB1DRO1 were also significantly reduced by submergence by 44.7%, 34.7%, and 32.7%, respectively. No significant difference was observed between the submerged and control plants of NIL-SUB1 for panicle number and shoot dry weight. Similarly, under drought treatment, no significant difference was found between the drought and control plants of NIL-DRO1 for shoot dry weight (Table 1). The panicle number, shoot dry weight, and root dry weight of IR64 was also significantly reduced under drought, by 63.8%, 40.0%, and 78.0%, respectively, while those of NIL-SUB1DRO1 were also reduced significantly but to a lesser extent, by 45.8%, 29.7%, and 40.0%, respectively. The combined treatment reduced the panicle number, shoot dry weight, and root dry weight of all genotypes, with respective reductions of 54.1%, 44.5%, and 27.3% for NIL-SUB1DRO1 and 72.5%, 63.7%, and 76.1% for IR64. The panicle length of IR64 was significantly reduced by 5.2, 20.6, and 8.3 cm following submergence, drought, and combined treatments, respectively. In contrast, no significant difference was observed in panicle length between drought-treated and combined stress-treated NIL-SUB1DRO1 and its control plants. Notably, the 100-grain weight did not significantly differ due to submergence, drought, or their combination, compared with the control plants. No significant difference was found between the studied genotypes for plant length before submergence (Fig. 7 Left). IR64 had fewer leaves compared to NIL-SUB1DRO1 and NIL-SUB1 after recovery from submergence (Fig. 7 Right). The different treatments markedly reduced the growth and yield of IR64, NIL-SUB1, NIL-DRO1, and NIL-SUB1DRO1 compared to control (Figs. 8–10).
Figure 7: Plant growth before submergence and recovery in submergence experiment. Effect of soil flooding on the growth of rice plants before submergence (left) and after recovery (right) period IR64 (a), NIL-SUB1DRO1 (b), and NIL-SUB1 (c) under submergence
Figure 8: Plant growth at maturity in submergence experiment. Effect of soil flooding on the growth of rice plants during the ripening period. NIL-SUB1 (a), IR64 (b), NIL-SUB1DRO1 (c) in control, NIL-SUB1 (d), IR64 (e), and NIL-SUB1DRO1 (f) after submergence
Figure 9: Plant growth at maturity in drought experiment. Effect of soil drought on the growth of rice plants during the ripening period. NIL-DRO1 (a), IR64 (b), NIL-SUB1DRO1 (c) in control, NIL-DRO1 (d), IR64 (e), and NIL-SUB1DRO1 (f) after drought
Figure 10: Plant growth at maturity in submergence and drought combination experiment. Effect of soil flooding and drought on the growth of rice plants during the ripening period. IR64 (a), NIL-SUB1DRO1 (b) in control, IR64 (c), and NIL-SUB1DRO1 (d) after drought following submergence
The combined effects of submergence and drought are not considered to be greater than their individual effects [17]. Therefore, this study aimed to analyze the growth and yield of rice with submergence and drought tolerance genes. NIL-SUB1DRO1 is a breeding line developed from crossing IR64, FR13A, and Kinandang Patong. IR64 is a modern lowland cultivar developed by the International Rice Research Institute and grown widely in South and Southeast Asia owing to its high yield and good grain quality, but it is sensitive to abiotic stresses, including submergence and drought. IR64 was primarily developed for irrigated rice production. An indica cultivar FR13A is highly tolerant of flooding and can survive for up to two weeks of complete submergence owing to a major QTL designated Sub1 near the centromere of chromosome 9. Kinandang Patong contains DRO1, a major QTL involved in the deep rooting of rice under upland field conditions. This QTL controls the root system architecture and increases rice yield under drought conditions [9]. Introducing rice varieties with submergence and drought tolerance to farmers is crucial, given the increasingly unfavorable climate changes.
Drought and submergence stresses are important abiotic factors that adversely affect the growth and yield of rice [17]. These stresses reduce plant growth by affecting various physiological and biochemical processes, such as photosynthesis, respiration, translocation, ion uptake, synthesis of carbohydrates, nutrient metabolism, and overall plant growth [18]. In our study, submergence, drought, and their combination treatments markedly reduced the growth parameters and yield of the studied genotypes. Studies have shown that leaf photosynthetic rates of drought- and submergence-affected rice are reduced due to rapid declines in water availability, light intensity, and gas diffusion rates [2]. Consequently, the contents of non-structural carbohydrates in rice stems are reduced. Consistent with these studies, we found that submergence, drought, and their combination at the seedling and vegetative growth stages decreased the growth of rice genotypes, negatively affecting their grain yield. Grain yield is considered the most appropriate and direct criterion for selecting genotypes for drought tolerance due to the moderate to high heritability of this trait under drought conditions [19–22] showed that the tiller number declined sharply during submergence, which supports our findings that tiller numbers of the genotypes were significantly decreased by submergence, drought, and their combination compared to the control. Accordingly, the lower tiller numbers led to reduced panicle numbers at the maturity stage.
A common adverse effect of water stress on crop plants is a reduction in fresh and dry biomass production. Reference [23] showed that high dry weight under water stress conditions is a desirable characteristic for plant survival. This present study uncovered that the shoot and root dry weights of NIL-SUB1DRO1 were significantly higher than those of IR64 under stress conditions. Submerged IR64 showed significantly longer shoots after submergence but significantly shorter plants at harvest. According to the mechanism of submergence defined by [24–28], the elongation exhibited by IR64 represents transient submergence intolerance, whereas the relative quiescence exhibited by NIL-SUB1DRO1 represents tolerance. Shoot elongation during submergence, as experienced during flash flooding, is known to negatively impact flood tolerance due to the depletion of carbohydrates and the risk of lodging after desubmergence [23]. NIL-SUB1DRO1 carries the SUB1A gene, which limits plant elongation during submergence, enabling a greater accumulation of shoot dry matter, compared to IR64 [23]. The significantly lower plant length of IR64 at harvest under the different stresses compared to control plants could be attributed to a decrease in turgor that impairs cell elongation and expansion [17]. References [29–31] reported that chlorophyll is the medium for absorption, transformation, and light energy transmission in plants; therefore, under abiotic stresses, plants with higher chlorophyll content or SPAD values have better chances of carbohydrate synthesis. A general delay in flowering occurs after submergence in all plant materials, as it takes the surviving plants additional time to recover and resume normal vegetative growth, and to repair the damage caused during and after submergence [32]. In this study, the submergence-, drought-, and submergence–drought-tolerant genotypes, NIL-SUB1, NIL-DRO1, and NIL-SUB1DRO1, respectively, showed the least delay in flowering. This is likely because they experienced less damage from submergence, drought, and combined treatments compared to the sensitive variety, IR64. This shows that the introgression of SUB1A and DRO1 narrowed the delay in flowering caused by submergence and drought by maintaining healthier plants during and after stress. NIL-SUB1DRO1 was found to be unchanged in all variable measurements compared to IR64, NIL-SUB1, and NIL-DRO1 under control conditions. This indicates that the combination of SUB1A and DRO1 does not cause any negative effects on the new genotype.
Submergence, drought, and their combination during early season flooding and mid-season drought significantly affected the growth and yield of the rice genotypes studied. However, NIL-SUB1DRO1, NIL-SUB1, and NIL-DRO1 maintained stable growth and yield. In particular, NIL-SUB1DRO1 was found to be resistant to the combined stresses of early flooding and mid-season drought during the growing season. The study findings provide empirical data that can inform breeding strategies to enhance rice submergence and drought tolerance in the future.
Acknowledgement: We thank Dr. Yusaku Uga of the National Agriculture and Food Research Organization, Japan for providing IR64DRO1 used to breed NIL-SUB1DRO1 and the United Graduate School of Agricultural Sciences, Kagoshima University, Kagoshima, Japan for the research fund provided to carry out this study.
Funding Statement: The authors received no specific funding for this study.
Author Contributions: The authors confirm contribution to the paper as follows: study conception and design: Jun-Ichi Sakagami, Ibrahim Soe; data collection: Ibrahim Soe, Emmanuel Odama, Aquilino Lado Legge Wani, Alex Tamu; analysis and interpretation of results: Jun-Ichi Sakagami, Ibrahim Soe, Emmanuel Odama; draft manuscript preparation: Jun-Ichi Sakagami, Ibrahim Soe, Abdelbagi M. Ismail; rice genotypes provided: NIL-SUB1DRO1 is provided by Taiichiro Ookawa. All authors reviewed the results and approved the final version of the manuscript.
Availability of Data and Materials: The article includes all data collected during this study. All the data in this study are available upon request.
Ethics Approval: Not applicable.
Conflicts of Interest: The authors declare no conflicts of interest to report regarding the present study.
References
1. Ashfaq M, Haider MS, Khan AS, Allah SU. Breeding potential of the basmati rice germplasm under water stress conditions. Afri J Biotech. 2022;11(25):6647–57. doi:10.5897/AJB11.3698. [Google Scholar] [CrossRef]
2. Dixit S, Singh A, Sandhu N, Bhandari A, Vikram P, Kumar A. Combining drought and submergence tolerance in rice: marker-assisted breeding and QTL combination effects. Molec Breed. 2017;37(12):143. doi:10.1007/s11032-017-0737-2. [Google Scholar] [PubMed] [CrossRef]
3. Bailey-Serres J, Fukao T, Ronald P, Ismail A, Heuer S, Mackill D. Submergence tolerant rice: SUB1’s journey from landrace to modern cultivar. Rice. 2010;3(2–3):138–47. doi:10.1007/s12284-010-9048-5. [Google Scholar] [CrossRef]
4. Dessougi HE, Gasim S, Abuanja I, Abdella A, Nile E. Screening for complete submergence seedling tolerance in rice (Oryza sativa L.) genotypes collected from Sudan and South Sudan. Afri J Plant Sci. 2022;16(9):250–7. doi:10.5897/AJPS2022.2270. [Google Scholar] [CrossRef]
5. Hattori Y, Nagai K, Ashikari M. Rice growth adapting to deepwater. Curr Opin Plant Bio. 2011;14(1):100–5. doi:10.1016/j.pbi.2010.09.008. [Google Scholar] [PubMed] [CrossRef]
6. Nagai K, Hattori Y, Ashikari M. Stunt or elongate? Two opposite strategies by which rice adapts to floods. J Plant Res. 2010;123(3):303–9. doi:10.1007/s10265-010-0332-7. [Google Scholar] [PubMed] [CrossRef]
7. Nurrahma AHI, Yabuta S, Junaedi A, Sakagami J-I. Analysis of non-structural carbohydrates in relation with shoot elongation of rice under complete submergence. Sustainability. 2021;13(2):670. doi:10.3390/su13020670. [Google Scholar] [CrossRef]
8. Zhu R, Wu F, Zhou S, Hu T, Huang J, Gao Y. Cumulative effects of drought-flood abrupt alternation on the photosynthetic characteristics of rice. Env Exp Bot. 2020;169:103901. doi:10.1016/j.envexpbot.2019.103901. [Google Scholar] [CrossRef]
9. Uga Y, Okuno K, Yano M. Dro1, A major QTL involved in deep rooting of rice under upland field conditions. J Exp Bot. 2011;62(8):2485–94. doi:10.1093/jxb/erq429. [Google Scholar] [PubMed] [CrossRef]
10. Zhang X, Zhou S, Fu Y, Su Z, Wang X, Sun C. Identification of a drought-tolerant introgression line derived from Dongxiang common wild rice (O. rufipogon Griff.). Plant Molec Biol. 2006;62(1–2):247–59. doi:10.1007/s11103-006-9018-x. [Google Scholar] [CrossRef]
11. Zhang J, Zhang S, Cheng M, Jiang H, Zhang X, Peng C, et al. Effect of drought on agronomic traits of rice and wheat: a meta-analysis. Int J Env Res Public Hea. 2018;15(5):839. doi:10.3390/ijerph15050839. [Google Scholar] [PubMed] [CrossRef]
12. Li Q, Zhu P, Yu X, Xu J, Liu G. Physiological and molecular mechanisms of rice tolerance to salt and drought stress: advances and future directions. Int J Molec Sci. 2024;25(17):9404. doi:10.3390/ijms25179404. [Google Scholar] [PubMed] [CrossRef]
13. Meher, Shivakrishna P, Ashok Reddy K, Manohar Rao D. Effect of PEG-6000 imposed drought stress on RNA content, relative water content (RWCand chlorophyll content in peanut leaves and roots. Saudi J Bio Sci. 2018;25(2):285–89. doi:10.1016/j.sjbs.2017.04.008. [Google Scholar] [PubMed] [CrossRef]
14. Sarkar JP, De Datta SK. Drought tolerance in rice: screening technique and selection criteria. In: IRRI Saturday Seminar; 1975; Los Banos, Philippines. [Google Scholar]
15. Wang X, Samo N, Li L, Wang M, Qadir M, Jiang K, et al. Root distribution and its impacts on the drought tolerance capacity of hybrid rice in the sichuan basin area of China. Agronomy. 2019;9(2):79. doi:10.3390/agronomy9020079. [Google Scholar] [CrossRef]
16. Kumar A, Basu S, Ramegowda V, Pereira A. Mechanisms of drought tolerance in rice. In: Sasaki T, editor. Achieving sustainable cultivation of rice. UK: Burleigh Dodds Science Publishing Limited; 2016. doi:10.12688/f1000research.7678.1. [Google Scholar] [CrossRef]
17. Lesk C, Rowhani P, Ramankutty N. Influence of extreme weather disasters on global crop production. Nature. 2016;529(7584):84–7. doi:10.1038/nature16467. [Google Scholar] [PubMed] [CrossRef]
18. Lau SE, Hamdan MF, Pua TL, Saidi NB, Tan BC. Plant nitric oxide signaling under drought stress. Plants. 2021;10(2):360. doi:10.3390/plants10020360. [Google Scholar] [PubMed] [CrossRef]
19. Zain MNA, Ismail MR, Puteh A, Mahmood M, Islam MR. Drought tolerance and ion accumulation of rice following application of additional potassium fertilizer. Communi Soil Sci Plant Anal. 2014;45(19):2502–14. doi:10.1080/00103624.2014.932374. [Google Scholar] [CrossRef]
20. Fofana M, Sakariyawo LS, Popogbe MO, Oyekanmi AA, Azeez JO, Adegbehingbe FT. Physiological and agronomic responses of four rice varieties to drought in the rainforest. Not Sci Biologicae. 2018;10(2):220–7. doi:10.15835/nsb10210187. [Google Scholar] [CrossRef]
21. Zhu G, Chen Y, Ella ES, Ismail AM. Mechanisms associated with tiller suppression under stagnant flooding in rice. J Agro Crop Sci. 2019;205(2):235–47. doi:10.1111/jac.12316. [Google Scholar] [CrossRef]
22. Setter TL, Waters I, Wallace I, Bhekasut P, Greenway H. Submergence of rice I. Growth and photosynthetic response to CO2 enrichment of floodwater. Fun Plant Bio. 1989;16(3):251–63. doi:10.1071/PP9890251. [Google Scholar] [CrossRef]
23. Fukao T, Bailey-Serres J. Submergence tolerance conferred by SUB1A is mediated by SLR1 and SLRL1 restriction of gibberellin responses in rice. Proc Nati Acad Sci. 2008;105(43):16814–9. doi:10.1073/pnas.0807821105. [Google Scholar] [PubMed] [CrossRef]
24. Yutiao C, Jiayu S, Chuan Y, Xiaofu H. Effects of submergence stress at the vegetative growth stage on hybrid rice growth and grain yield in China. Chilean J Agri Res. 2021;81(2):191–201. doi:10.4067/S0718-58392021000200191. [Google Scholar] [CrossRef]
25. Yu Y, Meng Y, Chen P, Cao K. Submergence stress reduces the ability of rice to regulate recovery after disaster. Agronomy. 2024;14(6):1319. doi:10.3390/agronomy14061319. [Google Scholar] [CrossRef]
26. Sone C, Ito O, Sakagami JI. Characterizing submergence survival strategy in rice via chlorophyll fluorescence. J Agro Crop Sci. 2012;198(2):152–60. doi:10.1111/j.1439-037X.2011.00494.x. [Google Scholar] [CrossRef]
27. Nurrahma AHI, Yabuta S, Ahmad J, Sakagami JI. Characterizing the photosynthetic ability of the submergence-tolerant rice variety of Inpari30 via maximum quantum yield performance during transient flooding stress and recovery. Austra J Crop Sci. 2021;15(1):107–13. doi:10.21475/ajcs.21.15.01.2889. [Google Scholar] [CrossRef]
28. Khanal D, Bastakoti B, Banjade D. Impacts of submergence stress on rice plants and its adaptation: a review. Archives Agri Env Sci. 2024;9(3):626–31. doi:10.26832/24566632.2024.0903030. [Google Scholar] [CrossRef]
29. Farooq M, Hussein M, Wahid A, Siddique KH. Plant responses to drought stress. Berlin/Heidelberg: Springer; 2012. doi:10.1007/978-3-642-32653-0-1. [Google Scholar] [CrossRef]
30. Das KK, Panda D, Sarkar RK, Reddy JN, Ismail AM. Submergence tolerance in relation to variable floodwater conditions in rice. Env Exp Botany. 2009;66(3):425–34. doi:10.1016/j.envexpbot.2009.02.015. [Google Scholar] [CrossRef]
31. Panda D, Sharma SG, Sarkar RK. Chlorophyll fluorescence parameters, CO2 photosynthetic rate and regeneration capacity as a result of complete submergence and subsequent re-emergence in rice (Oryza sativa L.). Aquatic Bot. 2008;88(2):127–33. doi:10.1016/j.aquabot.2007.08.012. [Google Scholar] [CrossRef]
32. Ram PC, Singh BB, Singh AK. Submergence tolerance in rainfed lowland rice: physiological basis and prospects for cultivar improvement through marker-aided breeding. Field Crops Res. 2002;76(2–3):131–52. doi:10.1016/S0378-4290(02)00035-7. [Google Scholar] [CrossRef]
Cite This Article
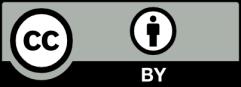
This work is licensed under a Creative Commons Attribution 4.0 International License , which permits unrestricted use, distribution, and reproduction in any medium, provided the original work is properly cited.