Open Access
ARTICLE
Determination of Fungal Species to Investigate the Aflatoxin Contamination in Rice (Oryza sativa L.)
1 Department of Biology, College of Science, Princess Nourah bint Abdulrahman University, P.O. Box 84428, Riyadh, 11671, Saudi Arabia
2 Institute of Botany, University of the Punjab, Lahore, 54000, Pakistan
3 Department of Plant Pathology, College of Agriculture, University of Sargodha, Sargodha, 40100, Pakistan
4 Université de La Réunion, Laboratoire CHEMBIOPRO (Chimie et Biotechnologie des Produits Naturels), ESIROI Département agroalimentaire, 15 avenue René Cassin, Saint-Denis, F-97490, France
5 Department of Biotechnology, Adamas University, Barasat-Barrackpore Road, 24 Parganas North, Jagannathpur, Kolkata, 700126, West Bengal, India
6 Natural and Medical Sciences Research Center, University of Nizwa, Nizwa, 616, Oman
7 Department of Food Science & Technology, Faculty of Food & Home Sciences, MNS-University of Agriculture, Multan, 630496, Pakistan
* Corresponding Authors: Aisha Umar. Email: ; Yasir Iftikhar. Email:
Phyton-International Journal of Experimental Botany 2025, 94(2), 407-420. https://doi.org/10.32604/phyton.2025.058035
Received 03 September 2024; Accepted 14 January 2025; Issue published 06 March 2025
Abstract
Aspergillus species produce aflatoxins and raise concerns about food safety in departmental stores and manufacturing mills. To address the risks posed by aflatoxins, and to advise the public on the highest quality rice that serves as a nutritious food source, an inquiry following the guidelines outlined in both local and international standards of food safety for the presence of aflatoxins is an essential requirement. Therefore, 16 white rice samples were selected randomly from low/high socio-economic departmental stores from 16 different localities. Grind powdered rice filtrate was extracted using chloroform. The filtrate applied on TLC plates and the amount of aflatoxin and moisture contents were determined. In the non-infected rice, moisture content was low (9.08%) whereas high [13.65% > 12% (standard value)] in infected ones. Four out of 8 samples of low-quality rice were contaminated with AFB1 and AFB2 (ranging from 22.2 to 29.3 µg/kg). All the samples except one (22.3 µg/kg) from high-quality rice were certified fit despite the contamination with AFB1. Furthermore, phylogenetic analysis showed Aspergillus flavus from unfit low (Long grain brown and Brown basmati) and high-quality (Basmati-198) rice whereas A. parasiticus from unfit low-quality Medium-grain brown rice. The presented research proves that the detection of fungi and aflatoxins in rice grains poses a huge risk to the health of consumers. Therefore, it is necessary to check the rice grains before distribution.Graphic Abstract
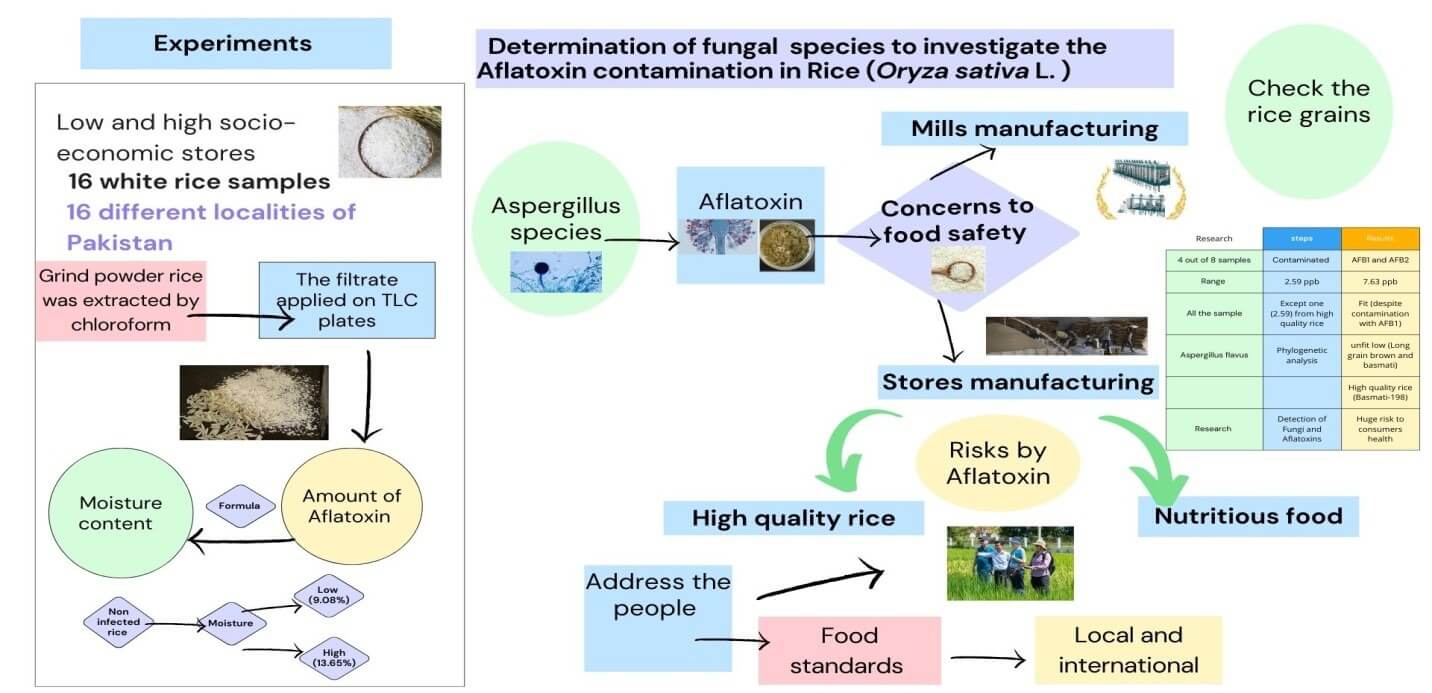
Keywords
Rice (Oryza sativa L.) belongs to the family Poaceae. It is an annual and perennial staple crop of tropical areas [1–3]. The Asian countries are not only the largest producers but also the exporters around the globe [4]. There are two types of rice such as; fine rice called “White Basmati Rice” which is globally renowned due to its strong aroma and grain extension during cooking [5] and the other one is “Brown Basmati Rice” in the form of whole grain without the inedible outer husk. Other rice varieties grown in worldwide are typically limited to specific geographic areas such as; basmati rice is grown in Pakistan and India, while jasmine rice is cultivated in Thailand [6]. The characteristic taste of basmati rice, reminiscent of pandan (Pandanus fascicularis leaf), is due to the aromatic compound 2-acetyl-1-pyrroline [7]. Basmati rice is a favorite foodstuff in the international community. Basmati rice is mainly exported to Qatar, the United Arab Emirates, Bahrain, Kuwait, Yemen, Malaysia, Saudi Arabia, the UK and USA [8]. The rice industry contributes 21.75% to the national economy through the production of 29.5% of the rice. However, post-harvest operations impact the overall quantity of rice produced and, consequently, the economy. If the seed quality does not meet international standards it affects the rice market and can reduce yield per acre due to outdated technologies [9].
The cultivation of Basmati rice takes place during the kharif season [10]. The damp conditions favor the plant fungal pathogens and the production of aflatoxins as well [11,12]. Therefore, environmental conditions of locations between 40° N and 40° S of the equator in the growing season of a crop play a vital role in the production of aflatoxins [13,14]. Adverse drought strongly favors the presence of aflatoxins produced by Aspergillus (A. flavus and A. parasiticus) in grains [15]. In aflatoxin, “A” denotes Aspergillus (Genus), “FLA” flavus (Species), and ‘TOXIN’ means poisons [16]. The major identified aflatoxins are B1, B2, G1 and G2. The B1 is normally predominant in cultures and food products. The most toxic aflatoxin is B1 leads to mutagenesis [17–19]. AFB1 has been found a serious threat in countries where food safety and hygiene were not imposed strictly [20]. Pure AFB1 is a crystalline solid that ranges from pale white to yellow and is odorless. A. flavus secretes AFB1 and AFB2, while A. parasiticus produces AFG1, AFG2, AFB1, and AFB2 [21]. AFB1 toxicity is associated with severe hepato toxigenicity and carcinogenicity. Bio-transformations of AFB1 in the liver through cytochrome P450 enzymes cause detoxification [22]. The optimum temperature for the growth of aflatoxin is 20°C–35°C. Temperatures above 40°C and below 10°C reduce the production of these toxins. Higher temperatures outside the optimal range favor the production of aflatoxin B1, while lower temperatures favor aflatoxin G1 (AFG1) [23]. Life becomes risky due to contaminated foodstuffs [24] containing B1, B2, G1, and G2 aflatoxins, which are responsible of spoilage numerous agricultural foods and feed products [25]. Rice is susceptible to the attack of fungus during harvesting in the humid season [26]. The combination of warm and damp environments is considered very conducive condition for the proliferation of toxigenic fungi that produce mycotoxins such as aflatoxin [27]. On the other hand, the consumption of unpolished rice increases nutritional excellence and health claims. However, improper drying, handling, packaging, storage, hygroscopic conditions, and transportation assist in aflatoxins production. Delays in drying cause moisture sustaining in it that ultimately leads to postharvest contamination [27,28]. Therefore, the current study involves the extraction (Chloroform) and quantification (TLC) of aflatoxins from both low- and high-grade rice samples, which were collected randomly from various regions. Furthermore, the Aspergillus species were phylogenetically identified from the unfit rice samples. The impact of toxicity of aflatoxins on public health along with the scarcity of data regarding aflatoxin contents in rice provided the needed base study to measure the concentration of aflatoxins in rice at local markets. This study aimed to address the concerns regarding the aflatoxin risk of rice (food) manufacturing and provide awareness to the public on highest quality rice and health issues posed by rice aflatoxins under guidelines outlined by local and international food safety standards.
Rice samples (n = 16) were selected randomly from 16 different locales of Punjab Pakistan, located at latitude of 31.17° N and longitude of 72.70° E. Eight samples of excellent quality taken from fertile locations and eight samples of low quality were taken from less fertile areas. The districts; Sialkot, Lahore, Narowal, Sheikhupura, Gujranwala, Hafizabad, Vehari, and Nankana Sahib for high-quality rice, whereas Chiniot, Mianwali, Jhang, Bhakkar, Khushab, Muzaffargarh, Rahim Yar Khan, and Layyah for low quality rice covered the sampling sites. High (low or minimum aflatoxins) and low (high aflatoxins) quality rice grades were given due to the presence of aflatoxins.
Pre-weighed an empty Petri dish and then weighed the petri dish with sample. Weigh the dish and sample (W1). After weighing, the Petri dish was placed in the oven at 100°C. Weigh the dish again (W2) after cooling it in a desiccator. The dish was placed again in the oven for more than 2 h and then weighed again. The procedure was repeated until constant reading was obtained [29] (Fig. 1).
Moisture (% )=Total weight (W1)−Dry weight(W2)Weight of sample×100
Figure 1: Moisture analysis of high white and low-quality rice
2.3 Culturing of Filamentous Species
Aspergillus species were isolated from seed samples through the agar plate method (ISTA, 1966). Nearly two hundred seeds from each sample were placed on plates with MEA (Malt extract agar) medium and incubated at room temperature or 30°C. The growth of Aspergillus species was observed after 6 days and further purified individually by subculturing on PDA slants. The pure culture of each fungal species was further identified by the ITS molecular method in laboratory of King Saud University with a specific voucher number (Table 1).
2.4 Molecular Physiology, Extraction of DNA and Sequence Alignment
A modified version of the Doyle and Doyle (1987) CTAB technique was utilized for the extraction of the DNA genome from the samples. Nuclear ribosomal ITS regions were used to identify fungal filamentous species. According to BioEdit ver. 7.2.5 (ITSIF & ITS4) in hall 1999, consensus was obtained while homology was performed using BLAST from the NCBI (National Center of Biotechnology Information). The Genbank site was used to download the literature and initial ITS outbreak dataset (BLAST) according to a previous study [30]. However, ClustalX 2.1 was used for alignment and editing of the sequences according to Larkin et al. 2007 [31] and Bioedit [32]. The Sequences were aligned to MAFFT v. 10 using http://mafft.cbrc.jp/alignment/server/index.html [33] (accessed on 30 September 2023). The position of alignment to establish was 596. A phylogenetic tree was built by using these sequences at maximum likelihood. Gibberella fujikuroi was accepted as an outgroup. Phylogeny was Performed in MEGA (ver. 10.0) in accordance with the previous report [34]. Bootstrap values are adjusted based on 1000 replicas. This phylogenetic tree is helpful in finding the exact position of fungi while less than 50% of replicates branches were collapsed.
In this study, aflatoxins in extracts of rice were estimated by comparing them to the standard official technique of AOAC method no. 977.16 [35]. Aflatoxins (B1, B2, G1, and G2) crystalline powder standards were purchased from ‘Sigma–Aldrich (St. Louis, MO, USA)’. Standards were prepared by dissolving 1 mg powder in 100 mL benzene:acetonitrile (98:2; v/v). Aflatoxins (B1, G1) working standard (1 μg/mL) was individually prepared by mixing 100 μL of individual stock solution (10 μg/mL) and 900 µL of ‘benzene: acetonitrile (98:2; v/v)’, while B2 and G2 standards prepared by mixing ‘0.5 μg/mL’ separately by adding 50 μL of stock solution (10 μg/mL) in 950 µL of benzene:acetonitrile (98:2; v/v).
2.6 Thin Layer Chromatography (TLC)
Pre–coated TLC plates of silica gel 60 (layer thickness 0.25 mm, 20 cm × 20 cm) were purchased from E. Merck (Germany). These plates were pre-washed for a surety that no additional compounds were present in them. Each rice sample was thoroughly mixed and ground into fine powder for experimental work via a Romer grinding mill. The 50 g of grounded sample was taken into a 500 mL conical flask, and 25 mL of water was added followed by adding the 25 g diatomaceous earth and 200 mL of chloroform. The flask was shaken for 30 min. The flask was removed from the shaker and allowed the sample to settle down. This agitated mixture was filtered and 50 mL CHCl3 was added and placed in a steam bath for evaporation. Dried extracts were solubilized in 100 μL benzene:acetonitrile (98:2; v/v) and vortexed. TLC plates were spotted immediately by this sample and standardized above from the baseline (1.5 cm above the base). The spot of 5 μL was applied on the TLC plate. Two TLC tanks were taken and 50 mL ether was added in one tank, while the second tank acted as a mobile phase filled up to 50 mL with acetone:chloroform (1:9) (v/v). The TLC plate was placed on one side of the hot plate and the solution was placed on TLC by the micro-syringe. The process was carried out on the hot plate to prevent the drop from spreading on a plate. In developing first tank, plates (up till half or 3/4th solvent run) were developed with anhydrous ether for removal of impurities followed by removal of the plate for air drying. The plate was placed in the second tank upon drying. This was known as mobile phase. After the completion of this phase, the plate was taken out and again dried. Re-develop the plates in the same direction through an acetone-chloroform mixture in a TLC tank to modify Rf value of aflatoxins. The developed plates were observed under UV (λ = 254 and 366 nm) light for the presence or absence of spots of the test solution and authentic aflatoxin [29].
2.7 Quantitative Determination of Aflatoxin
Approximately, all spots of the same sizes of test and authentic standard aflatoxin corresponding to aflatoxins observed on preliminary plates were noticed.
The concentration of aflatoxins was determined by following common formula:
B1,B2,G1,G2(μg/kg)=Matchingofstandardspot×intensity×dilutionvol.ofsample×effectiveweight
All the experiments were performed in triplicates. The mean square was used to determine the significant difference (p < 0.05) among samples and presented as mean ± standard deviation (GraphPad Prism5 software Version 5.03). PCA (principal component analysis) was used to analyse the effect of low- and high-quality rice on aflatoxins content. PCA matrices were divided into 16 rows and 3 columns for the statistical analysis of data. An optimum number of components was determined using Cattel’s criterion. The input matrices were automatically scaled and measured at significant levels of a = 0.05% (correlation and PCA).
Rice is a staple food in most of the Asian countries. The requirement depends on quality, vendors and price. The consumer preferred contaminant-free rice. However, random purchase of rice showed contamination of aflatoxins in this present study.
Moisture plays an important role in the contamination of rice and stimulates AFs. The moisture level was determined by the AOAC method [35] (Fig. 1). The healthy rice contained less moisture. It was considered fit because it did not support the growth of Aspergillus flavus and A. parasiticus. Infected rice demonstrated a high value of moisture that favored the fungal growth. The results of our study were in accordance with Oyedele and Adeoti [36].
3.2 Identification of Aspergillus Species
Aspergillus species were identified by using ITS regions. The phylogenetic tree determined the exact position of these filamentous fungi (Fig. 2).
Figure 2: Phylogram of A. flavus and A. parasiticus identified from Low- and High-Quality rice contaminated with aflatoxins (R1-Long-grain brown rice, R2-Brown basmati rice, R3-Basmati-198, R4-Medium-grain brown rice, R5-Kalijira rice grains)
3.3 Determination of Aflatoxin
Aflatoxin is a major contaminant of rice due to high moisture required for its growth. The results were checked in the rice samples by the standard method of TLC by AOAC (Tables 1, 2). The results were similar to the study of Asghar et al. [27]. The detectable limit of aflatoxin is ≥1 μg/kg. Those rice samples were fit for human consumption as per MTL (<20 μg/kg) assigned by the USA (FDA and FAO) and Pakistan (PSQCA).
3.4 Aflatoxin in Low Quality Rice
The rice sample of low quality was highly contaminated (Table 1). In this study, four rice samples were found unfit for human consumption owing to AFs contamination in the range of 23.1 to 29.3 μg/kg including Kalijira rice, Long-grain brown rice, Brown basmati rice, and Medium-grain brown rice contaminated with aflatoxins and proved unfit for health. The highest value of AFB1 and AFB2 in a rice sample was 29.3 to 1.52 µg/kg in the case of Long-grain brown rice, respectively, higher than the standard value (>2.0). Kalijira rice (24.9 ± 0.01 µg/kg), Brown basmati rice (23.1 ± 0.02 µg/kg), and Medium-grain brown rice (25.9 ± 1.6 µg/kg) showed a significantly high amount of AFB1 with respect to Aromatic jasmine rice, Nutty-sweet red rice, Haiga-mai, and Short grain rice samples.
3.5 Aflatoxin in High Quality Rice
The high-quality rice was found to be good for health because of comparatively less contaminated (Table 2). The highest value of aflatoxins was found in Basmati-198, which was 22.3 µg/kg higher than the standard value. Five samples were contaminated with AFs but proved fit for consumption.
The results of the PCA analysis determined the relationship between low and high-quality rice and the content of aflatoxins. Three new variables were found, and the first two main components were described as much as 99.91% variability of the system. PC1 showed the largest percentage of system variability at 76.28%, while the second principal component PC2 described the system variability at 23.64%. As you can see, all parameters have a large impact on variability of the system, because these were placed in the red circle (Fig. 3A). The first principal component distinguished the fit and unfit form of rice due to the presence or absence of fungal species. Positive PC1 values exhibited the fit rice and no fungus occurrence, while negative values of this component described the unfit rice with fungus occurrence. A strong positive correlation was found between B1 and the total, and a slightly weaker but positive correlation between them and B2. The PCA analysis explained the negative values of the first principal component (PC1) and described the occurrence of aflatoxins, while the second principal component exhibited their types (Fig. 3B). The second principal component PC2 distinguished one unfit Long-grain brown rice variety from other unfit rice varieties. The B1, B2, and Total parameters were correlated with the unfit rice type and the occurrence of fungal species. Parameter B2 characterized the unfit Long-grain brown rice, and the remaining parameters B1 and totally characterized the remaining unfit varieties (Fig. 3A and B). The effects of aflatoxin in a correlation matrix were shown in Fig. 4. Rice quality based on aflatoxins was also analyzed by PCA. The collinearity of the variables was estimated by the determinant of the correlation matrix (−1 to 0 to +1). The 0 showed a weaker correlation while 1 exhibited a strong correlation. Negative and positive signs dictated the direction of correlation.
Figure 3: Loading plot (A) and score plot (B) of the principal component analysis (PC1, PC2) carried out for treatments and research parameters
Figure 4: Correlation matrix for the tested parameters of the effect of aflatoxin content
Many countries have established standard levels for aflatoxins (AFs) because of their high prevalence, risk, and toxicity to human health. For example, in the European Union (EU), the maximum level of about 4 μg/kg total has been set for aflatoxins (mainly AFB1, AFB2, AFG1, and AFG2) in cereals and AFB1 of about 2 μg/kg [37]. However, the maximum level of aflatoxins in rice up to standard was implied to be 20 μg/kg in USA [38] FDA, FAO, and PSQCA [38,39]. A previous study indicated the concomitant absence of AFB2, AFG1, and AFG2 [40]. Similarly, the rice contaminating A. flavus in our study produced one type of AF. These results were in contrast to previous studies indicating the ability of A. flavus strains to produce only AFB1 and AFB2 [40–42]. However, our results were consistent with the production of AFB1 by A. parasiticus strains [43]. It has also been reported that a high percentage of A. flavus strains synthesize AFG1, while a minor group accumulates AFG2 [44]. The results of this study are consistent with studies indicating that AFB1 is the most prevalent AF contaminant and has the highest concentration among rice-infecting A. flavus [45,46]. Firdous et al. [47] evaluated aflatoxin content in super kernel basmati rice. They determined the toxicity of AFB1 in 13.3% of samples ranged from 1.1–32.9 µg kg−1, while up to 1.0–8.1 µg kg−1 of 1.9% of AFB2 toxins were found. Only one sample showed AFG1. In basmati rice, contamination of AFB1 was 1.0–15.4 µg kg−1 in 18.3% of samples while 2% of AFG1 was also found. This study indicated the four unfit rice samples viz., Long-grain brown rice, Brown basmati, and White basmati-198 due to the presence of AFB1 the values of 29.3, 23.1 and 22.3 µg kg−1 rice, respectively. Whereas, AFB2 was undetectable in these samples. The filamentous fungal species identified in these rice samples was A. flavus, which was also reported in similar work of Nayak et al. [48]. In their study, 50% A. flavus and 55% A. flavus were observed in raw rice samples, which revealed the higher level of contamination in rice and rice-based commodities. Similarly, Asghar et al. [27] showed 95.4% AFB1 (1.07–24.65 µg/kg) in 250 samples and 7.6% AFB2 (0.52–2.62 µg/kg) in 20 brown rice samples, similar trend was found in our study where aflatoxins AFG1 and AFG2 were unidentified. Likewise, in another study, the Tehran retail market determined AFB1 (4.17 ng/g) in rice, and the level of contamination was >5 ng/g [49]. Asghar et al. [50] performed an experiment on export–quality basmati rice for AFs (B1 B2 G1 and G2) and detected 73.3% AFB1 (1.17 to 6.91 µg/kg) contamination in 2047 samples with the maximum tolerated level at 2 µg/kg. The AFB1 level in 2.3% of samples ranged from 2.05–3.36 µg/kg, 0.44% AFB1 up to 4.07–6.91 μg/kg range AFB1, while the remaining samples (26.7%) were within the detectable limit (>1 µg/kg). Tansakula et al. [51] also determined the aflatoxin (AF) B1, B2, G1, and G2 in 120 samples of unpolished rice and glutinous rice in Thai commodities. The total aflatoxins content determined in samples were 0.16 and 25.43 µg kg−1 levels of aflatoxins (particularly AFB1 and B2). Likewise, the district Mandi, Himachal Pradesh, India, was explored to determine the mycotoxins contamination in stored rice grains. The highest density and frequency of AFB1 and AFB2 was 28% and 48%, respectively, in 72% of the samples [52]. Therefore, this study aligned completely with the current study and undoubtedly witnessed the presence of Afs in the Brown basmati rice. Reddy et al. [53] performed tests on 1200 paddy rice samples. Aflatoxin (B1) concentrations up to 0.1–308.0 μg kg−1 were detected in 67.8% of rice. Fredlund et al. [54] tested 99 rice samples purchased from the retail market in Swedish for determination of aflatoxins. The conducted research has shown that as much as 71% of Basmati rice contained detectable levels of AFB1 up to 0.1 µg/kg of rice. The MTL of about 5 ng/g is assigned by the Institute of Standard and Industrial Research of Iran (ISIRI) and Mohammadi et al. [55] analyzed 4 types of AFs (B1, B2, G1, G2) in 152 samples of Iran. Their study showed contaminated levels of aflatoxin B1 (0.09–3.3 ng/g) in 75% of rice samples that were not above the MTL. The results of this study were totally against the findings of our study. The current study also indicated that Kalijira rice, Brown basmati rice, and Long and Medium grain brown rice from brown rice samples, whereas white Basmati-198 was contaminated with AFB1, and therefore, these rice samples were unfit for human consumption. Approximately, 200 samples of various varieties of rice were collected from Canada, Thailand, the United States, and India, which includes red, white, black, brown, jasmine, basmati and wild rice [56]. AFB1 with the incidence of 56% and 43% was found at levels of 0.19 and 0.17 ng g−1, respectively with 0.002 ng g−1 set as LOD (limit of detection). With a similar LOD, 23 samples found in the second year were contaminated with AFB2. AFB1 in both years was contaminated at levels of 1.44–7.14 ng g−1 and 1.45–3.48 ng g−1 in 5 samples, which are the most contaminated; this includes red and black rice taken from Thailand and basmati from India [57]. Reiter et al. [58] analyzed AFs in different varieties and fractions of a total of 81 samples of rice including whole, short, long grain rice, basmati rice, and puffed rice in Vienna. Results analysed a detectable number of aflatoxins in 24 samples out of the total in which 15 samples contained AFB1 and only one in AFB2, while B1 and B2 were found in a range of 0.45–9.86 and 1.5 μg kg−1, respectively. AFG1 and AFG2 was not determined in the samples. These samples exceeded the limits of AFB1 set by EU, e.g., one at a range of 2.16 μg kg−1, 2nd in the range of 2.85 μg kg−1 while 3rd was about 9.86 μg kg−1. The results of Reiter et al. [58] were contradictory to our findings as our study indicated the contamination of AFB1 in Long-grain brown rice, while short grains of rice were contaminated free from any type of aflatoxin. The results are also in close agreement as reported by Castells et al. [59] work, which studied two artificially contaminated varieties of husked rice with AFs. AFs of about 356–818 μg/kg and 244–645 μg/kg were found in medium-grain and long-grain rice, respectively. Yazdanpanah et al. [49] recommended the regular screening of AFB1-infected rice, because the consumption of a high quantity of AFB1 is toxic to the liver, cyclic nucleotide phosphodiesterase activity in the brain, liver, heart, and kidney tissues can be inhibited by AFB1. These all result in severe impairment of the metabolism of proteins, carbohydrates, and lipids. Previous studies have shown that rice grains are susceptible to AFs (AFB1 and AFB2) accumulation [60,61]. Both the present observations and previous results indicate that rice grains are a major source of AF contamination. These results confirm that unless appropriate steps are taken, the risk of AF poisoning in humans from rice infected by toxic A. flavus strains cannot be eradicated. Since rice consumption is common in many nations, it is highly advised to keep an eye on the levels of AFB1 in rice grains that have been stored as a preventive step [62]. The presence of AFs in the majority of the samples examined here suggests that regular, national-level programs to track the levels of AFs in rice grains are necessary. By using appropriate storage procedures, the concentration of AFs should be decreased [63].
The determination of fungal species associated with aflatoxin contamination in rice (Oryza sativa L.) is critical for ensuring food safety and public health. This study revealed that certain fungal species, particularly Aspergillus spp., were the primary culprits and responsible for the production of aflatoxins in rice. Identifying these fungi through advanced molecular and microbiological techniques not only helps in understanding the contamination pathways but also assists in developing effective prevention and control strategies. Given the global consumption of rice as a staple food, the presented study strongly endorses that mitigating aflatoxin contamination is essential for reducing the risk of liver cancer, immunosuppression, and other health problems linked to aflatoxin exposure. Future efforts should focus on improving agricultural practices, post-harvest handling, and storage conditions to minimize fungal growth and aflatoxin production, ensuring rice remains a safe and nutritious food source. Further research into biocontrol methods and genetic resistance in rice varieties could also offer sustainable solutions to this critical issue.
Acknowledgement: None.
Funding Statement: This work was supported by Princess Nourah bint Abdulrahman University Researchers Supporting Project Number (PNURSP2025R317), Princess Nourah bint Abdulrahman University, Riyadh, Saudi Arabia.
Author Contributions: Eman Alhomaidi: Formal analysis, writing—review & editing, resources, funding acquisition. Aisha Umar and Mustansar Mubeen: Investigation, methodology, data curation, writing original manuscript, writing—review & editing, validation, finalization. Laurent Dufossé: Conceptualization, writing—review & editing. Yasir Iftikhar: Software, writing—review & editing. Arpita Das and Soumya Ghosh: Visualization, writing—review & editing. Muhammad Sibt-e-Abbas: Project administration, writing—review & editing. All authors reviewed the results and approved the final version of the manuscript.
Availability of Data and Materials: The data will be available on request.
Ethics Approval: Not applicable.
Conflicts of Interest: The authors declare no conflicts of interest to report regarding the present study.
References
1. Debangshi U, Ghosh P. Rice wheat cropping systems-constraints and strategies: a review. Plant Arch. 2022;22(1):09725210. doi:10.51470/PLANTARCHIVES.2022.v22.no1.004. [Google Scholar] [CrossRef]
2. Sharabiani VR, Khorramifar A, Karami H, Lozano J, Tabor S, Darvishi Y, et al. Non-destructive test to detect adulteration of rice using gas sensors coupled with chemometrics methods. Int Agrophys. 2023;37:235–44. doi:10.31545/intagr/166009. [Google Scholar] [CrossRef]
3. Zaresani H, Sayyah AHA, Zareiforoush H, Khorramifar A, Gancarz M, Tabor S, et al. Vis/NIR and FTIR spectroscopy supported by machine learning techniques to distinguish pure from impure Iranian rice varieties. Int Agrophys. 2024;38:203–11. doi:10.31545/intagr/185392. [Google Scholar] [CrossRef]
4. Ahmad H, Zafar SA, Naeem MK, Shokat S, Inam S, Rehman MAU, et al. Impact of pre-anthesis drought stress on physiology, yield-related traits, and drought-responsive genes in green super rice. Front Genet. 2022;13:832542. doi:10.3389/fgene.2022.832542. [Google Scholar] [PubMed] [CrossRef]
5. Kaimba GK, Mithöfer D, Muendo KM. Commercialization of underutilized fruits: baobab pulp supply response to price and non-price incentives in Kenya. Food Policy. 2021;99:101980. doi:10.1016/j.foodpol.2020.101980. [Google Scholar] [CrossRef]
6. Prodhan ZH, Samonte SOP, Sanchez DL, Talukder SK. Profiling and improvement of grain quality traits for consumer preferable basmati rice in the United States. Plants. 2024;13(16):2326. doi:10.3390/plants13162326. [Google Scholar] [PubMed] [CrossRef]
7. Wimonmuang K, Lee YS. Absolute contents of aroma-affecting volatiles in cooked rice determined by one-step rice cooking and volatile extraction coupled with standard-addition calibration using HS-SPME/GC-MS. Food Chem. 2024;440:138271. doi:10.1016/j.foodchem.2023.138271. [Google Scholar] [PubMed] [CrossRef]
8. Irshad MS, Xin Q, Arshad H. Competitiveness of Pakistani rice in international market and export potential with global world: a panel gravity approach. Cogent Econ Financ. 2018;6:1486690. doi:10.1080/23322039.2018.1486690. [Google Scholar] [CrossRef]
9. Ali S, Murtaza WAMI, Khan A, Hamdullah SAS. Determinants of rice yield in central Khyber Pakhtunkhwa, Pakistan. Sarhad J Agric. 2022;38(1):117–27. [Google Scholar]
10. Hassan MIU, Irum A, Ashraf F, Hassan Z, Waqar M, Peerzado MB, et al. Evolution of breeding research in Basmati Rice (Oryza sativa L.) in Punjab, Pakistan. Biol Clin Sci Res J. 2024;683. doi:10.54112/bcsrj.v2024i1.683. [Google Scholar] [CrossRef]
11. Singh BK, Delgado-Baquerizo M, Egidi E, Guirado E, Leach JE, Liu H, et al. Climate change impacts on plant pathogens, food security and paths forward. Nat Rev Microbiol. 2023;21:640–56. doi:10.1038/s41579-023-00900-7. [Google Scholar] [PubMed] [CrossRef]
12. Xu J, Jiang M, Wang P, Kong Q. The Gene vepN regulated by global regulatory factor veA that affects aflatoxin production, morphological development and pathogenicity in Aspergillus flavus. Toxins. 2024;16(4):174. doi:10.3390/toxins16040174. [Google Scholar] [PubMed] [CrossRef]
13. Mato L, Damani Z, Spahiu J, Halimi E, Seiti B, Topi D. High prevalence of mycotoxigenic fungi and Aflatoxin B1 contamination in corn and wheat grains grown to albania: implications for food safety. J Food Qual Hazards Control. 2024. doi:10.18502/jfqhc.11.1.14996. [Google Scholar] [CrossRef]
14. Lizárraga-Paulín EG, Moreno-Martínez E, Miranda-Castro SP. Aflatoxins and their impact on human and animal health: an emerging problem. Aflatoxins-Biochem Mol Biol. 2011;13:255–62. doi:10.5772/26196. [Google Scholar] [CrossRef]
15. Moutia I, Lakatos E, Kovács AJ. Impact of dehydration techniques on the nutritional and microbial profiles of dried mushrooms. Foods. 2024;13(20):3245. doi:10.3390/foods13203245. [Google Scholar] [PubMed] [CrossRef]
16. Barontini JM, Zanon MSA, Druetta MA, Posse AMR, Torrico AK, del Pilar Monge M, et al. Differential response of maize hybrids to field infection with Aspergillus flavus and aflatoxin accumulation in the Chaco Semi-arid region of Argentina. Crop Prot. 2022;156:105960. doi:10.1016/j.cropro.2022.105960. [Google Scholar] [CrossRef]
17. Doménech E, Martorell S. Review of the terminology, approaches, and formulations used in the guidelines on quantitative risk assessment of chemical hazards in food. Foods. 2024;13(5):714. doi:10.3390/foods13050714. [Google Scholar] [PubMed] [CrossRef]
18. Lyon F. IARC monographs on the evaluation of carcinogenic risks to humans, World health organization, International agency for research on cancer; 2014 [cited 2025 Jan 10]. Available from: publication@iarc.fr. [Google Scholar]
19. Raghoonundon B, Raspé O, Thongklang N, Hyde KD. Phlebopus (Boletales, Boletinellaceaea peculiar bolete genus with widely consumed edible species and potential for economic development in tropical countries. Food Biosci. 2021;41:100962. doi:10.1016/j.fbio.2021.100962. [Google Scholar] [CrossRef]
20. Więckowska M, Cichon N, Szelenberger R, Gorniak L, Bijak M. Ochratoxin A and its role in cancer development: a comprehensive review. Cancers. 2024;16(20):3473. doi:10.3390/cancers16203473. [Google Scholar] [PubMed] [CrossRef]
21. Mdindikasi Z, Mwamahonje A, Mpore FJ, James A, Mahinda A. Management practices to mitigate aflatoxin contamination in groundnut: a review. Cogent Food Agric. 2024;10(1):2366383. doi:10.1080/23311932.2024.2366383. [Google Scholar] [CrossRef]
22. Roberts AG, Stevens JC, Szklarz GD, Scott EE, Kumar S, Shah MB, et al. Four decades of Cytochrome P450 2B research: from protein adducts to protein structures and beyond. Drug Metab Dispos. 2023;51(1):111–22. doi:10.1124/dmd.122.001109. [Google Scholar] [PubMed] [CrossRef]
23. Balan B, Dhaulaniya AS, Kumar M, Kumar M, Kumar P. Aflatoxins in food: prevalence, health effects, and emerging trends in its mitigation—an updated review. Food Saf Health. 2024;2(1):39–71. doi:10.1002/fsh3.12030. [Google Scholar] [CrossRef]
24. Chang J, Luo H, Li L, Zhang J, Harvey J, Zhao Y, et al. Mycotoxin risk management in maize gluten meal. Crit Rev Food Sci Nutr. 2024;64(22):7687–706. doi:10.1080/10408398.2023.2190412. [Google Scholar] [PubMed] [CrossRef]
25. Zhang K, Banerjee K. A review: sample preparation and chromatographic technologies for detection of aflatoxins in foods. Toxins. 2020;12(9):539. doi:10.3390/toxins12090539. [Google Scholar] [PubMed] [CrossRef]
26. Wu Q, Zhu Y, Fang C, Wijffels RH, Xu Y. Can we control microbiota in spontaneous food fermentation?–Chinese liquor as a case example. Trends Food Sci Technol. 2021;110:321–31. doi:10.1016/j.tifs.2021.02.011. [Google Scholar] [CrossRef]
27. Asghar MA, Iqbal J, Ahmed A, Khan MA. Occurrence of aflatoxins contamination in brown rice from Pakistan. Iran J Public Health. 2014;43:291. [Google Scholar] [PubMed]
28. Trucksess M, Abbas H, Weaver C, Shier W. Distribution of aflatoxins in shelling and milling fractions of naturally contaminated rice. Food Addit Contam: Part A. 2011;28:1076–82. doi:10.1080/19440049.2011.576441. [Google Scholar] [PubMed] [CrossRef]
29. AOAC. Official methods of analysis. 19th ed. Arlington, VA, USA: Association of Official Analytical Chemists; 2012. [Google Scholar]
30. Benson DA, Cavanaugh M, Clark K, Karsch-Mizrachi I, Lipman DJ, Ostell J, et al. GenBank. Nucleic Acids Res. 2016;45:D37–42. doi:10.1093/nar/gkw1070. [Google Scholar] [PubMed] [CrossRef]
31. Larkin MA, Blackshields G, Brown NP, Chenna R, McGettigan PA, McWilliam H, et al. Clustal W and Clustal X version 2.0. Bioinformatics. 2007;23:2947–8. doi:10.1093/bioinformatics/btm404. [Google Scholar] [PubMed] [CrossRef]
32. Hall TA. BioEdit: a user-friendly biological sequence alignment editor and analysis program for windows 95/98/NT. Oxford: Nucleic acids symposium series; 1999. p. 95–8. [Google Scholar]
33. Katoh K, Standley DM. MAFFT multiple sequence alignment software version 7: improvements in performance and usability. Mol Biol Evol. 2013;30:772–80. doi:10.1093/molbev/mst010. [Google Scholar] [PubMed] [CrossRef]
34. Tamura K, Peterson D, Peterson N, Stecher G, Nei M, Kumar S. MEGA5: molecular evolutionary genetics analysis using maximum likelihood, evolutionary distance, and maximum parsimony methods. Mol Biol Evol. 2011;28:2731–9. doi:10.1093/molbev/msr121. [Google Scholar] [PubMed] [CrossRef]
35. AOAC International. Method 971.22. 15th ed. Arlington, TX, USA: Association of Official Analytical Chemists; 2005. [Google Scholar]
36. Oyedele O, Adeoti O. Investigation into optimum moisture content and parboiling time for milling Igbemo rice. J Rice Res. 2013;1:101. doi:10.4172/jrr.1000101. [Google Scholar] [CrossRef]
37. E.C.R. (ECRAmending Regulation (EC) No 1881/2006. Setting maximum levels for certain contaminants in foodstuffs as regards flatoxins. Official J Eur Union. 2010;50:8–12. [Google Scholar]
38. Shabbir MA, Anjum FM, Khan MR, Nadeem M, Saeed M. Assessment of heavy metals and aflatoxin levels in export quality Indica rice cultivars with different milling fractions. Afr J Agri Res. 2013;8:3236–44. [Google Scholar]
39. Arshad MW, Moazzam M, Raziq MM, Ahmed W. Linking the willingness of smallholder dairy farmers to adopt minimum food safety and quality standards to the country’s export potential. Int J Food Sci Tech. 2023;58:5557–67. [Google Scholar]
40. Dhanasekaran D, Shanmugapriya S, Thajuddin N, Panneerselvam A. Aflatoxins and aflatoxicosis in human and animals. In: Aflatoxins-biochemistry and molecular biology. Ramón Gerardo Guevara-González, InTech; 2011. [Google Scholar]
41. Alwan N, Bou Ghanem H, Dimassi H, Karam L, Hassan HF. Exposure assessment of aflatoxin B1 through consumption of rice in the United Arab Emirates. Int J Environ Res Public Health. 2022;19(22):15000. doi:10.3390/ijerph192215000. [Google Scholar] [PubMed] [CrossRef]
42. Frisvad JC, Skouboe P, Samson RA. Taxonomic comparison of three different groups of aflatoxin producers and a new efficient producer of Aflatoxin B1, Sterigmatocystin and 3-Omethylsterigmatocystin, Aspergillus rambellii sp. nov. Syst Appl Microbiol. 2005;28:442–53. doi:10.1016/j.syapm.2005.02.012. [Google Scholar] [PubMed] [CrossRef]
43. González-Salgado A, González-Jaén T, Vázquez C, Patiño B. Highly sensitive PCR-based detection method specific for Aspergillus flavus in wheat flour. Food Addit Contam A. 2008;25:758–64. doi:10.1080/02652030701765715. [Google Scholar] [PubMed] [CrossRef]
44. Karaaslan M, Arslanğray Y. Aflatoxins B1, B2, G1, and G2 contamination in ground red peppers commercialized in Sanliurfa. Turkey Environ Monit Assess. 2015;187:184. doi:10.1007/s10661-015-4402-0. [Google Scholar] [PubMed] [CrossRef]
45. Lai X, Zhang H, Liu R, Liu C. Potential for Aflatoxin B1 and B2 production by Aspergillus flavus strains isolated from rice samples. Saudi J Boil Sci. 2015;22:176–80. doi:10.1016/j.sjbs.2014.09.013. [Google Scholar] [PubMed] [CrossRef]
46. Sun XD, Su P, Shan H. Mycotoxin contamination of rice in China. J Food Sci. 2017;82:573–84. doi:10.1111/1750-3841.13631. [Google Scholar] [PubMed] [CrossRef]
47. Firdous S, Ashfaq A, Khan SJ, Khan N. Aflatoxins in corn and rice sold in Lahore, Pakistan. Food Addit Contam: Part B. 2014;7:95–8. doi:10.1080/19393210.2013.851123. [Google Scholar] [PubMed] [CrossRef]
48. Nayak S, Dhua U, Samanta S. Occurrence of aflatoxigenic A. flavus in stored rice and rice based products of coastal Odisha, India. Int J Curr Microbiol Appl Sci. 2014;3:170–81. doi:10.1111/jfpp.14127. [Google Scholar] [CrossRef]
49. Yazdanpanah H, Zarghi A, Shafaati AR, Foroutan SM, Aboul-Fathi F, Khoddam A, et al. Analysis of aflatoxin B1 in Iranian foods using HPLC and a monolithic column and estimation of its dietary intake. Iran J Pharm Res. 2013;12:83–9. [Google Scholar] [PubMed]
50. Asghar MA, Iqbal J, Ahmed A, Shamsuddin ZA, Khan MA. Incidence of aflatoxins in export quality basmati rice collected from different areas of Pakistan. Sci, Tech and Dev. 2013;32(2):110–9. doi:10.4315/0362-028X.JFP-16-290. [Google Scholar] [CrossRef]
51. Tansakula N, Limsuwanc S, Böhmb J, Hollmannb M, Fazelid ER. Aflatoxins in selected Thai commodities. Food Addit Contam: Part B: Surveill. 2013;6(4):254–9. doi:10.1080/19393210.2013.812148. [Google Scholar] [PubMed] [CrossRef]
52. Gautam AK, Gupta H, Soni Y. Screening of fungi and mycotoxins associated with stored rice grains in Himachal Pradesh. Int J Theor Appl Sci. 2012;4:128–33. doi:10.18805/BKAP406. [Google Scholar] [CrossRef]
53. Reddy K, Reddy C, Muralidharan K. Detection of Aspergillus spp. and aflatoxin B1 in rice in India. Food Microbiol. 2009;26:27–31. doi:10.1016/j.fm.2008.07.013. [Google Scholar] [PubMed] [CrossRef]
54. Fredlund E, Thim A-M, Gidlund A, Brostedt S, Nyberg M, Olsen M. Moulds and mycotoxins in rice from the Swedish retail market. Food Addit Contam. 2009;26:527–33. doi:10.1080/02652030802562912. [Google Scholar] [PubMed] [CrossRef]
55. Mohammadi M, Mohebbi G, Hajeb P, Akbarzadeh S, Shojaee I. Aflatoxins in rice imported to Bushehr, a southern port of Iran. Am-Eurasian J Toxicol Sci. 2012;4:31–5. doi:10.5829/idosi.aejts.2012.4.1.56350. [Google Scholar] [CrossRef]
56. Iqbal SZ, Asi MR, Hanif U, Zuber M, Jinap S. The presence of aflatoxins and ochratoxin A in rice and rice products; and evaluation of dietary intake. Food Chem. 2016;210:135–40. doi:10.1016/j.foodchem.2016.04.104. [Google Scholar] [PubMed] [CrossRef]
57. Bansal J, Pantazopoulos P, Tam J, Cavlovic P, Kwong K, Turcotte A-M, et al. Surveys of rice sold in Canada for aflatoxins, ochratoxin A and fumonisins. Food Addit Contam. 2011;28:767–74. doi:10.1080/19440049.2011.559279. [Google Scholar] [PubMed] [CrossRef]
58. Reiter EV, Vouk F, Böhm J, Razzazi-Fazeli E. Aflatoxins in rice-a limited survey of products marketed in Austria. Food Control. 2010;21:988–91. doi:10.1016/j.foodcont.2009.12.014. [Google Scholar] [CrossRef]
59. Castells M, Ramos AJ, Sanchis V, Marín S. Distribution of total aflatoxins in milled fractions of hulled rice. J Agric Food Chem. 2007;55:2760–4. doi:10.1021/jf063252d. [Google Scholar] [PubMed] [CrossRef]
60. Boudjaber K, Miri YB, Benabdallah A, Bennia N, Hamadi C, Soumati B, et al. Evaluation of Antifungal and anti-aflatoxin B1 efficacy of some crude extracts of Chamaerops humilis L. against Aspergillus flavus isolated from peanuts (Arachis hypogea L.). Food Control. 2023;152:109858. [Google Scholar]
61. Kristiningrum E, Nurcahyo R, Susanto D, Isharyadi F, Mulyono AB, Anggraeni P, et al. Aflatoxin in rice: a publication review. IOP Conf Series: Earth Environ Sci. 2023;1133:012035. doi:10.1088/1755-1315/1133/1/012035. IOP Publishing. [Google Scholar] [CrossRef]
62. Phan LT, De Saeger S, Eeckhout M, Jacxsens L. Public health risk due to aflatoxin and fumonisin contamination in rice in the Mekong Delta, Vietnam. Food Saf Risk. 2023;10(1):4. doi:10.1186/s40550-023-00104-0. [Google Scholar] [CrossRef]
63. Monger A, Mongar P, Dorji T, Chhetri V. The occurrence and human health risk assessment of total and aflatoxin B1 in selected food commodities in Bhutan. Sci Rep. 2024;14(1):16258. [Google Scholar] [PubMed]
Cite This Article
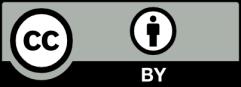
This work is licensed under a Creative Commons Attribution 4.0 International License , which permits unrestricted use, distribution, and reproduction in any medium, provided the original work is properly cited.