Open Access
ARTICLE
Salicylic Acid Improved the Growth of Soybean Seedlings by Regulating Water Status and Plant Pigments and Limiting Oxidative Injury under Salinity Stress
1 Department of Agronomy, Khulna Agricultural University, Khulna, 9100, Bangladesh
2 Institute of Plant Science and Resources, Okayama University, Kurashiki, 710-0046, Japan
3 Department of Agricultural Chemistry, Khulna Agricultural University, Khulna, 9100, Bangladesh
4 Department of Genetics and Plant Breeding, Khulna Agricultural University, Khulna, 9100, Bangladesh
5 Department of Biochemistry and Molecular Biology, Bangladesh Agricultural University, Mymensingh, 2202, Bangladesh
6 Department of Seed Science and Technology, Bangladesh Agricultural University, Mymensingh, 2202, Bangladesh
7 Department of Botany and Microbiology, College of Science, King Saud University, P.O. Box 2455, Riyadh, 11451, Saudi Arabia
8 Department of Botany, Hindu College Moradabad (Mahatma Jyotiba Phule Rohilkhand University Bareilly), Moradabad, 244001, India
9 Department of Biology, Faculty of Science, University of Tabuk, Tabuk, 71491, Saudi Arabia
10 Soil Science Division, Bangladesh Wheat and Maize Research Institute, Dinajpur, 5200, Bangladesh
* Corresponding Authors: Shahin Imran. Email: ; Akbar Hossain. Email:
(This article belongs to the Special Issue: Abiotic Stress Impacts on Plant Physiology and Their Alleviation)
Phyton-International Journal of Experimental Botany 2024, 93(9), 2251-2266. https://doi.org/10.32604/phyton.2024.055736
Received 05 June 2024; Accepted 14 August 2024; Issue published 30 September 2024
Abstract
Soybean (Glycine max) is a potential legume crop, but it cannot thrive in mild salinity. Salicylic acid (SA) is a renowned plant growth hormone that improves tolerance to saline conditions. Hence, the study was performed to understand the functions of priming seeds and supplementation of SA in modulating salt tolerance in soybean seedlings. When exposed to salt stress, soybean seedlings showed considerably higher contents of hydrogen peroxide (H2O2) and malondialdehyde (MDA) while having decreased germination and growth factors, water contents, and photosynthetic pigments. The germination rate, final germination percentage, germination index, germination energy, and seed vigor index considerably improved while the mean germination time decreased in the SA-primed seeds. The results also revealed that SA supplementation increased seedling traits, leaf water content, chlorophyll, and carotenoids and lessened H2O2 and MDA content under salt stress. Germination of seeds, seedlings growth traits, plant pigments, H2O2, and MDA content with the NaCl and SA treatments were found to substantially interact with each other according to both hierarchical clustering and principal component analysis. Based on the results, SA might be used as a seed priming and exogenous chemical to assist soybeans grow faster under salinity stress.Keywords
Salinity is considered a critical issue for the growth and development of crops worldwide [1,2]. Munns and Tester [3] reported that approximately 2000 million acres of land are anticipated to be negatively impacted by salinity globally. Owing to its detrimental impact on agricultural plants’ ability to absorb water and reduce soil fertility, soil salinity is a developing threat worldwide [4].
Excessive salt creates serious problems and hampers the development and production of different plant species [5]. High salt concentrations lead to water scarcity, ionic toxicity, depleted photosynthesis, lower transpiration rates, and decreased stomatal conductance, which disrupt physiological processes and cause nutrient imbalances in plants [6–8]. Additionally, salt stress can result in leaf necrosis and stunted growth due to reduced water content and the accumulation of Na+ ions [9]. Furthermore, elevated Na+ levels hinder the formation of the root cell plasma membrane, leading to poor nutrient uptake by stunted roots [10]. Compared with other field crops, legumes are particularly susceptible to salt stress. The most important cultivated legume crop, soybean, is crucial to global agriculture and nutrition because of its versatile applications and nutritional value. They serve as a significant source of oil and protein, which are essential for human and animal consumption [11,12]. Cultivating of soybeans contributes to sustainable agricultural practices, including soil fertility improvement and crop rotation strategies [13]. Moreover, soybeans play a pivotal role in food security by providing ingredients for a wide array of products, from tofu and soy milk to animal feed and biodiesel [11]. According to previous reports, when the soil salinity exceeds 50 mM, soybean growth and production decrease, and at a soil salinity level of 80 mM, the advancement of the soybean life cycle is delayed, and seeds are not produced [14]. Recent research has shown that priming seeds or seedlings with some exogenous protective chemicals, including plant hormones, might considerably influence plant responses to certain abiotic challenges [15].
Salicylic acid (SA) is a frequently used plant growth stimulus that increases the growth and development of numerous crops when they are exposed to abiotic stress [16]. SA influences seed germination by modulating various physiological and biochemical processes. It improves germination rates and seedling vigor by regulating hormonal balances, such as increasing the levels of gibberellins and decreasing abscisic acid, which are critical under saline conditions [17]. Moreover, SA also enhances the activity of enzymes that break down seed storage reserves, thus providing necessary nutrients for germinating seeds [18]. During the seedling stage, SA plays a pivotal role in regulating water status, which is crucial in salt stress. Additionally, SA enhances the expression of aquaporins, which are proteins that facilitate water transport across cell membranes, thereby improving water uptake and retention in seedlings [19]. This regulation helps maintain cell turgor and prevents dehydration, which is essential for sustaining growth under salt stress. Moreover, SA significantly influences the stability and synthesis of plant pigments, particularly chlorophyll. Salinity stress often leads to chlorophyll degradation, impairing photosynthesis and energy production. However, SA application has been shown to preserve chlorophyll content by enhancing gene expression involved in chlorophyll biosynthesis and reducing the activity of chlorophyll-degrading enzymes [20]. This preservation of chlorophyll ensures that the photosynthetic machinery remains functional, thereby supporting growth and development even under stress conditions. In addition to water regulation and pigment stabilization, SA plays a pivotal role in mitigating the oxidative stress induced by salinity. Salinity stress raises the production of reactive oxygen species (ROS), which can cause oxidative injury to cellular components such as proteins, lipids, and nucleic acids. In contrast, SA enhances the activity of antioxidant enzymes, which work collectively to neutralize ROS and minimize oxidative injury [21]. This antioxidant defense mechanism is crucial for maintaining cellular integrity and function during stress. However, this comprehensive approach, which combines improved water management, pigment stabilization, and oxidative stress mitigation, underscores the vital role of SA in increasing germination of seeds and seedling growth under salinity stress. The supplementation of SA is increasingly recognized as a promising strategy to increase crop resilience and productivity in saline environments, offering a sustainable solution to the challenges posed by soil salinization [22].
Based on the above discussion and the importance of soybean, this research investigated the ability of SA to mitigate salinity stress on the germination of seeds and early seedling growth by regulating the leaf water status, plant pigments, and oxidative injury of soybean plants under saline conditions.
2.1 Study Location and Treatment Conditions
An experiment using Petri dishes and hydroponics was performed at the Agronomy Laboratory at Khulna Agricultural University, Khulna, from December 2022 to January 2023. The popular “BARI Soybea-5” type of high-yielding and moderately susceptible soybean was collected for the experiment from the Bangladesh Agricultural Research Institute, Gazipur, Bangladesh. The seeds (12% moisture content) were pre-treated with 1% NaOCl for 5 min to eliminate microorganisms from the seed surface. Seeds of soybean were primed with water or with 1- or 2-mM salicylic acid (SA) at the root temperature for 60 min. Thereafter, distilled water was used to wash the seeds. The seeds were dried back to their initial moisture content at room temperature. Based on preliminary screening results, the SA levels were chosen (Fig. A1). Based on previous research, 150 mM NaCl stress was applied [12,23]. For each treatment, thirty soybean seeds were used for priming and placed on 150 mm × 25 mm diameter Petri dishes. Three-layered tissue paper was used in the Petri dishes. Each dish was moistened with 5 mL of 150 mM NaCl for the salinity treatment and the 1- and 2-mM SA treatments, and one dish was filled with water (8 mL) for non-saline conditions. All the treatments consisted of three replications. The study included the following treatments: control (C), 1 mM SA (SA1), 2 mM SA (SA2), 150 mM NaCl (salt), 1 mM SA+150 mM NaCl (SA1+salt), and 2 mM SA+150 mM NaCl (SA2+salt).
2.2 Seed Germination Parameter Measurement
The germinated seeds were counted every 24 h from initial germination to the seventh day. The GR (germination rate), FGP (final germination %), MGT (mean germination time), GI (germination index), and GE (germination energy) were calculated from the obtained data as described previously [24–26]. Using plant height, the SVI (seed vigour index) was calculated [27]. The following formulas were used for the GR, FGP, MGT, GI, GE and SVI calculations:
where GP1 = germination percentage on the 1st day after sowing.
GPx = germination percentage on the xth day after sowing.
where N = No. of seeds on day Da.
Da = No. of days from the 1st germination.
where Tj1 = number of seeds germinated on the 1st day.
Nj = No. of total seeds.
SVI = final germination percentage × plant height (cm)
where plant height = length of shoot + length of root.
A modified hydroponic method was utilized for growing sprouted seeds in 3 L pots as previously described [23]. Each pot contained three seedlings. Thirty-day-old seedlings were subsequently exposed to a ten-day period of stress with 150 mM NaCl. During the period of ten days of stress, 1- or 2-mM SA was sprayed on the plants (sprayed every day at 10:00 and 22:00; 3 mL per spray per plant). Following ten days of SA spraying, one seedling per pot was used to measure the length of the roots (RL), fresh weight of the roots (RFW), length of the shoots (SL), fresh weight of the shoots (SFW), and relative water content (RWC). Fresh leaves were collected from another plant to analyse plant pigments, H2O2, and the MDA content. Upon completion of a 72-h drying period at 60°C, the root and shoot dry weight (RDW and SDW) were determined via an electric balance.
2.4 Estimation of Leaf Water Status
The remaining one seedling from per pot was used to measure the leaf water status. The relative water content (RWC) was calculated as previously described [28]. The fresh weight (FLW) of a single leaf per treatment was noted, and then the leaf was overwhelmed for 1 and 2 h in distilled water. The turgid weight (TLW) was quickly noted after all water was blotted with the same tissue. The dry weight (DLW) of the leaves was noted after 48 h of drying at 72°C. The following formula was used to calculate the RWC:
Subsequent to the relative water content calculation, the relative water loss (RWL) was derived via the bellow formula [29]:
2.5 Estimation of Photosynthetic Pigments
Approximately 500 mg of leaf material was put into a 15 mL tube containing 80% ethanol (10 mL). For pigment extraction, the tubes were left in darkness for 10 days. Photosynthetic pigments were quantified via spectrophotometers operated at 663, 645, and 480 nm (Shimadzu UV-2550, Kyoto, Japan) via the Ibiang et al. [30] and Ridley [31] methods:
Chlorophyll a = (Abs. at 663 × 0.999 − Abs. at 645 × 0.0989)
Chlorophyll b = {Abs. at 663 x (−0.328) + Abs. at 645 × 1.77}
Total Chlorophyll = Chlorophyll a + Chlorophyll b
Carotenoids = {(Abs. at 663 × 0.114 − Abs. at 645 × 0.638) + Abs. at 480}
2.6 Determination of H2O2 and MDA Contents
Malondialdehyde (MDA) and hydrogen peroxide (H2O2) were assessed in soybean seedlings as described by Zhang et al. [32] and Velikova et al. [33], respectively. A UV–VIS spectrophotometer with wavelengths of 532 and 390 nm (T80, PG Instruments, China) was used to measure the MDA and H2O2 contents, respectively.
The data were analysed via one-way ANOVA, and Tukey’s HSD test (p < 0.05) was employed to distinguish means. A heatmap was generated in R 4.2.3 via the ‘pheatmap’ package. Principal component analysis (PCA) was conducted via the ‘GGally’and ‘factoextra’ packages.
3.1 SA Supplementation Enhances the Germination Properties of Soybean under Salt Stress
The effects of priming seeds with SA on the germination metrics of soybean seeds under NaCl stress are presented in Fig. 1. The results demonstrated that the germination rate (GR) substantially decreased under salt condition compared with the control condition. However, the results revealed that SA1 and SA2 priming under salt stress substantially increased GR (Fig. 1A). In terms of the final germination percentage (FGP), salt stress substantially abated the FGP in comparison with that in the control condition (Fig. 1B). Compared with salinity stress, the seeds primed with SA substantially increased the FGP (Fig. 1B). Moreover, the mean germination time (MGT) substantially increased under condition of salinity stress compared with that under non-stress condition. The results revealed that MGT substantially decreased under the SA1+salt and SA2+salt conditions compared with the salt condition (Fig. 1C). Salinity stress substantially decreased the germination index (GI); however, SA1 and SA2 priming substantially increased the GI during salt stress (Fig. 1D). Similarly, priming with SA1 and SA2 substantially increased the germination energy (GE) under salt stress and control condition compared with that under condition of salt stress alone (Fig. 1E). In contrast to the salt stress scenario, priming with SA increased the seed vigor index (SVI), whereas salt stress drastically abated the SVI. Compared with that under salt stress, the SVI substantially increased for SA1+salt and SA2+salt (Fig. 1F). These findings demonstrated that, under conditions of salinity stress, SA2 resulted in greater germination metrics than did SA1.
Figure 1: Effects of NaCl and SA on the germination rate (A), final germination percentage (B), mean germination time (C), germination index (D), germination energy (E), and seed vigour index (F). The mean values of triplicate samples ± SEs are presented (n = 30). Treatments differences were determined via Tukey’s HSD (p < 0.05), with distinct letters indicating significance
3.2 SA Supplementation Boosts the Traits of Soybean Seedlings under Salt Stress
We measured the root length (RL), root fresh weight (RFW), root dry weight (RDW), shoot length (SL), shoot fresh weight (SFW), shoot dry weight (SDW), and plant height (PH) of the soybean seedlings to determine the consequences of NaCl stress and SA actions for stress mitigation (Table 1). Compared with non-stressed plants, salt-treated plants presented substantially reduced RL. Administering SA substantially increased RL in SA1+salt- and SA2+salt-treated plants. Moreover, RFW under condition of salt stress was substantially lower than that under the control condition, but supplementation with SA2 substantially increased RFW under salt condition. Moreover, the RDW was substantially greater in the SA1+salt- and SA2+salt-treated plants than in the salt-induced plants.
A substantial reduction in SL was noticed in salt-induced plants compared with non-stressed plants, and supplementation of plants with SA1 and SA2 substantially increased SL under salt condition. Additionally, SFW was substantially affected and reduced in salt-induced plants compared with non-stressed plants. Exogenous supplementation with SA1 and SA2 helped plants recover from injury and resulted in a substantial increase in SFW under salt condition. Moreover, the SDW was substantially increased by the application of SA1 and SA2 to the salt-stressed plants. Salt stress substantially affects PH. However, compared with salt conditions, supplementation with SA1 and SA2 substantially increased plant height under salt condition. The results also indicated that SA2 outperformed SA1 in terms of seedling growth traits under salt condition.
3.3 Effects of SA Supplementation on Leaf Water Status under Salt Stress
This study assessed RWC and RWL both in the presence and absence of NaCl using SA to determine the soybean leaf water status (Table 1). The results indicated that RWC was greatly decreased by salt addition relative to the control condition at both 1 and 2 h. The lowest RWC (46.677%) at 1 h and 44.636% at 2 h was measured for the salt-stressed plants compared with the non-stressed plants. However, during salt stress condition, the application of SA resulted in a substantial increase in RWC at both 1 and 2 h in the SA1+salt- and SA2+salt-treated plants compared with the salt-stressed plants. Alternatively, a substantial increase in RWL was also recorded in the salt-stressed plants at both 1 and 2 h. The highest RWL values (53.323%) at 1 h and 55.364% at 2 h were observed for the salt-treated plants, in contrast with those of the non-treated plants. Compared with those of salt-stressed plants, substantially lower RWLs were measured for SA1+salt- and SA2+salt-treated plants. These outcomes indicated that SA2 upheld better water status in soybean than did SA1 under salt condition.
3.4 SA Supplementation Enhances Plant Pigment and Lessens the Oxidative Stress of Soybean Subjected to Salt Stress
Significant differences in photosynthetic pigment levels were noticed as a result of salt stress (Fig. 2). The outcomes demonstrated that the chlorophyll a level was substantially lower in salt-treated plants than in non-stressed plants. However, SA supplementation substantially increased the chlorophyll a level in SA2+salt-treated plants compared with that in salt-induced plants (Fig. 2A). Compared with non-stressed plants, salt-stressed plants presented substantially lower pigment levels. In contrast, compared with the salt-induced plants, the SA1+salt- and SA2+salt-stressed plants presented substantially increased chlorophyll b contents (Fig. 2B). The total chlorophyll content was also substantially affected by salt stress relative to non-stressed conditions (Fig. 2C). Compared with salt-treated plants, SA supplementation substantially increased the total chlorophyll content in the leaves of the SA1+salt-treated and SA2+salt-treated plants (Fig. 2C). Moreover, the carotenoid content was substantially lower in salt-induced plants than in untreated plants. Despite these findings, SA1 and SA2 spraying resulted in substantially greater contents of carotenoids in the leaves of the soybean plants under salt condition than in those of the salt-stressed plants (Fig. 2D).
Figure 2: Effects of NaCl and SA on chlorophyll a (A), chlorophyll b (B), total chlorophyll (C), and carotenoids (D). The mean values of triplicate samples ± SEs are presented (n = 3). Treatments differences were determined via Tukey’s HSD (p <0.05), with distinct letters indicating significance
We subsequently investigated the effects of SA supplementation on alleviating the oxidative damage induced by NaCl in soybean leaves by measuring H2O2 and MDA contents. The findings of the present study revealed that, compared with non-treated plants, salt-treated plants substantially increased the production of H2O2 (Fig. 3A). Conversely, SA supplementation of salt-induced plants hindered the onset of oxidative injury, as evidenced by substantially lower levels of H2O2 in SA1+salt- and SA2+salt-treated plants than in salt-stressed plants. (Fig. 3A). Under salt stress, the MDA content in the leaves of soybean plants significantly increased. In contrast, the SA1+salt- and SA2+salt-stressed plants presented considerably lower MDA levels (Fig. 3B). The upper results also revealed that SA2 supplementation raised the photosynthetic pigments and decreased the H2O2 and MDA contents much more than SA1 supplementation did under saline condition.
Figure 3: Effects of NaCl and SA on the H2O2 (A) and MDA (B) contents of soybean. The mean values of triplicate samples ± SEs are presented (n = 3). Treatments differences were determined via Tukey’s HSD (p < 0.05), with distinct letters indicating significance
3.5 Heatmap and PCA-Based Interaction Estimation for Treatment-Variables
The average values of the studied parameters were employed to generate a heatmap and perform principal component analysis (PCA) (Fig. 4). Along the variable axis, two groups (Clusters a and b) were identified via hierarchical clustering (Fig. 4A). Cluster-a consists of the H2O2, RWL2, RWL1, MDA, and MGT parameters. The Cluster-a parameters tended to increase in the salt-stressed plants, with a downwards trend observed in the C, SA1, SA2, SA1+salt, and SA2+salt-stressed plants. Cluster b included the variables SVI, GI, FGP, SFW, RL, RDW, PH, SL, RWC2, RWC1, Caro, RFW, TChl, SDW, Chl b, GE, and Chl a. The Cluster b factors tended to increase in C, SA1, SA2, SA1+salt, and SA2+salt-stressed plants but decreased in salt-stressed plants. In addition, PCA was conducted to analyse the relationships among the studied parameters and the treatments (Fig. 4B). The PCA scores were divided into six treatments according to their PC1 and PC2 positive and negative values. PC1 together with PC2 collectively represented 97.32% of the data variability across the treatments and all of the soybean seedling parameters investigated. In this scenario, PC1 accounted for 93.79% of the data variability and separated C, SA1, and SA2 from salt and SA1+salt and SA2+salt treatments on the basis of their positive and negative PCA scores (Fig. 4B). Furthermore, PC2 showed only 3.53% data variability (Fig. 4B).
Figure 4: Interaction estimation for treatment-variables. (A) Heatmap and (B) PCA results
Salinity is a vital abiotic factor that negatively influences the germination process, seedling development and, ultimately, crop yield. Many studies have confirmed that increasing salinity levels negatively impact germination and cause significant damage during various stages of plant growth [34,35]. However, SA functions as a signalling molecule that affects several reactions of physiological processes during seed germination [36]. This study was conducted to assess the impacts of SA priming on soybean seeds exposed to salinity stress. The observations revealed a substantial decline in germination properties and seedling growth traits due to salt stress (Fig. 1; Table 1). The findings of the present study demonstrated that SA priming and supplementation substantially increased the GR, FGP, GI, GE, SVI, RL, RFW, RDW, SL, SFW, SDW, and PH of soybean plants under salt stress. In our current investigation, priming with SA significantly reduced the mean germination time (MGT), highlighting its effectiveness in mitigating the adverse effects of salinity on germination. Shakirova et al. [37] studied wheat seed priming and reported a greater germination rate and better seedling development under SA treatment. Moreover, Ceritoğlu et al. [38] reported that chickpea seeds primed with SA under salinity presented better germination attributes. Other studies have critically evaluated the functions of SA pre-treatment in increasing crop growth under salinity stress conditions [39,40]. Additionally, Anaya et al. [41] reported that when Vicia faba seeds were treated with SA in conjunction with NaCl stress, there was an increase in total germination and a decrease in MGT. Previous studies have revealed that SA supplementation substantially improved kidney bean growth traits under saline conditions [42]. Moreover, research has indicated that SA supplementation leads to increases in both the height and dry weight of wheat plants [43]. Additionally, the heatmap analysis in the present study revealed that SA priming and supplementation enhanced the germination of seeds and growth traits (Fig. 4A), and PCA revealed a strong association between germination and seedling traits (Fig. 4B).
Ensuring adequate water content during salt stress is essential for maintaining proper plant growth [42], and RWC is an essential factor of plant water status [44]. Research indicates that salinity lowers the RWC and increases the RWL in plants. On the other hand, SA supplementation has been found to significantly increase RWC in kidney beans subjected to salinity stress [42]. The present results revealed that salinity lowered the RWC and increased the RWL (Table 1), which was attributed to structural injury to the cell wall that interferes with proper water uptake [45]. The present findings also indicated that SA supplementation substantially increased RWC and decreased RWL in soybean (Table 1). The results of the present study are consistent with earlier findings that SA application increased RWC and decreased RWL in baby corn [46] and stevia [47].
The chlorophyll content plays an essential role in photosynthesis. When plants experience stress, their photosynthetic pigment levels decrease [20]. In our study, the results demonstrated that salinity substantially lowered the pigment content in soybean leaves relative to that in the control condition. Kordrostami et al. [48] reported such a reduction in pigment content due to increased activity of the chlorophyllase enzyme, which inhibits chlorophyll synthesis. However, our results demonstrated that the application of SA under salt stress led to a significant increase in both chlorophyll and carotenoid levels (Fig. 2). This occurred because SA might support photosynthesis by protecting chloroplast pigments from the toxicity caused by salinity [49]. Several studies have also reported that SA application improved the chlorophyll content in wheat [50], kidney bean [42], and mung bean [51]. The heatmap and PCA also revealed their interaction with treatment and stress conditions (Fig. 4). SA2 was more effective than SA1 in increasing pigments during salt stress.
The results of the current study demonstrated that salinity stress accelerated oxidative injury in soybean plants with increased H2O2 and MDA contents. Oxidative injury is caused by excess ROS production under different environmental stresses [52]. As observed in this study, SA supplementation hindered the onset of oxidative injury, as illustrated by the substantial reduction in H2O2 and MDA levels under salinity stress (Fig. 3). Several studies reported similar reductions in H2O2 and MDA via SA application under salt stress conditions in Dianthus superbus [53], black bean [54], and mungbean [51]. However, SA might raise the activity of various enzymatic and nonenzymatic antioxidants, helping to decrease damage caused by stress-induced ROS and MDA, thereby improving plant tolerance to stress [55]. Collectively, the outcomes of the present study revealed that 2 mM SA outperforms 1 mM SA in enhancing germination, seedling growth traits, and physiological parameters and reducing oxidative injury under stressful or non-stressful conditions. Based on the current findings, we constructed a simplified flowchart of SA-mediated regulation of morphological, physiological and biochemical attributes under salinity (Fig. 5). Moreover, to validate these results, large-scale experiments at the field level are recommended.
Figure 5: A simplified flowchart of SA-mediated regulation of morphophysiological and biochemical attributes under salt stress
Our findings demonstrated that salinity stress reduced the germination and seedling growth characteristics, RWC, and photosynthetic pigments of soybeans while increasing the mean germination time and RWL, H2O2, and MDA contents. SA seed priming and supplementation improved the germination and seedling growth characteristics, RWC, and photosynthetic pigments of soybean plants during salt stress. In addition, SA decreased H2O2 and MDA contents in leaves and reduced oxidative injury in soybean plants. These findings suggest that 2 mM SA is more effective at minimizing the impacts of salinity and encouraging plant growth by controlling the leaf water status, photosynthetic pigments, H2O2 content, and MDA content in soybeans.
Acknowledgement: The authors extend their appreciation to the Researchers Supporting Project Number (RSP2025R385), King Saud University, Riyadh, Saudi Arabia.
Funding Statement: This research was funded by the Ministry of Science and Technology, Bangladesh, for providing financial support to carry out this work (No. 39.0000.012.002.07.21.62, Reg. No. 17). This project was supported by Researchers Supporting Project Number (RSP2025R385), King Saud University, Riyadh, Saudi Arabia.
Author Contributions: Methodology: Shahin Imran and Mohammad Saidur Rhaman; experiment conduct: Shahin Imran, Md. Asif Mahamud, and Newton Chandra Paul; formal analysis: Shahin Imran, Saleh H. Salmen, Sulaiman Ali Alharbi, Mohammad Javed Ansari, Mohammed Ali Alshehri, and Akbar Hossain; writing—original draft preparation: Shahin Imran, Md. Asif Mahamud, Newton Chandra Paul, Prosenjit Sarker, and Nazmul Islam; writing—review and editing: Shahin Imran, Md. Tahjib-Ul-Arif, Shahin Imran, Saleh H. Salmen, Sulaiman Ali Alharbi, Mohammad Javed Ansari, Mohammed Ali Alshehri, Akbar Hossain, and Mohammad Saidur Rhaman; supervision: Shahin Imran, Saleh H. Salmen, Sulaiman Ali Alharbi, Mohammad Javed Ansari, Mohammed Ali Alshehri, and Akbar Hossain; funding acquisition: Shahin Imran, Saleh H. Salmen, Sulaiman Ali Alharbi, Mohammad Javed Ansari, Mohammed Ali Alshehri, and Akbar Hossain. All authors reviewed the results and approved the final version of the manuscript.
Availability of Data and Materials: Data may be available upon request to the corresponding author.
Ethics Approval: Not applicable.
Conflicts of Interest: The authors declare that they have no conflicts of interest to report regarding the present study.
References
1. Singh A. Soil salinity: a global threat to sustainable development. Soil Use Manag. 2022;38(1):39–67. doi:10.1111/sum.12772. [Google Scholar] [CrossRef]
2. Rabbi RHM, Aktar N, Mahamud MA, Paul NC, Halder D, Imran S. Impact of different zinc concentrations on growth, yield, fruit quality, and nutrient acquisition traits of tomato (Lycopersicon esculentum L.) grown under salinity stress. Arch Biol Sci. 2024;76(1):71–82. doi:10.2298/ABS240101003R. [Google Scholar] [CrossRef]
3. Munns R, Tester M. Mechanisms of salinity tolerance. Annu Rev Plant Biol. 2008;59(1):651–81. doi:10.1146/annurev.arplant.59.032607.092911. [Google Scholar] [PubMed] [CrossRef]
4. Yuvaraj M, Bose KSC, Elavarasi P, Tawfik E, Meena RS, Datta R. Soil salinity and its management. In: Meena RS, Datta R, editors. Soil moisture importance. London, UK: IntechOpen; 2021. p. 109. doi:10.5772/intechopen.93329. [Google Scholar] [CrossRef]
5. Petretto GL, Urgeghe PP, Massa D, Melito S. Effect of salinity (NaCl) on plant growth, nutrient content, and glucosinolate hydrolysis products trends in rocket genotypes. Plant Physiol Biochem. 2019;141:30–9. doi:10.1016/j.plaphy.2019.05.012. [Google Scholar] [PubMed] [CrossRef]
6. Dos Santos TB, Ribas AF, de Souza SGH, Budzinski IGF, Domingues DS. Physiological responses to drought, salinity, and heat stress in plants: a review. Stresses. 2022;2(1):113–35. doi:10.3390/stresses2010009. [Google Scholar] [CrossRef]
7. Malakar P, Chattopadhyay D. Adaptation of plants to salt stress: the role of the ion transporters. J Plant Biochem Biot. 2021;30(4):668–83. doi:10.1007/s13562-021-00741-6. [Google Scholar] [CrossRef]
8. Rahneshan Z, Nasibi F, Moghadam AA. Effects of salinity stress on some growth, physiological, biochemical parameters and nutrients in two pistachio (Pistacia vera L.) rootstocks. J Plant Interact. 2018;13(1):73–82. doi:10.1080/17429145.2018.1424355. [Google Scholar] [CrossRef]
9. Acosta-Motos JR, Ortuño MF, Bernal-Vicente A, Diaz-Vivancos P, Sanchez-Blanco MJ, Hernandez JA. Plant responses to salt stress: adaptive mechanisms. Agronomy. 2017;7(1):18. doi:10.3390/agronomy7010018. [Google Scholar] [CrossRef]
10. Assaha DVM, Ueda A, Saneoka H, Al-Yahyai R, Yaish MW. The role of Na+ and K+ transporters in salt stress adaptation in glycophytes. Front Physiol. 2017;8:509. doi:10.3389/fphys.2017.00509. [Google Scholar] [PubMed] [CrossRef]
11. Heuzé V, Tran G, Bastianelli D, Lebas F. Soybean meal. Feedipedia, a programme by INRAE, CIRAD, AFZ and FAO; 2020. Available from: https://www.feedipedia.org/node/41. [Accessed 2020]. [Google Scholar]
12. Pagano MC, Miransari M. The importance of soybean production worldwide. In: Miransari M, editor. Abiotic and biotic stresses in soybean production. San Diego, CA, USA: Academic Press; 2016. p. 1–26. doi:10.1016/B978-0-12-801536-0.00001-3. [Google Scholar] [CrossRef]
13. Yuan M, Bi Y, Han D, Wang L, Wang L, Fan C, et al. Long-term corn-soybean rotation and soil fertilization: impacts on yield and agronomic traits. Agronomy. 2022;12(10):2554. doi:10.3390/agronomy12102554. [Google Scholar] [CrossRef]
14. Bustingorri C, Lavado RS. Soybean growth under stable versus peak salinity. Sci Agric. 2011;68(1):102–8. doi:10.1590/S0103-90162011000100015. [Google Scholar] [CrossRef]
15. Rhaman MS, Tania SS, Imran S, Rauf F, Kibria MG, Ye W, et al. Seed priming with nanoparticles: an emerging technique for improving plant growth, development, and abiotic stress tolerance. J Soil Sci Plant. 2022;22(4):4047–62. doi:10.1007/s42729-022-01007-3. [Google Scholar] [CrossRef]
16. Rhaman MS, Imran S, Rauf F, Khatun M, Baskin CC, Murata Y, et al. Seed priming with phytohormones: an effective approach for the mitigation of abiotic stress. Plants. 2020;10(1):37. doi:10.3390/plants10010037. [Google Scholar] [PubMed] [CrossRef]
17. Sharma A, Kohli SK, Khanna K, Ramakrishnan M, Kumar V, Bhardwaj R, et al. Salicylic acid: a phenolic molecule with multiple roles in salt-stressed plants. J Plant Growth Regul. 2023;42(8):4581–605. doi:10.1007/s00344-022-10902-z. [Google Scholar] [CrossRef]
18. Dawood MG. Stimulating plant tolerance against abiotic stress through seed priming. In: Rakshit A, Singh H, editors. Advances in seed priming. Singapore: Springer; 2018. p. 147–83. doi:10.1007/978-981-13-0032-5_10. [Google Scholar] [CrossRef]
19. Kapilan R, Vaziri M, Zwiazek JJ. Regulation of aquaporins in plants under stress. Biol Res. 2018;51(1):1–11. doi:10.1186/s40659-018-0152-0. [Google Scholar] [PubMed] [CrossRef]
20. Kwon EH, Adhikari A, Imran M, Lee DS, Lee CY, Kang SM, et al. Exogenous SA applications alleviate salinity stress via physiological and biochemical changes in St John’s wort plants. Plants. 2023;12(2):310. doi:10.3390/plants12020310. [Google Scholar] [PubMed] [CrossRef]
21. Alam P, Balawi TA, Faizan M. Salicylic acid’s impact on growth, photosynthesis, and antioxidant enzyme activity of Triticum aestivum when exposed to salt. Molecules. 2022;28(1):100. doi:10.3390/molecules28010100. [Google Scholar] [PubMed] [CrossRef]
22. Chen S, Zhao CB, Ren RM, Jiang JH. Salicylic acid had the potential to enhance tolerance in horticultural crops against abiotic stress. Front Plant Sci. 2023;14:1141918. doi:10.3389/fpls.2023.1141918. [Google Scholar] [PubMed] [CrossRef]
23. Imran S, Mahamud MA, Paul NC, Chakrobortty J, Sarker P, Paul S, et al. Seed priming and exogenous application of citric acid enhance seedling growth and photosynthetic pigments and mitigate oxidative damage of soybean (Glycine max) under salt stress. Arch Biol Sci. 2023;75(4):408–18. doi:10.2298/ABS230804033I. [Google Scholar] [CrossRef]
24. Tanveer A, Sibtain M, Javaid MM, Ali HH. Germination ecology of wild onion: a rainfed crop weed. Planta Daninha. 2014;32(1):69–80. doi:10.1590/S0100-83582014000100008. [Google Scholar] [CrossRef]
25. Pradheeban L, Nissanka NAASP, Suriyagoda LDB. Clustering of rice (Oryza sativa L.) varieties cultivated in Jaffna district of Sri Lanka based on salt tolerance during germination and seedling stages. Trop Agric Res. 2015;25(3):358–75. doi:10.4038/tar.v25i3.8045. [Google Scholar] [CrossRef]
26. Zahedifar M, Zohrabi S. Germination and seedling characteristics of drought-stressed corn seed as influenced by seed priming with potassium nanochelate and sulfate fertilizers. Acta Agric Slov. 2016;107(1):113–28. doi:10.14720/aas.2016.107.1.12. [Google Scholar] [CrossRef]
27. Kartika K, Lakitan B, Ria RP. Hydroand osmo-priming effects on upland rice exposed to drought conditions at vegetative and reproductive stages. CMUJ Nat Sci. 2021;20(3):e2021053. [Google Scholar]
28. Mostofa MG, Fujita M. Salicylic acid alleviates copper toxicity in rice (Oryza sativa L.) seedlings by upregulating antioxidative and glyoxalase systems. Ecotoxicology. 2013;22(6):959–73. doi:10.1007/s10646-013-1073-x. [Google Scholar] [PubMed] [CrossRef]
29. Hietz P, Rosner S, Sorz J, Mayr S. Comparison of methods to quantify loss of hydraulic conductivity in Norway spruce. Ann For Sci. 2008;65(5):502. doi:10.1051/forest:2008023. [Google Scholar] [CrossRef]
30. Ibiang SR, Sakamoto K. Modulation of phytochemicals and essential trace elements in fruits of different tomato cultivars by the endophytic fungus Penicillium pinophilum EU0013. Microbes Environ. 2022;37(3):ME22026. doi:10.1264/jsme2.ME22026. [Google Scholar] [PubMed] [CrossRef]
31. Ridley SM. Interaction of chloroplasts with inhibitors: induction of chlorosis by diuron during prolonged illumination in vitro. Plant Physiol. 1977;59(4):724–32. [Google Scholar] [PubMed]
32. Zhang Z, Huang R. Analysis of malondialdehyde, chlorophyll proline, soluble sugar, and glutathione content in Arabidopsis seedling. Bioprotocol. 2013;3(14):e817. doi:10.21769/BioProtoc.817. [Google Scholar] [CrossRef]
33. Velikova V, Yordanov I, Edreva AJPS. Oxidative stress and some antioxidant systems in acid rain-treated bean plants: protective role of exogenous polyamines. Plant Sci. 2000;151(1):59–66. doi:10.1016/S0168-9452(99)00197-1. [Google Scholar] [CrossRef]
34. Ceritoglu M, Erman M, Yildiz F. Effect of salinity on germination and some agro-morphological traits in chickpea seedling. ISPEC J Agric Sci. 2020;4(1):82–96. doi:10.46291/ISPECJASvol4iss1pp82-96. [Google Scholar] [CrossRef]
35. Chakrobortty J, Imran S, Mahamud MA, Sarker P, Paul NC. Effect of citric acid (CA) priming and exogenous application on germination and early seedling growth of okra (Abelmoschus esculentus L.) plants under salinity stress condition. Arch Agric Environ Sci. 2022;7(3):318–26. doi:10.26832/24566632.2022.070303. [Google Scholar] [CrossRef]
36. Asadi M, Heidari MA, Kazemi M, Filinejad AR. Salicylic acid induced changes in some physiological parameters in chickpea (Cicer arietinum L.) under salt stress. J Adv Agric Technol. 2013;9:311–16. [Google Scholar]
37. Shakirova FM, Hayat S, Ahmad A. Role of hormonal system in the manifestation of growth promoting and antistress action of salicylic acid, Salicylic acid: a plant hormone. Netherlands: Springer; 2007. p. 69– 89. doi:10.1007/1-4020-5184-0_4. [Google Scholar] [CrossRef]
38. Ceritoğlu M, Erman M. Mitigation of salinity stress on chickpea germination by salicylic acid priming. Int J Agric Wildlife Sci. 2020;6(3):582–91. doi:10.24180/ijaws.774969. [Google Scholar] [CrossRef]
39. Azooz MM. Salt stress mitigation by seed priming with salicylic acid in two faba bean genotypes differing in salt tolerance. Int J Agric Biol. 2009;11(4):343–50. [Google Scholar]
40. Mahboob W, ur Rehman H, Basra SMA, Afzal I, Abbas MA, Naeem M, et al. Seed priming improves the performance of late sown spring maize (Zea mays) through better crop stand and physiological attributes. Int J Agric Biol. 2015;17(3):491–98. doi:10.17957/IJAB/17.3.14.283. [Google Scholar] [CrossRef]
41. Anaya F, Fghire R, Wahbi S, Loutfi K. Influence of salicylic acid on seed germination of Vicia faba L. under salt stress. J Saudi Soc Agric Sci. 2018;17(1):1–8. doi:10.1016/j.jssas.2015.10.002. [Google Scholar] [CrossRef]
42. Tania SS, Rauf F, Rahaman MM, Kabir MH, Hoque MA, Murata Y. Alleviation of salt-inhibited germination and seedling growth of kidney bean by seed priming and exogenous application of salicylic acid (SA) and hydrogen peroxide (H2O2). Seeds. 2022;1(2):87–98. doi:10.3390/seeds1020008. [Google Scholar] [CrossRef]
43. Hamid M, Ashraf MY, Arashad M. Influence of salicylic acid seed priming on growth and some biochemical attributes in wheat grown under saline conditions. Pak J Bot. 2008;40:361–7. [Google Scholar]
44. Li Y, Li H, Li Y, Zhang S. Improving water-use efficiency by decreasing stomatal conductance and transpiration rate to maintain higher ear photosynthetic rate in drought-resistant wheat. Crop J. 2017;5(3):231–9. doi:10.1016/j.cj.2017.01.001. [Google Scholar] [CrossRef]
45. Parvin K, Hasanuzzaman M, Bhuyan MB, Nahar K, Mohsin SM, Fujita M. Comparative physiological and biochemical changes in tomato (Solanum lycopersicum L.) under salt stress and recovery: role of antioxidant defense and glyoxalase systems. Antioxidants. 2019;8(9):350. doi:10.3390/antiox8090350. [Google Scholar] [PubMed] [CrossRef]
46. Islam AT, Ullah H, Himanshu SK, Tisarum R, Cha-um S, Datta A. Effect of salicylic acid seed priming on morpho-physiological responses and yield of baby corn under salt stress. Sci Hortic. 2022;304:111304. doi:10.1016/j.scienta.2022.111304. [Google Scholar] [CrossRef]
47. Janah I, Elhasnaoui A, Issa Ali O, Lamnai K, Aissam S, Loutfi K. Physiochemical responses of Stevia rebaudiana Bertoni subjected to sodium chloride (NaCl) salinity and exogenous salicylic acid application. Gesunde Pflanzen. 2021;73(4):509–20. doi:10.1007/s10343-021-00570-6. [Google Scholar] [CrossRef]
48. Kordrostami M, Rabiei B, Hassani Kumleh H. Biochemical, physiological and molecular evaluation of rice cultivars differing in salt tolerance at the seedling stage. Physiol Mol Biol Plants. 2017;23(3):529–44. doi:10.1007/s12298-017-0440-0. [Google Scholar] [PubMed] [CrossRef]
49. Foyer CH, Shigeoka S. Understanding oxidative stress and antioxidant functions to enhance photosynthesis. Plant Physiol. 2011;155(1):93–100. doi:10.1104/pp.110.166181. [Google Scholar] [PubMed] [CrossRef]
50. Loutfy N, Sakuma Y, Gupta DK, Inouhe M. Modifications of water status, growth rate and antioxidant system in two wheat cultivars as affected by salinity stress and salicylic acid. J Plant Res. 2020;133(4):549–70. doi:10.1007/s10265-020-01196-x. [Google Scholar] [PubMed] [CrossRef]
51. Ogunsiji E, Umebese C, Stabentheiner E, Iwuala E, Odjegba V, Oluwajobi A. Salicylic acid enhances growth, photosynthetic performance and antioxidant defense activity under salt stress in two mungbean [Vigna radiata (L.) R. Wilczek] variety. Plant Signal Behav. 2023;18(1):2217605. doi:10.1080/15592324.2023.2217605. [Google Scholar] [PubMed] [CrossRef]
52. Marschner H. Marschner’s mineral nutrition of higher plants. London, UK: Academic Press; 2011. doi:10.1016/C2009-0-63043-9. [Google Scholar] [CrossRef]
53. Ma X, Zheng J, Zhang X, Hu Q, Qian R. Salicylic acid alleviates the adverse effects of salt stress on Dianthus superbus (Caryophyllaceae) by activating photosynthesis, protecting morphological structure, and enhancing the antioxidant system. Front Plant Sci. 2017;8:600. doi:10.3389/fpls.2017.00600. [Google Scholar] [PubMed] [CrossRef]
54. Heidarian F, Roshandel P. Salicylic acid improves tolerance against salt stress through boosting antioxidant defense system in black bean. Int J Hortic Sci. 2021;8(2):175–89. doi:10.22059/ijhst.2020.297885.345. [Google Scholar] [CrossRef]
55. Khan MIR, Fatma M, Per TS, Anjum NA, Khan NA. Salicylic acid-induced abiotic stress tolerance and underlying mechanisms in plants. Front Plant Sci. 2015;6:462. doi:10.3389/fpls.2015.00462. [Google Scholar] [PubMed] [CrossRef]
Appendix A
Figure A1: Screening for selecting suitable salicylic acid (SA) doses for the experiment. The data are presented as means of 3 replicates ± SE, with a sample size n = 15 for each replicate. By using Tukey HSD (p < 0.05), different letters between treatments were examined
Cite This Article
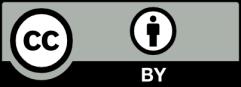
This work is licensed under a Creative Commons Attribution 4.0 International License , which permits unrestricted use, distribution, and reproduction in any medium, provided the original work is properly cited.