Open Access
ARTICLE
Effect of Organic Waste and Inorganic Additives on Organic Matter Transformation and Mineral Availability in Composting Green Waste
1 Department of Biology, Multidisciplinary Faculty of Nador, Mohammed First University, Nador, 62700, Morocco
2 Center of Agrobiotechnology and Bioengineering, Research Unit Labeled CNRST (Centre Agro-Biotech-URL-CNRST-05), “Physiology of Abiotic Stresses” Team, Cadi Ayyad University, Marrakech, 40000, Morocco
3 Laboratoire Agro-Alimentaire, Biotechnologies et Valorisation des Bioressources Végétales, Faculty of the Sciences Semlalia, Cadi Ayyad University, Marrakech, 40000, Morocco
4 Department of Plant Protection, Phytopathology Unit, Ecole Nationale d’Agriculture de Meknès, Km10, Rte Haj Kaddour, BP S/40, Meknès, 50001, Morocco
5 African Sustainable Agriculture Research Institute (ASARI), Mohammed VI Polytechnic University (UM6P), Laayoune, 70000, Morocco
* Corresponding Authors: Rachid Lahlali. Email: ; Abdelilah Meddich. Email:
Phyton-International Journal of Experimental Botany 2024, 93(9), 2227-2249. https://doi.org/10.32604/phyton.2024.055629
Received 03 July 2024; Accepted 15 August 2024; Issue published 30 September 2024
Abstract
Applying organic waste and inorganic additives during composting can be an effective and easy-to-control strategy for optimizing humification, maturation, and the availability of essential mineral elements in compost. In this respect, this study aims to evaluate the effect of organic (olive-mill waste and horse manure) and inorganic (phosphogypsum) additives on the evolution of maturity indices, aromatic compounds, and nutrient availability during composting. Four mixtures [horse manure + green waste (M1), olive-mill + green waste (M2), sewage-sludge + phos-phogypsum + green waste (M3), and green waste (M4)] were carried out. Physicochemical (temperature, pH, phosphorus, nitrogen, and carbon-to-nitrogen (C/N) ratio), infrared-spectroscopic, and phytotoxicity were monitored. The results showed that (in)organic additives have a positive effect on maturity and humification indices, pH stability, and the phosphorus and nitrogen availability in the composts produced. The horse manure additive reduced the carbon and increased the nitrogen, reducing the C/N ratio. Phosphogypsum and olive-mill reduced phytotoxicity, boosting the nitrogen and phosphorus availability of in composts. Fourier transform infrared spectroscopy (FT-IR) analysis revealed that phosphogypsum and olive-mill addition improved the aromatic compounds and reduced the aliphatic ones in M3 and M4 com-posts. These results suggest new approaches to promoting maturity and stability, as well as phosphorus and nitrogen availability in composts, through better use of organic waste and inorganic additives in composting.Keywords
Currently, the amount of organic waste produced is rising annually. Nevertheless, over 80 million tons of waste and organic by-products pile up yearly at landfills [1]. In Morocco, landfilling is the chosen method for waste disposal. This process leads to the generation of leachates—liquids that seep through the waste mass—which contain elevated concentrations of pollutants, including dissolved organic matter, inorganic macro-components, trace elements, and xenobiotic organic compounds [2]. These contaminants, which include both organic waste and inorganic additives, can adversely affect public health and the environment [3]. Other significant by-products are continuously produced in large quantities by industrial activities, including livestock effluents, sewage sludge, phosphogypsum, horse manure, and olive milk. These by-products deserve to be recovered and explored. Additionally, the accumulation of waste in landfills results in the loss of valuable organic matter that could otherwise be used as an effective soil amendment [4]. Recently, the recycling and valorization of organic waste and inorganic additives have gained increasing attention from scientists, environmentalists, and policymakers. This focus is driven by the need to conserve natural resources, protect the environment, and ensure human health and safety [5–7]. Indeed, the valorization of these by-products through the composting process is an interesting and promising strategy for minimizing the pollution of natural ecosystems (soil, water, and air) and producing high-quality, stable organic soil improvers (composts) rich in essential minerals [8–10]. Consequently, this approach represents a simple, effective, and sustainable solution on all levels: agricultural, environmental, ecological, and socio-economic. Moreover, the optimization and successful progression of the composting process requires a careful balance of carbon and nitrogen as major elements necessary for microbial decomposition [11–13]. In contrast, horse manure, and olive mill waste are rich in organic matter and nutrients, particularly phosphorus (P) and nitrogen (N). The valorization and optimization of the rates of these by-products during the composting process can be considered as a source for the production of a mature and stable compost, rich in nutrients and humic substances that help improve crop yields [14–16]. In addition, the use of inorganic additives such as sewage sludge and phosphogypsum can help to adjust and optimize the pH for the growth of microorganisms involved in lignin and cellulose degradation. However, the organic matter and nutrients contained in those by-products, together with their buffering capacity and microbial activity, can create optimal conditions for the production of mature compost, rich in humic substances and elements essential for crop growth and productivity. In fact, the importance of recycling and recovering organic waste and inorganic additives by-products has become a necessary environmental act for the restoration of soil ecosystems poor in humus and mineral elements. Indeed, it is generally accepted that organic waste and inorganic additives should be recycled to improve soil properties [17–19]. Many authors have pointed out that using organic waste and inorganic additives by-products as fertilizer is a highly effective strategy for restoring and enhancing the organic and mineral composition of soils [20,21]. However, little national or international research has demonstrated that the addition of organic waste and inorganic additives improves the maturity and mineral content of composts after 3 months of composting.
Organic waste composting is a widely recognized method for recycling residual materials and enriching soils. However, the exclusive use of organic waste in composting presents several significant drawbacks. One major issue is the imbalance in the carbon-to-nitrogen (C/N) ratio, which can slow down the decomposition process [22]. This inadequate C/N ratio can compromise the quality of the final compost, making it less nutritious and less stable [23]. To address these limitations, the integration of inorganic additives with organic waste in the composting process has been explored. Sewage sludge, horse manure, and olive mill residues, which are rich in nitrogen and organic matter [14–16], can help balance the C/N ratio promote microbial activity, and speed up the process of mineralization as well as the rapid humification of hard-to-degrade organic compounds during the green waste composting process [24–27]. Additionally, the use of these additives is pivotal in accelerating the development of compost maturity in the short term [28]. Optimizing composting conditions contributes to a more uniform and faster decomposition of organic materials, thereby reducing the time needed to produce mature and stable compost. This not only enhances the quality of the final compost but also enables quicker and more effective use of the compost in agricultural or horticultural applications. The present study was to monitor the biotransformation of various organic wastes through the composting process and to assess the effect of the nature of the by-products on composting progress, as well as the effect of additional sewage sludge and phosphogypsum on compost quality. We hypothesized that (in)organic additives added to green organic waste during composting could adjust the pH, accelerate maturity, and increase the phosphorus and nitrogen content available in the final compost after three months of composting.
The co-composting experiments were conducted during 3 months on a 2400 m2 composting platform of enclosedby a metal frame, at the municipal nursery located 10 km from Marrakech, Morocco. Four mixtures were prepared using various organic wastes: horse manure, sewage sludge, green waste and olive-mill waste. These wastes were collected from the urban commune. Green waste based on quackgrass was the main composting raw material for all the mixtures. Quackgrass is one of the most invasive and abundant fast-growing species, widely available in all green and agricultural areas of Morocco.
Mixture 1 (M1): 75% of horse manure and 25% of green wastes.
Mixture 2 (M2): 75% of green wastes and 25% of olive mill wastes.
Mixture 3 (M3): 70% of green wastes, 25% of sewage sludge and 5% of phosphogypsum.
Mixture 4 (M4): 100% of green wastes.
The main characteristics of all used substrates in co-composting are presented in Table 1. Each mixture was uniformly blended and positioned on plastic sheeting to minimize runoff and leaching during the moistening process.
The windrows were covered with additional perforated plastic to minimize evaporation and heat loss. Humidity was then maintained at 60%—the ideal level for composting—throughout the process, as suggested by various experts [29,30]. The aeration was provided by regular manual mixing every week during 3 months of co-composting. Homogeneous 1 kg samples were prepared by thoroughly mixing multiple sub-samples collected from various points (both height and length) within the windrow, using the quartage method [31]. The samples were kept at −20°C before analysis.
2.2 Physicochemical Parameters Analysis
The temperature readings were taken daily at various depths and locations within the windrow using a metal probe thermometer. The moisture content was determined by drying 60 g sample at 105°C for 48 h [32]. The pH of a 10 g sample suspension in 20 mL of distilled water was measured at room temperature. Total organic carbon content was of the samples was determined by calcining them in a muffle furnace at 600°C for 6 h [33]. Total Kjeldahl nitrogen (TKN) was determined by using classical Kjeldahl procedure. Olsen’s method was used for available phosphorus [34].
The phytotoxicity of obtained composts was conducted as recommended by Tiquia [35]. Germination tests were carried out with Lepidium sativum L using extracts prepared in water of different composts (M1, M2, M3 and M4) prepared by soaking 10 g of fresh sample in 50 mL of sterile distilled water. The tests were performed in the dark at room temperature (27°C) for 72 h [36,37]. Thus, 20 Lepidium sativum seeds, disinfected with 10% of sodium hypochlorite for 10 min and subsequently rinsed several times with distilled water, were placed on filter paper in Petri dishes, imbibed with water-soluble extracts. Three replicates were conducted for each stage of the four composts. calculated based on the percentage of viable seeds. This involved monitoring seedling emergence, counting the number of germinated seeds (NGe for seeds germinated in water-soluble extracts and NGw for seeds in distilled water) after 24 h, and measuring root growth (LRe for root length in soluble extracts and LRw for root length in distilled water) after 72 h, using the following equation [37,38]:
2.4 Fourier Transform Infra-Red Spectroscopy Analysis (FTIR)
Infrared spectra for each stage of composting were recorded using an FT-IR Fourier Transform spectrometer, covering wavenumbers from 4000 to 400 cm−1. Pellets were prepared by mixing 1 mg of dry ground sample mixed with 99 mg of dried potassium bromide (KBr) at 105°C for 72 h. The mixture was then compressed under vacuum for 10 min to form pellets. The granules were analyzed with a Vortex 70 DTGS spectrometer, which extends over the frequency range between 4000 and 400 cm−1 [39,40].
3.1 Physicochemical Characterization
Fig. 1 shows the evolution of temperature at different phases of composting of the various organic wastes, in accordance with the three classic composting phases. Indeed, after 3 days of the mesophilic phase of composting, a rapid rise in temperature was recorded for all the mixtures processed. For the M1 mixture containing green waste and the organic additive olive mill, the temperature reached its highest value (58°C) after 13 days of composting. In addition, the addition of the inorganic additive (phosphogypsum) resulted in an increase to 57.7°C after 14 days of composting. For the M2 and M4 mixtures, a rapid increase of 45°C and 39°C respectively was observed between 5 and 7 days of composting. Thereafter, the temperature gradually decreased in all mixes, reaching values between 25°C and 35°C. Mixture M2 then showed a second maximum temperature increase to over 40°C after 26 days of composting. In addition, mixtures M1, M3 and M4 have a second maximum temperature over the same period. It has been reported that after turning substrates during composting processes increases windrow temperature. In addition, maintaining an appropriate turning frequency in the composting windrow ensures sufficient oxygen supply, which boosts microbial activity and metabolism. This promotes more efficient degradation of organic matter and generates significant heat [41,42]. After 1 month of composting, the mixtures reached values closer to ambient temperature, indicating the maturation phase.
Figure 1: Temperature during co-composting of mixtures: M1: horse-manure and green-waste; M2: olive-mill and green-waste; M3: sewage-sludge, phosphogypsum and green-waste; M4: green-waste and T. amb: Ambient temperature
In agreement with several authors [43,44], temperature is a key factor for determining the success of composting process. It controls the biodegradation of organic material leading to determine the microbial activity responsible to this biodegradation [45]. While a temperature between 40°C and 45°C (as observed in M2) is optimal for composting progress, it is not sufficient to sanitize the compost by eliminating pathogens and parasites While a temperature between 40°C and 45°C (as observed in M2) is optimal for composting progress but it is not sufficient to sanitize the compost by eliminating pathogens and parasites [46,47]. According to Ravindran and Sekaran [48], composting at temperatures exceeding 55°C, as observed in M1 and M3, facilitates the elimination of parasites and pathogens, thereby ensuring optimal sanitary conditions. As temperature between 45°C and 55°C favors the biodegradation and that between 35°C and 40°C (case of M4) improves the microbial diversity [46,49]. Moreover, it is known that the temperature evolution during composting process can be affected by several operational parameters such as moisture, C/N ratio, pH, composting mass [50,51], pile volume, aeration strategy, compost porosity [52] and the nature of composted substrate [48]. However, the addition of green waste alone in M4, which is rich in lignocellulosic materials, may have influenced bacterial activity during composting. This resulted in a shorter thermophilic phase and a more pronounced cooling phase, with fungal activity becoming more dominant [53]. Thus, on the basis of the results obtained (Table 2), we can attribute the slight rise in temperature in the three mixes M2 and M4 to the probable effect of initial moisture content, mixture composition and initial C/N ratio. Indeed, the moisture content of these mixtures was lower than that of mixtures M1 and M3. In fact, the initial moisture content of the M1 and M3 mixtures exceeded 60%, which is the optimum value for successful composting. On the other hand, several studies have reported that the optimum moisture content lies between 40% and 60%, depending on the type or shape of the waste [29,30,54–56]. In fact, when the moisture content is too high, the pore spaces are closed and microbial activity is stifled due to the hindrance of oxygen movement, and the composting process tends towards anaerobiosis with decreasing temperature [56,57]. It has also been observed that temperature evolution in M1 and M3 mixtures can also be related to the initial C/N ratio. According to the literature [58–60], the initial C/N ratio should be between 20 and 40. A low C/N ratio leads to ammonia production, thus inhibiting the growth and activity of nutrient bacteria during the thermophilic phase [61], which in turn leads to a decrease in temperature. Similarly, a higher C/N ratio reduces the biodegradation process and the rise in temperature (case of M4) [51,58,62]. The effect of the initial C/N ratio can be confirmed by the study carried out by Goyal et al. [63], who recorded a maximum temperature rise of 46°C during the co-composting of five mixtures from various organic wastes, with an initial C/N ratio of between 13 and 51 and a moisture content of 60%. The M1 and M3 mixtures were able to maintain temperatures above 50°C for more than a week, thus promoting equipment disinfection [64]. In addition, both mixtures met the conditions recommended by Böhm [65], according to which temperatures should be above 55°C for more than a week. The higher temperature values recorded in the M1 and M3 mixtures are mainly attributed to the roles of the organic waste and inorganic additives, which are mainly involved in windrow aeration. In M1, the addition of horse manure as an organic additive led to a rapid temperature increase, which accelerated the composting process and improved overall efficiency. This effect is likely due to the high concentration of active microorganisms present in the horse manure [66]. The increase in composting temperature may be attributed to the addition of horse manure, which introduced numerous pores into the mixture. This enhancement in aeration and substrate permeability within the windrow created a more favorable environment for microbial activity [25]. These findings were corroborated by research conducted by Du et al. [67], which demonstrated that horse manure can enhance microbial and enzymatic activity and optimize temperature profiles for windrows. In addition, horse manure represents a balanced source of carbon and nitrogen, which are essential elements that meet the energy needs of microorganisms and therefore have a considerable impact on the growth and reproduction of microorganisms and the quality of composts produced [67–69]. Similarly, sewage sludge and phosphogypsum added to the M3 mixture provide a nutrient source that stimulates the growth and activity of beneficial microorganisms [70,71]. These microbes play a crucial role in the decomposition of the organic matter to be composted, thus facilitating the composting process [72]. In addition, the presence of sewage sludge can introduce a wide range of enzymes into the compost, further accelerating the decomposition of complex organic compounds and consequently increasing windrow temperatures. Lei et al. [73] reported that the addition of 10% phosphogypsum increased bacterial and fungal diversity in compost windrows. In addition, numerous studies have demonstrated that inorganic additives can enhance bacterial and fungal communities by modifying physiochemical properties such as temperature, total Kjeldahl nitrogen and pH. These changes contribute to more effective degradation and humification of organic matter [73–75]. However, M4 mixtures based on green waste alone, with no organic waste and inorganic additives, contain large quantities of lignin and cellulose and are low in the nitrogen required for micro-organism activity, which makes them difficult to break down during composting and generally results in poor-quality, long-lasting compost [74]. In summary, incorporating (in)organic additives into composting systems can enhance microbial and enzymatic activity, leading to more efficient decomposition and better temperature management. This optimization contributes to the production of high-quality compost and ensures a more efficient composting process overall.
The pH variations in the four mixtures over the 3 months of composting are shown in Table 2. These pH variations are most pronounced in mixtures M2 and M3, dropping from 8.2 and 8.3 to 7.8 and 7.7 respectively during the first month of composting. On the other hand, in mixture M4, the trend is opposite, with pH rising from 7.7 to 8.1 after 1 month’s composting. The final composts of mixes M2, M3 and M4 reached pH values of 7.3, 7.2 and 7.5, respectively, which are within the pH range 6–8 for mature compost. During composting of the M4 mixture which contained green waste alone, a slight acidification was recorded. Lazcano et al. [76] observed that nitrogen and phosphorus compound mineralization can lead to a reduction in pH. Zhang et al. [77] reported that oxygen deficiencies between turnings can result in the acids production. This can explain the observed evolution of pH values for all mixtures. The addition of olive mill (M2) and sewage sludge (M3) to composting systems creates an optimal environment for aeration by improving air circulation and promoting the growth of acid-generating bacteria [78,79]. These bacteria promote the rapid decomposition of organic matter, resulting in the organic acid production [80]. As a result, the pH of the compost decreases, stimulating microbial activity and enhancing the efficiency of the composting process [81,82]. In addition, the inclusion of organic waste and inorganic additives balances the carbon/nitrogen ratio in compost, contributing to more efficient decomposition and nutrient stabilization [83,84]. The addition of horse manure composting can neutralize excess acidity, creating a more favorable environment for microorganisms [85]. Studies have shown that horse manure application helps to accelerate the organic matter decomposition and improved compost stability. It also aids in controlling the production of undesirable acidic by-products during the composting process. The addition of manure (M1) and sewage sludge (M3) stimulates enzymatic activity and promotes a greater microbial diversity, leading to a more complete decomposition of organic waste [86–89]. This decomposition process enables organic acids to be liberated, thus lowering the compost’s pH. This pH improvement through the addition of organic waste and inorganic additives can increase enzymatic activity, particularly of cellulases and laccases, accelerating the decomposition of complex green waste components such as cellulose and lignin [90]. This enhanced degradation facilitates a faster and more uniform transformation of organic materials into compost. Furthermore, an enriched microbial diversity improves the dynamics of microbial communities, optimizing the decomposition and maturation processes of the compost [91]. The resulting pH reduction from organic acid production creates a more stable compost environment, while inhibiting the growth of pathogens and undesirable organisms [92]. These conditions contribute to producing high-quality compost that is nutrient-rich and well-aerated, making it suitable for agricultural applications. Adding phosphogypsum with a very acidic pH to M3 can help reduce the pH of the compost, which is advantageous in preventing excessively high pH levels. This adjustment fosters a more balanced environment, optimizing conditions for microbial activity and promoting effective decomposition [93]. The addition of phosphogypsum led to a gradual reduction in the pH of the compost. The acidification caused by phosphogypsum promotes faster, more uniform decomposition of organic waste, contributing to better compost maturation [93,94]. The elevated moisture maintained through the process leading to the decrease in the oxygen uptake rate, consequently, continuous production of acids [56]. According to Muktadirul Bari Chowdhury et al. [52], if pH exceeds 7.5, loss of ammonia gas during the composting process increases. This pH increase can be caused by ammonia accumulation resulting from protein degradation [95], which may liberate compounds naturally present in the organic waste [63,96]. Thus, the increase of the pH during co-composting of the mixture M4 after one month’s can be explained by nitrogen accumulation. Yang et al. [94] showed that the complete organic matter degradation with little acid produced, the coupled with NH3 continuous release may be associated with the increase in pH observed during the composting completion phase. This indicates that the final pH value in M4 was in the range of satisfactory pH values (7–8) [97]. Generally, after 3 months of co-composting, the pH of the four mixtures was stabilized around the neutrality. According to Hachicha et al. [95], mature compost should have a pH of between 6 and 8.5, which ensure the compatibility with the majority of plant, and is in favor of better microbial decomposition of organic matter [98,99]. Others works noted that the final pH value indicating a good progress of composting should be in the range of 8 and 9 [100,101]. Nevertheless, Rich et al. [102] observed that the pH of the compost was not a crucial variable for stability. Adding organic waste and inorganic additives to the green waste composting optimizes the process by regulating the pH, which promotes more efficient decomposition of difficult-to-degrade organic matter, creating a more favorable environment for decomposing micro-organisms and better compost quality.
3.1.3 Available Phosphorus and Total Nitrogen Evolution
Table 2 illustrates the evolution of available phosphorus and total nitrogen concentrations in all mixtures which increased during the composting. Indeed, the accumulation of total nitrogen concentrations was most pronounced in the M3 mixture containing organic (green wastes) and inorganic (sewage sludge and phosphogypsum) additives, followed by the M2 mixture containing olive mill after 3 months of composting. However, the M4 mixture composed exclusively by green waste presents the least pronounced nitrogen accumulation at composting end.
It is important here noted that the decrease in nitrogen concentration after 1 month of composting in the M1 and M3 mixtures may be attributed to the relatively high temperature and pH as well as NH3 emissions during composting thermophilic phase, which do not favor nitrification and denitrification [103,104], while the increase in nitrogen concentration at the composting end was related to the nitrogenous organic matter degradation and the concentration effect during the curing phase [105]. It has been suggested that the thermophilic phase above 55°C (M1 and M3) promotes intense microbial activity and accelerates organic matter decomposition [106–109]. This decomposition produces significant quantities of NH3 as a by-product of protein and amino acid degradation which explains the reduction in nitrogen [110,111]. Furthermore, the M2 mixtures exhibited absolutely no significant decrease in nitrogen content over the course of the composting phase, indicating that volatile NH3 was likely immobilized by compost substrates amended with organic olive mill additives [112]. Organic additives from olive mill showed a notable ability to immobilize volatile ammonia. These additives include residues such as olive mill, which contain various organic compounds that can interact with NH3 [113]. The acidic additive (phosphogypsum in the M3 mixture) was expected to reduce NH3 levels and rapidly increase nitrogen content in the composting. Similarly, Jiang et al. [114] noted an increase in nitrogen concentrations in the range of 0.9 to 2.59 mg/g in the compost product when composted with acidic calcium superphosphate additives. The high nitrogen level in the M3 could be attributed to the a acid additives added to promote the NH3 to NH4+ conversion [115]. In addition, the increase in nitrogen could also be achieved by the carbonisation of phosphogypsum in M3 [109]. The addition of phosphogypsum showed a tendency to decrease CH4 and CO2 emissions. This is probably due to better nitrogen management and a reduction in the anaerobic degradation processes that produce CH4 [116]. In addition, available phosphorus concentrations were highest in the M3 mixture containing sewage sludge and phosphogypsum at the composting end. The addition of phosphogypsum enables more precise control of nutrient release in composts, increasing their efficiency and availability [93]. In addition, the addition of phosphogypsum can help improve nutrient retention in compost, reducing the risk of leaching and improving the fertility of the final compost [116]. Indeed, the use of rock phosphate increased the compost’s content of essential nutrients, such as phosphorus, calcium and potassium [117]. In contrast, the concentration of available phosphorus was lower in mixture 4 containing just green waste than in mixtures containing organic waste and inorganic additives at the composting end. It should be noted that at the composting end, the concentrations of available phosphorus in M3 were four times higher than in M4. These results concur with those obtained by Samet et al. [118], who showed that the phosphogypsum addition increased phosphorus in the composts produced. This increase could be explained by the effect of adding phosphogypsum, which increases the number of fungi and bacteria [119–121]. The phosphogypsum addition increased phosphorus level in the composts produced. Phosphogypsum, produced as a phosphoric acid derivative, contains phosphates and calcium sulfate trace. When incorporated into composting systems, phosphogypsum enhances phosphorus availability by reacting with soil and compost components to form more soluble and readily available phosphate compounds. This increase in phosphorus is crucial for plant nutrition, as phosphorus is a vital macronutrient that supports root development, energy transfer, and photosynthesis. Furthermore, the elevated phosphorus levels help improve the overall compost nutrient profile, resulting in a more effective organic fertilizer. The use of phosphogypsum also aids in balancing the nutrient content of composts.
In Table 2, the C/N evolution in all mixtures demonstrated a decrease over the course of the composting process. This reduction was more pronounced in mixtures containing the organic additives horse manure (M1) and olive mill (M2), with 57% and 64% respectively after 3 months of composting. Results obtained by Lakhtar et al. [122] showed that the C/N of mixtures containing olive mill is reduced to 13.5 after two months of composting. It has been shown that organic carbon and total nitrogen mineralization processes performed by micro-organisms probably account for this decrease over time [123]. Carmona et al. [124] reported that the final C/N of manure-containing mixtures (M1 case) is between 19.4 and 15.3. The addition of horse manure generally led to a more reduction in the C/N in the M1 compost. Horse manure is rich in nitrogen, which helps balance the C/N ratio of the composted substrates. By providing a significant source of nitrogen, it helps reduce the relative excess of carbon, thereby facilitating a more balanced C/N ratio that promotes effective decomposition [27]. In the same way, the addition of olive mill led to a more reduction in the C/N ratio in the compost M2. Olive mill, being rich in nitrogenous compounds and decomposable organic matter, helps lower the C/N ratio, thereby creating a more favorable environment for decomposition by microorganisms [125]. However, the M3 mixture containing the inorganic additive (sewage-sludge and phosphogypsum) underwent a reduction of 51% at the composting end. The reduction in C/N during composting was more pronounced in composts amended with sewage sludge and phosphogypsum. This is due to the fact that these inorganic additives contribute to a more stable nutrient environment. Sewage sludge provides a source of organic nitrogen and carbon, which can slow the rate of C/N reduction by enhancing microbial activity and nutrient retention [25,30,126]. Indeed, it has been shown that phosphogypsum compounds can interact with the organic constituents of green waste, facilitating more controlled degradation and preventing too rapid a reduction in the C/N [127]. Phosphogypsum influences nutrient dynamics and the decomposition process by providing calcium and sulfur, which help balance the composting conditions [109]. The C/N remains more stable in the presence of these additives compared to composts without them. Indeed, most studies conclude that compost is mature by taking into account the final value recorded during final composting. Dimambro et al. [128] and Wang et al. [129] have considered a ratio between 8 and 15 to be characteristic of mature compost. Others consider that a ratio of less than 12 is generally regarded as an indication of maturity [107,108]. On the other hand, Rashad et al. [130] considered that a C/N of less than 20 indicates a good level of maturity, and that about 15 or lower is more appropriate. Nevertheless, a C/N of 20 may also be an indication of advanced maturity [48]. In view of these results, only the M4 mixture is not yet mature, which could be linked to its high content of difficult to biodegrade organic matter, which would limit microbial activity, and hence slower biodegradation and lower maturity. As a result, longer composting would be required to reach maturity. When the C/N is maintained within the optimal range, the green waste composting is accelerated. This is due to improved nutrient availability for decomposer microorganisms playing a pivotal role in organic matter decomposition [131].
Germination index (GI) was largely adopted to estimate compost phytotoxicity. An increased GI indicates lower phytotoxicity and therefore a more mature product [35]. The GI is a factor of relative seed germination and relative root elongation [35,132]. It proved to be the most sensitive of these parameters, reflecting low toxicity during root growth, high toxicity during the germination process and compost maturity [133]. GI values less than or equal to 100% indicate that the compost is not mature or that the sample has some degree of toxicity, while values greater than 100% indicate that the compost is mature or that the sample is not toxic [133,134]. Aggelis et al. [135] proposed that if the GI value is <25, the substrate is characterized highly phytotoxic. Values between 26 and 65 are phytotoxic, while if the GI value is between 66 and 100, it can be considered agriculturally non-phytotoxic, stable and usable. Finally, GI higher than 101 of compost is in favor of its phytonutrient and/or phyto stimulant proprieties and it can be applied in agricultural as fertilizer. Our results showed that the most high GI was recorded for the mixture M2 (114.8%), following by M1, M3 and M4 (101.2%, 93.7% and 74.5%, respectively) (Fig. 2). This increase in germination index could be linked to the strong reduction in phytotoxic substances at the composting end. Liu et al. [67] found that composts made by adding olive mill had a high germination index, due to a strong reduction in phenolic compounds and lignin during the composting [136]. Compost derived from olive mill, which contained a high level of phenolic compounds, had the lowest concentration of phenolic compounds because of their elimination in the composting phase [137]. The high level of the GI translates the great reduction of phytotoxic substances. Some studies showed that the stimulatory effect of water-soluble extracts on radical germination and growth, when GI is above 100%, may be due to the availability of stable organic matter and the accumulation of humic substances and nutrients in the cooling phase [138,139]. Therefore, both mixtures M2 and M1 reached an advanced maturity than mixtures M3 and M4, for which longer composting would be required to reach the same level of maturity as M2 and M1. Siles-Castellano et al. [136] revealed that phenolic compounds reached the highest values in composts derived from plant residues and sewage sludge. Indeed, while the phenolic compounds present may contribute to phytotoxicity of the compost product, this was certainly not the case for composts M3 and M4, which were not phytotoxic (GI 93.7% and 74.5%, respectively). However, compost that is unstable will not necessarily suppress [132], while toxins can only be produced at certain stages of decomposition and tend to be rapidly inactivated [37,132]. Based on these results, we observed that the addition of organic waste and inorganic additives led to the production of composts with enhanced maturity and reduced toxicity levels. These additives improved the efficiency of the decomposition of harmful compounds and optimized the germination index, resulting in composts that are less likely to exhibit harmful phytotoxicity.
Figure 2: Phytotoxicity variation during co-composting of mixtures: M1: horse manure and green waste; M2: olive-mill and green waste; M3: sewage sludge, phosphogypsum and green waste; and M4 green waste
The IRFT spectra of the four composts (Fig. 3) showed very similar peak profiles except for M3 compost, but the relative intensity of the absorption bands varied significantly with the dominance of the peaks or bands at 3500–3400, 2930–2920, 1650–1600 and 1100–1020 cm−1, showing that the four compounds are rich in phenolic, aromatic, aliphatic and polysaccharide structures. At high frequencies, the band at 3500–3400 cm−1 reflects the vibrations of alcoholic hydrogen OH, carboxyl (COOH) or phenol groups, as well as the N-H vibrations of amide functions [53,140]. In olive-mill sludge rich in organic matter, this band was attributed to phenols and carboxylic acid hydroxyl [141]. The band at 2930–2920 cm−1 corresponds to stretching vibrations of C-H fatty acids, waxes and a variety of aliphatic components [142]. The band between 1650–1600 cm−1, is a characteristic of aromatic C=C vibrations, in addition to quinines, carbons, N-H of primary amines and amides and conjugated ketones [37]. The band at 1650–1600 cm−1, is a characteristic of aromatic C=C vibrations, in addition to quinines, carbons, N-H of primary amines and amides and conjugated carboxyls and ketones [143]. The peak around 1460–1430 cm−1 due to aliphatic C-H structures, CH3 and CH2 aromatic and fatty acids [98]. The significant presence of broad band centred at 1100–1020 cm−1 can be attributed to silicates Si-O-Si, aromatics ethers as well as polysaccharides (CH stretching) and to Si-O-C [144]. The intensity of certain peaks in the different spectra of the IRFT stabilizes or decreases. The maximum intensity of the different structures is associated with a combination of a concentration effect, following the disappearance of the most readily decomposable components, and an increase in the oxidized lignin derivatives involved in the humification process. During the composting process, the mixtures M1, M2 and M4 generally have the same number of strips with changes in the absorbance intensity of these peaks. On the other hand, the intensity of the two peaks 1420 and 1044 cm−1 increased remarkably in all spectra. This tendency to change correlated with the greater levels found in the compost samples at the composting end with the exception of M3 compost. M3 compost presented an increased absorbance peak at 1100–1040 cm−1 in the IRFT spectra at zero time. This compost did not show any significant change in the intensity of the peaks observed except for the intensity of the peak around 1040 cm−1, which was marked by a very significant decrease between the initial and final phase of the composting process. Studies reported by Elouear et al. [145] showed that the 1044 cm−1 peak observed on the compost spectrum could be mainly related to phosphate rock residues. In addition, M3 showed a decrease in the intensity of the OH group stretching vibration bands, which are located around 3500 cm−1 that certainly represents the sludge’s fatty acids and lipid compounds [146]. FTIR analyses have revealed that the addition of sewage sludge to lignocellulosic residues from green waste significantly alters the chemical structure of the composts. Specifically, the characteristic bands associated with lipid functional groups show notable changes during composting. Lignin, a major component of lignocellulosic residues from green waste, interacts with lipid compounds in the sewage sludge, affecting the degradation and transformation of fatty acids [147]. Cui et al. [148] confirmed that the composting of sewage sludge with lignocellulosic materials led to a more pronounced aromatization of the compost. This transformation is attributed to the interaction between lignocellulosic residues and sewage sludge, facilitating the decomposition of more complicated molecules down to simpler aromatic structures. Additionally, recent studies have shown that the incorporation of sewage sludge in composting not only accelerates the stabilization of organic matter but also improves humification degree [44,149–151]. Similarly, the addition of sewage sludge to lignocellulosic residues from green waste promotes the formation of more stable organic structures and reduces the levels of difficult-to-degrade organic compounds that may be harmful or undesirable in the final compost [149]. M1 compost showed a slight decrease in peak intensity in the vicinity of 2930–2920 cm−1 resulting from the accelerated decomposition of aliphatic, lipid as well as carbohydrate structures like polysaccharides, cellulose and hemicelluloses by microorganisms participating in the composting process [152,153]. During composting of horse manure, amino acids and polysaccharides break down easily to form humic acids [154]. On the other hand, M2, M3 and M4 composts showed a decrease in peak intensity in the vicinity of 2930–2920 cm−1 after two months of composting and then the intensity of the three composts increases, indicating the presence of material that is still extremely strong against microbiological degradation, most likely associated with molecules of plant origin such as cutins, suberins or lignins [155]. The intensity of polysaccharide or carbohydrate bands (1100–1020, 1420 and 1650-1600 cm−1 for M3) decreased with composting time. At the same time, there has been a significant increase in the intensity of the bands, which absorb around 1020–1100, 1420 and 1600–1650 cm−1 in the case of composts M1, M2 and M4. These peaks showed the enrichment of aromatic C=C in relation to aliphatic carbon [140]. This enrichment in aromatic structures has been confirmed in previous studies on the characterization of humic and fulvic acids, which were considered as indicator of an increasing degree of humification of organic matter under natural biodegradation conditions [140]. In the composting, the increased stability and maturity of composts and their transformation into a highly humified substrate might be associated with the same increase [143,156]. As IRFT findings for M2 and M4 final composts show no significant variation, the similarity of these two composts can be used as an excellent compost maturity indicator. In fact, Ouatmane et al. [152] described compost as a mixture of minerals and humic matter with characteristics independent of the nature of the original material. In addition, in the case of M2 and M4 composts, the increase in peaks 1460, 1430–1400, 1100–1040 cm−1 may be associated with the release of polyosidic and aliphatic structures following the transformation of the lignocellulosic complex under the effect of the degradation of substrates that are difficult for microorganisms to biodegrade [153]. This explains why M2 compost is more mature than the other composts. Indeed, Fersi et al. [157] demonstrated that aliphatic functional groups, including hydroxyls, alcohols, and carboxyls, were predominant in composts made from green waste and olive mill. This predominance indicates a significant presence of these groups in the chemical composition of the composts, reflecting the chemical transformation processes occurring during the composting of these organic materials. The ratio (Table 3), 1650/2930 (C=C aromatic, COO-/C-H aliphatic), evolved in the same way for the three composts (M1: 1.53 to 2.18, M2: 0.98 to 1.92 and M4: 1.91 to 2.44). The increase in these ratios may be caused by a reduction in aliphatic carbon by degradation of aliphatic structures readily accessible to microorganisms, while its decrease (case of compost M3: 7.73 to 4.53) may be due to the release of aliphatic structures not readily accessible to microorganisms after the attack of the ligno-cellulosic complex. For the ratio 1416/1040 (COO-, C-O/C-O polysaccharides), there is an increase for M2, M3 and M4 composts and a decrease in M1 compost towards the end of composting. The resulting increase in this ratio may be related to a decrease in aliphatic carbons, whereas the decrease may be linked to an increase in aromatic and aliphatic carbons at the same time. Spectroscopic analysis of dissolved organic matter in green waste composting offers critical insights into the processes of degradation and stabilization. This analysis highlights the significant role of both organic and inorganic additives in accelerating the degradation and humification processes, which are essential for enhancing compost maturation.
Figure 3: IRTF spectra of mixtures: M1: horse manure and green waste; M2: olive-mill and green waste; M3: sewage sludge, phosphogypsum and green waste and M4: green waste at different composting stages (1, 2, and 3 months)
The study investigated the effects of adding organic waste and inorganic additives to green waste during the composting process, focusing on physicochemical properties, phytotoxicity, aromatic compounds, and nutrient availability. The findings indicate that combining organic additives like manure and olive mill waste with inorganic additives such as phosphogypsum and sewage sludge can significantly optimize composting conditions without negatively impacting or maturity of the compost. Specifically, these additives enhance the decomposition and mineralization of organic matter, thereby reducing phytotoxicity and aliphatic compounds and improving the availability of the mineral elements essential for nitrogen and phosphorus in the composts produced during composting.
The implications of these results are substantial for both composting practices and sustainable agriculture. By improving the decomposition and stabilization of organic matter, these additives contribute to the production of higher-quality compost that is more mature and less toxic. The enhanced availability of essential nutrients, particularly nitrogen and phosphorus, can improve soil fertility and plant growth, making the compost a more effective and sustainable fertilizer. Additionally, the reduction of phytotoxicity and aromatic compounds helps in producing compost that is safer for use in various agricultural applications. This approach not only optimizes composting efficiency but also supports environmental sustainability by recycling organic waste more effectively.
Acknowledgement: None.
Funding Statement: The authors received no specific funding for this study.
Author Contributions: Abderrahim Boutasknit and Mohamed Anli carried out the experiments, contributed to the design and development of the work, carried out the analysis, interpreted the data and wrote the manuscript. Abdelilah Meddich designed and supervised the research. Rachid Lahlali and Abdelilah Meddich revised and finalized the article. All authors reviewed the results and approved the final version of the manuscript.
Availability of Data and Materials: All data obtained or analyzed in this study are available in the article.
Ethics Approval: Not applicable.
Conflicts of Interest: The authors declare that they have no conflicts of interest to report regarding the present study.
References
1. Afilal ME, Mouncif M, Benyamna A. Valorisation des déchets organiques par fermentation méthanique. Revue Des Energies Renouvelables. 2007;7:9–12 (In French). [Google Scholar]
2. Renou S, Poulain S, Gagnaire De Cadarache J, Marrot B, Moulin P. Lixiviat de centre de stockage: déchet généré par des déchets. L’eau, l’industrie, Les Nuisances. 2008;310:37–43 (In Spanish). [Google Scholar]
3. Rashmi I, Roy T, Kartika KS, Pal R, Coumar V, Kala S, et al. Organic and inorganic fertilizer contaminants in agriculture: Impact on soil and water resources. In: Naeem M, Ansari A, Gill S, editors. Contaminants in agriculture: sources, impacts and management. Cham: Springer; 2020. p. 3–41. doi:10.1007/978-3-030-41552-5_1. [Google Scholar] [CrossRef]
4. Hiranmai RY, Neeraj A, Vats P. Improvement of soil health and crop production through utilization of organic wastes: a sustainable approach. Int J Recycl Org Waste Agric. 2022;13:132401. doi:10.57647/j.ijrowa.2024.1301.01. [Google Scholar] [CrossRef]
5. Rachid S, Ali-Ahmed FM, Kanane M, Hammoum A. Agricultural valorization of composts produced by recycling organic waste. Int J Recycl Org Waste Agric. 2023;12:209–19. doi:10.30486/ijrowa.2022.1949750.1398. [Google Scholar] [CrossRef]
6. Thygesen A, Tsapekos P, Alvarado-Morales M, Angelidaki I. Valorization of municipal organic waste into purified lactic acid. Bioresour Technol. 2021;342:125933. doi:10.1016/j.biortech.2021.125933. [Google Scholar] [PubMed] [CrossRef]
7. Gil A. Current insights into lignocellulose related waste valorization. Chem Eng J Adv. 2021;8:100186. doi:10.1016/j.ceja.2021.100186. [Google Scholar] [CrossRef]
8. Nardi S, Schiavon M, Francioso O. Chemical structure and biological activity of humic substances define their role as plant growth promoters. Molecules. 2021;26:2256. doi:10.3390/molecules26082256. [Google Scholar] [PubMed] [CrossRef]
9. Yang Y, Li T, Wang Y, Cheng H, Chang SX, Liang C, et al. Negative effects of multiple global change factors on soil microbial diversity. Soil Biol Biochem. 2021;156:108229. doi:10.1016/j.soilbio.2021.108229. [Google Scholar] [CrossRef]
10. Yildirim E, Ekinci M, Turan M, Ağar G, Dursun A, Kul R, et al. Humic + Fulvic acid mitigated Cd adverse effects on plant growth, physiology and biochemical properties of garden cress. Sci Rep. 2021;11:8040. doi:10.1038/s41598-021-86991-9. [Google Scholar] [PubMed] [CrossRef]
11. Sundberg C. Improving compost process efficiency by controlling aeration, temperature and pH (Doctoral Thesis). Swedish University of Agricultural Sciences: Swedis; 2005. [Google Scholar]
12. Xu Q, Ling N, Chen H, Duan Y, Wang S, Shen Q, et al. Long-term chemical-only fertilization induces a diversity decline and deep selection on the soil bacteria. Msystems. 2020;5(4):10–1128. doi:10.1128/mSystems.00337-20. [Google Scholar] [PubMed] [CrossRef]
13. Manu MK, Li D, Liwen L, Jun Z, Varjani S, Wong JWC. A review on nitrogen dynamics and mitigation strategies of food waste digestate composting. Bioresour Technol. 2021;334:125032. doi:10.1016/j.biortech.2021.125032. [Google Scholar] [PubMed] [CrossRef]
14. Moral R, Moreno-Caselles J, Perez-Murcia MD, Perez-Espinosa A, Rufete B, Paredes C. Characterisation of the organic matter pool in manures. Bioresour Technol. 2005;96:153–8. doi:10.1016/j.biortech.2004.05.003. [Google Scholar] [PubMed] [CrossRef]
15. Diacono M, Persiani A, Testani E, Montemurro F, Ciaccia C. Recycling agricultural wastes and by-products in organic farming: biofertilizer production, yield performance and carbon footprint analysis. Sustainability. 2019;11:1–17. doi:10.3390/su11143824. [Google Scholar] [CrossRef]
16. Bashir A, Rizwan M, Zia ur Rehman M, Zubair M, Riaz M, Qayyum MF, et al. Application of co-composted farm manure and biochar increased the wheat growth and decreased cadmium accumulation in plants under different water regimes. Chemosphere. 2020;246:125809. doi:10.1016/j.chemosphere.2019.125809. [Google Scholar] [PubMed] [CrossRef]
17. Weber J, Kocowicz A, Bekier J, Jamroz E, Tyszka R, Debicka M, et al. The effect of a sandy soil amendment with municipal solid waste (MSW) compost on nitrogen uptake efficiency by plants. Eur J Agron. 2014;54:54–60. doi:10.1016/j.eja.2013.11.014. [Google Scholar] [CrossRef]
18. Alluvione F, Fiorentino N, Bertora C, Zavattaro L, Fagnano M, Chiarandà FQ, et al. Short-term crop and soil response to C-friendly strategies in two contrasting environments. Eur J Agron. 2013;45:114–23. doi:10.1016/j.eja.2012.09.003. [Google Scholar] [CrossRef]
19. Clapp CE, Hayes MHB, Ciavatta C. Organic wastes in soils: biogeochemical and environmental aspects. Soil Biol Biochem. 2007;39:1239–43. doi:10.1016/j.soilbio.2006.12.001. [Google Scholar] [CrossRef]
20. Kumar S, Lawrence DM, Dirmeyer PA, Sheffield J. Less reliable water availability in the 21st century climate projections. Earth’s Future. 2014;2:152–60. doi:10.1002/2013ef000159. [Google Scholar] [CrossRef]
21. Hernández T, Chocano C, Moreno JL, García C. Towards a more sustainable fertilization: combined use of compost and inorganic fertilization for tomato cultivation. Agric Ecosyst Environ. 2014;196:178–84. doi:10.1016/j.agee.2014.07.006. [Google Scholar] [CrossRef]
22. Azis FA, Choo M, Suhaimi H, Abas PE. The effect of initial carbon to nitrogen ratio on kitchen waste composting maturity. Sustainability. 2023;15:6191. doi:10.3390/su15076191. [Google Scholar] [CrossRef]
23. Siles-Castellano AB, López MJ, Jurado MM, Suárez-Estrella F, López-González JA, Estrella-González MJ, et al. Industrial composting of low carbon/nitrogen ratio mixtures of agri-food waste and impact on compost quality. Bioresour Technol. 2020;316:123946. doi:10.1016/j.biortech.2020.123946. [Google Scholar] [PubMed] [CrossRef]
24. Zhang Z, Duan C, Liu Y, Li A, Hu X, Chen J, et al. Green waste and sewage sludge feeding ratio alters co-composting performance: emphasis on the role of bacterial community during humification. Bioresour Technol. 2023;380:129014. doi:10.1016/j.biortech.2023.129014. [Google Scholar] [PubMed] [CrossRef]
25. Ucaroglu S, Ozbek B. Bioconversion of organic wastes: treatment sludges, animal manures, and agricultural wastes. Biomass Convers Biorefin. 2024;1–12. doi:10.1007/s13399-024-05883-w. [Google Scholar] [CrossRef]
26. Keskinen R, Saastamoinen M, Nikama J, Särkijärvi S, Myllymäki M, Salo T, et al. Recycling nutrients from horse manure: effects of bedding type and its compostability. Agr Food Sci. 2017;26:68–78. doi:10.23986/afsci.60443. [Google Scholar] [CrossRef]
27. Mohd Sokri SS, Tee TP, Nurin AMY, Azhar S, Nayan N, Hj Suyub IB. Optimization on co-composting of horse manure with pineapple waste composition using response surface methodology (RSM). IOP Conf Ser Earth Environ Sci. 2023;1160:012026. doi:10.1088/1755-1315/1160/1/012026. [Google Scholar] [CrossRef]
28. Abdellah YAY, Shi ZJ, Sun SS, Sen LY, Yang X, Hou WT, et al. An assessment of composting conditions, humic matters formation and product maturity in response to different additives: a meta-analysis. J Clean Prod. 2022;366:132953. doi:10.1016/j.jclepro.2022.132953. [Google Scholar] [CrossRef]
29. Liang C, Das KC, McClendon RW. The influence of temperature and moisture contents regimes on the aerobic microbial activity of a biosolids composting blend. Bioresour Technol. 2003;86:131–7. doi:10.1016/S0960-8524(02)00153-0. [Google Scholar] [PubMed] [CrossRef]
30. Thomas C, Idler C, Ammon C, Amon T. Effects of the C/N ratio and moisture content on the survival of ESBL-producing Escherichia coli during chicken manure composting. Waste Manag. 2020;105:110–8. doi:10.1016/j.wasman.2020.01.031. [Google Scholar] [PubMed] [CrossRef]
31. AFNOR. Amendements organiques, dénominations, spécifications et marquages. Tome 1: Normalisation Dans Recueil Normes et Réglementatio; 1999. p. 15 (In Spanish). [Google Scholar]
32. AFNOR (Association Française de Normalisation). Amendements du sol et supports-Préparation des échantillons pour les essais physiques et chimiques, détermination de la teneur en matière sèche, du taux d'humidité et de la masse volumique compactée en laboratoire. NF EN 13040 2000. p. 1–15 (In France). [Google Scholar]
33. Aubert G. Méthodes d’analyse des Sols. Marseilles, France: Edition C.R.D.P.; 1978. p. 360 (In Spanish). [Google Scholar]
34. Olsen SR, Cole CV, Watanabe FS, LAD. Estimation of available phosphorus in soils by extraction with sodium bicarbonate. Washington DC, USA: USDA Circular 939 US Government Printing Office; 1954. [Google Scholar]
35. Tiquia SM. Reduction of compost phytotoxicity during the process of decomposition. Chemosphere. 2010;79:506–12. doi:10.1016/j.chemosphere.2010.02.040. [Google Scholar] [PubMed] [CrossRef]
36. Luo Y, Liang J, Zeng G, Chen M, Mo D, Li G, et al. Seed germination test for toxicity evaluation of compost: its roles, problems and prospects. Waste Manag. 2018;71:109–14. doi:10.1016/j.wasman.2017.09.023. [Google Scholar] [PubMed] [CrossRef]
37. Zucconi F, Pera A, Forte M, de Bertoldi M. Evaluating toxicity of immature compost. Biocycle. 1981;22:54–7. [Google Scholar]
38. Wang G, Kong Y, Liu Y, Li D, Zhang X, Yuan J, et al. Evolution of phytotoxicity during the active phase of co-composting of chicken manure, tobacco powder and mushroom substrate. Waste Manag. 2020;114:25–32. doi:10.1016/j.wasman.2020.06.034. [Google Scholar] [PubMed] [CrossRef]
39. Hsu JH, Lo SL. Chemical and spectroscopic analysis of organic matter transformations during composting of pig manure. Environ Pollut. 1999;104:189–96. doi:10.1016/S0269-7491(98)00193-6. [Google Scholar] [CrossRef]
40. Carballo T, Gil MV, Gómez X, González-Andrés F, Morán A. Characterization of different compost extracts using fourier-transform infrared spectroscopy (FTIR) and thermal analysis. Biodegradation. 2008;19:815–30. doi:10.1007/s10532-008-9184-4. [Google Scholar] [PubMed] [CrossRef]
41. Cai R, Zuo S, Cao X, Jiang X, Xu C. Effects of turning frequency on fermentation efficiency and microbial community metabolic function of sheep manure composting on the Qinghai-Tibet Plateau. Bioresour Bioprocess. 2023;10:53. doi:10.1186/s40643-023-00675-y. [Google Scholar] [PubMed] [CrossRef]
42. Tan Z, Zhu H, He X, Xi B, Tian Y, Sun X, et al. Effect of ventilation quantity on electron transfer capacity and spectral characteristics of humic substances during sludge composting. Environ Sci Pollut Res. 2022;29:70269–84. doi:10.1007/s11356-022-20808-8. [Google Scholar] [PubMed] [CrossRef]
43. Sikora LJ, Sowers MA. Effect of temperature control on the composting process. J Environ Qual. 1985;14:434–9. doi:10.2134/jeq1985.00472425001400030025x. [Google Scholar] [CrossRef]
44. Zhao XL, Li BQ, Ni JP, Xie DT. Effect of four crop straws on transformation of organic matter during sewage sludge composting. J Integr Agric. 2016;15:232–40. doi:10.1016/S2095-3119(14)60954-0. [Google Scholar] [CrossRef]
45. Yu S, Clark OG, Leonard JJ. A statistical method for the analysis of nonlinear temperature time series from compost. Bioresour Technol. 2008;99:1886–95. doi:10.1016/j.biortech.2007.03.061. [Google Scholar] [PubMed] [CrossRef]
46. Rao JR, Watabe M, Stewart TA, Millar BC, Moore JE. Pelleted organo-mineral fertilisers from composted pig slurry solids, animal wastes and spent mushroom compost for amenity grasslands. Waste Manag. 2007;27:1117–28. doi:10.1016/j.wasman.2006.06.010. [Google Scholar] [PubMed] [CrossRef]
47. Millner P, Ingram D, Mulbry W, Arikan OA. Pathogen reduction in minimally managed composting of bovine manure. Waste Manag. 2014;34:1992–9. doi:10.1016/j.wasman.2014.07.021. [Google Scholar] [PubMed] [CrossRef]
48. Ravindran B, Sekaran G. Effect of aeration rate, C/N ratio and moisture content on the stability and maturity of compost. Waste Manag. 2010;30:2622–30. doi:10.1016/j.wasman.2010.07.013. [Google Scholar] [CrossRef]
49. Ma Y, Zhang JY, Wong MH. Microbial activity during composting of anthracene-contaminated soil. Chemosphere. 2003;52:1505–13. doi:10.1016/S0045-6535(03)00489-2. [Google Scholar] [PubMed] [CrossRef]
50. Xu Z, Li G, Huda N, Zhang B, Wang M, Luo W. Effects of moisture and carbon/nitrogen ratio on gaseous emissions and maturity during direct composting of cornstalks used for filtration of anaerobically digested manure centrate. Bioresour Technol. 2020;298:122503. doi:10.1016/j.biortech.2019.122503. [Google Scholar] [PubMed] [CrossRef]
51. Alkarimiah R, Suja F. Effects of technical factors towards achieving the thermophilic temperature stage in composting process and the benefits of closed rector system compared to conventional method–a mini review. Appl Ecol Environ Res. 2019;17:9979–96. doi:10.15666/aeer/1704_99799996. [Google Scholar] [CrossRef]
52. Muktadirul Bari Chowdhury AKM, Akratos CS, Vayenas DV, Pavlou S. Olive mill waste composting: a review. Int Biodeterior Biodegrad. 2013;85:108–19. doi:10.1016/j.ibiod.2013.06.019. [Google Scholar] [CrossRef]
53. Asses N, Farhat A, Cherif S, Hamdi M, Bouallagui H. Comparative study of sewage sludge co-composting with olive mill wastes or green residues: process monitoring and agriculture value of the resulting composts. Process Saf Environ. 2018;114:25–35. doi:10.1016/j.psep.2017.12.006. [Google Scholar] [CrossRef]
54. Richard TL, (Bert) Hamelers HVM, Veeken A, Silva T. Moisture relationships in composting processes. Compost Sci Util. 2002;10:286–302. doi:10.1080/1065657X.2002.10702093. [Google Scholar] [CrossRef]
55. Bernal MP, Alburquerque JA, Moral R. Composting of animal manures and chemical criteria for compost maturity assessment. A review. Bioresour Technol. 2009;100:5444–53. doi:10.1016/j.biortech.2008.11.027. [Google Scholar] [PubMed] [CrossRef]
56. Onwosi CO, Igbokwe VC, Odimba JN, Eke IE, Nwankwoala MO, Iroh IN, et al. Composting technology in waste stabilization: on the methods, challenges and future prospects. J Environ Manage. 2017;190:140–57. doi:10.1016/j.jenvman.2016.12.051. [Google Scholar] [PubMed] [CrossRef]
57. Kulcu R, Yaldiz O. Determination of aeration rate and kinetics of composting some agricultural wastes. Bioresour Technol. 2004;93:49–57. doi:10.1016/j.biortech.2003.10.007. [Google Scholar] [PubMed] [CrossRef]
58. Mbuligwe SE, Kassenga GR, Kaseva ME, Chaggu EJ. Potential and constraints of composting domestic solid waste in developing countries: findings from a pilot study in Dar es Salaam. Tanzania Resour Conserv Recy. 2002;36:45–59. doi:10.1016/S0921-3449(02)00009-5. [Google Scholar] [CrossRef]
59. Sadaka S, El-Taweel A. Effects of aeration and C: n ratio on household waste composting in Egypt. Compost Sci Util. 2003;11:36–40. doi:10.1080/1065657X.2003.10702107. [Google Scholar] [CrossRef]
60. Antizar-Ladislao B, Lopez-Real J, Beck AJ. In-vessel composting-bioremediation of aged coal tar soil: effect of temperature and soil/green waste amendment ratio. Envir Int. 2005;31:173–8. doi:10.1016/j.envint.2004.09.012. [Google Scholar] [PubMed] [CrossRef]
61. Huang GF, Wong JWC, Wu QT, Nagar BB. Effect of C/N on composting of pig manure with sawdust. Waste Manag. 2004;24:805–13. doi:10.1016/j.wasman.2004.03.011. [Google Scholar] [PubMed] [CrossRef]
62. Mobaligh M, Meddich A, Imziln B, Fares K. The use of phosphate washing sludge to recover by composting the leachate from the controlled landfill. Processes. 2021;9:1735. doi:10.3390/pr9101735. [Google Scholar] [CrossRef]
63. Goyal S, Dhull SK, Kapoor KK. Chemical and biological changes during composting of different organic wastes and assessment of compost maturity. Bioresour Technol. 2005;96:1584–91. doi:10.1016/j.biortech.2004.12.012. [Google Scholar] [PubMed] [CrossRef]
64. Koutsoumanis K, Allende A, Bolton D, Bover-Cid S, Chemaly M, Herman L, et al. Evaluation of alternative methods of tunnel composting (submitted by the European Composting Network) II. EFSA J. 2024;22:1–37. doi:10.2903/j.efsa.2024.8745. [Google Scholar] [PubMed] [CrossRef]
65. Bohm K. Compost quality determination using infrared spectroscopy and multivariate data analysis. Universität Für Bodenkultur Wien: Wien; 2009. [Google Scholar]
66. Siebielec S, Marzec-Grządziel A, Siebielec G, Ukalska-Jaruga A, Kozieł M, Gałązka A, et al. Microbial community response to various types of exogenous organic matter applied to soil. Int J Mol Sci. 2023;24:1–22. doi:10.3390/ijms241914559. [Google Scholar] [PubMed] [CrossRef]
67. Du G, Feng W, Cai H, Ma Z, Liu X, Yuan C, et al. Exogenous enzyme amendment accelerates maturity and changes microflora succession in horse and wildlife animal manure co-composting. Environ Sci Pollut Res. 2021;28:21610–20. doi:10.1007/s11356-020-11568-4. [Google Scholar] [PubMed] [CrossRef]
68. Chen X, Du G, Wu C, Li Q, Zhou P, Shi J, et al. Effect of thermophilic microbial agents on nitrogen transformation, nitrogen functional genes, and bacterial communities during bean dregs composting. Environ Sci Pollut Res. 2022;29:31846–60. doi:10.1007/s11356-021-17946-w. [Google Scholar] [PubMed] [CrossRef]
69. Grenier V, Gonzalez E, Brereton NJB, Pitre FE. Dynamics of bacterial and archaeal communities during horse bedding and green waste composting. PeerJ. 2023;11:e15239. doi:10.7717/peerj.15239. [Google Scholar] [PubMed] [CrossRef]
70. Bello A, Han Y, Zhu H, Deng L, Yang W, Meng Q, et al. Microbial community composition, co-occurrence network pattern and nitrogen transformation genera response to biochar addition in cattle manure-maize straw composting. Sci Total Environ. 2020;721:137759. doi:10.1016/j.scitotenv.2020.137759. [Google Scholar] [PubMed] [CrossRef]
71. Hou T, Zhou Y, Du R, Liu J, Li W, Zhang S, et al. Insights into effects of thermotolerant nitrifying and sulfur-oxidizing inoculants on nitrogen-sulfur co-metabolism in sewage sludge composting. J Environ Sci. 2024;144:76–86. doi:10.1016/j.jes.2023.08.036. [Google Scholar] [PubMed] [CrossRef]
72. Tian H, Liu J, Zhang Y, Yue P. A novel integrated industrial-scale biological reactor for odor control in a sewage sludge composting facility: performance, pollutant transformation, and bioaerosol emission mechanism. Waste Manag. 2023;164:9–19. doi:10.1016/j.wasman.2023.03.021. [Google Scholar] [PubMed] [CrossRef]
73. Lei L, Gu J, Wang X, Song Z, Wang J, Yu J, et al. Microbial succession and molecular ecological networks response to the addition of superphosphate and phosphogypsum during swine manure composting. J Environ Manage. 2021;279:111560. doi:10.1016/j.jenvman.2020.111560. [Google Scholar] [PubMed] [CrossRef]
74. Yin Z, Zhang L, Li R. Effects of additives on physical, chemical, and microbiological properties during green waste composting. Bioresour Technol. 2021;340:125719. doi:10.1016/j.biortech.2021.125719. [Google Scholar] [PubMed] [CrossRef]
75. Qiu X, Zhou G, Zhang J, Wang W. Microbial community responses to biochar addition when a green waste and manure mix are composted: a molecular ecological network analysis. Bioresour Technol. 2019;273:666–71. doi:10.1016/j.biortech.2018.12.001. [Google Scholar] [PubMed] [CrossRef]
76. Lazcano C, Gómez-Brandón M, Domínguez J. Comparison of the effectiveness of composting and vermicomposting for the biological stabilization of cattle manure. Chemosphere. 2008;72:1013–9. doi:10.1016/j.chemosphere.2008.04.016. [Google Scholar] [PubMed] [CrossRef]
77. Zhang S, Wang J, Chen X, Gui J, Sun Y, Wu D. Industrial-scale food waste composting: effects of aeration frequencies on oxygen consumption, enzymatic activities and bacterial community succession. Bioresour Technol. 2021;320:124357. [Google Scholar] [PubMed]
78. Bouhia Y, Hafidi M, Ouhdouch Y, El Boukhari MEM, El Fels L, Zeroual Y, et al. Microbial community succession and organic pollutants removal during olive mill waste sludge and green waste co-composting. Front Microbiol. 2022;12:1–12. doi:10.3389/fmicb.2021.814553. [Google Scholar] [PubMed] [CrossRef]
79. Milanović V, Osimani A, Cardinali F, Taccari M, Garofalo C, Clementi F, et al. Effect of inoculated azotobacteria and Phanerochaete chrysosporium on the composting of olive pomace: microbial community dynamics and phenols evolution. Sci Rep. 2019;9:16966. doi:10.1038/s41598-019-53313-z. [Google Scholar] [PubMed] [CrossRef]
80. Tortosa G, Fernández-González AJ, Lasa AV, Aranda E, Torralbo F, González-Murua C, et al. Involvement of the metabolically active bacteria in the organic matter degradation during olive mill waste composting. Sci Total Environ. 2021;789:147975. doi:10.1016/j.scitotenv.2021.147975. [Google Scholar] [PubMed] [CrossRef]
81. Pandiyan B, Mangottiri V, Narayanan N. Carbon transformations of biochar based co-composting—a review. Mini Rev Org Chem. 2021;18:465–78. doi:10.2174/1570193X17999200928221205. [Google Scholar] [CrossRef]
82. Wang H, Lu Y, Xu J, Liu X, Sheng L. Effects of additives on nitrogen transformation and greenhouse gases emission of co-composting for deer manure and corn straw. Environ Sci Pollut Res. 2021;28:13000–20. doi:10.1007/s11356-020-11302-0. [Google Scholar] [PubMed] [CrossRef]
83. Greff B, Szigeti J, Nagy Á., Lakatos E, Varga L. Influence of microbial inoculants on co-composting of lignocellulosic crop residues with farm animal manure: a review. J Environ Manage. 2022;302:114088. doi:10.1016/j.jenvman.2021.114088. [Google Scholar] [PubMed] [CrossRef]
84. Su J, Zhan Y, Chang Y, Chang S, Luo Y, Chen P, et al. Phosphate additives promote humic acid carbon and nitrogen skeleton formation by regulating precursors and composting bacterial communities. Bioresour Technol. 2024;399:130617. doi:10.1016/j.biortech.2024.130617. [Google Scholar] [PubMed] [CrossRef]
85. Kostic B, Stevanovic G, Lutovac M, Lutovac B, Ketin S, Biocanin R. Animal manure and environmrnt. Fresenius Environ Bull. 2020;29:1289–96. [Google Scholar]
86. Du J, Zhang Y, Qu M, Yin Y, Fan K, Hu B, et al. Effects of biochar on the microbial activity and community structure during sewage sludge composting. Bioresour Technol. 2019;272:171–9. doi:10.1016/j.biortech.2018.10.020. [Google Scholar] [PubMed] [CrossRef]
87. Ma C, Hu B, Wei MB, Zhao JH, Zhang HZ. Influence of matured compost inoculation on sewage sludge composting: enzyme activity, bacterial and fungal community succession. Bioresour Technol. 2019;294:122165. doi:10.1016/j.biortech.2019.122165. [Google Scholar] [PubMed] [CrossRef]
88. Urra J, Alkorta I, Lanzén A, Mijangos I, Garbisu C. The application of fresh and composted horse and chicken manure affects soil quality, microbial composition and antibiotic resistance. Appl Soil Ecol. 2019;135:73–84. doi:10.1016/j.apsoil.2018.11.005. [Google Scholar] [CrossRef]
89. Zhang J, Chen J, Ma R, Kumar V, Wah Tong Y, He Y, et al. Mesophilic and thermophilic anaerobic digestion of animal manure: integrated insights from biogas productivity, microbial viability and enzymatic activity. Fuel. 2022;320:123990. doi:10.1016/j.fuel.2022.123990. [Google Scholar] [CrossRef]
90. Wei Y, Wu D, Wei D, Zhao Y, Wu J, Xie X, et al. Improved lignocellulose-degrading performance during straw composting from diverse sources with actinomycetes inoculation by regulating the key enzyme activities. Bioresour Technol. 2019;271:66–74. doi:10.1016/j.biortech.2018.09.081. [Google Scholar] [PubMed] [CrossRef]
91. Sun C, Wei Y, Kou J, Han Z, Shi Q, Liu L, et al. Improve spent mushroom substrate decomposition, bacterial community and mature compost quality by adding cellulase during composting. J Clean Prod. 2021;299:126928. doi:10.1016/j.jclepro.2021.126928. [Google Scholar] [CrossRef]
92. Ayilara MS, Olanrewaju OS, Babalola OO, Odeyemi O. Waste management through composting: challenges and potentials. Sustainability. 2020;12:1–23. doi:10.3390/su12114456. [Google Scholar] [CrossRef]
93. Zhao B, Wang Y, Ma L, Li Y, Deng Y, Chen X, et al. Adding an appropriate proportion of phosphogypsum ensured rice husk and urea composting to promote the compost as substrate utilization. Bioresour Technol. 2022;344:126301. doi:10.1016/j.biortech.2021.126301. [Google Scholar] [PubMed] [CrossRef]
94. Yang F, Li G, Shi H, Wang Y. Effects of phosphogypsum and superphosphate on compost maturity and gaseous emissions during kitchen waste composting. Waste Manag. 2015;36:70–6. doi:10.1016/j.wasman.2014.11.012. [Google Scholar] [PubMed] [CrossRef]
95. Hachicha R, Hachicha S, Trabelsi I, Woodward S, Mechichi T. Evolution of the fatty fraction during co-composting of olive oil industry wastes with animal manure: maturity assessment of the end product. Chemosphere. 2009;75:1382–6. doi:10.1016/j.chemosphere.2009.02.064. [Google Scholar] [PubMed] [CrossRef]
96. Chalhoub M, Garnier P, Coquet Y, Mary B, Lafolie F, Houot S. Increased nitrogen availability in soil after repeated compost applications: use of the PASTIS model to separate short and long-term effects. Soil Biol Biochem. 2013;65:144–57. doi:10.1016/j.soilbio.2013.05.023. [Google Scholar] [CrossRef]
97. Masó MA, Blasi AB. Evaluation of composting as a strategy for managing organic wastes from a municipal market in Nicaragua. Bioresour Technol. 2008;99:5120–4. doi:10.1016/j.biortech.2007.09.083. [Google Scholar] [PubMed] [CrossRef]
98. Zhang L, Sun X. Influence of bulking agents on physical, chemical, and microbiological properties during the two-stage composting of green waste. Waste Manag. 2016;48:115–26. doi:10.1016/j.wasman.2015.11.032. [Google Scholar] [PubMed] [CrossRef]
99. Senesi N, Plaza C. Role of humification processes in recycling organic wastes of various nature and sources as soil amendments. Clean Soil Air Water. 2007;35:26–41. doi:10.1002/clen.200600018. [Google Scholar] [CrossRef]
100. Juarez FDM, Prähauser B, Walter A, Insam H, Franke-Whittle IH. Co-composting of biowaste and wood ash, influence on a microbially driven-process. Waste Manag. 2015;46:155–64. doi:10.1016/j.wasman.2015.09.015. [Google Scholar] [PubMed] [CrossRef]
101. Som MP, Lemée L, Amblès A. Stability and maturity of a green waste and biowaste compost assessed on the basis of a molecular study using spectroscopy, thermal analysis, thermodesorption and thermochemolysis. Bioresour Technol. 2009;100:4404–16. doi:10.1016/j.biortech.2009.04.019. [Google Scholar] [PubMed] [CrossRef]
102. Rich N, Bharti A. Assessment of different types of in-vessel composters and its effect on stabilization of MSW compost. Int J Eng Res. 2015;2:805–13. [Google Scholar]
103. Li Y, Luo W, Li G, Wang K, Gong X. Performance of phosphogypsum and calcium magnesium phosphate fertilizer for nitrogen conservation in pig manure composting. Bioresour Technol. 2018;250:53–9. doi:10.1016/j.biortech.2017.07.172. [Google Scholar] [PubMed] [CrossRef]
104. Cáceres R, Malińska K, Marfà O. Nitrification within composting: a review. Waste Manag. 2018;72:119–37. doi:10.1016/j.wasman.2017.10.049. [Google Scholar] [PubMed] [CrossRef]
105. Li R, Wang Q, Zhang Z, Zhang G, Li Z, Wang L, et al. Nutrient transformation during aerobic composting of pig manure with biochar prepared at different temperatures. Environ Technol. 2015;36:815–26. doi:10.1080/09593330.2014.963692. [Google Scholar] [PubMed] [CrossRef]
106. Paredes C, Roig A, Bernal MP, Sánchez-Monedero MA, Cegarra J. Evolution of organic matter and nitrogen during co-composting of olive mill wastewater with solid organic wastes. Biol Fertil Soils. 2000;32:222–7. doi:10.1007/s003740000239. [Google Scholar] [CrossRef]
107. Yang F, Li GX, Yang QY, Luo WH. Effect of bulking agents on maturity and gaseous emissions during kitchen waste composting. Chemosphere. 2013;93:1393–9. doi:10.1016/j.chemosphere.2013.07.002. [Google Scholar] [PubMed] [CrossRef]
108. Yuan J, Chadwick D, Zhang D, Li G, Chen S, Luo W, et al. Effects of aeration rate on maturity and gaseous emissions during sewage sludge composting. Waste Manag. 2016;56:403–10. doi:10.1016/j.wasman.2016.07.017. [Google Scholar] [PubMed] [CrossRef]
109. Lei L, Gu J, Wang X, Song Z, Yu J, Wang J, et al. Effects of phosphogypsum and medical stone on nitrogen transformation, nitrogen functional genes, and bacterial community during aerobic composting. Sci Total Environ. 2021;753:141746. doi:10.1016/j.scitotenv.2020.141746. [Google Scholar] [PubMed] [CrossRef]
110. Nie E, Gao D, Zheng G. Effects of lactic acid on modulating the ammonia emissions in co-composts of poultry litter with slaughter sludge. Bioresour Technol. 2020;315:123812. doi:10.1016/j.biortech.2020.123812. [Google Scholar] [PubMed] [CrossRef]
111. Xu Z, Liang W, Zhang X, Yang X, Zhou S, Li R, et al. Effects of magnesite on nitrogen conversion and bacterial community during pig manure composting. Bioresour Technol. 2023;384:129325. doi:10.1016/j.biortech.2023.129325. [Google Scholar] [PubMed] [CrossRef]
112. Wang Q, Awasthi MK, Ren X, Zhao J, Li R, Wang Z, et al. Combining biochar, zeolite and wood vinegar for composting of pig manure: the effect on greenhouse gas emission and nitrogen conservation. Waste Manag. 2018;74:221–30. doi:10.1016/j.wasman.2018.01.015. [Google Scholar] [PubMed] [CrossRef]
113. Pereira JLS, Costa T, Figueiredo V, Marques F, Perdigão A, Brás I, et al. Influence of different agro-food waste on ammonia and greenhouse gas emissions during composting. Agronomy. 2024;14:220. doi:10.3390/agronomy14010220. [Google Scholar] [CrossRef]
114. Jiang J, Huang Y, Liu X, Huang H. The effects of apple pomace, bentonite and calcium superphosphate on swine manure aerobic composting. Waste Manag. 2014;34:1595–602. doi:10.1016/j.wasman.2014.05.002. [Google Scholar] [PubMed] [CrossRef]
115. Asch J, Johnson K, Mondal S, Asch F. Comprehensive assessment of extraction methods for plant tissue samples for determining sodium and potassium via flame photometer and chloride via automated flow analysis. J Soil Sci Plant Nutr. 2022;185:308–16. doi:10.1002/jpln.202100344. [Google Scholar] [CrossRef]
116. Yang Y, Yin Z, Li L, Li Y, Liu Y, Luo Y, et al. Effects of dicyandiamide, phosphogypsum and superphosphate on greenhouse gas emissions during pig manure composting. Sci Total Environ. 2022;846:157487. doi:10.1016/j.scitotenv.2022.157487. [Google Scholar] [PubMed] [CrossRef]
117. Zheng G, Wang X, Chen T, Yang J, Yang J, Liu J, et al. Passivation of lead and cadmium and increase of the nutrient content during sewage sludge composting by phosphate amendments. Environ Res. 2020;185:109431. doi:10.1016/j.envres.2020.109431. [Google Scholar] [PubMed] [CrossRef]
118. Samet M, Karray F, Mhiri N, Kamoun L, Sayadi S, Gargouri-Bouzid R. Effect of phosphogypsum addition in the composting process on the physico-chemical proprieties and the microbial diversity of the resulting compost tea. Environ Sci Pollut Res. 2019;26:21404–15. doi:10.1007/s11356-019-05327-3. [Google Scholar] [PubMed] [CrossRef]
119. Mohamed TA, Wu J, Zhao Y, Elgizawy N, El Kholy M, Yanga H, et al. Insights into enzyme activity and phosphorus conversion during kitchen waste composting utilizing phosphorus-solubilizing bacterial inoculation. Bioresour Technol. 2022;362:127823. [Google Scholar] [PubMed]
120. Zhan Y, Zhang Z, Ma T, Zhang X, Wang R, Liu Y, et al. Phosphorus excess changes rock phosphate solubilization level and bacterial community mediating phosphorus fractions mobilization during composting. Bioresour Technol. 2021;337:125433. [Google Scholar] [PubMed]
121. Jin Y, Yang D, Wu Y, Zhou F, Yu J, Chi R, et al. Preparation of biofertilizer with phosphogypsum and straw: microbial community changes and plant growth effects. J Soil Sci Plant Nutr. 2024;24:3873–88. doi:10.1007/s42729-024-01806-w. [Google Scholar] [CrossRef]
122. Lakhtar H, Kharbouch B, Askarne L, Hamza MA, El Mousadik A. Effect of thermophilic composting duration on vermiconversion of olive mill wastewater using Eisenia andrei. Bioresour. Technol Rep. 2023;23:101560. doi:10.1016/j.biteb.2023.101560. [Google Scholar] [CrossRef]
123. Song Z-W, Sheng T, Deng W-J, Wang J. Investigation of rice straw and kitchen waste degradation through vermicomposting. J Environ Manage. 2019;243:269–72. doi:10.1016/j.jenvman.2019.04.126. [Google Scholar] [PubMed] [CrossRef]
124. Carmona I, Aguirre I, Griffith DM, García-Borrego A. Towards a circular economy in virgin olive oil production: valorization of the olive mill waste (OMW) alpeorujo through polyphenol recovery with natural deep eutectic solvents (NADESs) and vermicomposting. Sci Total Environ. 2023;872:162198. doi:10.1016/j.scitotenv.2023.162198. [Google Scholar] [PubMed] [CrossRef]
125. Mekersi N, Kadi K, Casini S, Addad D, Bazri KE, Marref SE, et al. Effects of single and combined olive mill wastewater and olive mill pomace on the growth, reproduction, and survival of two earthworm species (Aporrectodea trapezoides, Eisenia fetida). Appl Soil Ecol. 2021;168:104123. doi:10.1016/j.apsoil.2021.104123. [Google Scholar] [CrossRef]
126. Mondal A, Goswami L, Hussain N, Barman S, Kalita E, Bhattacharyya P, et al. Detoxification and eco-friendly recycling of brick kiln coal ash using Eisenia fetida: a clean approach through vermitechnology. Chemosphere. 2020;244:125470. doi:10.1016/j.chemosphere.2019.125470. [Google Scholar] [PubMed] [CrossRef]
127. Medina J, Monreal CM, Antilén M, Calabi-Floody M, Velasco-Molina M, Meier S, et al. Influence of inorganic additives on wheat straw composting: characterization and structural composition of organic matter derived from the process. J Environ Manage. 2020;260:110137. doi:10.1016/j.jenvman.2020.110137. [Google Scholar] [PubMed] [CrossRef]
128. Dimambro ME, Lillywhite RD, Rahn CR, Dimambro ME. The physical, chemical and microbial characteristics of biodegradable municipal waste derived composts. Compost Sci Util. 2007;15:243–52. doi:10.1080/1065657X.2007.10702340. [Google Scholar] [CrossRef]
129. Wang X, Pan S, Zhang Z, Lin X, Zhang Y, Chen S. Effects of the feeding ratio of food waste on fed-batch aerobic composting and its microbial community. Bioresour Technol. 2017;224:397–404. doi:10.1016/j.biortech.2016.11.076. [Google Scholar] [PubMed] [CrossRef]
130. Rashad FM, Saleh WD, Moselhy MA. Bioconversion of rice straw and certain agro-industrial wastes to amendments for organic farming systems: 1. Composting, quality, stability and maturity indices. Bioresour Technol. 2010;101:5952–60. doi:10.1016/j.biortech.2010.02.103. [Google Scholar] [PubMed] [CrossRef]
131. Yu K, Li S, Sun X, Kang Y. Maintaining the ratio of hydrosoluble carbon and hydrosoluble nitrogen within the optimal range to accelerate green waste composting. Waste Manag. 2020;105:405–13. doi:10.1016/j.wasman.2020.02.023. [Google Scholar] [PubMed] [CrossRef]
132. Moharana PC, Biswas DR. Assessment of maturity indices of rock phosphate enriched composts using variable crop residues. Bioresour Technol. 2016;222:1–13. doi:10.1016/j.biortech.2016.09.097. [Google Scholar] [PubMed] [CrossRef]
133. Young BJ, Rizzo PF, Riera NI, Della TV, López VA, Molina CD, et al. Development of phytotoxicity indexes and their correlation with ecotoxicological, stability and physicochemical parameters during passive composting of poultry manure. Waste Manag. 2016;54:101–9. doi:10.1016/j.wasman.2016.05.001. [Google Scholar] [PubMed] [CrossRef]
134. Qian X, Shen G, Wang Z, Guo C, Liu Y, Lei Z, et al. Co-composting of livestock manure with rice straw: characterization and establishment of maturity evaluation system. Waste Manag. 2014;34:530–5. doi:10.1016/j.wasman.2013.10.007. [Google Scholar] [PubMed] [CrossRef]
135. Aggelis G, Ehaliotis C, Nerud F, Stoychev I, Lyberatos G, Zervakis G. Evaluation of white-rot fungi for detoxification and decolorization of effluents from the green olive debittering process. Appl Microbiol Biotechnol. 2002;59:353–60. doi:10.1007/s00253-002-1005-9. [Google Scholar] [PubMed] [CrossRef]
136. Siles-Castellano AB, López MJ, López-González JA, Suárez-Estrella F, Jurado MM, Estrella-González MJ, et al. Comparative analysis of phytotoxicity and compost quality in industrial composting facilities processing different organic wastes. J Clean Prod. 2020;252:119820. doi:10.1016/j.jclepro.2019.119820. [Google Scholar] [CrossRef]
137. Barje F, El Fels L, El Hajjouji H, Winterton P, Hafidi M. Biodegradation of organic compounds during co-composting of olive oil mill waste and municipal solid waste with added rock phosphate. Environ Technol. 2013;34:2965–75. doi:10.1080/09593330.2013.796009. [Google Scholar] [PubMed] [CrossRef]
138. Guo XX, Liu HT, Wu SB. Humic substances developed during organic waste composting: formation mechanisms, structural properties, and agronomic functions. Sci Total Environ. 2019;662:501–10. doi:10.1016/j.scitotenv.2019.01.137. [Google Scholar] [PubMed] [CrossRef]
139. Yangui T, Dhouib A, Rhouma A, Sayadi S. Potential of hydroxytyrosol-rich composition from olive mill wastewater as a natural disinfectant and its effect on seeds vigour response. Food Chem. 2009;117:1–8. doi:10.1016/j.foodchem.2009.03.069. [Google Scholar] [CrossRef]
140. Ait Baddi G, Alburquerque JA, Gonzálvez J, Cegarra J, Hafidi M. Chemical and spectroscopic analyses of organic matter transformations during composting of olive mill wastes. Int Biodeterior Biodegrad. 2004;54:39–44. doi:10.1016/j.ibiod.2003.12.004. [Google Scholar] [CrossRef]
141. Hagemann N, Subdiaga E, Orsetti S, de la Rosa JM, Knicker H, Schmidt HP, et al. Effect of biochar amendment on compost organic matter composition following aerobic compositing of manure. Sci Total Environ. 2018;613–614:20–9. doi:10.1016/j.scitotenv.2017.08.161. [Google Scholar] [PubMed] [CrossRef]
142. Lv B, Xing M, Yang J, Qi W, Lu Y. Chemical and spectroscopic characterization of water extractable organic matter during vermicomposting of cattle dung. Bioresour Technol. 2013;132:320–6. doi:10.1016/j.biortech.2013.01.006. [Google Scholar] [PubMed] [CrossRef]
143. Droussi Z, D’orazio V, Provenzano MR, Hafidi M, Ouatmane A. Study of the biodegradation and transformation of olive-mill residues during composting using FTIR spectroscopy and differential scanning calorimetry. J Hazard Mater. 2009;164:1281–5. doi:10.1016/j.jhazmat.2008.09.081. [Google Scholar] [PubMed] [CrossRef]
144. Wang K, Li W, Gong XJ, Li Y, Wu C, Ren N. Spectral study of dissolved organic matter in biosolid during the composting process using inorganic bulking agent: UV-vis, GPC, FTIR and EEM. Int Biodeterior Biodegrad. 2013;85:617–23. doi:10.1016/j.ibiod.2013.03.033. [Google Scholar] [CrossRef]
145. Elouear Z, Bouzid J, Boujelben N, Feki M, Jamoussi F, Montiel A. Heavy metal removal from aqueous solutions by activated phosphate rock. J Hazard Mater. 2008;156:412–20. doi:10.1016/j.jhazmat.2007.12.036. [Google Scholar] [PubMed] [CrossRef]
146. Ramdani N, Lousdad A, Hamou A. Study of the biodegradation and fertility of the co-composting produced from sewage sludge and green waste and its effects on the speciation of heavy metals. J Mater Environ Sci. 2015;6:1310–20. [Google Scholar]
147. Li D, Jiang W, Ye Y, Luo J, Zhou X, Yang L, et al. A change in substance and microbial community structure during the co-composting of kitchen waste anaerobic digestion effluent, sewage sludge and Chinese medicine residue. Sci Total Environ. 2024;907:167679. doi:10.1016/j.scitotenv.2023.167679. [Google Scholar] [PubMed] [CrossRef]
148. Cui Y, Zeng Y, Hu H, Zhang Y, Wang D, Feng D. Biochar, phosphate, and magnesium oxide in seaweed and cornstarch dregs co-composting: enhancing organic matter degradation, humification, and nitrogen retention. Waste Manag. 2024;187:207–17. doi:10.1016/j.wasman.2024.07.024. [Google Scholar] [PubMed] [CrossRef]
149. Wang W, Hou Y, Huang W, Liu X, Wen P, Wang Y, et al. Alkali lignin and sodium lignosulfonate additives promote the formation of humic substances during paper mill sludge composting. Bioresour Technol. 2021;320:124361. doi:10.1016/j.biortech.2020.124361. [Google Scholar] [PubMed] [CrossRef]
150. Zhang D, Xu Z, Wang G, Huda N, Li G, Luo W. Insights into characteristics of organic matter during co-biodrying of sewage sludge and kitchen waste under different aeration intensities. Environ Technol Innov. 2020;20:101117. doi:10.1016/j.eti.2020.101117. [Google Scholar] [CrossRef]
151. Liu X, Hou Y, Li Z, Yu Z, Tang J, Wang Y, et al. Hyperthermophilic composting of sewage sludge accelerates humic acid formation: elemental and spectroscopic evidence. Waste Manag. 2020;103:342–51. doi:10.1016/j.wasman.2019.12.053. [Google Scholar] [PubMed] [CrossRef]
152. Ouatmane A, Provenzano M, Hafidi M, Senesi N. Compost maturity assessment using calorimetry, spectroscopy and chemical analysis. Compost Sci Util. 2000;8:124. [Google Scholar]
153. Li Q, Lu Y, Guo X, Shan G, Huang J. Properties and evolution of dissolved organic matter during co-composting of dairy manure and Chinese herbal residues. Environ Sci Pollut Res. 2017;24:8629–36. doi:10.1007/s11356-017-8540-5. [Google Scholar] [PubMed] [CrossRef]
154. Lanno M, Klavins M, Purmalis O, Shanskiy M, Kisand A, Kriipsalu M. Properties of humic substances in composts comprised of different organic source material. Agriculture. 2022;12:1797. doi:10.3390/agriculture12111797. [Google Scholar] [CrossRef]
155. Martín-Mata J, Lahoz-Ramos C, Bustamante MA, Marhuenda-Egea FC, Moral R, Santos A, et al. Thermal and spectroscopic analysis of organic matter degradation and humification during composting of pig slurry in different scenarios. Environ Sci Pollut Res. 2016;23:17357–69. doi:10.1007/s11356-016-6838-3. [Google Scholar] [PubMed] [CrossRef]
156. Huang GF, Wu QT, Wong JWC, Nagar BB. Transformation of organic matter during co-composting of pig manure with sawdust. Bioresour Technol. 2006;97:1834–42. doi:10.1016/j.biortech.2005.08.024. [Google Scholar] [PubMed] [CrossRef]
157. Fersi M, Hajji R, Mbarki K, Louati I, Jedidi N, Hassen A, et al. Spectroscopic and microscopic characterization of humic acids from composts made by co-composting of green waste, spent coffee and OMWW sludge. Environ Technol. 2024;2024:1–14. doi:10.1080/09593330.2024.2320622. [Google Scholar] [PubMed] [CrossRef]
Cite This Article
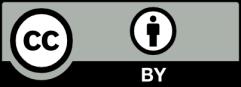
This work is licensed under a Creative Commons Attribution 4.0 International License , which permits unrestricted use, distribution, and reproduction in any medium, provided the original work is properly cited.