Open Access
ARTICLE
Inhibitory Effect of Ponkan (Citrus reticulata ‘Ponkan’) Flavonoids on Oral Ulcers through Jak2-Stat3 Signaling Pathway
1 Quzhou Academy of Agriculture and Forestry Science, Quzhou, 324003, China
2 Laboratory of Fruit Quality Biology/The State Agriculture Ministry Laboratory of Horticultural Plant Growth, Development and Quality Improvement, Fruit Science Institute, College of Agriculture and Biotechnology, Zhejiang University, Hangzhou, 310058, China
3 Hainan Institute of Zhejiang University, Sanya, 572025, China
4 Stomatology Hospital, Zhejiang University School of Medicine, Hangzhou, 310006, China
* Corresponding Authors: Dengliang Wang. Email: ; Yue Wang. Email:
(This article belongs to the Special Issue: Phytochemical and Pharmacological Research in Medicinal Plants)
Phyton-International Journal of Experimental Botany 2024, 93(9), 2499-2518. https://doi.org/10.32604/phyton.2024.054449
Received 28 May 2024; Accepted 30 July 2024; Issue published 30 September 2024
Abstract
Oral ulcers, a common mucosal affliction, severely diminish patients’ quality of life due to pain and discomfort. Despite existing treatments, the frequent recurrence of oral ulcers underscores the need for more effective therapeutic options. This study aimed to investigate the inhibitory effects of Ponkan (Citrus reticulata ‘Ponkan’) extracts and their flavonoid components on oral ulcers, exploring their potential as a natural therapy. Twenty different Ponkan cultivars were selected for flavonoid extraction and analysis. Ultra-performance liquid chromatography (UPLC) and high-resolution mass spectrometry (HRMS) were utilized for substance identification, leading to the discovery of 16 flavonoid compounds. Using an oral ulcer model established in Wistar rats, Ponkan extracts were found to effectively reduce ulcer area and pain sensitivity. The study revealed that four polymethoxyflavones (PMFs) were particularly effective in treating oral ulcers, while hesperidin did not show significant improvement. Mechanistic studies indicated that PMFs could inhibit the phosphorylation of Jak2 and Stat3, whereas hesperidin lacked this effect. This research supports the potential use of Ponkan and its flavonoids as a natural and effective alternative therapy for oral ulcers, with a focus on the role of PMFs in modulating the Jak2-Stat3 pathway, highlighting the possibilities for targeted natural treatments.Graphic Abstract

Keywords
Supplementary Material
Supplementary Material FileNomenclature
UPLC | Ultra-performance liquid chromatography |
HRMS | High resolution mass spectrometry |
PMFs | Polymethoxyflavones |
TNFα | Tumor necrosis factor alpha |
IL-1β | Interleukin 1 beta |
IL-6 | Interleukin 6 |
Jak2 | Janus kinase 2 |
p-Jak2 | Phosphorylated janus kinase 2 |
Stat3 | Signal transducer and activator of transcription 3 |
p-Stat3 | Phosphorylated signal transducer and activator of transcription 3 |
RAS | Recurrent aphthous stomatitis |
Oral ulcer, a common mucosal disease, brings significant pain and discomfort to patients, thus seriously affecting their quality of life [1]. Despite the availability of various treatment methods for oral ulcers, many patients still experience frequent recurrence. This highlights the importance of seeking safer and more effective treatment options [1–3]. In recent years, natural products have garnered growing interest from researchers due to their unique biological activities and minimal side effects [4].
Ponkan (Citrus reticulata ‘Ponkan’), a citrus species extensively cultivated in southern China, is rich in nutrients in both its peel and pulp, which contribute to its recognized unique medicinal value. Previous studies have indicated that the flavonoid compounds in Ponkan exhibit various biological activities such as antioxidant [5], anti-inflammatory [6], and anti-tumor effects [7], suggesting the potential role of Ponkan in the treatment of oral ulcers. However, to date, there have been no reports on the inhibitory effects and specific mechanisms of Ponkan and its extracts on oral ulcers, which serves as the starting point for this study.
In this study, we aimed to investigate the inhibitory effects of Ponkan extracts on oral ulcers through extraction and flavonoid component analysis of different cultivars of Ponkan. Advanced UPLC-HRMS technology was employed for qualitative and quantitative analysis of flavonoids in Ponkan peel, elucidating the differences in the types and contents of flavonoids among different cultivars of Ponkan. We also established a rat model of oral ulcers, we evaluated the alleviating effects of Ponkan extracts and its main flavonoid monomers on oral ulcers and explored their potential mechanisms of action. We expect this study to clarify how Ponkan extracts and flavonoids inhibit oral ulcers and to elucidate the underlying mechanisms. Furthermore, it will provide scientific evidence supporting the use of Ponkan in oral ulcer treatment and offer new directions for researching natural products in oral disease therapy.
Fruits of 20 different cultivars of Ponkan were collected in December 2023 from the Fruit Tree Research Institute of Quzhou Academy of Agriculture and Forestry Science. They were promptly transported to the Laboratory of Fruit Science Institute at Zhejiang University, within 24 h. Fruits without mechanical damage or diseases and pests were selected for washing, freeze-dried, ground, and stored for further use. Male Wistar rats were purchased from the Experimental Platform of the Laboratory Animal Center at Zhejiang University.
Vicenin 2, narirutin, hesperidin, neohesperidin, didymin, isosinensetin, sinensetin, nobiletin, tangeretin, 5-demethylnobiletin, tween 20, and capsaicin were purchased from Shanghai Yuanye Bio-Technology Co., Ltd. (Shanghai, China) Sep-pak® C18 columns were obtained from Waters Corporation. Chromatographic grade methanol and acetonitrile were sourced from Sigma-Aldrich (Shanghai,China) for use in our experiments. PrimeScript™ RT reagent Kit with gDNA Eraser was acquired from Takara (Beijing, China). SsoFast™ EvaGreen® Supermix kit was bought from BIO-RAD. ELISA kits including TNFα, IL-1β, IL-6, Jak2, p-Jak2, Stat3, p-Stat3 were purchased from Abcam (Shanghai, China). Glutathione (GSH) concentration and antioxidant enzyme (SOD and GSH-Px) activities kits were purchased from Nanjing Jiancheng Bio Co. (Jiangsu, China). Other solvents and reagents were of analytical grade, sourced from China National Pharmaceutical Group Corporation (Beijing, China). The water used in the experiments was double distilled water prepared in the laboratory.
2.2 Extraction of Pokan Fruit Flavedos
The method of Ponkan extraction was referred to our previous study [8].
2.3 Identification and Quantification of Pokan Fruit Flavedos
Qualitative and quantitative detection of Ponkan extracts were performed using UPLC-HRMS refer to the methods of Yan et al. [9], with slight modifications. The gradient elution program was: 0~5 min, 20% B; 5~8 min, 20%~36% B; 8~20 min, 36%~50% B; 20~22 min, 50%~80% B; 22~23 min, 80%~100% B; 23~25 min, 100%~20% B; 25~30 min, 20% B.
Standards of vicenin 2, narirutin, hesperidin, neohesperidin, didymin, isosinensetin, sinensetin, nobiletin, tangeretin, and 5-demethylnobiletin were used for comparison, and the chromatographic peak area standard curve method was employed for content determination. The content of the stellarin 2 quantified relative to the peak area of hesperidin, while the other 5 PMFs was were quantified relative to the peak area of nobiletin.
2.4 Establishment of Oral Ulcer Model
The method for oral ulcer induction was adapted from the study by Hitomi et al. [10]. Male Wistar rats, each weighing approximately 250 g, were utilized for the study, having been bred at Zhejiang University’s experimental animal facility (Animal experiments are subject to ethical approval, experimental code ZJU20240156). Breeding conditions were meticulously controlled, with temperatures maintained at 21°C~23°C, humidity at 55%~65%, and a consistent light-dark cycle of 12 h. The rats were divided into 22 groups, including control group, model group and treatment groups, with 5 rats in each group. Rats had free access to food and water. Following pentobarbital anesthesia administration (50 mg/kg, intraperitoneally), eight-week-old rats underwent a 30-second application of filter paper (3 mm × 3 mm) soaked in 50% acetic acid to the labial fornix area beneath the lower incisors. Obvious ulceration in the treated oral mucosal region was observed within two days following the acetic acid application. In the control group, rats received a sham treatment consisting solely of pentobarbital anesthesia, with no application of acetic acid. For intragastric administration, citrus extract dissolved in physiological saline with 1% Tween 20 was employed. Administration was carried out daily via gavage at a dose of 100 mg/kg BW. The control group received normal saline with 1% Tween 20 via gavage. Gastric administration commenced on the second day post-modeling. The ulcer’s diameter was measured with a vernier caliper on the first, third, and fifth days of gastric administration, with its area being calculated to quantify the change in ulcer size.
2.5 Evaluation of Pain Sensitivity in Rats
The method for pain sensitivity was adapted from the study by Hitomi et al. [10]. On the first day after treatment, the oral administration method was employed to record the duration of rubbing behavior in rats as a measure of pain intensity. A water-soluble capsaicin solution was diluted to 3%, and 0.05 mL of the solution was administered onto the ulcerated area of the rats using a syringe. Five minutes after the first dose, the total time of mouth rubbing behavior of rats in each group within 3 min was recorded.
The method of RT-PCR to our previous study [8]. The primer sequences were listed in Table 1. Each experiment was set up with three technical replicates and repeated at least three times, using β-Actin as an internal reference. The relative gene expression levels were calculated using the 2−ΔΔCt method.
The expression levels of inflammatory cytokines, including Tumor Necrosis Factor alpha (TNFα), Interleukin 1 beta (IL-1β), and Interleukin 6 (IL-6), as well as the proteins Janus kinase 2 (Jak2), phosphorylated Janus kinase 2 (p-Jak2), Signal transducer and activator of transcription 3 (Stat3), and phosphorylated Signal transducer and activator of transcription 3 (p-Stat3), were quantified using commercially available ELISA kits (Abcam). Each assay was conducted using protein extracts from the oral mucosa of five rats per group, following the standard curve method for analysis. The experimental conditions, such as temperature and incubation times, were stringently controlled to minimize variability and ensure the accuracy of the measurements. The absorbance was read at the specified wavelength according to the kit’s protocol, and the concentration of each cytokine was calculated based on the standard curve derived from the kit’s provided standards.
The quantification of antioxidants glutathione (GSH) and the activities of key antioxidant enzymes (SOD and GSH-Px) was performed using commercially available assay kits and a microplate reader, in accordance with the manufacturer’s instructions.
All experiments were conducted with a minimum of three replicates. Results in tables and figures are presented as mean ± standard deviation. All data were statistically evaluated using SPSS version 20.0 and visualized using OriginPro 2022. Differences were assessed using one-way analysis of variance (ANOVA). Multiple comparisons between groups were performed using Student t’s method.
3.1 Qualitative and Quantitative Analysis of Flavonoids in Ponkan
The Ponkan, the predominant wide-skinned citrus variety cultivated in China, encompasses numerous cultivars. However, little research has compared the active substance content among these cultivars. In this study, we collected 20 distinct cultivars of Ponkan (Fig. 1) and performed qualitative and quantitative analysis of flavonoids in their citrus peel using UPLC-HRMS. As shown in Fig. 2 and Table 2, a total of 16 flavonoid compounds were identified in Ponkan peel, including 6 flavanones, 1 dihydroflavone, and 9 flavones. It is worth noting that both dihydroflavones and flavones are polymethoxyflavones (PMFs) with 4 or more methoxy groups.
Figure 1: Photos of 20 cultivars of Ponkan fruit
Figure 2: UPLC spectrum of different cultivars of Ponkan fruit (280 nm)
The quantitative analysis of 16 flavonoids was conducted. As illustrated in Fig. 2 and Table S1, the flavonoid content in all citrus cultivars ranged from 33.35 to 52.68 mg/g DW. Among them, the cultivars ‘PaiXiTou No.7’, ‘QuPeng No.2’, ‘JiangXi WuHe’, and ‘XiangXi WuHe’ exhibited the highest content, while ‘HuTouGan’ and ‘YuanHong’ displayed the lowest content levels. Hesperidin, nobiletin, and tangeretin were identified as the three most abundant flavonoids with concentrations ranging from 6.35 to 16.50 mg/g DW for hesperidin, 7.78 to 13.03 mg/g DW for nobiletin, and 5.25 to 6.61 mg/g DW for tangeretin, respectively. The above results demonstrate significant variations in flavonoid content among different Ponkan cultivars.
3.2 The Alleviating Effects of Ponkan Extracts on Oral Ulcers
To assess the therapeutic effect of Ponkan extracts on oral ulcers, we induced ulceration in Wistar rat oral cavities using acetic acid. As shown in Table 3, acetic acid induced ulcers in rat oral cavities. The ulcer areas subsequently increased on the second day post-induction. Ulcer areas in all groups increased by 1.94 to 7.17 mm2 compared to the modeling period, with no significant differences among the groups on Day 1 (the day of first gavage treatment). Starting from the third day of gavage treatment, the ulcer areas in the treatment groups showed a significant reduction compared to the model group. This reduction was even more pronounced by the fifth day. Although the ulcer areas in the model group also exhibited reductions on the third and fifth days compared to both modeling and first day of treatment, with decreases of 10.16 and 12.43 mm2, respectively, the treatment groups demonstrated more substantial reductions ranging from 11.58 to 17.25 mm2 on the third day and from 15.47 to 21.77 mm2 on the fifth day when compared to their respective initial measurements. Notably, statistically significant differences were observed among all treatment groups on the third and fifth days. Among them, ‘LiPeng No.2’ showed the best effect, reducing the ulcer area from 24.83 mm2 on Day 1 to 7.58 mm2 on Day 3 and 3.06 mm2 on Day 5.
Additionally, we recorded the evoked mouth rubbing time of rats after treatment with water-soluble capsaicin as an indicator of pain sensitivity. As shown in Fig. 3A, the evoked mouth rubbing time in the control group was 14.3 ± 1.5 s/3 min, while in the model group, it reached 50.2 ± 7.2 s/3 min, indicating a significant increase in oral pain sensitivity due to modeling. The evoked mouth rubbing time in the treatment groups ranged from 15.58 to 37.54 s/3 min, significantly reducing oral pain sensitivity.
Figure 3: Effects of feeding Ponkan extracts on mouth-rubbing (pain index) (A) and oral inflammatory factors TNF-α (B), IL-1β (C) and IL-6 (D) of rats with oral ulcer model. * represents a significant difference with the model group (p < 0.05), # represents a significant difference with the control group (p < 0.05)
Given that oral ulcers are a form of oral inflammation, the accumulation of inflammatory factors represents a significant characteristic. We quantified the expression levels of oral inflammatory factors, as depicted in Fig. 3B–D. Induction of oral ulcers resulted in respective 2.41-fold, 8.45-fold, and 3.09-fold increases in TNFα, IL-1β, and IL-6 expression levels, indicating an exacerbation of oral inflammation. Gratefully, administration of Ponkan extracts via gavage significantly attenuated the accumulation of inflammatory factors associated with oral ulcers.
3.3 Regulatory Effects of Ponkan Extract on Jak2-Stat3 Pathway
To investigate the underlying mechanism by which Ponkan extract mitigates oral ulcers, the expression of three inflammatory factor genes was evaluated using q-PCR. As shown in Fig. 4A–C, modeling of oral ulcers significantly upregulated the expression of TNFα, IL-1β, and IL-6 genes. However, treatment with Ponkan extract notably suppressed the expression of these three pivotal inflammatory factors.
Figure 4: Effects of feeding Ponkan extract on inflammatory genes including TNF-α (A), IL-1β (B), IL-6 (C), Jak2-Stat3 pathway (D to I) and antioxidants evaluation (J to L) of rats with oral ulcer model. * represents a significant difference with the model group (p < 0.05), # represents a significant difference with the control group (p < 0.05)
The Jak2-Stat3 pathway plays a crucial role in inflammation, and we investigated the expression of Jak2 and Stat3 genes as well as proteins. The results depicted in Fig. 4D–I demonstrate a significant upregulation of Jak2 and Stat3 gene expression upon oral ulcer modeling, accompanied by an elevation in the levels of phosphorylated Jak2 and Stat3 proteins. Notably, treatment with Ponkan extract effectively suppressed the expression of Jak2 and Stat3 genes while also mitigating the levels of both total and phosphorylated forms of Jak2 and Stat3 proteins. These findings provide mechanistic insights into the anti-inflammatory properties of Ponkan extracts along with their potential therapeutic effects on oral ulcers. Oxidative stress commonly accompanies inflammation as a coexisting phenomenon. Henceforth, our investigation focused on assessing how oral ulcers impact glutathione (GSH) levels, as well as glutathione peroxidase (GSH-Px) and superoxide dismutase (SOD) activity within an organism (Fig. 4J–L). Our findings revealed a significant inhibition in GSH levels due to oral ulcers. Notably, administration with Ponkan extract led to markedly elevated GHS levels compared to those observed in untreated models—indicating its substantial potential for ameliorating oxidative stress resulting from oral ulcers. Furthermore, our analysis demonstrated considerably reduced activity levels for both GHS-Px and SOD within an experimental model featuring oral ulcers when contrasted with a control group. Supplementation with Ponkan extract resulted in notable increases in both enzyme activities. Therefore, we speculated that Ponkan extract could improve the content of GSH by regulating the activity of antioxidant enzymes, thereby improving the oxidative stress induced by oral ulcers.
3.4 Alleviating Effects of Ponkan Flavonoid Monomers on Oral Ulcers
The citrus flavonoids can be categorized into two primary groups: flavanones and flavones. In this study, we have specifically selected the top 5 flavonoids with the highest abundance in Ponkan, including hesperidin, sinensetin, nobiletin, tangeretin, and 5-demethylnobiletin, to assess their potential for alleviating oral ulcers. The results were presented in Table 4 and Fig. 5. On Day 3, the ulcer areas in the groups treated with the four PMFs exhibited a significant reduction compared to the model group, ranging from 7.94 to 9.67 mm2. By Day 5, further decrease in ulcer areas was observed, with tangeretin demonstrating the smallest ulcer area at 2.55 ± 0.20 mm2, which significantly surpassed the effects observed in other treatment groups. In contrast, hesperidin displayed a marginal decrease in ulcer area on both Day 3 and Day 5 without any significant difference compared to the model group.
Figure 5: Effects of feeding Ponkan flavonoid monomers on mouth-rubbing (pain index) (A) and oral inflammatory factors TNF-α (B), IL-1β (C) and IL-6 (D) of rats with oral ulcer model. * represents a significant difference with the model group (p < 0.05), # represents a significant difference with the control group (p < 0.05)
In relation to pain sensitivity (Fig. 5A), all 4 PMFs exhibited the ability to alleviate oral pain sensitivity in rats, except for hesperidin which did not demonstrate a significant impact on improving pain sensitivity. Regarding inflammatory factors (Fig. 5B–D), all 4 PMF monomers significantly reduced the levels of TNFα, IL-1β, and IL-6. However, while hesperidin decreased the level of TNFα, it did not show a significant improvement in the levels of IL-1β and IL-6.
These findings suggest that the amelioration of oral ulcers by Ponkan flavonoid extracts may be attributed to PMFs, whereas the impact of hesperidin may be limited.
3.5 Regulatory Effects of Ponkan Flavonoid Monomer on Jak2-Stat3 Pathway
The mechanistic investigation revealed a significant inhibitory effect of PMFs on the expression of TNFα, IL-1β, and IL-6 genes (Fig. 6A–C), while no significant effect was observed for hesperidin in regulating the gene expression of these three inflammatory factors. In the Jak2-Stat3 pathway (Fig. 6D–I), all 4 PMFs exhibited significant attenuation of the aberrant upregulation of Jak2 and Stat3 gene expression induced by oral ulcers, as well as downregulation of Jak2 and Stat3 protein levels and their phosphorylation states. However, hesperidin specifically reduced the gene and protein expression of Jak2 and Stat3 without exerting a significant effect on their phosphorylation levels. The findings of this study suggested that the 4 PMFs possess the ability to modulate gene and protein expression of inflammatory factors via the Jak2-Stat3 pathway, thereby mitigating symptoms associated with oral ulcers. In contrast, hesperidin’s inability to inhibit phosphorylation levels of Jak2 and Stat3 results in a comparatively weaker impact on inflammatory factors and the alleviation of oral ulcers.
Figure 6: Effects of feeding Ponkan flavonoid monomers on inflammatory genes including TNF-α (A), IL-1β (B), IL-6 (C), Jak2-Stat3 pathway (D to I) and antioxidant evaluation (J to L) of rats with oral ulcer model. * represents a significant difference with the model group (p < 0.05), # represents a significant difference with the control group (p < 0.05)
In terms of regulating oxidative stress, all four PMFs demonstrated the ability to regulate antioxidant enzyme activity and enhance GSH content. However, hesperidin exhibited weak regulation of oxidative stress, with no significant difference in GSH content and the activities of GSH-PX and SOD compared to those in the model group (Fig. 6J–L).
Citrus fruits are highly valued for both their nutritional benefits and their bioactive properties, offering applications in food and medicine. Different categories and varieties of citrus contain diverse bioactive substances with varying functional effects. Therefore, careful consideration should be given to the selection of specific varieties for application [18]. Mandarins (Citrus reticulata), an important member of the citrus family, has garnered considerable attention in terms of its medicinal applications owing to its notable bioactive effects [8]. Particularly in inflammation inhibition and oxidative stress regulation, it has demonstrated therapeutic and regulatory potential for various diseases and sub-health conditions [19]. Although researchers have compared the bioactive effects among different categories of citrus [18,19], there are limited studies on the cultivar differences within the same category or variety itself. As one of the most representative mandarin varieties cultivated worldwide, Ponkan has over 20 cultivars in China, as shown in Fig. 1. Ponkan peel serves as a crucial constituent of the traditional Chinese medicine ‘citrus peel’, necessitating a comparative analysis of active substance content and activity across different cultivars. Our study revealed that while the substances present in 20 Ponkan peel cultivars were similar, their respective contents exhibited significant variation. Based on prior research, we speculated that there exist substantial variations in flavonoid content among different Ponkan varieties, potentially attributable to differential enzyme expression within their metabolic pathways.
The production of flavonoids in plants is a well-established secondary metabolic process that is part of the propane metabolic pathway. It begins with L-phenylalanine, which is derived from the shikimate pathway and is transformed into trans-cinnamic acid by the action of phenylalanine ammonia-lyase (PAL). This trans-cinnamic acid is then converted into p-coumaroyl-CoA through a series of enzymatic reactions involving cinnamic acid 4-hydroxylase (C4H) and 4-coumarate: coenzyme A ligase (4CL) [20]. This conversion is pivotal as it links the shikimate pathway to the phenylpropane metabolic pathway and also delineates it from the lignin metabolic pathway [21].
The enzyme chalcone synthase (CHS) plays a crucial role in the subsequent steps by catalyzing the condensation of p-coumaroyl-CoA with three molecules of malonyl-CoA, resulting in the formation of naringenin chalcone, a key intermediate in flavonoid biosynthesis [21]. This chalcone is then converted to the flavanone naringenin by the enzyme chalcone isomerase (CHI). Flavanones serve as essential precursors for the production of various types of flavonoids, including flavones, dihydroflavonols, flavonols, and isoflavones [22], which are synthesized through the catalytic actions of different enzymes such as flavone synthase (FNS), flavanone 3-hydroxylase (F3H), flavonol synthase (FLS), and isoflavone synthase (IFS) [12].
The conversion of naringenin to apigenin is facilitated by the activity of flavonoid hydroxylase (FH), and further modifications occur at various positions on the flavone nucleus through the influence of enzymes like O-methyltransferases (OMTs), glycosyltransferases (GTs), acyltransferases (ATs), and prenyltransferases (PTs). These modifications lead to the formation of a diverse array of PMFs (prenylated flavonoids) and flavone glycosides, enriching the variety and functionality of flavonoids in plants [12,20,23].
Oral ulcers, also known as recurrent aphthous stomatitis (RAS), are a prevalent oral mucosal disease characterized by the presence of one or more small, painful ulcers. These ulcers typically exhibit a round or oval shape with a white or yellow center and red periphery, often accompanied by tactile sensitivity. They can manifest in various locations within the oral cavity, including the lips, tongue, gums, and buccal mucosa. The exact cause of oral ulcers is not fully understood, but it is thought to involve a range of factors. These include immune system dysregulation that leads to inflammation. Management strategies for oral ulcers primarily focus on symptom relief and facilitation of wound healing. While most cases resolve spontaneously within one to two weeks, researchers have been exploring natural products and other interventions to expedite the healing process [1,3]. However, previous evaluation methods of oral ulcers have primarily focused on cell models, with limited emphasis on animal models. In our study, we proposed three comprehensive methods for evaluating oral ulcers, encompassing ulcer area measurement, pain sensitivity assessment, and inflammatory factor content. Notably, these approaches are also supported by the findings reported by previous reports [10,24].
Previous reports have indicated the therapeutic and palliative effects of citrus on digestive tract ulcers, with a predominant focus on gastric ulcers [25,26]. Nonetheless, very limited attention has been given to oral ulcer studies [27], particularly in terms of evaluating cell migration ability which presents significant differences between cell models and animal models. In our study, we employed Ponkan and flavonoid monomers to investigate the therapeutic efficacy of oral ulcer amelioration in rats. Our findings show that both Ponkan extracts and flavonoid monomers have substantial efficacy in improving oral ulcers. They reduced the ulcer area, alleviated pain sensitivity as shown by decreased mouth rubbing time, and mitigated inflammation by reducing the accumulation of inflammatory factors.
The Jak2-Stat3 pathway is a pivotal cellular signaling pathway that plays a crucial role in various biological processes. Jak2, a non-receptor tyrosine kinase, works in conjunction with its receptor to stimulate the production of cytokines and growth factors. These signal molecules subsequently activate downstream signaling mechanisms, including Stat3, which acts as a transcription factor responsive to diverse cytokines such as interferon and interleukin-6 (IL-6). Upon binding of extracellular signals to the target cell receptor, Jak2 is initially activated and phosphorylates Stat3 within the cytoplasm. Subsequently, two phosphorylated Stat3 molecules dimerize and translocate into the nucleus where they bind to the regulatory region of corresponding target genes, thereby initiating downstream gene expression. Particularly in ulcerative inflammation, the Jak2-Stat3 signaling pathway assumes an indispensable role by serving as a critical conduit for signal transduction mediated by multiple cytokines and inflammatory mediators closely associated with inflammatory responses [28,29]. However, the role of Jak2-Stat3 pathway in oral ulcers, especially the regulation of flavonoids on oral ulcer-induced Jak2-Stat3 pathway, has not been reported. Our study found that Ponkan extracts and flavonoid monomers could inhibit the gene and protein expression of Jak2 and Stat3. While 4 PMFs monomers including sinensetin, nobiletin, tangeretin and 5-demethylnobiletin could inhibit the phosphorylation of Jak2 and Stat3, thereby inhibiting the gene and protein expression of downstream inflammatory factors. However, hesperidin had no significant effect on the phosphorylation of these two key proteins, corresponding to its limited formation of ameliorative effects on oral ulcers.
Oxidative stress and inflammation are mutually reinforcing complex biological processes, and their interaction constitutes a dynamic cycle. Oxidative stress triggers the inflammatory response by activating the inflammatory signaling pathway, damaging cell membranes and DNA, and altering cytokine production [30]. Additionally, activated immune cells release large amounts of reactive oxygen species (ROS) during the inflammatory process, further exacerbating oxidative stress [31]. Inflammatory mediators such as cytokines and chemokines not only promote the attraction and activation of inflammatory cells but also increase the expression of oxidases, leading to excessive ROS production [32]. Furthermore, inflammation can inhibit the activity of antioxidant systems, reduce the expression of antioxidant enzymes, decrease glutathione (GSH) levels, thereby weakening cellular antioxidant capacity [33]. The positive feedback loop between oxidative stress and inflammation results in their sustained escalation. Tissue damage caused by inflammation releases damage-associated molecular patterns (DAMPs), which can activate signaling pathways for both inflammation and oxidative stress [34]; meanwhile, ROS generated during oxidative stress-induced ferroptosis can further promote inflammation [9]. Mitochondrial dysfunction and Fe2+ release are also important factors that exacerbate oxidative stress during inflammation [35]. This interplay leads to a mutual intensification of both inflammation and oxidative stress which play critical roles in the pathogenesis of many diseases including autoimmune diseases [36], metabolic disorders [37], cardiovascular diseases [38], and neurodegenerative diseases [39].
In the study of citrus, the citrus extract of Exocarpium Citri Grandis (ECG) has shown significant protective effects in the treatment of acute lung injury (ALI). It enhanced the antioxidant capacity by inhibiting the inflammation-associated Jak2-Stat3 signaling pathway, reduced the levels of inflammatory factors such as IL1β, IL6, and TNF-α, and increased the levels of antioxidant enzymes like superoxide dismutase (SOD) and glutathione (GSH) [40]. Furthermore, ECG suppressed ferroptosis by activating the Nrf2-GPX4 signaling axis, thereby further alleviating damage to lung tissue. Our research represents the first study to validate that Ponkan extract, particularly its PMFs, could mitigate oral inflammation resulting from ulcers via the Jak2-Stat3 pathway. Additionally, Ponkan extracts and PMFs could improve oxidative stress conditions, enhanc GSH-Px and SOD activity, and augment GSH content.
Our study introduces a pioneering approach to oral ulcer treatment through the exploration of Ponkan (Citrus reticulata ‘Ponkan’) flavonoids as a natural therapeutic agent. The innovative aspects of our research are multifaceted, beginning with the comprehensive analysis of 16 flavonoids across 20 distinct Ponkan cultivars, a depth of investigation previously uncharted in the field. The identification of polymethoxyflavones (PMFs) as key bioactive compounds with potential to modulate the Jak2-Stat3 signaling pathway represents a significant breakthrough, offering a molecular target for natural anti-inflammatory interventions. Furthermore, our comparative cultivar study provides novel insights into the variability of flavonoid content within a single citrus variety, which could guide future breeding programs for enhanced medicinal properties. The unique focus on PMFs’ therapeutic efficacy, as opposed to hesperidin, presents a new direction for oral health product development, underpinned by robust scientific findings.
Despite the novel contributions, our study acknowledges several limitations that warrant consideration. The scope of our investigation was confined to 20 Ponkan cultivars, which, while extensive, may not encompass the full genetic diversity of Citrus reticulata. The utilization of a rat model, while valuable for preliminary efficacy assessment, may not fully encapsulate the human oral ulcer condition, suggesting a need for clinical trials to validate these findings. Additionally, the specific mechanisms by which flavonoids interact with cellular pathways, beyond the Jak2-Stat3 axis, require further elucidation. Lastly, while PMFs showed promise, the study did not explore the synergistic or antagonistic effects of flavonoid combinations, which could be significant in a complex biological environment. These limitations provide a clear roadmap for future research to expand upon our findings and to refine the therapeutic potential of Ponkan flavonoids for oral ulcer treatment.
This study successfully demonstrated the inhibitory effects of Ponkan flavonoids on oral ulcers, providing a promising natural treatment option. Through comprehensive analysis using UPLC and HRMS, we identified 16 flavonoids in 20 different Ponkan cultivars. The therapeutic potential of these extracts was evaluated in a rat model of oral ulcers, revealing a significant reduction in ulcer area and alleviation of pain sensitivity. Mechanistic insights uncovered that the beneficial effects are mediated through the Jak2-Stat3 signaling pathway by reducing inflammatory factors TNFα, IL-1β, and IL-6. Individual flavonoid monomers were also investigated, with 4 PMFs effectively treating oral ulcers while hesperidin did not show significant improvement due to differences in their structure. The ability of PMFs to inhibit the phosphorylation of Jak2 and Stat3 was identified as the underlying mechanism for their anti-inflammatory properties and therapeutic applications. In contrast, hesperidin reduced expression but could not inhibit phosphorylation resulting in a less pronounced effect on oral ulcers. In conclusion, this research provides evidence for using Ponkan and its flavonoids in treating oral ulcers while shedding light on their mechanisms.
Acknowledgement: None.
Funding Statement: This work was supported by the Zhejiang Province Natural Science Foundation (LTGN24C020002), the National Natural Science Foundation of China (32101932, 32072132), the Zhejiang Province Natural Science Foundation Joint Fund Project (LHZSD24C150001), the National Key R&D Program of China (2023YFD2300604), the Fundamental Research Funds for the Central Universities (226-2022-00215), Quzhou Characteristic Citrus Fruit Quality Detection Project (2023-KYY-516103-0008).
Author Contributions: The authors confirm contribution to the paper as follows: Lili Liu: Resources, Writing—original draft; Can Hu: Methodology; Huilin Cheng: Resources; Chuangju Ma: Resources; Jiancheng Sun: Resources; Yunyi Chen: Visualization; Manxi Wu: Visualization; Han Yang: Visualization; Cui Sun: Project administration; Jinping Cao: Data curation; Dengliang Wang: Conceptualization, Supervision, Writing—review & editing; Yue Wang: Conceptualization, Supervision, Writing—review & editing; Chongde Sun: Visualization, Supervision. All authors reviewed the results and approved the final version of the manuscript.
Availability of Data and Materials: All data generated or analyzed during this study are included in this published article (and its supplementary information files).
Ethics Approval: The animal study protocol was approved by the Animal Experimentation Committee at Zhejiang University (protocol code ZJU20240156).
Conflicts of Interest: The authors declare that they have no conflicts of interest to report regarding the present study.
Supplementary Materials: The supplementary material is available online at https://doi.org/10.32604/phyton.2024.054449.
References
1. Stoopler ET, Sollecito TP. Recurrent oral ulcers. JAMA. 2015;313(23):2373–4. doi:10.1001/jama.2015.1637. [Google Scholar] [PubMed] [CrossRef]
2. Lyu X, Guan XB. Gingival ulceration. New Engl J Med. 2021;385(11):E33. [Google Scholar] [PubMed]
3. Yin W, Yang YM, Chen H, Li X, Wang Z, Cheng L, et al. Oral health status in Sichuan province: findings from the oral health survey of Sichuan, 2015–2016. Int J Oral Sci. 2017;9(1):10–5. doi:10.1038/ijos.2017.6. [Google Scholar] [PubMed] [CrossRef]
4. Ferreira AS, Macedo C, Silva AM, Delerue-Matos C, Costa P, Rodrigues F. Natural products for the prevention and treatment of oral mucositis—a review. Int J Mol Sci. 2022;23(8):4385–416. doi:10.3390/ijms23084385. [Google Scholar] [PubMed] [CrossRef]
5. Huang R, Zhang Y, Shen SY, Zhi ZJ, Cheng H, Chen SG, et al. Antioxidant and pancreatic lipase inhibitory effects of flavonoids from different citrus peel extracts: an study. Food Chem. 2020;326:12675. [Google Scholar]
6. Chang YH, Seo J, Song E, Choi HJ, Shim E, Lee O, et al. Bioconverted Jeju Hallabong tangor (Citrus kiyomi × ponkan) peel extracts by cytolase enhance antioxidant and anti-inflammatory capacity in RAW 264.7 cells. Nutr Res Pract. 2016;10(2):131–8. doi:10.4162/nrp.2016.10.2.131. [Google Scholar] [PubMed] [CrossRef]
7. Du QZ, Chen H. The methoxyflavones in Citrus reticulata Blanco cv. ponkan and their antiproliferative activity against cancer cells. Food Chem. 2010;119(2):567–72. doi:10.1016/j.foodchem.2009.06.059. [Google Scholar] [CrossRef]
8. Wang Y, Jin R, Chen JB, Cao JP, Xiao JB, Li X, et al. Tangeretin maintains antioxidant activity by reducing CUL3 mediated NRF2 ubiquitination. Food Chem. 2021;365:130470. doi:10.1016/j.foodchem.2021.130470. [Google Scholar] [PubMed] [CrossRef]
9. Yan QJ, Chen YY, Wu MX, Yang H, Cao JP, Sun CD, et al. Phenolics and terpenoids profiling in diverse loquat fruit varieties and systematic assessment of their mitigation of alcohol-induced oxidative stress. Antioxidants. 2023;12(10):1795–821. doi:10.3390/antiox12101795. [Google Scholar] [PubMed] [CrossRef]
10. Hitomi S, Ono K, Yamaguchi K, Terawaki K, Imai R, Kubota K, et al. The traditional Japanese medicine hangeshashinto alleviates oral ulcer-induced pain in a rat model. Arch Oral Biol. 2016;66:30–7. doi:10.1016/j.archoralbio.2016.02.002. [Google Scholar] [PubMed] [CrossRef]
11. Gao YH, Ma K, Zhu ZB, Zhang Y, Zhou Q, Wang J, et al. Modified Erchen decoction ameliorates cognitive dysfunction in vascular dementia rats inhibiting JAK2/STAT3 and JNK/BAX signaling pathways. Phytomedicine. 2023;114:154797. doi:10.1016/j.phymed.2023.154797. [Google Scholar] [PubMed] [CrossRef]
12. Zhu CQ, Chen JB, Zhao CN, Liu XJ, Chen YY, Liang JJ, et al. Advances in extraction and purification of citrus flavonoids. Food Front. 2023;4(2):750–81. doi:10.1002/fft2.v4.2. [Google Scholar] [CrossRef]
13. Zhang J, Wu XF, Qu JQ, Zhang L, Zhang YT, Qu XH, et al. Comprehensive comparison on the chemical profile of Guang Chen Pi at different ripeness stages using untargeted and pseudotargeted metabolomics. J Agr Food Chem. 2020;68(31):8483–95. doi:10.1021/acs.jafc.0c02904. [Google Scholar] [PubMed] [CrossRef]
14. Cao YH, Chen X, Pan FQ, Wang MY, Zhuang HW, Chen JN, et al. Xinmaikang-mediated mitophagy attenuates atherosclerosis via the PINK1/Parkin signaling pathway. Phytomedicine. 2023;119:154955. doi:10.1016/j.phymed.2023.154955. [Google Scholar] [PubMed] [CrossRef]
15. Iwashita M, Shioi R, Sugiyama M, Hashizume K, Kan T, Naito S, et al. Monodemethylated metabolites of orally administered nobiletin: identification and quantitation in rat plasma and tissues. J Agr Food Chem. 2023;71(26):10028–36. doi:10.1021/acs.jafc.3c00096. [Google Scholar] [PubMed] [CrossRef]
16. Santos FA, Viana AFSC, Nunes PIG, Portela BYM, Alves APNN, Viana DD, et al. UPLC-QTOF-MS/MS-based metabolomic approach and gastroprotective effect of two chemotypes of Egletes viscosa (L.) less. against ethanol-induced gastric ulcer in mice. J Ethnopharmacol. 2023;309:116348. doi:10.1016/j.jep.2023.116348. [Google Scholar] [PubMed] [CrossRef]
17. de Queiroz LN, Da Fonseca ACC, Wermelinger GF, da Silva DPD, Pascoal ACRF, Sawaya ACHF, et al. New substances of Equisetum hyemale L. extracts and their in vivo antitumoral effect against oral squamous cell carcinoma. J Ethnopharmacol. 2023;303:116043. doi:10.1016/j.jep.2022.116043. [Google Scholar] [PubMed] [CrossRef]
18. Li LJ, Yan X, Chen FY, Zheng LY, Hu Y, He F, et al. A comprehensive review of the metabolism of citrus flavonoids and their binding to bitter taste receptors. Compr Rev Food Sci F. 2023;22(3):1763–93. doi:10.1111/crf3.v22.3. [Google Scholar] [CrossRef]
19. Deng YD, Tu YL, Lao SH, Wu MT, Yin HT, Wang LQ, et al. The role and mechanism of citrus flavonoids in cardiovascular diseases prevention and treatment. Crit Rev Food Sci. 2022;62(27):7591–614. doi:10.1080/10408398.2021.1915745. [Google Scholar] [PubMed] [CrossRef]
20. Zhao CN, Liu XJ, Gong Q, Cao JP, Shen WX, Yin XR, et al. Three AP2/ERF family members modulate flavonoid synthesis by regulating type IV chalcone isomerase in citrus. Plant Biotechnol J. 2021;19(4):671–88. doi:10.1111/pbi.v19.4. [Google Scholar] [CrossRef]
21. Tohge T, Watanabe M, Hoefgen R, Fernie AR. The evolution of phenylpropanoid metabolism in the green lineage. Crit Rev Biochem Mol. 2013;48(2):123–52. doi:10.3109/10409238.2012.758083. [Google Scholar] [PubMed] [CrossRef]
22. Austin MB, Noel JP. The chalcone synthase superfamily of type III polyketide synthases. Nat Prod Rep. 2003;20(1):79–110. doi:10.1039/b100917f. [Google Scholar] [PubMed] [CrossRef]
23. Fu MQ, Xu YJ, Chen YL, Wu JJ, Yu YS, Zou B, et al. Evaluation of bioactive flavonoids and antioxidant activity in Pericarpium Citri Reticulatae (Citrus reticulata ‘Chachi’) during storage. Food Chem. 2017;9(1):649–56. [Google Scholar]
24. Xu J, Zhang Y, Fang XH, Liu Y, Huang YB, Ke ZL, et al. The oral bacterial microbiota facilitates the stratification for ulcerative colitis patients with oral ulcers. Ann Clin Microb Anti. 2023;22(1):99. doi:10.1186/s12941-023-00646-3. [Google Scholar] [PubMed] [CrossRef]
25. Naser AA, Younis E, El-Feky A, Elbatanony M, Hamed M. Management of Citrus sinensis peels for protection and treatment against gastric ulcer induced by ethanol in rats. Biomarkers. 2020;25(4):349–59. doi:10.1080/1354750X.2020.1759693. [Google Scholar] [PubMed] [CrossRef]
26. Selmi S, Rtibi K, Grami D, Sebai H, Marzouki L. Protective effects of orange (Citurs sinensis L.) peel aqueous extract and hesperidin on oxidative stress and peptic ulcer induced by alcohol in rat. Lipids Health Dis. 2017;16:1–12. [Google Scholar]
27. Tsai HC, Li YC, Young TH, Chen MH. Citrus polyphenol for oral wound healing in oral ulcers and periodontal diseases. J Formos Med Assoc. 2016;115(2):100–7. doi:10.1016/j.jfma.2015.01.003. [Google Scholar] [PubMed] [CrossRef]
28. Núñez P, Quera R, Yarur AJ. Safety of janus kinase inhibitors in inflammatory bowel diseases. Drugs. 2023;83(4):299–314. doi:10.1007/s40265-023-01840-5. [Google Scholar] [PubMed] [CrossRef]
29. Liu CS, Xia T, Luo ZY, Wu YY, Hu YN, Chen FL, et al. Network pharmacology and pharmacokinetics integrated strategy to investigate the pharmacological mechanism of Xianglian pill on ulcerative colitis. Phytomedicine. 2021;82:153458. doi:10.1016/j.phymed.2020.153458. [Google Scholar] [PubMed] [CrossRef]
30. Schieber M, Chandel NS. ROS function in redox signaling and oxidative stress. Curr Biol. 2014;24(10):R453–62. doi:10.1016/j.cub.2014.03.034. [Google Scholar] [PubMed] [CrossRef]
31. Nathan C, Cunningham-Bussel A. Beyond oxidative stress: an immunologist’s guide to reactive oxygen species. Nat Rev Immunol. 2013;13(5):349–61. doi:10.1038/nri3423. [Google Scholar] [PubMed] [CrossRef]
32. Mittal M, Siddiqui MR, Tran K, Reddy SP, Malik AB. Reactive oxygen species in inflammation and tissue injury. Antioxid Redox Sign. 2014;20(7):1126–67. doi:10.1089/ars.2012.5149. [Google Scholar] [PubMed] [CrossRef]
33. Rahman I, MacNee W. Oxidative stress and regulation of glutathione in lung inflammation. Eur Respir J. 2000;16(3):534–54. doi:10.1034/j.1399-3003.2000.016003534.x. [Google Scholar] [PubMed] [CrossRef]
34. Dela Cruz CS, Kang MJ. Mitochondrial dysfunction and damage associated molecular patterns (DAMPs) in chronic inflammatory diseases. Mitochondrion. 2018;41(7):37–44. doi:10.1016/j.mito.2017.12.001. [Google Scholar] [PubMed] [CrossRef]
35. Sun L, Wang H, Xu D, Yu SS, Zhang L, Li XP. Lapatinib induces mitochondrial dysfunction to enhance oxidative stress and ferroptosis in doxorubicin-induced cardiomyocytes via inhibition of PI3K/AKT signaling pathway. Bioengineered. 2022;13(1):48–60. doi:10.1080/21655979.2021.2004980. [Google Scholar] [PubMed] [CrossRef]
36. Smallwood MJ, Nissim A, Knight AR, Whiteman M, Haigh R, Winyard PG. Oxidative stress in autoimmune rheumatic diseases. Free Radical Bio Med. 2018;125:3–14. doi:10.1016/j.freeradbiomed.2018.05.086. [Google Scholar] [PubMed] [CrossRef]
37. Rani V, Deep G, Singh RK, Palle K, Yadav UCS. Oxidative stress and metabolic disorders: pathogenesis and therapeutic strategies. Life Sci. 2016;148:183–93. doi:10.1016/j.lfs.2016.02.002. [Google Scholar] [PubMed] [CrossRef]
38. Dubois-Deruy E, Peugnet V, Turkieh A, Pinet F. Oxidative stress in cardiovascular diseases. Antioxidants. 2020;9(9):864. doi:10.3390/antiox9090864. [Google Scholar] [PubMed] [CrossRef]
39. Emerit J, Edeas A, Bricaire F. Neurodegenerative diseases and oxidative stress. Biomed Pharmacother. 2004;58(1):39–46. doi:10.1016/j.biopha.2003.11.004. [Google Scholar] [PubMed] [CrossRef]
40. Xu ZB, Li JY, Zhou KL, Wang KY, Hu HY, Hu YJ, et al. Exocarpium Citri Grandis ameliorates LPS-induced acute lung injury by suppressing inflammation, NLRP3 inflammasome, and ferroptosis. J Ethnopharmacol. 2024;329:118162. doi:10.1016/j.jep.2024.118162. [Google Scholar] [PubMed] [CrossRef]
Cite This Article
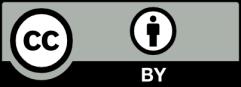
This work is licensed under a Creative Commons Attribution 4.0 International License , which permits unrestricted use, distribution, and reproduction in any medium, provided the original work is properly cited.