Open Access
ARTICLE
Grasslands Response to Livestock Grazing Intensity in the Austral Pampas (Argentina): Testing the Intermediate Disturbance Hypothesis
1 Grupo de Investigación en Ecosistemas de Pastizal (GIEP), CERZOS, CONICET, Bahía Blanca, 8000, Argentina
2 Departamento de Biología, Bioquímica y Farmacia, Universidad Nacional del Sur, Bahía Blanca, 8000, Argentina
* Corresponding Author: Ana Elena de Villalobos. Email:
(This article belongs to the Special Issue: Ecology of Rangelands in Argentina)
Phyton-International Journal of Experimental Botany 2024, 93(8), 2037-2050. https://doi.org/10.32604/phyton.2024.053928
Received 14 May 2024; Accepted 19 July 2024; Issue published 30 August 2024
Abstract
Livestock grazing has a significant impact on natural grasslands, with approximately one-third of the world’s land area dedicated to this industry. Around 20% of global grasslands are highly degraded due to overgrazing, affecting their productivity and conservation capacity. Best practices are required to ensure sustainable livestock production that supports biodiversity. The Intermediate Disturbance Hypothesis (IDH) suggests that environments with moderate levels of disturbance exhibit a higher species diversity. Moderate grazing can reduce the dominance of certain species, thereby enhancing plant diversity. However, concerns arise regarding the increase of exotic and unpalatable species under moderate grazing levels, complicating grassland conservation efforts. The impact of livestock grazing on the functional structure of grasslands depends on factors such as grazing intensity, livestock species, and environmental conditions. Variations in grazing intensity may increase specific and functional diversity under moderate grazing, potentially masking the presence of invasive exotic species. In the Austral Pampas (Pampean phytogeographic province, Buenos Aires, Argentina), grasslands face various pressures from domestic livestock grazing that endanger their integrity if not properly managed. Therefore, our study aims to investigate potential differences in species richness and diversity, functional diversity, exotic plant abundance, and the number and distribution of plant functional groups across varying grazing intensities. The IDH is utilized as a tool to regulate livestock pressure for grassland conservation. Species and functional diversity indices were used to assess the impact of grazing on grassland diversity. Moderate grazing increased species and functional diversity, while intensively grazed or ungrazed areas showed reduced diversity. Livestock presence influenced the balance between native and exotic plants, with ungrazed areas having higher native plant abundance and grazed areas exhibiting higher exotic plant abundance. Grazing also influenced the composition of functional groups, with grazing-avoiding species being more prevalent in heavily grazed areas. Principal Component Analysis revealed a clear association between vegetation composition and livestock grazing intensity. These findings offer valuable insights into effectively managing grazing intensity for biodiversity conservation purposes.Keywords
Supplementary Material
Supplementary Material FileLivestock farming, as the predominant global land use activity, has a significant impact on natural grasslands, with approximately one-third of the world’s land dedicated to this industry [1]. According to [2], about 20% of the world’s grasslands are highly degraded, with overgrazing generally being the most significant factor in this degradation [3–6]. Therefore, it is necessary to recognize best practices to enhance livestock farming efficiency and develop a sustainable livestock production system that supports biodiversity. Maintaining stability in this system is a significant challenge that requires careful consideration and strategic planning [2,5].
Connecting ecological theories of species diversity to land use has emerged as a research line and management priority, focused on enhancing species diversity and promoting the sustainable utilization of natural systems [7]. The Intermediate Disturbance Hypothesis (IDH) is one of the key theories used in ecosystem diversity studies. This theory predicts that the highest species diversity should be observed in environments with moderate disturbance levels [8,9]. The extensive management of grasslands, particularly through grazing practices, is in line with this established ecological concept [10]. Under this theoretical framework, it is assumed that there is potential to generate sustainable livestock production that supports a diverse array of plant species through adequate management of the pastoral systems [11,12]. The explanation is grounded in the idea that disturbances such as livestock grazing can decrease the relative abundance of dominant species, favoring those plants capable of either avoiding or tolerating grazing. These plants are typically found in lightly grazed or ungrazed systems, but in low abundance [4,11]. Reduced grazing levels may result in low diversity due to competitive exclusion and the dominance of long-lived species, such as perennial tussock grasses, while excessive grazing can eliminate palatable species that are unable to recolonize and grow quickly [4,13]. Therefore, livestock grazing acts as a selective disturbance that reduces interspecific competition [14] and enhances species traits associated with strategies for resisting herbivory, with the extent of enhancement depending on grazing intensity [15–17]. However, there is a concern that moderate disturbance intensities may lead to an increase in plant diversity values due to the higher abundance of exotic and invasive plants [17]. Consequently, disturbances may simultaneously facilitate invasions by weedy plant species [18] and plants that exhibit avoiding-grazing or tolerant-grazing attributes [16]. It becomes a challenge to achieve a balance between preserving species diversity and rangeland management if higher species diversity at intermediate disturbance levels is maintained by the coexistence of grassy perennial species with weedy annual species, usually exotic plants, that often have little forage value [19].
It is important to clarify that the IDH does not always predict the response of grassland plant diversity to livestock grazing accurately. Many authors discuss these controversial results [20,21]. Other factors may explain these anomalies, such as environmental conditions, plant responses to grazing intensity along climatic gradients, and the evolutionary history of grazing [22]. Another aspect that is not always contemplated in the IDH is the effect of livestock grazing on the functional structure of grassland ecosystems. This impact depends on factors such as grazing intensity, livestock type, and environmental conditions [4,23]. The evolutionary history of large herbivore grazing also influences ecosystem properties and processes [22,24]. Grazing by domestic livestock at different intensities selectively alters grassland functioning, thereby influencing its structure. These impacts are assessed by functional diversity and redundancy indices [25]. These indices have the potential to unveil the processes that shape plant communities through their response to different disturbances, such as varying intensities of livestock grazing [26]. Functional diversity encompasses the quantity, type, and distribution of functions carried out by plants within a natural system. It can be expressed as the proportion of functional groups present in relation to the total abundance of all functional groups recorded in the system [27]. On the other hand, functional redundancy is determined by the ratio between species richness and the number of functions within an assemblage unit, such as a plant community [28]. High values of these indices in both cases indicate increased stability and resilience of the systems against disturbances [29]. Therefore, in order to assess the impact of domestic livestock grazing intensity on natural grassland diversity, it is essential to evaluate both species and functional diversity.
The undesirable effects of domestic livestock grazing intensity variations on natural grasslands may be masked by increased levels of specific and functional diversity under moderate grazing intensities, as predicted by the IDH [30]. This could obscure the presence of invasive exotic plants or species with low forage quality. Thus, a comprehensive assessment of the structural and functional attributes of natural grasslands in relation to livestock grazing intensity should include quantifying the abundance of exotic species and categorizing species into functional groups based on attributes that respond to grazing impacts [31].
The Pampean phytogeographic province comprises the main temperate grassland ecosystems in South America [32]. It is distributed mainly in Buenos Aires, Argentina. This area is renowned for its exceptional fertility as one of Central Argentina’s most productive natural grasslands. Livestock farming is a key economic activity, especially in the southeastern part of this province (Austral district), leading to a long-standing tradition of continuous grazing that dates back to the 19th century. The history of grazing in this area is characterized by significant levels of intensity [33]. Studies have shown that sustained livestock presence can induce alterations in both the specific composition and functional structure of the natural grasslands in the Austral Pampas [34,35]. To preserve the productivity of these grasslands, it is crucial to gain a comprehensive understanding of the impacts of livestock grazing on ecosystems, as well as its effects on various response variables, especially when considering moderate grazing intensity as a conservation management strategy [36]. Therefore, it is valuable research work for the investigation of potential differences in species richness and diversity, functional diversity, exotic plant abundance, and the number and distribution of plant functional groups across varying grazing intensities. Particularly within the context of utilizing IDH as a tool to regulate livestock pressure for the conservation of natural grasslands. Therefore, in order to elucidate these aspects and their relationship with grazing intensity, we propose the following study hypotheses: (1) Specific and functional diversity is higher in grasslands subject to moderate grazing intensity compared to intensively grazed or ungrazed areas with domestic livestock; (2) Exotic species abundance is lower in pastures with moderate grazing compared to intensively grazed grasslands; and (3) Functional groups linked to grazing avoidance and tolerance strategies are more prevalent in intensively grazed grasslands than in those grazed moderately or without domestic livestock.
This study was carried out in the natural grasslands of the foothills of Ventania mountains (Southwest of Buenos Aires, Argentina). Ventania consists of a series of parallel low peaks ranging from approximately 300 to 1200 meters above sea level. This mountain chain extends over 150 km long and 40 km wide. The area is considered a sky island in the southwest of Buenos Aires province, characterized by natural grassland adapted to rocky soils surrounded by plains [37]. Ventania is part of the Austral District or Austral Pampas (Pampean biogeographic province). The climate is temperate and oceanic. Annual mean temperature is 14°C and precipitation varies between 600 and 800 mm, from southwest to northeast, most of it occurring during spring and autumn, and occasional snowfalls in winter [38,39]. The area has a high percentage of endemic and native species. The predominant vegetation are perennial tussock grasses, particularly species of Nasella E.Desv. and Piptochaetium J.Presl., and the dominant woody species are shrubs, such as Discaria americana Gillies & Hook. and Acanthostyles buniifolius (Hook. & Arn.) R.M. King & H. Rob [40,41]. The native herbivores, including the Pampas Deer (Ozotoceros bezoarticus L.) and Guanaco (Lama guanicoe Müller), which have historically inhabited the Pampean grasslands since prehistoric times, are currently found in low numbers or have become extinct [29]. In contrast, domestic livestock, primarily cattle (Bos Taurus L.) and horses (Equus caballus L.), became abundant in the 19th century and are now the principal herbivores in the area [42].
2.2 Experimental Design and Sampling Methods
We selected the following three sampling areas: Ernesto Tornquist Provincial Park (ETPP) (38°03′00″S 62°02′00″W), created in 1942, covering approximately 6700 hectares, primarily grazed by feral horses and cows; Sierras Grandes Protected Natural Area (SGAP) (38°10′14″S 61°54′06″W), created in 2013, with 2300 hectares, grazed by domestic livestock (cows and domestic horses); and finally, the lower part of Ceferino Hill (CHPG) with approx. 650 ha (38°07′36″S 61°47′20″W), devoid of domestic livestock presence over the last 20 years. These three areas encompass sections of the western mountain chain within Ventania. ETPP is separated 25 km from SGAP and Ceferino Hill. The latter two are 5 km apart. The sampling areas did not differ in their topographic, edaphic, or climatic aspects [38]. According to local records, they also did not show differences in annual rainfall and mean temperature records during the sampling period (Table S1).
Within the areas mentioned above, we established six site locations of 4 hectares each as replicates of each sampling site: two grasslands intensively grazed by unmanaged livestock (feral horses and cattle; 0.4 livestock units per hectare (UG)) located in ETPP, two grasslands moderately grazed by managed domestic livestock in SGAP (0.2 livestock units per hectare (UG)), and two grasslands excluded from domestic livestock grazing in CHPG, both as a control treatment. The site locations were not situated close to waterholes or other sources of water where cattle visits were more frequent, in order to avoid bias in grazing intensity between site replicas and grazing intensity treatments. In each site, we randomly distributed 20 plots (1 m2) from early spring to fall 2018. The plots were separated from each other by about 10 m to ensure that the experimental plots are independent and interspersed across each of the experimental areas [43]. The vegetation present in each experimental plot was sampled during spring and summer. A cover for each identified species was recorded using the Braun-Blanquet scale, and the ordinal classes obtained were transformed into average percentage covers for each class. Species with less than 5% cover were excluded from the analyses [44]. Species taxonomy and nomenclature were standardized according to [45]. Simultaneously, the percentage of bare soil (without standing vegetation) was recorded in each plot. The percentages of native and exotic plants, as well as the native-to-exotic ratio, were also calculated for each of the plots.
We classified all identified species into five functional groups, following the criteria of [46], based on their response to grazing: 1. Avoidance: characterized by the presence of thorns, toxic secondary compounds, and unpalatable traits (such as silica deposition in leaves, high C/N ratio, prostrate or rosette growth). 2. Tolerants: exhibiting regrowth strategies after grazing, presence of stolons or underground buds, with rhizomes, and active central tussocks in the case of bunchgrass species. 3. Sensitives: unable to tolerate or avoid grazing, highly palatable and frequently consumed, with a low C/N ratio. 4. Favored: directly or indirectly benefiting from grazing, seed dispersal mechanisms (endo-and exozoochory), dormant seeds that are scarified by the digestive tract of grazers, or benefiting from reduced competition from palatable species. 5. Neutral: apparently not exhibiting a clear strategy that is favored or disfavored by grazing.
The necessary information for each of the recorded species was obtained through bibliographic research and web surveys. The compositional structure of each site was characterized by means of species richness, Shannon’s diversity index [44], and the uniformity index [47]. The impact of grazing on grassland diversity was assessed using species and functional diversity indices [48]. Functional diversity was determined by the total number of species within each functional group, while functional redundancy was determined by the similarity of functions among species in the plant community. These indices were calculated using the F-Diversity software [49].
Differences in species richness, diversity (Shannon index), and uniformity (Pielou index), as well as functional diversity and redundancy, were analyzed using a two-way analysis of variance with a completely randomized design. The factors considered in the analysis were grazing intensity (intense grazing, moderate grazing, and exclusion from domestic livestock) and the location of sampling sites (two locations for each grazing intensity treatment). Following the analysis of variance, Tukey tests were employed to identify significantly different means [50]. The same analysis was conducted to distinguish between the percentage of bare soil, exotic plant percentage, native plant percentage, and the ratio of exotic to native plants among areas with varying grazing intensities. All data were previously square root transformed to improve normality and homoscedasticity. The figures and tables present the results with raw data.
The proportion of each functional group, categorizing plant species based on their response to grazing livestock, was compared within each of the three types of grazing intensity using a simple analysis of variance with a completely randomized design, and means were compared using a Tukey test [50]. Data were transformed using the arcsine square root transformation.
The variation in species composition across grasslands with varying grazing intensities was assessed using Principal Component Analysis (PCA) with the Vegan package in RStudio [51], employing a correlation matrix [52]. Data were transformed using the arccosine of the square root to rectify Euclidean distance distortion in spatial representation. Plant species occurring in less than 5% of total plots were excluded. Species significantly correlated (p < 0.05) with at least one principal component were considered as explanatory variables for PCA outcomes [53]. To determine if the ordination of plant species could be explained by differential response to domestic livestock grazing intensity, a Pearson’s correlation test was conducted between ordination outcomes and the abundance of plant functional groups.
The species composition and functional structure of Austral Pampas grasslands were influenced by the intensity of livestock grazing, regardless of site locations. There were no differences between sampling sites with the same grazing intensity (site location), and no significant interaction between grazing intensity and site location was observed for any of the parameters examined (Table S2). The lack of significant interactions indicated the independence of these factors from each other and from the site location. Therefore, all experimental plots were considered independent and treated as replicates for the intensity analysis [40].
Species diversity, uniformity, and functional richness were significantly higher in the moderately grazed grasslands (F = 3.11, p < 0.05; F = 7.9, p < 0.005; and F = 7.2, p < 0.005, respectively) (Fig. 1). Conversely, functional redundancy was significantly greater (F = 7.1, p < 0.005) in the grasslands without domestic livestock than in the grasslands intensively grazed (Fig. 2). No differences in species richness were observed across grasslands with varying grazing intensities (F = 1.2, p > 0.20). Native plants were more abundant (F = 5.4, p < 0.05) in grasslands without domestic livestock, while higher abundances of exotic plants were found in grasslands with heavy and moderate grazing (F = 4.4, p < 0.05 and F = 7.4, p < 0.005, respectively) (Fig. 1). As anticipated, the ratio of native to exotic plants was higher (F = 12.6, p < 0.001) in the grasslands without domestic livestock (Fig. 1). Additionally, the percentage of bare soil was higher (F = 17.01, p < 0.0005) in intensively grazed grasslands (Fig. 2).
Figure 1: Average (±SE) of species richness expressed as the number of species, exotic and native plant species (%), bare soil (%), and the native-to-exotic ratio in the sampling areas excluded from domestic livestock grazing (exc), moderately grazed (mg), and intensively grazed by domestic livestock (ig). Columns with different letters are significantly different (p < 0.05)
Figure 2: Average (±SE) of the functional redundancy, functional diversity, uniformity, and species diversity indices in the sampling areas excluded from domestic livestock grazing (exc), moderately grazed (mg), and intensively grazed by domestic livestock (ig). Columns with different letters are significantly different (p < 0.05)
We recorded a total of 143 plant species. All recorded species were classified in five functional groups defined by their response to grazing: avoidance (54 species), tolerant (18 species), sensitive (33 species), favored (18 species) and neutral (20 species) (Table S3). Grazing intensity significantly affected the relative abundance of functional groups. In both grasslands, intensively and moderately grazed by livestock, there was a higher proportion of grazing-avoiding species (F = 12.3, p < 0.005 and F = 9.3, p < 0.001, respectively), represented by herbaceous plants with prostrate growth, rosettes, spines, or with the presence of toxic secondary compounds or unpalatable to livestock (Fig. 3). Conversely, in livestock-free grasslands, a higher proportion of grazing-sensitive species (F = 10.9, p < 0.005) was recorded, mostly represented by palatable perennial grasses (Fig. 3).
Figure 3: Average (±SE) proportion of neutral, sensitive, favored, tolerant, and avoidance species in the sampling areas excluded from domestic livestock grazing (exc), moderately grazed (mg), and intensively grazed by domestic livestock (ig). Columns with different letters are significantly different (p < 0.05)
Principal Component Analysis revealed a pattern of variation in the vegetation samples from the study sites consistent with the intensity of livestock grazing. The first two principal components accounted for 70% of the total variance of the samples ordered based on species abundance. The first component (PC1) (47% of the variance) separated the samples into two groups based on the presence of domestic livestock, while ordination on the second component (PC2) (23% of the variance) allowed discrimination between heavily grazed grasslands and those with moderate grazing (Fig. 4). Samples from areas closed to domestic livestock grazing formed a group characterized by higher abundances of Piptochaetium hackelii (Arechav.) Parodi, Nassella neesiana (Trin. & Rupr.) Barkworth, Lathyrus tomentosus Lam., and Baccharis artemisioides Hook. & Arn., with significant positive correlations with PC1 and PC2. At the negative end of PC1, plots from sites with intense domestic livestock grazing were associated with a high cover of Echium plantagineum L., Discaria americana Gillies & Hook., Chaptalia integerrima (Vell.) Burkart and Dichondra sericea Sw. with negative correlations with PC2; while moderately grazed grasslands were significantly associated with Mimosa rocae Lorentz & Niederl., Conium maculatum L., and Carduus pycnocephalus L., with positive correlations with PC2 (Fig. 4 and Table S4).
Figure 4: Diagram illustrating the ordination of Principal Component Analysis (PCA) of sampled plots (circles) from the sampling areas categorized as excluded from domestic livestock grazing (exc), moderately grazed (mg), and intensively grazed by domestic livestock (ig), depicted in the plane defined by the first two components (PCA1 and PCA2). The plant species exhibiting significant (p < 0.05) correlations with the ordination axes are as follows: Baccharis artemisioides (Baar), Carduus pycnocephalus (Capy), Chaptalia integerrina (Chin), Conium maculatum (Coma), Discaria americana (Diam), Dichondra sericea (Dise), Echium plantagineum (Ecpl), Lathyrus tomentosus (Lato), Mimosa rocae (Miro), Nasella neesiana (Nane), and Piptochaetium hackelii (Piha)
Significant correlations (r = 0.33, p < 0.05) were found between the group of species sensitive to grazing with PC1 and PC2, associating this group with grasslands without domestic livestock. In contrast, species groups with grazing-avoidance or grazing-favored characteristics were negatively correlated with PC1 and PC2, being associated with heavily grazed grasslands (Fig. 5).
Figure 5: Heat map illustrating the correlations between plant functional groups and the first two principal components (PCA1 and PCA2). Dark red represents a strong negative correlation, while dark blue indicates a strong positive correlation. The size of the ellipses reflects the strength of the correlation. Pearson’s correlation coefficient r ≤ ±0.333; p < 0.05
According to our hypotheses, we found a significant relationship between grazing intensity of domestic livestock and the specific and functional structure of grasslands in the Austral Pampas. Moderate grazing increased species and functional diversity without altering species richness, while functional redundancy was lower than in grazed grasslands. High livestock grazing reduced plant diversity, agreeing with previous studies [34,35,54–56]. However, species richness did not vary with grazing pressure, suggesting it may not effectively indicate grazing intensity effects on the composition of Austral Pampas grasslands. Both species richness and species diversity are usually used to characterize the composition and its changes associated with the different historical uses of natural grasslands [57,58]. However, in our study, species richness was not sensitive to variations in grazing intensity, while specific and functional diversity indices were consistent with our hypothesis.
The Austral Pampas grasslands could be classified as sub-humid productive grasslands [24]. This classification suggests that moderate grazing would increase species diversity, uniformity, and functional redundancy in natural grasslands, allowing sensitive and tolerant plant species to coexist while preventing alien plant invasion [59]. Moderate grazing in semiarid grasslands in Uruguay enhances resilience to climate change [42], but our findings indicate a decrease in both resistance and resilience in grazed grasslands, reducing their ability to withstand disturbances like fires and droughts. The stability of natural systems depends on their resistance and resilience, which are influenced by functional responses to environmental changes [60]. Greater functional diversity, uniformity, and redundancy is critical for mitigating disturbances within ecosystems. However, moderate grazing areas in the Austral Pampas grasslands may reduce functional uniformity and redundancy, increasing vulnerability to disturbances. Anthropogenic influences, such as reduced native herbivore populations and the introduction of domestic ungulates, can alter the impact of herbivory on vegetation because the effects of introduced ungulates on plant communities can differ significantly from the effects of native species due to variations in habitat use and resource consumption [36]. Grasslands adapted to moderate grazing, like South American grasslands, are more prone to overgrazing compared to regions that coevolved with large herds of ungulates, such as African savannas and North American prairies [14,22]. Prior to European colonization, native ungulates like pampas deer and guanacos maintained the structure and composition of natural grasslands, but the replacement of these native grazers with domestic livestock at high stocking rates has intensified historical grazing pressure in the Austral Pampas, leading to structural and compositional changes in the grasslands by overcoming the ecological resistance of these grasslands [34,35].
The presence of domestic livestock influenced the balance between native and exotic plants in the grasslands of Austral Pampas, with higher abundances of native plants observed in areas without livestock and higher abundances of exotic plants in heavily and moderately grazed areas. Consequently, the ratio of native to exotic plants was higher in the grasslands without domestic livestock. This may have maintained high levels of both specific and functional diversity in grasslands under moderate grazing compared to those grazed intensively or without domestic livestock. Livestock grazing also favored the replacement of palatable plant species, primarily perennial grasses, with unpalatable species (prostrate perennial herbs, thorny plants, or shrubs), influencing changes in both specific and functional diversity. Our results are consistent with those found in other South American grasslands. For instance, in the Flooding Pampas, livestock grazing promoted an increase in plant species diversity due to the proliferation of exotic grasses [61], while in the Uruguayan grasslands, this increase was attributed to the growth of prostrate grasses and unpalatable herbs [62].
The proportion of bare ground was higher in the moderately and heavily grazed grasslands compared to livestock-free areas, creating low-competition microsites that increase the likelihood of exotic plant establishment or the replacement of desirable species with undesirable ones (non-palatable and shrubs). These microsites present opportunities for invasive species establishment and spread [63], particularly when other favorable environmental conditions align, such as sufficient precipitation, or the absence of fires [64,65]. In the grasslands of the Austral Pampas, the decline in perennial grass abundance due to overgrazing by feral horses, coupled with fire suppression, facilitated the invasion of Pinus halepensis [66] and Spartium junceum [67]. Therefore, the reduction of perennial grass cover by domestic livestock, whether at moderate or high intensity, may favor subordinate native species to the abundance of perennial grasses, but also the weeds that reduce the forage quality of these grasslands. The high-intensity grazing reduces the abundance of high-quality forage plant species in arid and semiarid regions of North America, allowing species that are resistant to high-intensity grazing to become dominant [68]. This leads to the spread of toxic species and an increase in bare soil, generating a positive feedback process that can only be reversed through the application of restoration techniques [69,70].
The intensity of livestock grazing regimes strongly influenced the representation of functional groups in the Austral Pampas grasslands. We identified three distinct functional associations, each linked to a specific grazing intensity. In grasslands under moderate grazing, high species diversity is supported by the coexistence of annual herbs and some subordinate perennials of medium to high forage value, as predicted by the IDH. As grazing pressure increases, species replacement occurs, favoring plants that can tolerate or avoid grazing over more palatable ones [3], leading to a reduction in species diversity. These changes in composition and structure were observed in grasslands of the Austral Pampas, even under a moderate grazing regime. Grazing-sensitive plants, such as Nassella neesiana and Piptochaetium hackelii, were abundant in livestock-free areas. They were replaced by grazing-avoiding plants in grazed grasslands, regardless of grazing pressure. Dichondra sericea (prostrate herb) and Discaria americana (spiny shrub) were abundant in areas with high grazing intensity, while Mimosa rocae (prostrate shrub) and Conium maculatum (toxic herb) were prevalent in moderately grazed areas. Livestock grazing has led to the selection of opportunistic species with grazing-avoidance strategies in the Austral Pampas grasslands, including exotic plants like Echium plantagineum in heavily grazed areas, and Carduus pycnocephalus in moderately grazed ones, both known for their toxicity and impact on pasture productivity [71,72]. Effective management of these species is essential to mitigate their economic impacts and maintain grassland productivity [73].
Our study provides a thorough examination of the Intermediate Disturbance Hypothesis as applied to grazing intensity and its impact on the productivity of grasslands in the Austral Pampas. Even moderate levels of grazing intensity result in undesirable changes to both the composition and structure of the grasslands, despite the increased functional and specific diversity and uniformity observed. When promoting the establishment and spread of undesirable plant species, their productive value may diminish and the system’s resilience to other disturbances may be reduced. To manage grazing intensity effectively with domestic livestock, in order to maintain the forage capacity of Southern Pampas grasslands, it is essential to assess vegetation response to different grazing intensities using indices that reflect both species diversity and functional diversity within the system. Furthermore, our findings indicate the development of distinct functional assemblages within the grazing community, each associated with varying grazing levels. This knowledge is crucial for devising successful conservation and management strategies to safeguard biodiversity and ecological integrity in the Pampas biome. In conclusion, our results suggest that livestock grazing in the Austral Pampas is unlikely to yield positive outcomes for ecosystem structure, function, and composition unless specific response variables are targeted through explicit management objectives. Rangeland managers should tailor grazing strategies to conserve plant diversity based on site-specific conditions, rather than adopting a one-size-fits-all approach as proposed by the Intermediate Disturbance Hypothesis.
Acknowledgement: We wish to thank the administrations of Ernesto Tornquist Provincial Park and Sierras Grandes Protected Area, Buenos Aires Province. We are especially grateful to Centro de Recursos Naturales Renovables de la Zona Semiárida-CERZOS (CONICET/UNS) and Departamento de Biología, Bioquímica y Farmacia, Universidad Nacional del Sur. We would like to express our gratitude to Luciana Caserta for her assistance in editing the figures and to Alexia Insua for reviewing the English in this article. Their contributions were invaluable in improving the quality of this research.
Funding Statement: This work was funded by CONICET (Consejo Nacional de Investigaciones Científicas y Técnicas) and SGCyT (Secretaría General de Ciencia y Tecnología, Universidad Nacional del Sur, Argentina).
Author Contributions: Ana Elena de Villalobos and María Andrea Long were involved in the study’s conception, design, data collection and analysis. All authors reviewed the results and approved the final version of the manuscript.
Availability of Data and Materials: All data included in this study are available upon request by contacting with the corresponding author.
Ethics Approval: Not applicable.
Conflicts of Interest: The authors declare that they have no conflicts of interest to report regarding the present study.
Supplementary Materials: The supplementary material is available online at https://doi.org/10.32604/phyton.2024.053928.
References
1. O’Mara FP. The role of grasslands in food security and climate change. Ann Bot. 2012;110(6):1263–70. doi:10.1093/aob/mcs209. [Google Scholar] [PubMed] [CrossRef]
2. Akash M, Hoque S, Mondal DB. Sustainable livestock production and biodiversity. In: Sukanta R, Lakhan S, editors. Emerging issues in climate smart livestock production. Cambridge: Academic Press; 2022. p. 91–108. [Google Scholar]
3. Gao J, Carmel Y. Can the intermediate disturbance hypothesis explain grazing-diversity relations at a global scale? Oikos. 2019;129(4):493–502. doi:10.1111/oik.06338. [Google Scholar] [CrossRef]
4. Eldridge DJ, Poore AGB, Ruiz-Colmenero M, Letnic M, Soliveres S. Ecosystem structure, function, and composition in rangelands are negatively affected by livestock grazing. Ecol Appl. 2016;26(4):1273–83. doi:10.1890/15-1234. [Google Scholar] [PubMed] [CrossRef]
5. Bardgett RD, Bullock JM, Lavorel S, Manning P, Schaffner U, Ostle N, et al. Combatting global grassland degradation. Nat Rev Earth Environ. 2021;2(10):720–35. doi:10.1038/s43017-021-00207-2. [Google Scholar] [CrossRef]
6. Tiscornia G, Jaurena M, Baethgen W. Drivers, process, and consequences of native grassland degradation: insights from a literature review and a survey in Río de la Plata grasslands. Agronomy. 2019;9(5):239. doi:10.3390/agronomy9050239. [Google Scholar] [CrossRef]
7. Kachler J, Benra F, Bolliger R, Isaac R, Bonn A, Felipe-Lucia MR. Can we have it all? The role of grassland conservation in supporting forage production and plant diversity. Landsc Ecol. 2023;38:4451–65. doi:10.1007/s10980-023-01729-4. [Google Scholar] [CrossRef]
8. Connell JH. Diversity in tropical rain forests and coral reefs. Science. 1978;199:1302–10. [Google Scholar] [PubMed]
9. Shea K, Roxburgh SH, Rauschert ESJ. Moving from pattern to process: coexistence mechanisms under intermediate disturbance regimes. Ecol Lett. 2004;7(6):491–508. [Google Scholar]
10. Zhang Y, Wuriliga LP, Fan R, Guo J, Liu L, Ding Y. Effect of grazing and climatic factors on biodiversity-ecosystem functioning relationships in grassland ecosystems–a case study of typical steppe in Inner Mongolia, China. Front Plant Sci. 2023;22(14):1297061. doi:10.3389/fpls.2023.1297061. [Google Scholar] [PubMed] [CrossRef]
11. Marull J, Tello E, Fullana N, Murray I, Jover G, Font C, et al. Long-term bio-cultural heritage: exploring the intermediate disturbance hypothesis in agro-ecological landscapes (Mallorca, c. 1850–2012). Biodivers Conserv. 2015;24(13):3217. [Google Scholar]
12. Gong X, Wang Y, Zhan T, Wang C, Li C, Liu Y. Advances in meta-analysis of the effects of grazing on grassland ecosystems in China. Agriculture. 2023;13(5):1084. doi:10.3390/agriculture13051084. [Google Scholar] [CrossRef]
13. Vesk PA, Westoby M. Predicting plant species’ responses to grazing. J Appl Ecol. 2001;38(5):897–909. doi:10.1046/j.1365-2664.2001.00646.x. [Google Scholar] [CrossRef]
14. Davies KW, Boyd CS. Ecological effects of free-roaming horses in North American rangelands. BioScience. 2019;69(7):558–65. doi:10.1093/biosci/biz060. [Google Scholar] [CrossRef]
15. Briske DD. Strategies of plant survival in grazed systems: a functional interpretation. In: Hodgson J, Illius A, editors. The ecology and management of grazed systems. Wallingford: CAB International; 1996. p. 37–67. [Google Scholar]
16. Díaz S, Lavorel S, McIntyre S, Falczuk V, Casanoves F, Milchunas DG, et al. Plant trait responses to grazing–a global synthesis. Glob Chang Biol. 2007;13(2):313–41. doi:10.1111/j.1365-2486.2006.01288.x. [Google Scholar] [CrossRef]
17. Graff P, Aguiar MR, Chaneton EJ. Shifts in positive and negative plant interactions along a grazing intensity gradient. Ecology. 2007;88(1):188–99. doi:10.1890/0012-9658(2007)88[188:SIPANP]2.0.CO;2. [Google Scholar] [CrossRef]
18. Hobbs RJ, Huenneke LF. Disturbance, diversity, and invasion implications for conservation. Conserv Biol. 1992;6:324–37. [Google Scholar]
19. Briske DD, Coppock DL, Illius AW, Fuhlendorf SD. Strategies for global rangeland stewardship: assessment through the lens of the equilibrium-non-equilibrium debate. J Appl Ecol. 2020;1–12. doi:10.1111/1365-2664.13610. [Google Scholar] [CrossRef]
20. Mackey RL, Currie DJ. The diversity-disturbance relationship: is it generally strong and peaked? Ecology. 2001;82(12):3479–92. [Google Scholar]
21. Sasaki T, Okubo S, Okayasu T, Jamsran U, Ohkuro T, Takeuchi K. Management applicability of the intermediate disturbance hypothesis across Mongolian rangeland ecosystems. Ecol Appl. 2009;19(2):423–32. [Google Scholar] [PubMed]
22. Milchunas DG, Sala OE, Lauenroth WK. A generalized model of the effects of grazing by large herbivores on grassland community structure. Am Nat. 1988;132(1):87–106. [Google Scholar]
23. Ding S, van der Plas F, Li J, Liu B, Xu M, Xu T, et al. Effects of grazing on the relationship between plant functional diversity and soil carbon sequestration are regulated by livestock species. J Plant Ecol. 2024;17(5):62. doi:10.1093/jpe/rtae016. [Google Scholar] [CrossRef]
24. Milchunas DG, Lauenroth WK. Quantitative effects of grazing on vegetation and soils over a global range of environments. Ecol Monogr. 1993;63(4):32–366. doi:10.2307/2937150. [Google Scholar] [CrossRef]
25. Bloor JMG, Pottier J. Grazing and spatial heterogeneity: implications for grassland structure and function. In: Mariotte P, Kardol P, editors. Grassland biodiversity and conservation in a changing world. New York: Nova Science Publishers; 2022. p. 135–62. [Google Scholar]
26. Mouchet MA, Villéger S, Mason NWH, Mouillot D. Functional diversity measures: an overview of their redundancy and their ability to discriminate community assembly rules. Funct Ecol. 2010;24(4):867–76. doi:10.1111/j.1365-2435.2010.01695.x. [Google Scholar] [CrossRef]
27. Ohlert T, Kimmel K, Avolio M, Chang C, Forrestel E, Gerstner B, et al. Exploring the impact of trait number and type on functional diversity metrics in real-world ecosystems. PLoS One. 2022;17(8):e0272791. doi:10.1371/journal.pone.0272791. [Google Scholar] [PubMed] [CrossRef]
28. Ricotta C, de Bello F, Moretti M, Caccianiga M, Cerabolini BEL, Pavoine S. Measuring the functional redundancy of biological communities: a quantitative guide. Methods Ecol Evol. 2016;7:1386–95. doi:10.1111/2041-210X.12604. [Google Scholar] [CrossRef]
29. Ellen A, Smith E, Emily M, Holden C, Brown C, James F, et al. Disturbance has lasting effects on functional traits and diversity of grassland plant communities. PeerJ. 2022;10:e13179. doi:10.7717/peerj.13179. [Google Scholar] [PubMed] [CrossRef]
30. Avramidou E, Karamichali I, Tsiripidis I, Abraham EM. Diversity levels under different grazing intensities in semi-wet grasslands. Land. 2024;13(4):488. doi:10.3390/land13040488. [Google Scholar] [CrossRef]
31. Song S, Zhu J, Zheng T, Tang Z, Zhang F, Ji C, et al. Long-term grazing exclusion reduces species diversity but increases community heterogeneity in an alpine grassland. Front Ecol Evol. 2020;8:66. doi:10.3389/fevo.2020.00066. [Google Scholar] [CrossRef]
32. Arana MD, Natale E, Ferretti N, Romano G, Oggero A, Martínez G, et al. Biogeographic scheme of the Republic of Argentina. Opera Lilloana. 2021;56:1–238 (In Spanish). [Google Scholar]
33. Bilenca DN, Miñarro F. Identification of valuable grassland areas (AVPs) in the pampas and fields of Argentina, Uruguay and southern Brazil. Buenos Aires: Fundación Vida Silvestre Argentina; 2004 (In Spanish). [Google Scholar]
34. de Villalobos AE, Zalba SM. Continuous feral horse grazing and grazing exclusion in mountain pampean grasslands in Argentina. Acta Oecol. 2010;36(5):514–9. doi:10.1016/j.actao.2010.07.004. [Google Scholar] [CrossRef]
35. de Villalobos AE, Schwerdt L. Feral horses and alien plants: effects on the structure and function of the Pampean Mountain grasslands (Argentina). Écoscience. 2017;25(1):1–12. doi:10.1080/11956860.2017.1409476. [Google Scholar] [CrossRef]
36. Lunt ID, Eldridge DJ, Morgan JW, Witt GB. A framework to predict the effects of livestock grazing and grazing exclusion on conservation values in natural ecosystems in Australia. Aust J Bot. 2007;55(4):401–15. doi:10.1071/BT06178. [Google Scholar] [CrossRef]
37. Guerrero EL, Apodaca MJ. The smallest area shaped a big problem: a revision of Ventania sky island placement in the biogeography of South America. La Plata: Universidad Nacional de La Plata; 2022 (In Spanish). [Google Scholar]
38. Kristensen MJ, Frangi JL. Vegetation and mesoclimates of rock outcrops in Ventania (Buenos Aires, Argentina). Bol Soc Argent Bot. 2015;50(1):35–46 (In Spanish). [Google Scholar]
39. Michalijos MP. Study of forest fire risk in a sector of the Sierra de la Ventana region using geotechnologies. Bahía Blanca: Universidad Nacional del Sur; 2019 (In Spanish). [Google Scholar]
40. Cabrera AL. Argentine phytogeographic regions. In: Argentine encyclopedia of agriculture and gardening. Buenos Aires: ACME; 1976 (In Spanish). [Google Scholar]
41. Frangi JL, Bottino OJ. Plant communities of the Sierra de la Ventana, Province of Buenos Aires, Argentina. Rev Fac Agron. 1995;71(1):93–133 (In Spanish). [Google Scholar]
42. Modernel P, Rossing WAH, Corbeels M, Dogliotti S, Picasso V, Tittonell P. Land use change and ecosystem service provision in Pampas and Campos grasslands of southern South America. Environ Res Lett. 2016;11:113002. doi:10.1088/1748-9326/11/11/113002. [Google Scholar] [CrossRef]
43. McDonald JH. Handbook of biological statistics. Maryland: Sparky House; 2014. [Google Scholar]
44. Krebs CJ. Ecology: the experimental analysis of distribution and abundance. New York: Pearson Benjamin Cummings; 2009. [Google Scholar]
45. Zuloaga FO, Belgrano MJ, Zanotti CA. Actualización del catálogo de las plantas vasculares del Cono Sur. Darwiniana, Nueva Serie. 2019;7(2):208–78 (In Spanish). doi:10.14522/darwiniana.2019.72.861. [Google Scholar] [CrossRef]
46. Diaz S, Briske D, McIntyre S. Range management and plant functional types. In: Hodgkinson K, Grice AC, editors. Global rangelands: progress and prospects. Wallingford, UK: CAB International; 2002. p. 81–100. [Google Scholar]
47. Pielou EC. Ecological diversity. New York: John Wiley & Sons; 1975. [Google Scholar]
48. Bricca A, Carranza ML, Varricchione M, Cutini M, Stanisci A. Exploring plant functional diversity and redundancy of mediterranean high-mountain habitats in the apennines. Diversity. 2021;13(10):466. doi:10.3390/d13100466. [Google Scholar] [CrossRef]
49. Casanoves F, Pla L, Di Rienzo JA, Díaz S. FDiversity: a software package for the integrated analysis of functional diversity. Methods Ecol Evol. 2010;1(4):416–9. doi:10.1111/j.2041-210X.2010.00082.x. [Google Scholar] [CrossRef]
50. Zar JH. Biostatistical analysis. 5th edUpper Saddle River: Prentice Hall; 2009. [Google Scholar]
51. Oksanen FJ, Blanchet G, Friendly M, Kindt R, Legendre P, McGlinn D, et al. Vegan: community ecology package. R package version 2.4-3; 2017. Available from: https://CRAN.R-project.org/package=vegan. [Accessed 2024]. [Google Scholar]
52. Legendre P, Legendre L. Numerical ecology. 2nd edAmsterdam: Elsevier; 1998. [Google Scholar]
53. Legendre P, Gallagher ED. Ecologically meaningful transformations for ordination of species data. Oecologia. 2001;129(2):271–80. doi:10.1007/s004420100716. [Google Scholar] [PubMed] [CrossRef]
54. Jordan SE, Palmquist KA, Burke IC, Lauenroth WK. Small effects of livestock grazing intensification on diversity, abundance, and composition in a dryland plant community. Ecol Appl. 2022;32(8):e2693. doi:10.1002/eap.2693. [Google Scholar] [PubMed] [CrossRef]
55. Pakeman RJ, Fielding DA, Everts L, Littlewood NA. Long-term impacts of changed grazing regimes on the vegetation of heterogeneous upland grasslands. J Appl Ecol. 2019;56(7):1794–805. doi:10.1111/1365-2664.13420. [Google Scholar] [CrossRef]
56. Sandoval-Calderon AP, Rubio Echazarra N, van Kuijk M, Verweij PA, Soons M, Hautier Y. The effect of livestock grazing on plant diversity and productivity of mountainous grasslands in South America–a meta-analysis. Ecol Evol. 2024;14(4):e11076. doi:10.1002/ece3.11076. [Google Scholar] [PubMed] [CrossRef]
57. Bruun HH, Fritzbøger B, Rindel PO, Hansen UL. Plant species richness in grasslands: the relative importance of contemporary environment and land-use history since the Iron Age. Ecography. 2001;24(5):569–78. doi:10.1111/j.1600-0587.2001.tb00491.x. [Google Scholar] [CrossRef]
58. Price JN, Sitters J, Ohlert T, Tognetti PM, Brown CS, Seabloom EW, et al. Evolutionary history of grazing and resources determine herbivore exclusion effects on plant diversity. Nat Ecol Evol. 2022;6(9):1290–8. doi:10.1038/s41559-022-01809-9. [Google Scholar] [PubMed] [CrossRef]
59. Cingolani AM, Noy-Meir I, Díaz S. Grazing effects on rangeland diversity: a synthesis of contemporary models. Ecol Appl. 2005;15(2):757–73. doi:10.1890/03-5272. [Google Scholar] [CrossRef]
60. Lin X, Zhao H, Zhang S, Singh VP, Li R, Luo M, et al. Global response of different types of grasslands to precipitation and grazing, especially belowground biomass. Agric Ecosyst Environ. 2024;363(1):108852. doi:10.1016/j.agee.2023.108852. [Google Scholar] [CrossRef]
61. Chaneton EJ, Perelman SB, Omacini M, León RJC. Grazing, environmental heterogeneity, and alien plant invasions in temperate Pampa grasslands. Biol Invasions. 2002;4(1/2):7–24. doi:10.1023/A:1020536728448. [Google Scholar] [CrossRef]
62. Rodríguez C, Leoni E, Lezama F, Altesor A. Temporal trends in species composition and plant traits in natural grasslands of Uruguay. J Veg Sci. 2003;14(3):433–40. doi:10.1111/j.1654-1103.2003.tb02169.x. [Google Scholar] [CrossRef]
63. Johnstone IM. Plant invasion windows: a time-based classification of invasion potential. Biol Rev. 1986;61(4):369–94. doi:10.1111/j.1469-185x.1986.tb00659.x. [Google Scholar] [CrossRef]
64. Keeley JE, Lubin D, Fotheringham CJ. Fire and grazing impacts on plant diversity and alien plant invasions in the southern Sierra Nevada. Ecol Appl. 2003;13(5):1355–74. doi:10.1890/02-5002. [Google Scholar] [CrossRef]
65. Kraaij T, Ward D. Effects of rain, nitrogen, fire and grazing on tree recruitment and early survival in bush-encroached savanna, South Africa. Plant Ecol. 2006;186(2):235–46. doi:10.1007/s11258-006-9125-4. [Google Scholar] [CrossRef]
66. de Villalobos AE, Zalba SM, Peláez DV. Pinus halepensis invasion in mountain pampean grassland: effects of feral horses grazing on seedling establishment. Environ Res. 2011;111(7):953–9. doi:10.1016/j.envres.2011.03.011. [Google Scholar] [PubMed] [CrossRef]
67. Sanhueza C, Zalba S. Banco de semillas, germinación y longevidad de semillas de retama (Spartium junceum, Fabaceaeimplicancias para su control. Bol Soc Argent Bot. 2014;49(1):67–76. doi:10.31055/1851.2372.v49.n1.7822. [Google Scholar] [CrossRef]
68. Porensky LM, McGee R, Pellatz DW. Long-term grazing removal increased invasion and reduced native plant abundance and diversity in a sagebrush grassland. Glob Ecol Conserv. 2020;24(7):e01267. doi:10.1016/j.gecco.2020.e01267. [Google Scholar] [CrossRef]
69. Callaway RM. Direct mechanisms for facilitation. In: Callaway RM, editor. Positive interactions and interdependence in plant communities. Dordrecht: Springer; 2007. p. 15–116. [Google Scholar]
70. Staude IR, Segar J, Temperton VM, Andrade BO, Dechoum MdS, Weidlich EWA, et al. Prioritize grassland restoration to bend the curve of biodiversity loss. Restor Ecol. 2023;31(5):e13931. doi:10.1111/rec.13931. [Google Scholar] [CrossRef]
71. Grigulis K, Sheppard AW, Ash JE, Groves RH. The comparative demography of the pasture weed Echium plantagineum between its native and invaded ranges. J Appl Ecol. 2001;38(2):281–90. doi:10.1046/j.1365-2664.2001.00587.x. [Google Scholar] [CrossRef]
72. Ghanizadeh H, Harrington KC. Weed management in New Zealand pastures. Agronomy. 2019;9(8):448. doi:10.3390/agronomy9080448. [Google Scholar] [CrossRef]
73. Boinot S, Alignier A, Storkey J. Landscape perspectives for agroecological weed management. A review. Agron Sustain Dev. 2024;44(7):1–5. doi:10.1007/s13593-023-00941-5. [Google Scholar] [CrossRef]
Cite This Article
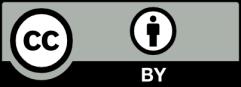
This work is licensed under a Creative Commons Attribution 4.0 International License , which permits unrestricted use, distribution, and reproduction in any medium, provided the original work is properly cited.