Open Access
ARTICLE
Rehabilitation of Semi-Arid Grasslands through the Perennialization of Lots by Implementing Perennial Forage Exotic Grass
1 Universidad Nacional de Río Negro, Sede Atlántica, CEANPa, Viedma, 8500, Argentina
2 Consejo Nacional de Investigaciones Científicas y Técnicas, CONICET, CABA, Buenos Aires, 1425, Argentina
3 Chacra Experimental Patagones, Ministerio de Desarrollo Agrario de la Provincia de Buenos Aires, Carmen de Patagones, 8504, Argentina
* Corresponding Authors: Juan Manuel Zeberio. Email: ; Guadalupe Peter. Email:
(This article belongs to the Special Issue: Ecology of Rangelands in Argentina)
Phyton-International Journal of Experimental Botany 2024, 93(8), 2115-2125. https://doi.org/10.32604/phyton.2024.053483
Received 01 May 2024; Accepted 07 August 2024; Issue published 30 August 2024
Abstract
Argentina is the country with the highest proportion of arid and semi-arid ecosystems in Latin America. In the rangelands of Southwestern Buenos Aires (Patagones Department), there is a clear advancement of the agricultural frontier to the detriment of the native forest in this region. Due to rainfall variation and seed acquisition, Thinopyrum ponticum is cultivated as a forage perennial crop in this region. Our objective was to evaluate the performance of T. ponticum as a facilitating crop for the medium-term rehabilitation of natural grasslands in semi-arid areas. The working hypotheses were that: 1) native perennial grass cover increases over the years and 2) diversity and specific richness of the vegetation are enhanced by the duration of Tall Wheatgrass implantation. Data were collected from commercial plots where T. ponticum was shown: recent implantation (5–8 years, RI); medium implantation (13–15 years, MI); and old implantation (20–22 years, OI). Thirty-four species were identified and classified into seven functional groups: Annual grasses, annual herbs, perennial herbs, exotic perennial herbs, perennial forage grasses, exotic perennial forage grasses, perennial forage exotic grasses, and non-forage perennial grasses. Thinopyrum ponticum’s total cover was between RI and OI. Total cover, species richness, and Shannon-Weaver diversity index showed no differences among treatments. Perennial forage grasses exhibited higher cover values in sites with greater implantation age and annual grasses showed the opposite response. Our results indicated that T. ponticum does not invade the sampled plots and enhances the colonization of the planted plots by perennial forage native species. However, even though the herbaceous cover had been recovered, the woody layer which could provide environmental services and specific values for conservation was not.Keywords
Arid and semi-arid zones are essential for human development in many parts of the world and they sustain approximately 50% of global livestock [1]. These areas exhibit a relationship between medium annual precipitation and medium potential evapotranspiration of less than 0.65 [2]. More than 65% of these ecosystems are used for grazing, with natural vegetation serving as forage for domestic livestock, whereas only 25% are dedicated to agriculture [1].
These ecosystems are complex and multifunctional, often resulting in the provision of a wide range of services overlooked during management planning. They are highly sensitive to the impact generated by anthropogenic activities, which brings about a loss of resilience [3]. Consequently, they have become degraded due to actions such as the introduction of agricultural or forage crops, often followed by the total or partial removal of native vegetation (woody and herbaceous), without consideration for soil and climatic characteristics that influence their stability and sustainability [4].
Argentina contains arid, semi-arid, and dry sub-humid areas, being the Latin American country with the highest proportion (75%) of these ecosystems [5]. Here, the primary use of semi-arid ecosystems is oriented towards livestock grazing [6]. The incorporation of large land areas in the transition zone between Monte and Espinal into new productive paradigms was possible through the deforestation of native vegetation. These changes in the use of the land have enhanced degradation, which causes an impact on the environmental, social, and economic aspects [7].
The rangelands of Southwestern Buenos Aires (Patagones Department) are part of the semi-arid regions of Argentina. The phytogeographical analysis proposed by Oyarzabal et al. [8] classifies this region into the vegetation unit known as Monte Oriental, which belongs to the phytogeographical province of Monte. The tree stratum is primarily represented by Geoffroea decorticans, while the shrub stratum includes Condalia microphylla, Neltuma flexuosa var. depressa, Larrea divaricata, and Chuquiraga erinacea, among others. Regarding the herbaceous stratum, it is dominated by the genera Jarava, Nassella, and Poa, although other species of high forage value such as Pappophorum caespitosum, Medicago minima, and Erodium cicutarium are also present [9].
Within the Patagones Department, the primary economic activity is oriented to livestock grazing [6]. However, there is a clear advancement of the agricultural frontier at the expense of the native forest in this region [10]. In 1975, dryland and irrigated agricultural lands accounted for 31% of the total surface area of the Department. This shift in land use resulted in a reduction of the area covered by native vegetation, which currently accounts for 20% [10].
In this semi-arid zone, agriculture productivity is highly dependent on precipitation, as the system is favored only if sowing coincides with a wet period [11].
In the area of study, the agricultural frontier advance includes woody plant cover management (reduction or total removal). Kröpfl et al. [12,13] observed that mechanically cleared sites exhibited symptoms of aridization and concluded that reversion may require active intervention by those managing the system. Rehabilitation and restoration of natural ecosystems consider pre-existing ecosystems as reference points. The difference is that while rehabilitation aims to recover processes, productivity, and ecosystem services, restoration pursues those goals and also seeks the reinstatement of the composition and structure of species from the original community [14].
Ecological restoration and rehabilitation employ native species adapted to the environmental conditions of the area to be restored [15]. Since seeds of native species are not commercially available, and harvesting in natural fields is technically difficult due to the absence of machines adapted to the morphology of the seeds and native plants, a commercially used exotic species was employed in this study. For the same reason, exotic species are cultivated for forage by farmers. One of the most used is Tall Wheatgrass (Thinopyrum ponticum), a perennial, tufted, glabrous grass, ranging from 0.70 to 1.40 m in height, whose growth is concentrated in the autumn. It is considered a forage with high nutritional value, high production, and resistance to grazing [16]. It is extremely tolerant to salinity and has only the drawback of some initial slow growth [17]. This species, alone or in association with others, requires a period to establish itself and be able to be used for direct grazing without the risk of losing grass due to damage from herbivores [17]. Patagones Department presents optimal an agro-climatic range for the development of this species because it possesses a precipitation range between 350 and 600 mm, and temperatures between 12°C and 20°C [18]. Its climatic characteristics, low natural reseeding capacity, and foraging pressure collectively limit the potential of T. ponticum to become an invasive species [18]. Some farmers in the area, upon observing native species colonization on the wheatgrass, have reseeded this pasture. This practice is carried out every 5 to 7 years to maintain the wheatgrass cultivation.
Taking advantage of the advancement of the native plant community on the implanted pasture, the objective of this work was to evaluate the performance of Tall Wheatgrass as a facilitating crop for medium-term natural grassland rehabilitation in semi-arid areas. The working hypotheses were that: 1) native perennial grass cover increases over the years and 2) diversity and specific richness of the vegetation are enhanced by the duration of Tall Wheatgrass implantation. The significance of this study lies in the complementary use of the facilitating crop until the rehabilitation of the system’s functions (e.g., soil protection, forage provision, and improvement of soil infiltration and fertility) through colonization by native forage grasses.
Sampling was conducted in commercial grazing properties located in Patagones Department in Buenos Aires province. The plots evaluated in this study have not been reseeded, and correspond to different implanting dates. The sowing was made in clear plots where it was used direct sowing with a density of 52 plants per square meter [19]. In the region, T. ponticum is used to perpetuate plots that have a history of use associated with extensive agriculture [6]. These plots were formerly used for the cultivation of winter or summer annual species (Avena spp., Sorghum spp.). Due to the inter and intra-annual variation in rainfall, farmers have changed their production practices and have begun to adopt perennial species [19]. According to their age, we have grouped them into three categories. Thus, data were collected from the vegetation of plots where T. ponticum was implanted 5, 7, and 8 years ago (A5, A7, A8); 13, 14, and 15 years ago (A13, A14, A15); and 20, 21, and 22 years ago (A20, A21, A22). Fig. 1 shows the geographical location of the sampling points. All plots where samples were taken correspond to plots where Thinopyrum ponticum was implanted and no further mechanical interventions were carried out on the soil, with the exception of grazing by sheep and cattle.
Figure 1: Sampling sites geographic location
The plots A8, A15, and A20 correspond to the same field, Patagones Experimental Ranch. Similarly, plots A5 and A14, as well as A13 and A7, correspond to identical fields. Samples were taken from a single plot in fields A22 and A21.
The point intercept method was used for field sampling [20]. A random site within the plot was selected to conduct the first 20-meter transect oriented North-South. This transect served as a reference to locate the other four transects following the same orientation and spaced 15 m apart. A touch was made every 20 cm along each 20-meter transect, resulting in 100 points per transect and a total of 500 points per plot. For each touch, the species name was recorded, as well as whether it touched bare soil, litter, or dung.
The species present in all sampling sites were classified into the following functional groups: Annual grasses (AG), annual herbs (AH), perennial herbs (PH), exotic perennial herbs (EPH), perennial forage grasses (PFG), exotic perennial forage grasses (EPFG), and non-forage perennial grasses (NFPG).
Following Peri et al. [21], total vegetation cover and cover by functional groups were estimated. Additionally, Shannon-Weaver diversity and species richness indices were calculated.
For data analysis, Infostat program [22] was used. The modified Shapiro-Wilk normality test and homoscedasticity test were performed to assess the normal distribution of the data. As the assumptions of normality and homoscedasticity were not met, the Kruskal-Wallis test was used. Fisher’s LSD test was employed to compare means with a significance level of p < 0.05.
The cover of Thynopirum ponticum was higher in sites where the implantation date was more recent (RI) than in sites of old implantation date (OI) (Fig. 2).
Figure 2: Cover (%) of Thynopirum ponticum in sites of recent (RI) and old (OI) implantation date. Different letters indicate statistically significant differences (p < 0.05)
Thirty-four species were identified and classified into the seven functional groups previously mentioned: annual grasses (AG), annual herbs (AH), perennial herbs (PH), exotic perennial herbs (EPH), perennial forage grasses (PFG), exotic perennial forage grasses (EPFG), and non-forage perennial grasses (NFPG) (Table 1).
Total cover of the sampled sites showed no significant differences among treatments (Table 2). Cover of the different functional groups identified in sites of varying ages showed a higher cover of annual grasses (AG) compared to older sites (OI) (Fig. 2). Species classified within this functional group included Eragrostis mexicana, Lolium multiflorum, Poa annua, and Schismus barbatus.
Perennial forage grasses (PFG) exhibited higher cover values in sites with greater age implantation with Thinopyrum ponticum (MI and OI) (Fig. 3). Species classified within this functional group included Distichlis scoparia, Jarava plumosa, Nassella longiglumis, Nassella tenuis, Pappophorum vaginatum, Pappophorum caespitosum, and Setaria mendocina.
Figure 3: Cover (%) of bare soil, litter, total vegetation, annual and perennial species as a function of implantation date. Note: BS, bare soil; AH, annual herbs; AG, annual grasses, EPFG, exotic perennials forage grasses; PFG, perennials forage grasses; EPH, exotic perennial herbs; PH, perennial herbs; NFPG, non forage perennial grasses
The remaining functional groups did not exhibit significant differences across the different implantation times of Thinopyrum ponticum (Table 2).
Species richness and Shannon-Weaver diversity index (H’) showed no differences among treatments. Twelve species were recorded in the youngest implantation sites (RI), eleven in the middle-aged ones (MI), and ten in the oldest ones (OI). The H’ values showed the same pattern (Table 1).
The date of establishment did not result in significant differences in terms of litter, bare soil and total cover, not proportion of soil covered by annual or perennial species (Fig. 3). As shown in Table 2, what was statistically significant was the proportion of native or exotic species within the perennial or annual categories, but not their total cover.
The fact that litter and total cover did not vary among treatments is indicative of the fact that the community of native perennial forage grasses replaced the cover of implanted exotic species. This is desirable in terms of ecosystem rehabilitation and it was the purpose of this study. Our results show that the cover of Thinopyrum ponticum decreases with implantation age (Fig. 2). Furthermore, this study demonstrates the potential of native species to establish themselves in old T. ponticum pastures and the resilience of semi-arid grasslands to soil disturbance and planting [23]. The reinstatement of natural grassland appears to be a medium-term process, as between 13 and 15 years after the planting of Thinopyrum ponticum crops, vegetation cover is predominantly composed of native grassland species (Table 2).
The removal of native plant communities and subsequent use of exotic species can cause environmental damage by altering patterns of ecosystem productivity, nutrient cycling, energy flows, and modifying community composition and structure [24]. Moreover, exotic species can displace native species and prevent them from surviving in new systems dominated by the former [25]. This does not seem to be the case with Thinopyrum ponticum, as census data from fields of varying planting ages show an increase in the total cover of PFG and AG.
Several studies conducted in arid and semi-arid areas have also reported a high level of replacement of cover of cultivated perennial species by natives within the first 10–20 years [26–29]. These results imply that recolonization processes in these hostile environments are not slow and show significant changes, resulting in native grass and herb communities within a few years after implantation.
A slight retraction in the cover of the functional group EPFG is observed after years of implantation. This retraction of cultivated species is observed across the MI (15 years old) and OI (>20 years) treatments and may be related to grazing use of these sampling sites and low natural reseeding capacity of the different cultivars of Thinopyrum ponticum used in the area [30].
Furthermore, in accordance with our initial hypothesis, there is an increase in species belonging to the functional group perennial forage grasses (PFG) ensuing planting age. This effect, combined with the retreat of AG, results in a change in pasture composition that has a positive effect by generating a more varied and uniform forage supply throughout the year, stabilizing forage production, and covering the soil from erosive effects caused by winds [31].
The rehabilitation of degraded ecosystems results in a community that is different from the original one, which includes arborous and shrubby strata [8], but shows a change towards the reconstitution of native grassland. The use of cultivated exotic species to regenerate native plant communities is considered an alternative to conservation without abandoning the productive use of intervened systems [32]. In seeking to rehabilitate ecosystems that were cleared for extensive dryland agriculture and subsequently abandoned due to loss of profitability, the implantation of Thinopyrum ponticum increases soil cover and restores productivity to previously degraded spaces.
The regeneration of natural grassland through perennialization with Thinopyrum ponticum could rehabilitate some functions of natural grassland, such as soil cover and net primary productivity of the system. These results contradict our second hypothesis because richness and diversity show no significant differences among implanting ages. However, they are higher compared to the initial monoculture of Thinopyrum ponticum and previously bare soil. By reducing the proportion of bare soil, erosion and soil, degradation could be avoided, thereby increasing the provision of ecosystem services. However, the results indicate that, within the studied timeframe, only the cover of grass and herb species were recovered, not the woody layer. Deliberate tree and shrub reintroduction efforts would be necessary to recover these layers.
The results of this study allow us to conclude that some native species in this semi-arid environment have the capacity to re-establish themselves after surface soil disturbance and planting of exotic species. Overgrazing leads to the degradation of these environments, resulting in low-diversity stable states, and applying a disturbance that initiates secondary succession processes could be an alternative for ecological restoration of these systems. In this case, the introduction of a perennial pasture such as Thinopyrum ponticum resulted in successions with high diversity and native cover within a period of 13 to 15 years. It is necessary to continue succession studies under different pasture management practices and evaluate environmental factors and interventions that promote the establishment of the shrub layer if restoration of woody vegetation is desired, which could provide environmental goods and services and specific values for conservation.
In conclusion, the rehabilitation of native perennial forage species cover is feasible through Thinopyrum ponticum implantation. In the future, it would be important to assess the provision of other ecosystem services (water infiltration and retention, soil fertility, etc.) that could be influenced by the perennialization of plots with native species.
Acknowledgement: We thank ranch owners for allowing us to carry out our field research on their lands.
Funding Statement: This work was supported by the National University of Río Negro (PI UNRN 40-C-873 GP, DAS and PI UNRN 40-C-1088 JMZ, GP, DAS).
Author Contributions: The authors confirm contribution to the paper as follows: study conception and design: Juan Manuel Zeberio, Guadalupe Peter; data collection: Juan Manuel Zeberio, Guadalupe Peter, Delfina Arancio Sidoti; analysis and interpretation of results: Juan Manuel Zeberio, Guadalupe Peter, Delfina Arancio Sidoti; draft manuscript preparation: Juan Manuel Zeberio, Guadalupe Peter, Delfina Arancio Sidoti. All authors reviewed the results and approved the final version of the manuscript.
Availability of Data and Materials: The data used for this study will be available via the CONICET repository.
Ethics Approval: Not applicable.
Conflicts of Interest: The authors declare that they have no conflicts of interest to report regarding the present study.
References
1. Millennium Ecosystem Assessment. Ecosystems and human well-being: desertification synthesis. USA: World Resources Institute; 2005. [Google Scholar]
2. Middleton NJ, Thomas DSG. World atlas of desertification. Edward Arnold, New York, NY, USA: U.N. Environment Programe; 1997. [Google Scholar]
3. López DR, Brizuela MA, Willems P, Aguiar MA, Siffredi G, Brand D. Linking ecosystem resistance, resilience, and stability in steppes of North Patagonia. Ecol Indic. 2013;24:1–11. doi:10.1016/j.ecolind.2012.05.014. [Google Scholar] [CrossRef]
4. Peláez DV, Blazquez FR, Tizón R. Considerations for the management and restoration of natural grasslands. Project BIRF TF 015041. The World Bank, Adaptation Fund, OPDS y Secretariat of Environment and Sustainable Development (Presidency of the Nation); 2019. p. 1–20. [Google Scholar]
5. Abraham ME, Corso ML, Maccagno P. Tierras secas y desertificación en Argentina. In: Evaluación de La Desertificación en Argentina. Argentina: Resultados Del Proyecto LADA/FAO; 2011. p. 11–64 (In Spanish). [Google Scholar]
6. Zeberio JM, Torres Robles SS, Calabrese GM. Uso del suelo y estado de conservación de la vegetación leñosa en el noreste patagónico. Ecol Austral. 2018;28(4):543–52 (In Spanish). [Google Scholar]
7. Gabella JI. Gestión territorial y degradación ambiental en áreas rurales de la diagonal árida templada argentina: partido de Patagones, provincia de Buenos Aires (Doctoral Thesis). Universidad Nacional del Sur: Argentina; 2014 (In Spanish). [Google Scholar]
8. Oyarzabal M, Clavijo L, Oakley F, Biganzoli P, Tognetti I, Barberis, et al. Unidades de vegetación de la Argentina. Ecol Austral. 2018;28:40–63 (In Spanish). [Google Scholar]
9. Zeberio JM, Pérez CA. Rehabilitación ecológica en el noreste patagónico: supervivencia y reclutamiento de especies nativas en suelos con diferentes texturas. Ecol Austral. 2021;31(1):491–504 (In Spanish). [Google Scholar]
10. Rodriguez LB, Torres Robles SS, Arturi MF, Grand ACH, Gasparri N, Biganzoli P. Plant cover as an estimator of above-ground biomass in semi-arid woody vegetation in Northeast Patagonia, Argentina. J Arid Land. 2021;13:918–33. doi:10.1007/s40333-021-0083-4. [Google Scholar] [CrossRef]
11. Gabella J, Campo A, Sili M. Gestión territorial y deterioro ambiental en el partido de Patagones, provincia de Buenos Aires. In: VIII Jornadas de Investigación y Debate. Buenos Aires: Memoria y oportunidades en el agro argentino: burocracia, tecnología y medio ambiente (1930–2010); 2011. [Google Scholar]
12. Funk FA, Torres Robles SS. Responses of vegetation to different land-use histories involving grazing and fire in the North-east Patagonian Monte, Argentina. Rangel J. 2013;35:273–83. doi:10.1071/RJ12093. [Google Scholar] [CrossRef]
13. Kröpfl A, Deregibus VA, Cecchi GA. Disturbios en una estepa arbustiva del Monte: cambios en la vegetación. Ecol Austral. 2007;17:257–68 (In Spanish). [Google Scholar]
14. Kröpfl A, Deregibus V, Cecchi G. A state and transition model for the Eastern Monte Phytogeographycal Province in Rio Negro. Phyton-Int J Exp Bot. 2015;84(2):390–6. doi:10.32604/phyton.2015.84.390. [Google Scholar] [CrossRef]
15. Zeberio JM, Pérez CA. Rehabilitation of degraded areas in northeastern Patagonia, Argentina: effects of environmental conditions and plant functional traits on performance of native woody species. J Arid Land. 2020;1(2):186–99. [Google Scholar]
16. Hobbs RJ, Cramer VA. Restoration ecology: interventionist approaches for restoring and maintaining ecosystem function in the face of rapid environmental change. Annu Rev Environ Resour. 2008;33:39–61. [Google Scholar]
17. Ochoa MA. Producción de forraje en suelos salinos. In: EEA INTA Rama Caída, Mendoza. 1994. Villa Mercedes: V Reunión de la Red Argentina de Salinidad: salinidad un desafío para el Semiárid; 2017 (In Spanish). [Google Scholar]
18. Karadağ Y, Tufan Y, Kurt AN. Tall wheatgrass (Thinopyrum ponticum (Podp.) Barkworth & D. R Dewey). In: Karadağ Y, Seydosoglu S, editors. Grasses. Ankara: Iksad; 2022. [Google Scholar]
19. Falasca SL, Miranda C, Pitta Alvarez S. Agro-ecological zoning for tall wheatgrass (Thinopyrum ponticum) as a potential energy and forage crop in salt-affected and dry lands of Argentina. Arch Crop Sci. 2017;1(1):10–9. [Google Scholar]
20. Oliva G, dos Santos E, Osiris S, Umaña F, Massara V, García Martinez G, et al. The MARAS dataset, vegetation and soil characteristics of drylands rangelands across Argentina. Sci Data. 2020;7:320. [Google Scholar]
21. Peri PL, Rosas YM, Lopez DR, Lencinas MV, Cavallero L, Martinez Pastur G. Conceptuel framework to define management strategies for sylvopastoral systems in native forest. Ecol Austral. 2022;32:749–66. doi:10.25260/EA.22.32.2.1.1872. [Google Scholar] [CrossRef]
22. Di Rienzo J, Casanoves F, Balzarini M, Gonzalez L, Tablada E, Robledo C. Grupo InfoStat, FCA. Universidad Nacional de Córdoba: Argentina; 2020. [Google Scholar]
23. Ferrante D, Álvarez Bento J, Vivar Miranda ME, Oliva G, Utrilla VR. Restauración por recolonización de especies nativas en pasturas sembradas en ambientes semiáridos en Patagonia. Semiárida. 2023;33(1):17–27 (In Spanish). [Google Scholar]
24. Vitousek P. Biological invasions and ecosystem processes: towards an integration of population biology and ecosystem studies. Oikos. 1990;57:7–13. doi:10.2307/3565731. [Google Scholar] [CrossRef]
25. Ding JQ. The mechanism of biological invasion and its effect on ecosystem safety. Rev Chin Agric Sci Technol. 2002;4:16–20 (In Chinese). [Google Scholar]
26. Bonet A. Secondary succession of semi-arid mediterranean old-fields in south-eastern Spain: insights for conservation and restoration of degraded lands. J Arid Environ. 2004;56(2):213–33. doi:10.1016/S0140-1963(03)00048-X. [Google Scholar] [CrossRef]
27. Castellanos AE, Martínez MJ, Llano JM, Halvorson WL, Espiricueta M, Espeje I. Successional trends in Sonoran Desert abandoned agricultural fields in northern Mexico. J Arid Environ. 2005;60(3):437–55. doi:10.1016/j.jaridenv.2004.06.004. [Google Scholar] [CrossRef]
28. Wong NK, Morgan JW, Dorrough J. A conceptual model of plant community changes following cessation of cultivation in semiarid grassland. Appl Veg Sci. 2010;13(4):389–402. doi:10.1111/j.1654-109X.2010.01080.x. [Google Scholar] [CrossRef]
29. Scott AJ, Morgan JW. Recovery of soil and vegetation in semi-arid Australian old fields. J Arid Environ. 2012;76:61–71. doi:10.1016/j.jaridenv.2011.08.014. [Google Scholar] [CrossRef]
30. González G, Luna MA, Hernández H. Wheatgrass in patagones. In: Qualitative and quantitative evaluation in four sites. Buenos Aires: Ediciones INTA; 2022. Technical Report No. 77. [Google Scholar]
31. Miñón DP, Silva MA, Collabelli MR, Gonzalez G, Enrique M, Viretto PE. Applied technology for the establishment and management of wheatgrass (Thinopyrum ponticum) pastures in rainfed environments of northeastern Patagonia.. Buenos Aires: Ediciones INTA; 2015. Technical Report No. 38. [Google Scholar]
32. Rey Benayas JM, Bullock JM, Newton AC. Creating woodland islets to reconcile ecological restoration, conservation, and agricultural land use. Front Ecol Environ. 2008;6:329–36. doi:10.1890/070057. [Google Scholar] [CrossRef]
Cite This Article
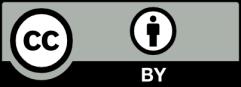
This work is licensed under a Creative Commons Attribution 4.0 International License , which permits unrestricted use, distribution, and reproduction in any medium, provided the original work is properly cited.