Open Access
ARTICLE
The Effect of Water and Salt Stress on Paspalum dilatatum, a Constituent of Pampas Natural Grasslands
1 Departamento de Química, Facultad de Agronomía, Universidad de Buenos Aires, Buenos Aires, C1417DSE, Argentina
2 Instituto de Investigaciones en Biociencias Agricolas y Ambientales (INBA), Facultad de Agronomía, Universidad de Buenos Aires, Buenos Aires, C1417DSE, Argentina
* Corresponding Author: Raul S. Lavado. Email:
(This article belongs to the Special Issue: Ecology of Rangelands in Argentina)
Phyton-International Journal of Experimental Botany 2024, 93(8), 2009-2018. https://doi.org/10.32604/phyton.2024.052874
Received 18 April 2024; Accepted 15 July 2024; Issue published 30 August 2024
Abstract
The effects of the salt stress on plant growth are usually increased by the water stress. We studied the impact of both stresses in simultaneous pulses of drought and salinity on Paspalum dilatatum. This forage species is native to South America, spread in grasslands in many tropical, subtropical, and temperate areas of the world, and very common in grasslands of the Flooding Pampas of Argentina. Mimicking what happens in nature. We compared a pot experiment, a non-stressed control against water stress for a month (midpoint between field capacity and wilting point), and two saline stresses (moderate, 6 d·Sm−1 and strong, 12 d·Sm−1), also for a month. Aerial biomass (green leaf; non-leaf green material, and dry material) and roots were harvested, weighed, and analyzed for nitrogen, phosphorus, and cations. The biomass of all components significantly decreased when both stresses were applied. Water plus strong saline stress reduced by half the total biomasses, compared to the control. The proportion of aerial biomass/root biomass ratio as well as aerial green component/dry materials ratio tend to decrease when subjected to both stresses. Nitrogen concentration in plants was not significantly affected, but phosphorus concentration increased in aerial biomass components, from 0.10 to 0.18 mg·kg−1 between the extreme treatments, but did not change in roots. Sodium concentration in plants increased (i.e., in green leave sodium (Na) increased from 0.27 to 2.01 mg·kg−1 between the extreme treatments), whereas other cations either did not change or decreased, affecting the ratios between them. Sodium performance allows us to infer that the Na accumulation of P. dilatatum behaves in an intermediate range, compared to very tolerant to salts or non-salt tolerant species of the Paspalum genus. In agreement, when salts were applied in the form of a pulse, P. dilatatum tolerated higher salinity than that found by other authors for the same species, using continuous salinity.Keywords
Nomenclature
AOAC | Association of Official Agricultural Chemists |
ANOVA | Analysis of Variance |
EC | Electrical Conductivity |
FAO | Food and Agriculture Organization |
FC | Field Capacity |
RWC | Relative Water Content |
SS | Salinity Stress |
WP | Permanent Wilting Point |
WS | Water Stress |
Paspalum dilatatum, known as Dallis grass, is a C4 forage grass native to South America. Although this species is spread all over the tropical, subtropical, and temperate areas of the world [1,2], it is a vigorous, persistent, and highly palatable species that withstands heavy grazing. This species is conspicuous or even co-dominant in most plant communities of the Flooding Pampas (Argentina). Different than other subregions of the Pampas, the Flooding Pampas remain covered mainly by temperate native grasslands, mainly due to the simultaneous occurrence of water and salt stress [3]. The area is characterized as a wet one, suffering commonly water logging conditions in winter and early spring, and even flooding, while soils show a low salt concentration. However, water and salt stresses are concurrent during most summers when drought occurs together with sudden increases in topsoil salinity. After late autumn rainfalls, water stress diminishes and salinity returns to its low current level, showing clearly the temporal variation of salinity in nature [3].
Water and salt stress impose an adverse impact on plant biomass production. They are usually related. Water stress has been identified as the single most biomass-reducing factor for the plants [4,5]. It is an almost universal plant stress, either permanent or transient, and periods of drought may occur frequently, even in regions characterized by high annual rainfall [4]. The present global warming situation increased drought occurrence around the globe [4]. Drought conditions affect the growth of plants by affecting photosynthetic and biochemical processes which result in a substantial decline in crop productivity [4,6]. Salt stress is usually localized from arid to humid areas, salt stress has a profound effect on both natural vegetation and crops, in certain conditions, salt stress may resemble water stress. Salts cause osmotic or water-deficit effects, in addition to salt-specific effects. The water deficit mediated by salts occurs because the presence of salts reduces the water uptake of plants which leads to a decrease in growth rate, accompanied by various metabolic changes similar to those induced by water stress [7]. The specific effects of salts are attributed to the intake of excess Na+ and Cl− ions [4,8]. Recent studies have revealed that the response of plants to a combination of water and salt stresses is unique and cannot be directly extrapolated from the response of plants to each of the different stresses applied individually [9,10].
Water and salt stresses can differentially affect the mineral nutrition of plants by reducing nutrient uptake by the roots and transport from the roots to the shoots, by restricting transpiration rates and impairing active transport, and membrane permeability [7,10]. Salinity stress also induces nutrient deficiency or causes nutrient imbalance due to the competition among them, such as potassium (K), calcium (Ca), and sodium (Na) [11]. Several studies have shown that P. dilatatum is tolerant of both floods and drought [12–14]. Cook et al. [15] indicated that this species is remarkably tolerant of drought because of its thick rhizomes. On the other hand, from the pioneering work of Gauch et al. [16] P. dilatatum is known as a species moderately resistant to salinity [17,18]; According to Lair [18], upper limits of this species survival is around 8–12 d·Sm−1. Other species of the genera, like Paspalum vaginatum Swartz, are highly tolerant to salts [19,20]. According to Chavarria et al. [21], Paspalum vaginatum reduces its performance by 62% on average, at Electrical conductivity (EC) as high as 45 d·Sm−1. It is remarkable that Paspalum vaginatum is also found in the Flooding Pampas grasslands, but is much less conspicuous than P. dilatatum [8]. While most studies were performed using a continuous salt supply, crops respond differently when coping with continuous salinity vs. peaks or pulses of salts [11].
Our objective was to determine the effects of drought and salinity on biomass production of the green and dry components and chemical composition of Paspalum dilatatum plantlets, mimicking the stress conditions in the form of pulses of water deficit and salt excess. We hypothesize that the simultaneous negative effect of water stress and salt stress on the biomass production of P. dilatatum increases with the magnitude of salt stress.
A pot experiment was carried out at a rainout shelter on the campus of the School of Agriculture, University of Buenos Aires, Buenos Aires, Argentina (34°36′S, 58°29′W). The pots were filled with 3 kg of the A horizon of a General Guido series Typic Natraquoll, collected near the town of Casalins (Buenos Aires Province). The soil is representative of the sodic soils widespread in the Flooding Pampas. The analysis of the A horizon samples was carried out using standard soil test methods. The analysis of the A horizon samples was carried out using standard soil test methods [22] for the physical and chemical properties: Particle size distribution (Pipette method) was 18.0% clay, 33.9% silt, and 48.1% sand, indicating a Loam texture; Water retention at Field Capacity (33 kPa) was 21.8% and at Permanent Wilting Point (1500 kPa) 11.0%; total carbon (Walkley and Black method) was 18.5 g·kg−1, pH was 7.0, available P (Kurtz and Bray method) was 12.5 mg·Kg−1 and EC was 0.5 d·Sm−1. Paspalum dilatatum plantlets were taken from the grassland community growing on that soil. The temperature in the shelter varied from a daily minimum of 13°C to 19°C to a maximum of 32°C to 42°C. Water was provided via irrigation.
We adopted a completely randomized design with 4 replicates of a factorial arrangement of two factors: two levels of water stress (WS) and three levels of salinity stress (SS). There were 6 treatments: Control, SS0/WS0 (natural soil with adequate water supply); Water stress, SS0/WS1; Moderately saline stress, SS1/WS0; water stress plus moderately saline stress SS1/WS1; Strong saline stress, SS2/WS0 and Water stress plus strong saline stress, SS2/WS1. Both saline and water stress were applied during the same time period. The water status was controlled by irrigation with distilled water, adding the water from the top. The pots of the Control (SS0/WS0) were maintained near field capacity during the course of the experiment, allowing the water to drain (Fig. A1). All pots have drainage holes in the bottom. The pots of the treatments with water stress were maintained equally around field capacity, from the start of the experiment on late spring. Subsequently, they were maintained in the middle point between field capacity and wilting point for a month (i.e., January) by weighing the pots on a daily basis and avoiding drainage. By early February the pots were irrigated again as indicated previously to reach field capacity. The salt stress treatments were irrigated initially with distilled water and in January both salt stress treatments were carried out by irrigation with saline solutions of two different EC: for the moderate salt level, 6 dSm1 and for the strong salt level, 12 dSm1. The saline stress treatments were obtained dissolving the quantity of sodium chloride in distilled water to obtain the desired EC. The salt concentration in the pots was maintained in all treatments allowing solution percolation, because in such a way the soil solution equals the percolating solution. For the treatments subjected simultaneously to both stresses, at that point, the addition of each salt solution was stopped and water was applied as indicated for water stress treatment, by weighing the pots. At the end of the thirty days period, distilled water was applied in excess to reduce salinity, making use of drainage holes in the bottom of the pots. The surplus of water (20% more than needed to maintain field capacity) flushed the salt out in few days, which was assured by checking the EC of percolates (data not shown). The pots were irrigated again to field capacity using distilled water, until the end of the experiment.
In March the experiment ended and the aerial biomass and roots were collected in all pots. The aerial biomass was divided into green leaves; non-leaf green material (shoots, inflorescences, etc.) and dry material (dry leaves, dry shoots, etc.). Roots were removed from the soil. All this material was dried at 70°C and weighed. The chemical determinations were carried out using standard techniques: nitrogen (N) (Kjeldhal method), phosphorus (P) (extracted by calcination and dissolution of the ashes, and determined by the Murphy and Riley colorimetric methodology), and Ca, Mg, K and Na digested in hot HClO4 and HNO3 and determined by atomic absorption spectrophotometer [22,23]. Previously, in January, when stress treatments were ending, Relative Water Content (RWC) was determined by oven drying at 80°C for 24 h samples of fresh, turgid green leaves [24].
The data of aerial biomass, root biomass N and P in aerial and root biomass and foliar Na, K, Ca and Mg were statistically analyzed using the RStudio software (version 1.1.453 for Windows RStudio Team 2015) for an ANOVA analysis, after testing the variables for normality and homogeneity of variance. When there was a significant effect of the treatment, contrasts (Student’s t-test) were used to compare the means.
The effects of the different treatments on total aerial biomass and its components and on root biomass are shown in Fig. 1 and in Table 1.
Figure 1: Biomass production of Paspalum dilatatum discriminated in green leaves, non-leaf green material (shoots, inflorescences, etc.), dry material (dry leaves, dry shoots, etc.) and roots, as influenced by the two factors studied (WS, SS). Bars represent standard deviation. Different letters mean significant differences
Fig. 1 shows that all plant components significantly decreased when subjected to both stresses while Table 2 shows the statistical significance of the found results. The extreme stress treatment (SS2/WS1) reduced to a half total biomass when compared to control. In all cases the whole aerial biomass was lower than the root biomass: the aerial biomass varied from 67% compared to root biomass in the check treatment to 37% in the treatment SS2/WS1.The proportion of dry rest to green components of the plants was also affected by the treatments. In all cases but in one, the dry materials were lower, from 18% to 83%, than the green components. The exception was treatment subjected to both water plus strong saline stress (SS2/WS1), in which the green components account 88% of the dry rest. Using the RWC as an indicator of water deficit, the significant effects of water and salt stress were verified on P. dilatatum (p < 0.001 and p < 0.02, respectively) (Fig. 2).
Figure 2: Influence of water and saline stress on relative water content in Paspalum dilatatum leaves, measured through the relative water content in green leaves. Different letters mean significant differences
Sodium, K, Ca and Mg concentration in plant components are shown in Table 2.
The N concentration in the different components of the plants showed no significant differences due to the imposed treatments. The green leaves and non-leaf green material contained to 0.85–1.39 mg N·kg−1, dry material to 0.21–0.60 mg N·kg−1 and roots to 0.81–1.10 mg N·kg−1. P concentration increased significantly in green leaves, non-leaf green material and dry material, as stress, especially salinity, increased. Conversely, P concentration in roots did not show significant differences in any of the treatments (Fig. 3).
Figure 3: Phosphorus concentration in green leaves, green rest (non-leaf green material—shoots, inflorescences, etc.), dry rest (dry material—dry leaves, dry shoots, etc.) and roots of Paspalum dilatatum, as influenced by the two factors studied (water stress and salinity stress). Bars represent standard deviation. Different letters mean significant differences
Potassium, Ca and Mg concentrations were not largely affected by the imposed treatments in green leaves, non-leaf green material and dry material, as compared with control (Table 2). Conversely, Na concentration increased significantly in these plant components as salinity stress increased. (Table 2). Consequently, the K/Na ratio in all aerial plant components (Fig. 4) as well as the Ca/Na (Fig. 5) and Mg/Na ratios (data not shown) decreased (p < 0.001). Sodium concentration did not increase in roots and as a consequent the Na/K and Ca/Mg ratios in roots were not affected by the treatments.
Figure 4: K/Na ratio in green leaves, green rest (non-leaf green material—shoots, inflorescences, etc.), dry rest (dry material—dry leaves, dry shoots, etc.) and roots of Paspalum dilatatum, as influenced by the two factors studied, water stress and salinity stress (WS, SS). Bars represent standard deviation. Different letters mean significant differences
Figure 5: Ca/Na ratio in green leaves, green rest (non-leaf green material—shoots, inflorescences, etc.), dry rest (dry material—dry leaves, dry shoots, etc.) and roots of Paspalum dilatatum, as influenced by the two factors studied, water stress and salinity (WS, SS). Bars represent standard deviation. Different letters mean significant differences
The effects of water stress on plants depend on its absolute value and temporal variation [25]. In present case plant components significantly decreased when subjected to both stresses, but different than reported [5] the whole aerial biomass was more affected than the root biomass.
In the present experiment the RWC measured at the end of the stress period corresponded to a level equivalent to initial wilting stage [19]. The RWC was more critical on salt stressed treatments, which agree with the fresh/dry weight ratio which diminishes under water limitation [19]. These results are similar to that found by Lee et al. [20] with different ecotypes of the salt tolerant Paspalum vaginatum, although when subjected to a salinity increase. results varied among ecotypes. The RWC decreased under water stress although somewhat less than with salt stress, in agreement with the moderately tolerance to drought of P. dilatatum [5,15]. Focusing on salt stress effects, the results show that P. dilatatum subjected to salt pulses, even including water stress, can tolerate more salinity than is shown by classical experiments using continuous salinity regime and without water stress [16–18]. Water stress is normally a coexisting factor with salt stress, however, the effect of the superposition of both on P. dilatatum cannot be quantified in this work. Anyway, different results show that due to plants subjected to pulses of salinity accumulate fewer ions in their tissues they can tolerate higher salinities than those subjected to continuous salinity [11].
Results showing that leaf N concentration did not vary due to the imposed treatments, but P concentration increased significantly confirm that the interactions between N and P nutrition and water and salt stress are complex. It depends on the magnitude of the stress and the stress tolerance of plants [26]. Yadav et al. [8] indicated that the water stress results in N absorption limitations. Salt stress (as well as other stresses) may reduce N accumulation [7,26] but in our experiment, the plant N concentrations were not affected by the treatments. The N concentration in green leaves was similar than that obtained previously in P. dilatatum from the Flooding Pampas by Ayala Torales et al. [27] and Vivanco et al. [28]. However, our foliar N concentration was lower than that found by Andrew et al. [29] in other latitudes and also lower than that recorded for Paspalum vaginatum [19]. The saline stress may decrease, increase or not affect P accumulation in plants [7,26]. In our case, the P concentration in plant tissues increased with the treatments but, this fact could be related to lower biomass production, as usually established: the lower the biomass, the higher the P concentration [30]. Phosphorus concentration in leaves was similar as those obtained by Vivanco et al. [28] and again lower than those found by Andrew et al. [29].
In general terms non-halophytic plants tend to absorb Na in excess in saline soils, causing a relative and even absolute decrease in K and Ca concentration. These processes are related to the K-Na antagonism [26]. The Ca/Na ratio, which is related to plant salt tolerance, is also affected [8,31]. In the present case, the Na concentration increased, except in the roots, while that of the rest of the cations was not substantially modified (Table 2), which caused the K/Na, Ca/Na and Mg/Na ratios to decrease in all components. aerials (Figs. 4 and 5). The highly salt tolerant Paspalum vaginatum can maintain a high concentration of K in its shoots and can limit the movement of Na toward shoots. Besides, this species accumulated more Na and Ca in its dead leaves than in the green leaves. The maintenance of a high K/Na ratio allow P. vaginatum to avoid the cation damage caused by salt stress [19], different than the non-salt tolerant P. scrobiculatum, which is unable to regulate the transfer of Na from the green leaves [32].
This suggested that one way by which P. vaginatum tolerates salinity may be by a mechanism of reducing Na transport to leaves and moving excess of cations from young leaves to older and possibly dying leaves [32]. In our experiments, the combined effects of water and salt stress on cationic concentrations was complex because there were large differences in its concentration between the different plant components. Most results in present experiment (Table 2) showed that P. dilatatum when subjected to salinity stress did not show the same behavior than the salt tolerant P. vaginatum, with which coexist in the grasslands. Different from that species, our results show that P. dilatatum does not accumulate Na and Ca in dead leaves.
We found that the representation of natural conditions in a controlled experiment provides some different results as compared with similar experiments but where is not considered the variability of salinity and the water stress. The comparison with other species of the same genus allowed us to advance with the way this species deals with salinity, but this is a further field to study. From a more applied point of view, the present data contribute to better knowledge of the species for a more rational management of natural grasslands.
P. dilatatum biomass production in the different plant components, green leaves, non-leaf green material, dry material, and roots, decreased when subjected to individual stress and decreased even more when both stresses were applied simultaneously. However, under the conditions of the present experiment, the hypothesis was not validated: the lower and the higher saline stress affected biomass in a globally similar way when subjected to simultaneous water stress. When salts were applied in the form of a pulse, mimicking the nature, P. dilatatum tolerated higher salinity than that found by other authors using non-realistic permanent salt levels. Besides this fact, the plant chemical composition related to the accumulation of cations in plant tissues allows us to infer that the Na accumulation of P. dilatatum behaves in an intermediate range, compared to salt-tolerant or non-salt tolerant species of the Paspalum genus.
Acknowledgement: The authors appreciate the contribution of the technical staff of the Soil Science Department.
Funding Statement: The authors received no specific funding for this study.
Author Contributions: The authors confirm contribution to the paper as follows: study conception and design: Gerardo Rubio and Raul S. Lavado; data collection: Claudia A. Porcelli and Flavio H. Gutiérrez Boem; analysis and interpretation of results: Claudia A. Porcelli, Gerardo Rubio, Raul S. Lavado and Flavio H. Gutiérrez Boem; draft manuscript preparation: Gerardo Rubio and Raul S. Lavado. All authors reviewed the results and approved the final version of the manuscript.
Availability of Data and Materials: Data are available on request from Raul S. Lavado.
Ethics Approval: Not applicable.
Conflicts of Interest: The authors declare that they have no conflicts of interest to report regarding the present study.
References
1. Burson BL, Watson VH. Bahiagrass, dallisgrass and other Paspalum species. In: Barnes RF, editor. Forages: an introduction to grassland agriculture. Ames, IA: Iowa State University Press; 1995. p. 431–40. [Google Scholar]
2. Loreti J, Oesterheld M. Intraspecific variation in the resistance to flooding and drought in populations of Paspalum dilatatum from different topographic positions. Oecologia. 1996;108:279–84. [Google Scholar] [PubMed]
3. Imbellone PA, Taboada MA, Damiano F, Lavado RS. Genesis, properties and management of salt-affected soils in the Flooding Pampas, Argentina. In: Taleisnik E, Lavado RS, editors. Saline and alkaline soils in latin america: natural resources, management and productive alternatives. Cham, Suizerland: Springer Nature; 2020. p. 191–208. [Google Scholar]
4. Iqbal MS, Singh AK, Ansari MI. Effect of drought stress on crop production. In: Amitava R, Sing HB, Singh AK, Singh US, Fraceto L, editors. New frontiers in stress management for durable agriculture. Singapore: Springer Nature; 2020. p. 35–48. [Google Scholar]
5. Rubio G, Pereyra F, Taboada M. Soils of the Pampean region. In: Rubio G, Lavado RS, Pereyra FX, editors. The soils of Argentina. Cham, Switzerland: Springer; 2019. p. 81–100. [Google Scholar]
6. Gupta R, Bano A, Rai S, Mishra R, Singh M, Sharma S, et al. Mechanistic insights of plant-microbe interaction towards drought and salinity stress in plants for enhancing the agriculture productivity. Plant Stress. 2022;4:100073. doi:10.1016/j.stress.2022.100073. [Google Scholar] [CrossRef]
7. Munns R. Comparative physiology of salt and water stress. Plant Cell Environ. 2002;25:239–50. [Google Scholar] [PubMed]
8. Yadav S, Atri N. Impact of salinity stress in crop plants and mitigation strategies. In: Amitava R, Sing HB, Singh AK, Singh US, Fraceto L, editors. New frontiers in stress management for durable agriculture. Singapore: Springer Nature; 2020. p. 49–64. [Google Scholar]
9. Mittlera R. Abiotic stress, the field environment and stress combination. Trends Plant Sci. 2006;11:15–9. [Google Scholar] [PubMed]
10. Hu Y, Schmidhalter U. Drought and salinity: a comparison of their effects on mineral nutrition of plants. J Plant Nutrition Soil Sci. 2005;168:541–9. [Google Scholar]
11. Bustingorri C, Lavado RS. Soybean growth under stable salinity versus peak salinity. Sci Agricola. 2011;68:102–8. [Google Scholar]
12. FAO. Grassland Index. A searchable catalogue of grass and forage legumes. Rome: FAO; 2010. [Google Scholar]
13. Insausti P, Grimoldi A, Chaneton EJ, Vasellati V. Flooding induces a suite of adaptive plastic responses in the grass Paspalum dilatatum. New Phytol. 2001;152:291–9. [Google Scholar]
14. Di Bella CE, Grimoldi AA, Striker GG. A quantitative revision of the waterlogging tolerance of perennial forage grasses. Crop Pasture Sci. 2022;73(10):1200–12. doi:10.1071/CP21707. [Google Scholar] [CrossRef]
15. Cook BG, Pengelly BC, Brown SD, Donnelly JL, Eagles DA, Franco MA, et al. Tropical forages. Brisbane, Australia: CSIRO, DPI&F(QldCIAT and ILRI; 2005. [Google Scholar]
16. Gauch HG, Wadleigh CH. Salt tolerance and chemical composition of Rhodes and Dallis grasses grown in sand culture. Bot Gaz. 1951;112(3):259–71. [Google Scholar]
17. Hu Y, Schmidhalter U. Opportunity and changes of phenotyping plant salt tolerance. Trends Plant Sci. 2023;28(5doi:10.1016/j.tplants.2022.12.010. [Google Scholar] [PubMed] [CrossRef]
18. Lair K. Salt tolerance value ranges for selected/example western reclamation and forage species. Available from: https://www.calflora.org/nrcs/help/SelectedSaltToleranceReferences.pdf. [Accessed 2024]. [Google Scholar]
19. Jiang K, Yang Z, Sun J, Liu H, Chen S, Zhao Y, et al. Evaluation of the tolerance and forage quality of different ecotypes of seashore Paspalum. Front Plant Sci. 2022;13:944894. doi:10.3389/fpls.2022.944894. [Google Scholar] [PubMed] [CrossRef]
20. Lee G, Carrow RN, Duncan RR. Growth and water relation responses to salinity stress in halophytic seashore Paspalum ecotypes. Sci Horticul. 2005;104:221–36. [Google Scholar]
21. Chavarria M, Wherley B, Thomas J, Chandra A, Raymer P. Salinity tolerance and recovery attributes of warm-season turfgrass cultivars. HortScience. 2019;54(9):1625–31. [Google Scholar]
22. Sarkar D, Haldar A. Physical and chemical methods in soil analysis. New Delhi: New Age International Publishers; 2023. [Google Scholar]
23. AOAC. Official methods of analysis of AOAC. Washington, DC, USA: AOAC; 2016. vol. 16. [Google Scholar]
24. Mullan D, Pietragalla J. Leaf relative water content. In: Pask A, Pietragalla J, Mullan D, Reynolds M, editors. Wheat: physiological breeding II: a field guide to wheat phenotyping. Mexico: CIMMYT; 2012. p. 25–35. [Google Scholar]
25. Panda R, Behera SK, Kashyap PS. Effective management of irrigation water for maize under stressed conditions. Agri Water Manage. 2004;66:181–203. [Google Scholar]
26. Grattan SR, Grieve CM. Mineral nutrient acquisition and response by plants under saline environments. In: Pessarakli M, editor. Handbook of plant and crop stress. New York: M. Dekker; 1993. p. 203–26. [Google Scholar]
27. Ayala Torales AT, Acosta GI, Deregibus VA, Moauro PM. Effects of grazing frequency on the production, nutritive value, herbage utilisation, and structure of a Paspalum dilatatum sward. New Zealand J Agri Res. 2000;43:467–72. [Google Scholar]
28. Vivanco L, Austin AT. Intrinsic effects of species on leaf litter and root decomposition: a comparison of temperate grasses from North and South America. Oecologia. 2006;150:97–107. [Google Scholar] [PubMed]
29. Andrew CS, Robins MF. The effect of phosphorus on the growth, chemical composition, and critical phosphorus percentages of some tropical pasture grasses. Aust J Agri Res. 1971;22:693–706. [Google Scholar]
30. Marschner H. Mineral nutrition of higher plants. London, UK: Academic Press; 1986. [Google Scholar]
31. Wakeel A, Farooq M, Qadir M, Schubert S. Potassium substitution by sodium in plants. Critical Rev Plant Sci. 2011;30(4):401–13. doi:10.1080/07352689.2011.587728. [Google Scholar] [CrossRef]
32. Shonubi OO. Possible roles of the leaves of two Paspalum species in salinity tolerance. Asian J Plant Sci. 2010;9:94–8. [Google Scholar]
Appendix A
Figure A1: Treatment application scheme
Cite This Article
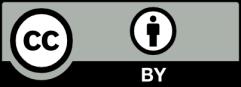
This work is licensed under a Creative Commons Attribution 4.0 International License , which permits unrestricted use, distribution, and reproduction in any medium, provided the original work is properly cited.