Open Access
ARTICLE
Seed Priming with Potassium Nitrate Can Enhance Salt Stress Tolerance in Maize
1 Institute of Botany, University of the Punjab, Lahore, 54590, Pakistan
2 Department of Biology, College of Science, Taif University, Taif, 21944, Saudi Arabia
3 Department of Botany, Emerson University, Multan, 60000, Pakistan
4 Biological Sciences Department, Faculty of Science and Arts, King Abdulaziz University, Rabigh, 21911, Saudi Arabia
5 Department of Biology, College of Science, Qassim University, Burydah, 52571, Saudi Arabia
6 Department of Chemistry Sciences, The University of Lahore, Lahore, 54590, Pakistan
7 Department of Environmental Sciences, The University of Lahore, Lahore, 54590, Pakistan
* Corresponding Author: Qamar uz Zaman. Email:
(This article belongs to the Special Issue: Abiotic Stress Impacts on Plant Physiology and Their Alleviation)
Phyton-International Journal of Experimental Botany 2024, 93(8), 1819-1838. https://doi.org/10.32604/phyton.2024.048780
Received 18 December 2023; Accepted 20 June 2024; Issue published 30 August 2024
Abstract
Salinity is a major abiotic stress that hinders plant development and productivity and influences agricultural yield. Seed priming is a technique used to boost germination and seedling growth under abiotic stress. A pot experiment was conducted to evaluate the impact of seed priming with potassium nitrate (KNO3) at various levels (0%, 0.50%, 1.00% and 1.50%) under salt stress (0, 75, 100 mM NaCl) on two maize verities (MNH360 and 30T60) for the growth, development and metabolic attributes results revealed that in maize variety MNH360, KNO3 priming’s significantly enhanced growth parameters than in maize variety 30T60 under control and salt-stressed conditions. Priming with KNO3 enhanced carotenoids and total chlorophyll in the 30T60 variety that protected the maize plants from salt stress. Nevertheless, it was shown that priming with 1.00% KNO3 acts as safeguarded to protect them from oxidative damage by salt stress minimizing reactive oxygen species (ROS) formation through increased levels of malondialdehyde (MDA), catalase (CAT), peroxidase (POD), ascorbate peroxidase (APX), and total soluble protein. The findings of the present study confirm that the use of the KNO3 seed priming technique is a low-cost, environmentally friendly technique for mitigating adverse impacts of salt stress in maize crops by activating the antioxidant defense system and improving chlorophyll and osmolyte contents.Keywords
Abiotic stress directly affects seed germination and crop establishment. Poor-vigor seeds produced in unfavorable settings thus tend to grow more slowly and with less speed. The degradation of soil is significantly influenced by soil salinization. Similar to other abiotic stresses, salt stress increases reactive oxygen species (ROS), including superoxide (O2), hydrogen peroxide (H2O2), and hydroxyl radicals (OH), which in turn promotes oxidative stress [1,2]. These ROS are exceedingly reactive and can alter the normal cellular uptake of oxidative damage to lipids, proteins, and nucleic acids [3]. Plants have developed a variety of antioxidative defense mechanisms, including superoxide dismutase (SOD), ascorbate peroxidase (APX), catalase (CAT), guaiacol peroxidase (GPX), and glutathione reductase (GR), to lessen the oxidative damage brought on by ROS [4]. SOD is the chief O2− scavenger and its enzymatic action results in H2O2 and O2 formation. The H2O2 produced is then scavenged by CAT and several classes of peroxidases. Catalase, which is established in peroxisomes, cytosol, and mitochondria, converts hydrogen peroxide (H2O2) into water (H2O) and O2 [5,6]. Maize is a frequently used cereal crop and it is cultivated in temperate zones and subtropical areas. It was stated that maize is a variable-stress tolerant plant species under extremes of the environment. Maize is the third vital cereal crop in Pakistan following wheat and rice [7]. The salinity causes significant reductions in photosynthesis [8,9]. When exposed to various stresses, soluble carbohydrates, proteins, and free amino acids are anticipated to operate as active solute components, and their accumulation is thought to act as an effective stress tolerance mechanism. The addition of these osmotic solutes facilitated the plant species’ ability to adjust to increasing salt applications in soil by reducing tissue osmotic potential [10,11].
Seed priming has long been proven as an efficient method for ensuring that plants grow uniformly [12]. Seed priming is a method of optimizing seed treatments by enhancing seed vigor and growth rates [13,14]. Mostly the priming techniques involve the pre-treatment of seeds with chemical solutions or physical methods to enhance their germination, vigor, and overall performance [15]. Chemical priming typically involves the application of solutions containing osmotic agents, which initiate osmotic stress and activate defense mechanisms within the seed [16]. The physical priming methods include techniques like hydropriming and osmopriming, where seeds are soaked in water or osmotic solutions, respectively, to initiate metabolic processes without causing irreversible damage [17]. These priming techniques exploit seed physiological responses to stress and hydration, ultimately enhancing seedling establishment, uniformity, and crop performance [18]. Plants require several macronutrients and micronutrients from seed germination to seed production [14]. Along with major macronutrients, potassium is one of the most important macronutrients. It is required for a variety of biochemical and physiological processes involved in plant development and growth [19]. Protein synthesis, enzyme activation, and glucose metabolism are the major key processes in plant growth that require potassium. It also has a protective role against abiotic conditions like drought, salinity, hot or cold temperatures, metal toxicity, and so on [20]. Yellowing of the leaf margins and slowed development are symptoms of K deficiency in plants. Furthermore, K insufficiency is linked to a weak root system, yield losses, and lodging [21]. During germination, seeds rely on stored nutrients, including nitrogenous compounds like proteins and amino acids, to fuel early growth stages. As germination initiates, enzymes mobilize these stored reserves, breaking down complex nitrogen-containing molecules into simpler forms that can be readily utilized by emerging seedlings [22]. Nitrogen is particularly vital for the synthesis of enzymes involved in metabolic processes, such as respiration and photosynthesis, which are essential for energy production and nutrient assimilation. Additionally, nitrogen regulates the expression of genes involved in germination, coordinating various physiological and biochemical pathways necessary for seedling establishment [23]. The use of KNO3 to prime maize seedlings resulted in improved growth and germination [24]. Seed priming has been shown to improve seedling development and growth, as well as germination percentage [25]. Seed priming has been demonstrated to promote seed germination and emergence in vegetables and tiny-seeded grasses [26]. Many field crops, including wheat, sugar beet, maize, zucchini, and sunflower, have been shown to benefit from priming [14,27,28]. Basra et al. [24] explored that the priming technique increases growth attributes significantly in various crops. Seed priming with chemical solutions such as urea and KNO3 was employed by [29] to examine the protein and protein content, seedling development responses, and germination of maize hybrids under salt and drought stress [29,30].
This study examines the interaction between potassium nitrate and salt stress tested into two maize varieties, as well as its potential to offer novel insights into enhancing the resilience and productivity of maize crops in stressed environments. The main objective of this study was to examine the potential of KNO3 as a seed priming agent at various levels for the mitigation of salt stress in maize verities throughout its growth period and to investigate the beneficial effects of KNO3 at various levels on the growth, physiology, and antioxidant system of maize verities grown under salt contaminated soil.
The experiment was arranged under a completely randomized design with a factorial arrangement replicated four times. The experimental treatments were comprised of two factors, i.e., various levels of salt stress (0, 75, and 100 mM NaCl) and different levels of KNO3 (0%, 0.50%, 1.00%, and 1.50%) as priming solutions on two maize varieties (MNH360 and 30T60) under greenhouse conditions.
Two varieties of maize seeds MNH360 and 30T60 were collected from Punjab Seed Corporation, Lahore, Pakistan. MNH360 is an Indian variety of maize while 30T60 is from Sahiwal, Punjab Pakistan. Seeds of two varieties were disinfected with sodium hypochlorite (NaOCl) for fifteen minutes followed by washing with distilled water three times. Seeds of both varieties were soaked with four different concentrations (0%, 0.50%, 1.00%, and 1.50%) of potassium nitrate solution (KNO3) of 100 mL for 24 h. After priming, the seeds were washed with distilled water and rehydrated at room temperature for twenty hours. The seeds which were soaked in the distilled water were considered as control seeds.
2.3 Experimental Setup and Growth Conditions
After the process of seed priming about 100 seeds of both varieties were packed in zipper bags labeled with different concentrations of priming solutions. Following, four replicates of twelve treatments with 96 pots (diameter 9 cm), were arranged by using Design Expert Software 11 (C0 = Control, S1 = 75 mM NaCl, S2 = 100 mM NaCl, S0P1 = 0 mM NaCl and 0.50% priming solution, S1P1 = 75 mM NaCl and 0.50% priming solution, S2P1 = 100 mM NaCl and 0.50% priming solution, S0P2 = 0 mM NaCl and 1.00% priming solution, S1P2 = 75 mM NaCl and 1.00% priming solution, S2P2 = 100 mM NaCl and 1.00% priming solution, S0P3 = 0 mM NaCl and 1.50% priming solution, S1P3 = 75 mM NaCl and 1.50% priming solution, S2P3 = 100 mM NaCl and 1.50% priming solution). The experiment was conducted in the Botanical Garden, University of the Punjab, Lahore, Pakistan carried out in the period between September and November with a temperature around 28°C ± 2°C. Before sowing, a detailed analysis of soil physicochemical properties (1 kg−1 pot) was evaluated. The soil used for this study was loam textured with EC (1.9 mScm−1), pH = 8, 0.87% organic matter, 2.5 mg kg−1 of P, 129 mg kg−1 of K. After thirty days of seed sowing, the salt stress of using the sodium chloride (NaCl) was applied with a level of 75 and 100 mM, Salt stress was applied four times after a one-week interval to avoid the salt injury. After 75 days of sowing the experiment was harvested. Seedlings were carefully uprooted for growth attributes and were preserved by using liquid nitrogen and stored at −80°C for biochemical analysis.
2.4 Determination of Growth Attributes
The root and shoot parts of both varieties of maize plants were separated. The growth attributes including plant length (cm), number of leaves, leaf width (cm), and leaf length (cm) were measured using the measuring tape. The leaf area (cm2) was measured by using the following formula:
Leaf Area = Leaf Length × Leaf Width × Correction Factor (0.75) [31]
2.5 Determination of Chlorophyll Contents
To estimate chlorophyll contents, the 0.1 g of crushed fresh leaves were dipped in 10 ml DMSO (dimethyl sulphoxide, (CH3)2SO) in falcon tubes for 48 h, in the dark. The extracts were tested for absorbance at 645 and 663 nm using a UV 1800 spectrophotometer. The amount of chlorophyll in the sample was calculated using Arnon’s formula [32], which is as follows:
2.6 Determination of Carotenoids
In falcon tubes, 0.1 g of fresh leaves was dissolved in 10 mL DMSO. For 48 h, the falcon tubes were kept in the dark. The absorbance at 480 nm of all extracts was measured using a UV 1800 spectrophotometer. The carotenoids were calculated using Wellburn’s formula [33], which is as follows:
2.7 Assessment of Total Soluble Protein Contents
In test tubes, 100 µL of extraction solution was mixed with 3 mL of color reagent (Coomassie Brilliant Blue G-250) to determine the total soluble protein. The reaction mixture was vortexed vigorously for one minute before incubation for five minutes. After 5 min, the absorbance of the solution was measured at 595 nm with a UV spectrophotometer (Shimadzu UV-1800). Using the protein standard curve, the total protein contents of the samples were determined.
2.8 Assessment of Ascorbate Peroxidase (APX) Activity
The oxidation of ascorbate was used to measure the activity of ascorbate peroxidase using the Nakano et al. technique [34,35]. About 100 mM of sodium acetate buffer (pH 5.8), 100 µL extraction solution, 3 µM ethylenediaminetetraacetic acid (EDTA), and 5 mM H2O2 were included in the reaction solution (3 mL) were taken. Using a UV spectrophotometer (Shimadzu UV 1800 Spectrophotometer), the variations in absorbance at 290 nm were measured after every 10 s for 60–80 s. The change in the absorbance of 0.01 per minute was defined as one unit of APX activity.
2.9 Assessment of Malondialdehyde (MDA) Contents
Malondialdehyde was determined according to the procedures described by Dhindsa et al. [36] with little modifications. Briefly, 1.0 mL of enzyme solution and 2.0 mL of reaction solution containing 20% (w/v) trichloroacetic acid (TCA) and 0.5% (w/v) thiobarbituric acid (TBA) were mixed. The solution was placed in a water bath at 95°C for 30 min. The solution was centrifuged at 12,000 rpm for 10 min. For the blank sample, about 1 mL buffer and 2 mL TBA solution were added. The absorbance of supernatant was measured at 532 and 600 nm. For the final value, the absorbance at 600 nm was subtracted from the 532 nm. The absorbance and the extinction coefficient of 155 mm−1 cm−1 were used to determine the amount of MDA present in the sample.
2.10 Determination of Catalase (CAT) Activity
Catalase activity was determined by using the method of Chance et al. [37] which was based on the oxidation of H2O2. The reaction solution (3 mL) contained H2O2, phosphate buffer (pH 7.0), and 100 µL of extracted solution. Using a Shimadzu UV 1800 Spectrophotometer, the variations in absorbance at 240 nm were measured every 10 s for 60–80 s, with each reading lasting 60–80 s. One unit of CAT activity was defined as a change in absorbance of 0.01 per minute in absorbance.
2.11 Evaluation of Guaiacol Peroxidase (POD) Activity
The guaiacol peroxidase (POD) activity was determined by using the method of Chance et al. [37] which is based on the oxidation of H2O2. The reaction solution (3 mL) contained Guaiacol 1 mL, 1.85 mL HAC-NaAC buffer (pH 5.0), enzyme extraction 50 µL and 0.75% H2O2 100 µL. Changes in absorbance at 460 nm were taken every 10 s for 60–80 s using a Shimadzu UV 1800 Spectrophotometer. One unit of POD activity was defined as the absorbance change of 0.01 per min.
SPSS Ver. 20 software was used to conduct the two-way-ANOVA. Data are four replications’ means ± standard error were used to express the mean values. Duncan’s multiple range test at p < 0.05 was used to statistically analyze differences across treatment means. Statistical analysis of data was performed by applying Fisher’s ANOVA and means of all treatments were compared by the highest significant differences (HSD) test using Statistix 8.1 software. To assess relationships between the studied variables, Pearson’s correlation analysis was used. The R studio software was used for the principal component analysis (PCA) and Pearson correlation coefficients among the observed variables.
From the statistical analysis, the interactions between leaf length, leaf width, leaf area, number of leaves, and plant height showed significant results for the interaction of levels of priming agent, salt stress, and various maize variety types. When the impacts of priming/stress (AB), varieties/priming (AC), and varieties/stress (BC) are compared, substantial variations between these results were noted, validating their agreement with the suggested model. For the maize variety MNH360, the interaction of salt stress and various levels of priming agents showed that with 100 mM NaCl and primed with 1.50% potassium nitrate solution, leaf length was significantly greater than when exposed to 75 mM NaCl and control. However, at 75 mM NaCl with 1.00% of priming solution, showed maximum leaf length, but it reduced as the concentration of priming agent was increased (Fig. 1). A similar pattern was also noticed in the 30T60 maize variety. The interaction of salt stress and various levels of priming agents revealed that at 100 mM NaCl salt stress and priming with 1.50% potassium nitrate solution, leaf length was significantly greater than other treatments. At 75 mM NaCl salt stress, 30T60 showed a better response comparable to MNH360, shown in the plot (Fig. 1).
Figure 1: The effect of KNO3 on leaf length (cm), leaf width (cm), number of leaves, leaf area, and plant height (cm) of MNH360 and 30T60 maize varieties under salt stress. Data exhibit means ± SE of 4 replicates. The statistical variations were calculated at each concentration as compared to the controls. The non-identical letters (a–c) and asterisk (*) signs indicate a significant difference compared to the controls (p ≤ 0.05), determined by the Duncan multiple range test. C0 = Control, S1 = 75 mM NaCl, S2 = 100 mM NaCl, S0P1 = 0 mM NaCl, and 0.5% priming, S1P1 = 75 mM NaCl and 0.5% priming, S2P1 = 100 mM NaCl and 0.5% priming, S0P2 = 0 mM NaCl and 1% priming, S1P2 = 75 mM NaCl and 1% priming, S2P2 = 100 mM NaCl and 1% priming, S0P3 = 0 mM NaCl and 1.5% priming, S1P3 = 75 mM NaCl and 1.5% priming, S2P3 = 100 mM NaCl and 1.5% priming
The interaction of salt stress and various KNO3 as priming agents on MNH360 showed that under controlled conditions without KNO3 priming and salt stress, leaf width had less response than the salt stress at 100 mM NaCl and at 75 mM, primed with 1.50% and 1.00% potassium nitrate. Similarly, the leaf width was also higher than the other priming levels and salt stress, at 75 mM NaCl with 1.00% priming solution and it increased with increasing priming level (Fig. 1). The interaction of salt stress and various levels of KNO3 priming on the 30T60 variety showed that the salt stress at 100 mM and 75 mM NaCl and priming with 0.50% potassium nitrate the leaf width was maximum as compared to other levels of KNO3 at 75 mM and 100 mM NaCl. However, seed priming at 1.50% without salt stress showed the lowest leaf width (Fig. 1).
The interaction of various levels of priming solution and salt stress on maize variety MNH360 showed that the salt stress at 100 mM NaCl and seed priming with 1.50% potassium nitrate showed the highest value of leaf area as compared with the rest of the treatments, while 75 mM NaCl salt stress with 1.00% priming solution showed higher leaf area and it showed increasing trend. While under control conditions (salt stress at 0 mM NaCl) showed less leaf area (Fig. 1). The interaction of salt stress and various levels of KNO3 for seed priming on variety 30T60 showed that the salt stress at 100 mM NaCl and seed priming with 1.50% potassium nitrate showed a higher response as compared to other priming levels. While 75 mM NaCl stress with 1.00% priming solution showed high leaf area and increased with increasing priming level. While salt stress at 100 mM and 75 mM NaCl at 0% priming showed statistically similar values for leaf area (Fig. 1).
The interaction of various levels of KNO3 priming and salt stress on maize variety MNH360 showed that the salt stress at 100 mM NaCl and seed priming with 1.50% potassium nitrate showed higher number of leaves than the other priming levels as well as salt stress levels, while 75 mM NaCl salt stress with 1.00% level for seed priming showed less number of leaves that increased with increasing priming level (Fig. 1). The interaction of salt stress and various levels of KNO3 priming on maize variety 30T60 showed that the salt stress at 100 mM NaCl and seed priming with 0.50% potassium nitrate solution showed a higher number of leaves as compared to other priming levels. While 75 mM NaCl stress with 1.00% KNO3 priming solution showed fewer number of leaves and decreased with increasing priming level (Fig. 1).
The interaction of various levels of KNO3 solutions and salt stress on maize variety MNH360 showed that the salt stress at 100 mM NaCl and seed priming with 1.50% potassium nitrate solution the plant height was higher as compared with other priming levels. While 75 mM NaCl stress with 0%, 0.50%, and 1.00% priming solutions showed more plant height than 100 mM and 0 mM NaCl stress and decreased with increasing seed priming level (Fig. 1). The interaction of salt stress and various levels of priming solutions on maize variety 30T60 showed that the stress at 75 mM NaCl and KNO3 seed priming with 1.00% and 0.50% potassium nitrate solution and salt stress at 100 mM NaCl and priming with 1.50% showed higher plant height than another priming level with 100 mM and 0 mM salt stress (Fig. 1).
3.2 Chlorophyll and Carotenoid Contents
From the finding, it was noticed that all the interactions for salt stress, various KNO3, and maize varieties for total chlorophyll content and carotenoid contents showed statistically significant differences from each other.
The interaction of salt stress and various levels of KNO3 for seed priming on maize variety MNH360 showed that the salt stress at 100 mM NaCl and priming with 1.50% potassium nitrate showed maximum chlorophyll content as compared to 75 mM NaCl and control without KNO3 priming and salt stress while 75 mM NaCl without priming showed higher chlorophyll contents and decreased with increasing concentration of KNO3 for seed priming (Fig. 2). The interaction of salt stress and various levels of KNO3 for seed priming on maize variety 30T60 showed that the salt stress at 100 mM NaCl and seed priming with 1.00% potassium nitrate the chlorophyll content was higher than the other treatments while at 75 mM NaCl stress maize variety 30T60 showed the same results as for maize variety MNH36 (Fig. 2).
Figure 2: The effect of KNO3 on total chlorophyll content (μgg−1 FW) and carotenoid content (μg/g FW) of MNH360 and 30T60 maize varieties under salt stress. Data exhibit means ± SE of 4 replicates. The statistical variations were calculated at each concentration as compared to the controls. The non-identical letters (a–d) and asterisk (*) signs indicate a significant difference compared to the controls (p ≤ 0.05), determined by the Duncan multiple range test. C0 = Control, S1 = 75 mM NaCl, S2 = 100 mM NaCl, S0P1 = 0 mM NaCl, and 0.5% priming, S1P1 = 75 mM NaCl and 0.5% priming, S2P1 = 100 mM NaCl and 0.5% priming, S0P2 = 0 mM NaCl and 1% priming, S1P2 = 75 mM NaCl and 1% priming, S2P2 = 100 mM NaCl and 1% priming, S0P3 = 0 mM NaCl and 1.5% priming, S1P3 = 75 mM NaCl and 1.5% priming, S2P3 = 100 mM NaCl and 1.5% priming
The interaction of various levels of KNO3 for seed priming and salt stress on maize variety MNH360 showed that the salt stress at 100 mM NaCl and seed priming with 1.50%, 1.00%, and 0.50% potassium nitrate showed higher carotenoid contents as compared with rest of treatments. While 75 mM and 100 mM NaCl stress with 0% priming level showed lesser carotenoid content than 0 mM salt stress (Fig. 2). The interaction of salt stress and various levels of KNO3 for seed priming on maize variety 30T60 showed that the salt stress at 100 mM NaCl and seed priming with 1.50%, 1.00%, and 0.50% potassium nitrate showed the highest carotenoid contents as compared to other salt stress levels. While 75 mM and 100 mM NaCl stress with 0% priming solution showed lower carotenoid contents than the rest of the treatments. By increasing the concentration of KNO3 for seed priming and salt stress level carotenoid content also increased (Fig. 2).
3.3 Ascorbate Peroxidase (APX) Activity
The interaction of various levels of KNO3 for seed priming and salt stress on APX activity for maize variety MNH360 showed that the salt stress at 100 mM NaCl and seed priming with 1.50%, 1.00%, and 0.50% potassium nitrate showed higher response of the APX activity as compared to 75 mM and 0 mM NaCl stress. Maximum APX activity (0.0352 U mg−1 protein) was observed with 1.50% potassium nitrate seed priming at 100 mM salt stress. While minimum APX activity (0.016 U mg−1 protein) was observed with 0% potassium nitrate seed priming at 100 mM salt stress (Fig. 3). The interaction of salt stress and various levels of KNO3 seed priming of APX activity for maize variety 30T60 showed the same results as the above interaction. However maximum APX activity (0.028 U mg−1 protein) was observed with 1.50% of potassium nitrate solution for seed priming at 100 mM salt stress. While minimum APX activity was (0.012 U mg−1 protein) observed with 0.50% of potassium nitrate solution for seed priming at 0 mM salt stress (Fig. 3).
Figure 3: The effect of KNO3 on APX activity (units/mg protein) of MNH360 and 30T60 maize varieties under salt stress. Data exhibit means ± SE of 4 replicates. The statistical variations were calculated at each concentration as compared to the controls. The non-identical letters (a–e) and asterisk (*) signs indicate a significant difference compared to the controls (p ≤ 0.05), determined by the Duncan multiple range test. C0 = Control, S1 = 75 mM NaCl, S2 = 100 mM NaCl, S0P1 = 0 mM NaCl, and 0.5% priming, S1P1 = 75 mM NaCl and 0.5% priming, S2P1 = 100 mM NaCl and 0.5% priming, S0P2 = 0 mM NaCl and 1% priming, S1P2 = 75 mM NaCl and 1% priming, S2P2 = 100 mM NaCl and 1% priming, S0P3 = 0 mM NaCl and 1.5% priming, S1P3 = 75 mM NaCl and 1.5% priming, S2P3 = 100 mM NaCl and 1.5% priming
3.4 Assessment of Malondialdehyde (MDA) Content
The interaction of various levels of KNO3 for seed priming and salt stress on the MDA content of maize variety MNH360 showed that the salt stress at 100 mM NaCl and seed priming with 1.50%, 1.00%, and 0.50% potassium nitrate the MDA content was higher as compared to 75 mM and 0 mM NaCl stress. Maximum MDA contents (4.47 mmol g−1 FW) were observed with 1.50% potassium nitrate solution for seed priming at 100 mM salt stress. While minimum MDA content (1.95 mmol g−1 FW) was observed at 0 mM NaCl and seed priming with 0.50% potassium nitrate solution (Fig. 4). The interaction of salt stress and various levels of KNO3 solution for seed priming on MDA content for maize variety 30T60 showed that the salt stress at 100 mM NaCl and seed priming with 1.50%, 1.00%, and 0.50% potassium nitrate for MDA content was higher as compared to 75 mM and 0 mM NaCl stress. Maximum MDA content (3.308 mmol g−1 FW) was observed with 1.50% potassium nitrate solution for seed priming at 100 mM salt stress. While minimum MDA content (1.55 mmol g−1 FW) was observed at 0 mM NaCl and seed priming with 1.50% potassium nitrate solution (Fig. 4).
Figure 4: The effect of KNO3 on MDA content (mmol/g FW) of MNH360 and 30T60 maize varieties under salt stress. Data exhibit means ± SE of 4 replicates. The statistical variations were calculated at each concentration as compared to the controls. The non-identical letters (a–c) and asterisk (*) signs indicate a significant difference compared to the controls (p ≤ 0.05), determined by the Duncan multiple range test. C0 = Control, S1 = 75 mM NaCl, S2 = 100 mM NaCl, S0P1 = 0 mM NaCl, and 0.5% priming, S1P1 = 75 mM NaCl and 0.5% priming, S2P1 = 100 mM NaCl and 0.5% priming, S0P2 = 0 mM NaCl and 1% priming, S1P2 = 75 mM NaCl and 1% priming, S2P2 = 100 mM NaCl and 1% priming, S0P3 = 0 mM NaCl and 1.5% priming, S1P3 = 75 mM NaCl and 1.5% priming, S2P3 = 100 mM NaCl and 1.5% priming
The interaction of various levels of KNO3 solution for seed priming and salt stress on the CAT activity on maize variety MNH360 showed that the salt stress at 100 mM NaCl and seed priming with 1.50% potassium nitrate the CAT activity was higher as compared to 75 mM and 0 mM NaCl stress. Maximum CAT activity (0.0249 U mg−1 protein) was observed with 1.50% potassium nitrate solution used for seed priming at 100 mM salt stress. Minimum CAT activity (0.013 U mg−1 protein) was observed with 0.50% potassium nitrate solution used for seed priming at 0 mM salt stress (Fig. 5). The interaction of salt stress and various levels of KNO3 solution for seed priming on CAT activity for maize variety 30T60 showed the same results as the above interaction. Maximum CAT activity (0.021 U mg−1 protein) was observed with 1.50% potassium nitrate solution used for seed priming at 100 mM salt stress. Minimum CAT activity (0.012 U mg−1 protein) was observed with 0% potassium nitrate solution used for priming at 75 mM salt stress (Fig. 5).
Figure 5: The effect of KNO3 on CAT activity (units/mg protein) of MNH360 and 30T60 maize varieties under salt stress. Data exhibit means ± SE of 4 replicates. The statistical variations were calculated at each concentration as compared to the controls. The non-identical letters (a–d) and asterisk (*) signs indicate a significant difference compared to the controls (p ≤ 0.05), determined by the Duncan multiple range test. C0 = Control, S1 = 75 mM NaCl, S2 = 100 mM NaCl, S0P1 = 0 mM NaCl, and 0.5% priming, S1P1 = 75 mM NaCl and 0.5% priming, S2P1 = 100 mM NaCl and 0.5% priming, S0P2 = 0 mM NaCl and 1% priming, S1P2 = 75 mM NaCl and 1% priming, S2P2 = 100 mM NaCl and 1% priming, S0P3 = 0 mM NaCl and 1.5% priming, S1P3 = 75 mM NaCl and 1.5% priming, S2P3 = 100 mM NaCl and 1.5% priming
3.6 Guaiacol Peroxidase Activity (POD) (U mg−1 Protein)
The interaction of various levels of KNO3 solution for seed priming and salt stress on POD activity of maize variety MNH360 showed that the salt stress at 100 mM NaCl and seed priming with 1.50% and 1.00% potassium nitrate solution the POD activity was higher as compared to 75 mM and 0 mM NaCl stress. Maximum POD activity (0.0224 U mg−1 protein) was observed with 1.50% potassium nitrate solution for seed priming at 100 mM salt stress. While minimum POD activity (0.0117 U mg−1 protein) was observed with 0.50% potassium nitrate solution for seed priming at 0 mM salt stress (Fig. 6). The interaction of salt stress and various levels of KNO3 for seed priming on maize variety 30T60 showed the same results as the above interaction. Maximum POD activity (0.0191 U mg−1 protein) was observed with 1.50% potassium nitrate solution for seed priming at 100 mM salt stress. While minimum POD activity (0.0112 U mg−1 protein) was observed with 0% potassium nitrate solution for seed priming at 75 mM salt stress (Fig. 6).
Figure 6: The effect of KNO3 on POD activity (units/mg protein) of MNH360 and 30T60 maize varieties under salt stress. Data exhibit means ± SE of 4 replicates. The statistical variations were calculated at each concentration as compared to the controls. The non-identical letters (a–f) and asterisk (*) signs indicate a significant difference compared to the controls (p ≤ 0.05), determined by the Duncan multiple range test. C0 = Control, S1 = 75 mM NaCl, S2 = 100 mM NaCl, S0P1 = 0 mM NaCl, and 0.5% priming, S1P1 = 75 mM NaCl and 0.5% priming, S2P1 = 100 mM NaCl and 0.5% priming, S0P2 = 0 mM NaCl and 1% priming, S1P2 = 75 mM NaCl and 1% priming, S2P2 = 100 mM NaCl and 1% priming, S0P3 = 0 mM NaCl and 1.5% priming, S1P3 = 75 mM NaCl and 1.5% priming, S2P3 = 100 mM NaCl and 1.5% priming
3.7 Total Soluble Protein Content
The interaction of various levels of KNO3 for seed priming and salt stress on maize variety MNH360 showed that the salt stress at 100 mM NaCl and seed priming with 1.00% potassium nitrate on total soluble protein content was higher as compared to the other priming levels. While salt stress at 100 mM NaCl showed higher total soluble protein content than 75 mM and 0 mM NaCl stress. The values of total soluble protein were initially increased then decreased and again slightly increased by increasing the level of KNO3 for seed priming for both 75 and 0 mM salt stress. On the other hand, with salt stress at 0 mM NaCl and seed priming with 0% potassium nitrate the total soluble protein content was higher than other priming levels (Fig. 7). The interaction of salt stress and seed priming of maize variety 30T60 showed the same results as the above interaction. The only difference was that the salt stress at 75 and 100 mM NaCl and seed priming with 1.50% potassium nitrate solution have the same values of total soluble protein as other seed priming and stress levels (Fig. 7).
Figure 7: The effect of KNO3 on Total Soluble Protein content (mgg-1FW) of MNH360 and 30T60 maize varieties under salt stress. Data exhibit means ± SE of 4 replicates. The statistical variations were calculated at each concentration as compared to the controls. The non-identical letters (a–d) and asterisk (*) signs indicate a significant difference compared to the controls (p ≤ 0.05), determined by the Duncan multiple range test. C0 = Control, S1 = 75 mM NaCl, S2 = 100 mM NaCl, S0P1 = 0 mM NaCl, and 0.5% priming, S1P1 = 75 mM NaCl and 0.5% priming, S2P1 = 100 mM NaCl and 0.5% priming, S0P2 = 0 mM NaCl and 1% priming, S1P2 = 75 mM NaCl and 1% priming, S2P2 = 100 mM NaCl and 1% priming, S0P3 = 0 mM NaCl and 1.5% priming, S1P3 = 75 mM NaCl and 1.5% priming, S2P3 = 100 mM NaCl and 1.5% priming
To evaluate the relation among the measured attributes correlation analysis of two varieties of maize, i.e., MNH360 (Fig. 8), and 30T60 (Fig. 9) was depicted., In the maize variety MNH360 the leaf chlorophyll contents were positively correlated with leaf length, protein content and number of leaves while being negatively correlated with CAT, POD, APX and leaf area. Similarly, plant height, leaf width, carotenoids, and MDA contents showed non-significant results with each other. For the maize variety 30T60 chlorophyll content of the plants has a positive correlation with leaf width, number of leaves, plant height, CAT, and MDA contents and has a negative correlation with leaf length, carotenoids, APX, and protein content. However, the POD and leaf area showed non-significant association with each other.
Figure 8: Relationship between different parameters of maize variety MNH360 under various application levels of potassium nitrate for seed priming grown under salt stress. CAT (Catalase activity); POD (Guaiacol peroxidase activity); MDA (Malondialdehyde contents); LA (Leaf area); LW (Leaf width); Car (Carotenoids contents); NOL (Number of leaves); Pro (Total soluble protein); PH (Plant height); APX (Ascorbate peroxidase activity); LL (Leaf length), CHL (Total chlorophyll content)
Figure 9: Relationship between different parameters of maize variety 30T60 under various application levels of potassium nitrate for seed priming grown under salt stress. CAT (Catalase activity); POD (Guaiacol peroxidase activity); MDA (Malondialdehyde contents); LA (Leaf area); LW (Leaf width); Car (Carotenoids contents); NOL (Number of leaves); Pro (Total soluble protein); PH (Plant height); APX (Ascorbate peroxidase activity); LL (Leaf length), CHL (Total chlorophyll content)
3.9 Principal Component Analysis
A principal component analysis (PCA) was used to demonstrate the effect of various levels of potassium nitrate for seed priming on various concentrations of NaCl toxicity in two varieties of maize (MNH360, 30T60) (Figs. 10 and 11). For variety 30T60 Dim-1 and Dim-2 exhibited maximum contribution and occupy more than 69.6% of differences. Among which Dim-1 exhibits (53.9%) and Dim-2 exhibits (15.7%), while MNH360 accounted for more than 69.4% of the total database in which Dim-1 accounted for (47.8%) and Dim-2 accounted for (21.6%) of the database. In both varieties, parameters leaf length, leaf area, leaf width, number of leaves, plant height, chlorophyll content, carotenoids, protein, MDA, APX, POD, and CAT showed a positive correlation in the database.
Figure 10: Loading plot of principal component analysis (PCA) on various attributes of MNH360 under various application levels of potassium nitrate grown under salt stress. CAT (Catalase activity); POD (Guaiacol peroxidase activity); MDA (Malondialdehyde contents); LA (Leaf area); LW (Leaf width); Car (Carotenoids content); NOL (Number of leaves); Pro (Total soluble protein); PH (Plant height); APX (Ascorbate peroxidase activity); LL (Leaf length); CHL (Total chlorophyll content)
Figure 11: Loading plot of principal component analysis (PCA) on various attributes of 30T60 under various application levels of potassium nitrate grown under salt stress. CAT (Catalase activity); POD (Guaiacol peroxidase activity); MDA (Malondialdehyde contents); LA (Leaf area); LW (Leaf width); Car (Carotenoids content); NOL (Number of leaves); Pro (Total soluble protein); PH (Plant height); APX (Ascorbate peroxidase activity); LL (Leaf length); CHL (Total chlorophyll content)
When plants are exposed to abiotic stress such as salinity stress, in response to that salt stress, reactive oxygen species (ROS) production exceeds the antioxidant system’s capability, resulting in oxidative stress, which causes cytotoxic protein damage and lipid peroxidation [38]. It was quite obvious that one of the most important environmental variables limiting agricultural production is the salinity which results in increased production of ROS [38,39]. To cope up with unfavorable conditions seed priming is cost effective and a technique to enhance the growth and development of crop plants [40]. Researchers noticed that seed priming improved germination in a variety of crops [41]. In similar way, it was noticed that seedling growth has been improved by seed priming with suitable priming agent under stress conditions [14,42].
It was noticed in the current study that salt stress significantly affected the growth attributes of maize verities, while the seed priming with the KNO3 not only minimized the detrimental effects of salt stress but also improved the growth variable. The findings are in line with the findings of various researchers who noticed that priming with inorganic salts improves not only seed germination but also growth, metabolic processes, and crop production in most of the crops [43], and increased germination percentages and seedling growth in sense of maximum fresh and dry biomass production of crop seedlings [2,44]. The results of current research revealed that the growth attributes of MNH360 showed more positive response than 30T60. However, both varieties showed a good response under various levels of KNO3 seed priming under salt stress. It has been described that priming treatment may enhance K+ ion uptake and maximize the osmotic potential gradient, enabling more rapid water absorption in the seed. Our experimental work showed that 1.50% KNO3 with 100 mM NaCl stress significantly increased the vegetative and biochemical parameters of maize verities. According to Aksu et al. [45], potassium is one of the most crucial main mineral contents required for plant growth and development. Additionally, it functions as an agent that reduces stress in response to a variety of abiotic conditions, including salt and drought stress [46], and control conditions in tomatoes [47]. Furthermore, it was observed that the exogenous application of KNO3 has the ability to produce substances within the plants that release NO, which acts as a signaler molecule plants that this involved in the regulation of plant growth and development, defense against pathogens and responses to various abiotic stresses [45].
From the findings of the current study, it was observed that the chlorophyll and carotenoid content in both varieties of maize were significantly affected, and treatments of various levels of priming solution with KNO3 showed results, indicating that under salt stress conditions, and has a good capability to maintain its chlorophyll and carotenoid content. Potassium is required for several metabolic processes, including stomatal opening and photosynthesis. Potassium has just been discovered to provide abiotic stress tolerance [45]. Under salt stress, K aids in the maintenance of ion homeostasis and the regulation of osmotic equilibrium. It was investigated by researchers that the efficiency of the assimilation of photosynthetic products and translocation was affected by salt stress [48]. In our research work, seed priming mitigates the effect of salt stress and enhanced the chlorophyll and carotenoid content, in both varieties. However, 30T60 maize variety showed more chlorophyll and carotenoid content than MNH360. Furthermore, enhanced chlorophyll and carotenoids contents in maize plants was essential for adjustment of osmotic potential and increased photosynthetic activities under salt stressed conditions [49]. Increased chlorophyll and carotenoids contents in maize plants justified their increased emergence, growth and seedling vigor under salt stressed conditions. Low malondialdehyde content in primed maize seeds suggested defense from lipid peroxidation and oxidative stress that might be the reason for minimum membrane damage and improved growth.
It was observed that ascorbic acid content in plants increased significantly in all treatments applied. Ascorbic acid is readily oxidized to monodehydroascorbic acid as part of its antioxidant function [10,50]. APX activity in our study was significantly increased by various levels of KNO3 seed priming under salt-stress conditions. Priming with the KNO3 plays a very significant role in all physiological and antioxidant activities of maize plants.
The level of MDA showed that the level of salt tolerance in plants under salt stress treatment [51]. The addition of ions and active ROS production is associated with an increase in MDA content [42]. In our experimental results, lipid peroxidation (MDA) content strongly supported the hypothesis that by seed priming with the KNO3, MDA content increased under salt stress conditions. Salinity treatment increased the activities of antioxidant enzyme such as CAT, GR, and POD [52,53]. This suggests that adaptation to salinity improves antioxidant ability, which may effectively reduce ROS damage during stressful environments [54]. The findings of the current study revealed that catalase activities in both varieties showed significantly different results with respect to various levels of priming agent. By increasing KON3 salt in the priming solution and stress levels, CAT activities were increased in both varieties, but MNH360 showed more CAT activity than 30T60 which might be due to genetic make-up of variety. The POD activity increased as a result of a decreased in ROS by seed priming under salt stress conditions [55]. In our research work, seed priming with KNO3 showed a positive response to POD activities under salt-stress conditions.
Many researchers noticed that salt stress causes an accumulation of toxic ions in the cell that causes an increase in ROS, which causes oxidative damage to various biomolecules and cell organelles. Antioxidant metabolism is one of the very common defense systems that plants adapt to compensate for the damage caused by ROS [56]. A decrease in ROS may cause an increase in enzymatic antioxidants (CAT, POD, GR, and SOD) as a result of seed priming under salt stress [57]. Our results supported this phenomenon, showing that seed priming with KNO3 enhanced the total soluble protein content under salt stress. Both varieties were significantly different, but the MNH360 variety contained more total soluble protein content than the 30T60 due to better genetic make-up. The biochemical parameters showed a positive response to KNO3 priming and salt stressed conditions. Plants treated with a higher level of KNO3 for seed priming and a higher level of salt stress showed differential responses as compared with the rest of treatments.
In summary, seed priming with KNO3 can help to alleviate the detrimental effect of salt stress on plant growth by maintaining the photosynthetic activity and increasing the production of soluble sugar. Overall, seed priming with KNO3 could be used as a simple and cost-effective strategy to minimize the NaCl-induced damage in maize verities. We recommend comparing plant responses to seed priming in soil and hydroponics to broaden applicability in agriculture.
Germination and plant growth were more significantly impacted by salt stress. Higher levels of salt stress and KNO3 solution significantly affected all the measured attributes of maize verities. Better response for maize verity MNH360 was noticed as compared to 30T60. Growth attributes were higher in the 1.00% and 1.50% KNO3 priming treatments among all the priming treatments. Furthermore, it was also noticed that lipid peroxidation and enzymatic antioxidants such as MDA, TSP, CAT, POD, and APX showed a better response in MNH360 than in 30T60, despite 30T60 having higher chlorophyll and carotenoid contents than MNH360. The findings of this study justified the reliability of KNO3 seed priming, an easy and affordable technique to be adopted by farmers in salt affected regions for improving emergence seedling growth under salt stressed conditions.
Acknowledgement: The authors extend their appreciation to Taif University, Saudi Arabia, for supporting this work through Project Number (TU-DSPP-2024-254).
Funding Statement: The authors extend their appreciation to Taif University, Saudi Arabia, for supporting this work through Project Number (TU-DSPP-2024-254).
Author Contributions: Conceptualization: Bushra Rehman and Asma Zulfiqar, methodology and formal analysis: Houneida Attia and Rehana Sardar, investigation: Muneera A. Saleh, Faisal Mehmood and Qamar uz Zaman, resources: Khalid H. Alamer and Ibtisam M. Alsudays, writing—original draft preparation: Bushra Rehman, Faisal Mehmood and Qamar uz Zaman, writing—review and editing: Bushra Rehman and Asma Zulfiqar, visualization: Faisal Mehmood and Qamar uz Zaman, funding acquisition: Khalid H. Alamer and Ibtisam M. Alsudays. All authors reviewed the results and approved the final version of the manuscript.
Availability of Data and Materials: No data was used during the current study.
Ethics Approval: Not applicable.
Conflicts of Interest: The authors declare that they have no conflicts of interest to report regarding the present study.
References
1. Neill SJ, Desikan R, Clarke A, Hurst RD, Hancock JT. Hydrogen peroxide and nitric oxide as signaling molecules in plants. J Exp Bot. 2002;53(372):1237–47. doi:10.1093/jexbot/53.372.1237. [Google Scholar] [CrossRef]
2. Alamer KH, Perveen S, Khaliq A, Zia Ul Haq M, Ibrahim MU, Ijaz B. Mitigation of salinity stress in maize seedlings by the application of vermicompost and sorghum water extracts. Plants. 2022;11(19):2548. doi:10.3390/plants11192548. [Google Scholar] [PubMed] [CrossRef]
3. Hasanuzzaman M, Fujita M. Plant oxidative stress: biology, physiology and mitigation. Plants. 2022;11(9):1185. doi:10.3390/plants11091185. [Google Scholar] [PubMed] [CrossRef]
4. Hussain S, Ahmed S, Akram W, Ahmad A, Yasin NA, Fu M, et al. The potential of selenium to induce salt stress tolerance in Brassica rapa: evaluation of biochemical, physiological and molecular phenomenon. Plant Stress. 2023;11:100331. doi:10.1016/j.stress.2023.100331. [Google Scholar] [CrossRef]
5. Hussain HA, Hussain S, Khaliq A, Ashraf U, Anjum SA, Men S, et al. Chilling and drought stresses in crop plants: implications, cross talk, and potential management opportunities. Front Plant Sci. 2018;9:393. doi:10.3389/fpls.2018.00393. [Google Scholar] [PubMed] [CrossRef]
6. Bano A, Gupta A, Rai S, Fatima T, Sharma S, Pathak N. Mechanistic role of reactive oxygen species and its regulation via the antioxidant system under environmental stress. Plant Stress Physiol Persp Agri. 2021;1–18. doi:10.5772/intechopen.101045. [Google Scholar] [CrossRef]
7. Rehman A, Chandio AA, Jingdong L, Hussain I. Economic perspectives of maize crop in Pakistan: a time series analysis (1970–2015). Int J Adv Biotechnol Res. 2016;7(3):968–74. [Google Scholar]
8. Liu J, Xie W, Yang J, Yao R, Wang X, Li W. Effect of different fertilization measures on soil salinity and nutrients in salt-affected soils. Water. 2023;15(18):3274. doi:10.3390/w15183274. [Google Scholar] [CrossRef]
9. Raza SH, Athar HR, Ashraf M, Hameed A. Glycinebetaine-induced modulation of antioxidant enzymes activities and ion accumulation in two wheat cultivars differing in salt tolerance. Environ Exp Bot. 2007;60(3):368–76. doi:10.1016/j.envexpbot.2006.12.009. [Google Scholar] [CrossRef]
10. Singh A, Rajput VD, Sharma R, Ghazaryan K, Minkina T. Salinity stress and nanoparticles: insights into antioxidative enzymatic resistance, signaling, and defense mechanisms. Environ Res. 2023;116585. doi:10.1016/j.envres.2023.116585. [Google Scholar] [PubMed] [CrossRef]
11. Acosta-Motos JR, Ortuño MF, Bernal-Vicente A, Diaz-Vivancos P, Sanchez-Blanco MJ, Hernandez JA. Plant responses to salt stress: adaptive mechanisms. Agronomy. 2017;7(1):18. doi:10.3390/agronomy7010018. [Google Scholar] [CrossRef]
12. Murungu F, Chiduza C, Nyamugafata P, Clark L, Whalley W, Finch-Savage W. Effects of ‘on-farm seed priming’ on consecutive daily sowing occasions on the emergence and growth of maize in semi-arid Zimbabwe. Field Crops Res. 2004;89(1):49–57. doi:10.1016/j.fcr.2004.01.020. [Google Scholar] [CrossRef]
13. Sardar R, Ahmed S, Yasin NA. Seed priming with karrikinolide improves growth and physiochemical features of Coriandrum sativum under cadmium stress. Environ Adv. 2021;5:100082. doi:10.1016/j.envadv.2021.100082. [Google Scholar] [CrossRef]
14. Mehmood F, Kousar H, Hassan F, Zaman QU. Synthesis of zinc oxide nanoparticles by precipitation and sol gel methods from different precursors and their comparison impact on seedling attributes of wheat. J Nano Res. 2022;72:25–35. doi:10.4028/v-lon9gt. [Google Scholar] [CrossRef]
15. Farooq M, Usman M, Nadeem F, ur Rehman H, Wahid A, Basra SM, et al. Seed priming in field crops: potential benefits, adoption and challenges. Crop Pasture Sci. 2019;70(9):731–71. doi:10.1071/CP18604. [Google Scholar] [CrossRef]
16. Araujo SDS, Paparella S, Dondi D, Bentivoglio A, Carbonera D, Balestrazzi A. Physical methods for seed invigoration: advantages and challenges in seed technology. Front Plant Sci. 2016;7:646. [Google Scholar] [PubMed]
17. Kouki R, Ayachi R, Ferreira R, Sleimi N. Behavior of Cucumis sativus L. in presence of Aluminum stress: germination, plant growth and antioxidant enzymes. Food Sci Nutr. 2021;9(6):3280–8. doi:10.1002/fsn3.v9.6. [Google Scholar] [CrossRef]
18. Alamer KH, Attia H. UV-C seed priming improves tomato plant growth against salt stress. J Taibah Uni Sci. 2022;16(1):1181–91. doi:10.1080/16583655.2022.2153443. [Google Scholar] [CrossRef]
19. Ahmad Z, Anjum S, Waraich EA, Ayub MA, Ahmad T, Tariq RMS, et al. Growth, physiology, and biochemical activities of plant responses with foliar potassium application under drought stress–a review. J Plant Nut. 2018;41(13):1734–43. doi:10.1080/01904167.2018.1459688. [Google Scholar] [CrossRef]
20. Sardans J, Peñuelas J. Potassium control of plant functions: ecological and agricultural implications. Plants. 2021;10(2):419. doi:10.3390/plants10020419. [Google Scholar] [PubMed] [CrossRef]
21. Hasanuzzaman M, Bhuyan MB, Nahar K, Hossain MS, Mahmud JA. Hossen MS potassium: a vital regulator of plant responses and tolerance to abiotic stresses. Agronomy. 2018;8(3):31. doi:10.3390/agronomy8030031. [Google Scholar] [CrossRef]
22. Ali AS, Elozeiri AA. Metabolic processes during seed germination. Adv Seed Biol. 2017;141–66. doi:10.5772/intechopen.70653. [Google Scholar] [CrossRef]
23. Kaur B, Kaur G, Asthir B. Biochemical aspects of nitrogen use efficiency: an overview. J Plant Nut. 2017;40(4):506–23. doi:10.1080/01904167.2016.1240196. [Google Scholar] [CrossRef]
24. Basra S, Ullah E, Warriach E, Cheema M, Afzal I. Effect of storage on growth and yield of primed canola (Brassica napus) seeds. Int J Agri Biol. 2003;5(2):117–20. [Google Scholar]
25. Mohammadi G. The influence of NaCl priming on seed germination and seedling growth of canola (Brassica napus L.) under salinity conditions. Am-Eurasian J Agri Environ Sci. 2009;5(5):696–700. [Google Scholar]
26. Açikbaş S, Özyazici MA, Biçakçi E, Özyazici G. Germination and seedling development performances of some soybean (Glycine max (L.) Merrill) cultivars under salinity stress. Turkish J Range Forage Sci. 2023;4(2):108–18. doi:10.51801/turkjrfs.1387963. [Google Scholar] [CrossRef]
27. Bankaji I, Hammouda IB, Attia H, Sleimi N. Effects of hydro-priming and hormonal priming on seedling growth and seed germination of Cucurbita pepo treated by mercury. Int J Life Sci Eng. 2018;3(3):59–63. [Google Scholar]
28. Sadeghian S, Yavari N. Effect of water-deficit stress on germination and early seedling growth in sugar beet. J Agron Crop Sci. 2004;190(2):138–44. doi:10.1111/jac.2004.190.issue-2. [Google Scholar] [CrossRef]
29. Anosheh HP, Sadeghi H, Emam Y. Chemical priming with urea and KNO3 enhances maize hybrids (Zea mays L.) seed viability under abiotic stress. J Crop Sci Biotechnol. 2011;14:289–95. doi:10.1007/s12892-011-0039-x. [Google Scholar] [CrossRef]
30. Shareen, Faraz A, Faizan M. Physiological impact of reactive oxygen species on leaf. In: Reactive oxygen species: prospects in plant metabolism. Singapore: Springer; 2023. p. 95–113. doi:10.1007/978-981-19-9794-5_6. [Google Scholar] [CrossRef]
31. Carleton AE, Foote WH. A comparison of methods for estimating total leaf area of barley plants. Crop Sci. 1965;5(6):602–3. doi:10.2135/cropsci1965.0011183X000500060041x. [Google Scholar] [CrossRef]
32. Arnon DI. Copper enzymes in isolatad cloroplasts. Polyphenoloxidase in Beta vulgaris. Plant Physiol. 1949;24:1–15. doi:10.1104/pp.24.1.1. [Google Scholar] [PubMed] [CrossRef]
33. Wellburn AR. The spectral determination of chlorophylls a and b, as well as total carotenoids, using various solvents with spectrophotometers of different resolution. J Plant Physiol. 1994;144(3):307–13. doi:10.1016/S0176-1617(11)81192-2. [Google Scholar] [CrossRef]
34. Nakano Y, Asada K. Hydrogen peroxide is scavenged by ascorbate-specific peroxidase in spinach chloroplasts. Plant Cell Physiol. 1981;22(5):867–80. [Google Scholar]
35. Heath RL, Packer L. Photoperoxidation in isolated chloroplasts: I. Kinetics and stoichiometry of fatty acid peroxidation. Arch Biochem Biophy. 1968;125(1):189–98. doi:10.1016/0003-9861(68)90654-1. [Google Scholar] [PubMed] [CrossRef]
36. Dhindsa RS, Plumb-Dhindsa P, Thorpe TA. Leaf senescence: correlated with increased levels of membrane permeability and lipid peroxidation, and decreased levels of superoxide dismutase and catalase. J Exp Bot. 1981;32(1):93–101. doi:10.1093/jxb/32.1.93. [Google Scholar] [CrossRef]
37. Chance B, Maehly A. Assay of catalases and peroxidases. Methods Enzymol. 1955;2:764–75. doi:10.1016/S0076-6879(55)02300-8. [Google Scholar] [CrossRef]
38. Gossett DR, Millhollon EP, Lucas MC. Antioxidant response to NaCl stress in salt-tolerant and salt-sensitive cultivars of cotton. Crop Sci. 1994;34(3):706–14. doi:10.2135/cropsci1994.0011183X003400030020x. [Google Scholar] [CrossRef]
39. Singh D. Juggling with reactive oxygen species and antioxidant defense system–a coping mechanism under salt stress. Plant Stress. 2022;5(5):100093. doi:10.1016/j.stress.2022.100093. [Google Scholar] [CrossRef]
40. Messedi D, Sleimi N, Abdelly C. Some physiological and biochemical aspects of salt tolerance of Sesuvium portulacastrum. In: Cash Crop Halophytes: Recent Studies: 10 Years after Al Ain Meeting, 2003; Dordrecht: Springer Netherlands; p. 1–77. [Google Scholar]
41. Parera CA, Cantliffe DJ. Presowing seed priming. Horti Rev. 1994;16(16):109–41. doi:10.1002/9780470650561. [Google Scholar] [CrossRef]
42. Farooq M, Basra S, Ahmad N, Hafeez K. Thermal hardening: a new seed vigor enhancement tool in rice. J Integ Plant Biol. 2005;47(2):187–93. doi:10.1111/j.1744-7909.2005.00031.x. [Google Scholar] [CrossRef]
43. Al-Muaini A, Green S, Dakheel A, Abdullah A-H, Sallam O, Abou Dahr WA. Water requirements for irrigation with saline groundwater of three date-palm cultivars with different salt-tolerances in the hyper-arid United Arab Emirates. Agric Water Manag. 2019;222:213–20. doi:10.1016/j.agwat.2019.05.022. [Google Scholar] [CrossRef]
44. Demir I, Mavi K, Ozcoban M, Okcu G. Effect of salt stress on germination and seedling growth in serially harvested aubergine (Solanum melongena L.) seeds during development. Israel J Plant Sci. 2003;51(2):125–31. doi:10.1560/EAD8-YE43-E5BE-WHHV. [Google Scholar] [CrossRef]
45. Aksu G, Altay H. The effects of potassium applications on drought stress in sugar beet. Sugar Tech. 2020;22(6):1092–102. doi:10.1007/s12355-020-00851-w. [Google Scholar] [CrossRef]
46. Alam A, Ullah H, Chaum S, Tisarum R, Datta A. Effect of seed priming with potassium nitrate on growth, fruit yield, quality and water productivity of cantaloupe under water-deficit stress. Sci Hortic. 2021;288:110354. doi:10.1016/j.scienta.2021.110354. [Google Scholar] [CrossRef]
47. Moaaz Ali M, Javed T, Mauro RP, Shabbir R, Afzal I, Yousef AF. Effect of seed priming with potassium nitrate on the performance of tomato. Agriculture. 2020;10(11):498. doi:10.3390/agriculture10110498. [Google Scholar] [CrossRef]
48. Xiong L, Zhu JK. Molecular and genetic aspects of plant responses to osmotic stress. Plant Cell Environ. 2002;25(2):131–9. doi:10.1046/j.1365-3040.2002.00782.x. [Google Scholar] [PubMed] [CrossRef]
49. Attia H, Alamer K, Algethami B, Zorrig W, Hessini K, Gupta K, et al. Gibberellic acid interacts with salt stress on germination, growth and polyamine gene expression in fennel (Foeniculum vulgare Mill.) seedlings. Physiol Mol Biol Plants. 2022;28(3):607–22. doi:10.1007/s12298-022-01140-4. [Google Scholar] [PubMed] [CrossRef]
50. Chaudhry S, Sidhu GPS. Climate change regulated abiotic stress mechanisms in plants: a comprehensive review. Plant Cell Rep. 2022;41(1):1–31. doi:10.1007/s00299-021-02759-5. [Google Scholar] [PubMed] [CrossRef]
51. Bor M, Özdemir F, Türkan I. The effect of salt stress on lipid peroxidation and antioxidants in leaves of sugar beet Beta vulgaris L. and wild beet Beta maritima L. Plant Sci. 2003;164(1):77–84. doi:10.1016/S0168-9452(02)00338-2. [Google Scholar] [CrossRef]
52. Misra N, Gupta AK. Effect of salinity and different nitrogen sources on the activity of antioxidant enzymes and indole alkaloid content in Catharanthus roseus seedlings. J Plant Physiol. 2006;163(1):11–8. doi:10.1016/j.jplph.2005.02.011. [Google Scholar] [PubMed] [CrossRef]
53. Balla K, Bencze S, Janda T, Veisz O. Analysis of heat stress tolerance in winter wheat. Acta Agron Hungarica. 2009;57(4):437–44. doi:10.1556/AAgr.57.2009.4.6. [Google Scholar] [CrossRef]
54. Amirjani M. Biochemical and physiological responses of rice to cadmium. Int J Forest Soil Erosion. 2012;2(3):128–32. [Google Scholar]
55. Almeselmani M, Deshmukh P, Sairam R. High temperature stress tolerance in wheat genotypes: role of antioxidant defence enzymes. Acta Agron Hungarica. 2009;57(1):1–14. doi:10.1556/AAgr.57.2009.1.1. [Google Scholar] [CrossRef]
56. Bouslimi H, Ferreira R, Dridi N, Brito P, Martins-Dias S, Cacador I, et al. Effects of barium stress in Brassica juncea and Cakile maritima: the indicator role of some antioxidant enzymes and secondary metabolites. Phyton-Int J Exp Bot. 2021;90:145–58. doi:10.32604/phyton.2020.011752. [Google Scholar] [CrossRef]
57. Khan I, Raza MA, Awan SA, Shah GA, Rizwan M, Ali B. Amelioration of salt induced toxicity in pearl millet by seed priming with silver nanoparticles (AgNPsthe oxidative damage, antioxidant enzymes and ions uptake are major determinants of salt tolerant capacity. Plant Physiol Biochem. 2020;156:221–32. doi:10.1016/j.plaphy.2020.09.018. [Google Scholar] [PubMed] [CrossRef]
Cite This Article
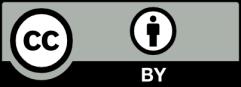
This work is licensed under a Creative Commons Attribution 4.0 International License , which permits unrestricted use, distribution, and reproduction in any medium, provided the original work is properly cited.