Open Access
ARTICLE
Enhancing Canola Yield and Photosynthesis under Water Stress with Hydrogel Polymers
1 Field Crops Research Department, National Research Centre, Cairo, 12622, Egypt
2 Botany Department, National Research Centre, Cairo, 12622, Egypt
3 Plant Production Department, College of Food and Agriculture Sciences, King Saud University, Riyadh, 12271, Saudi Arabia
4 Department of Agronomy, Hajee Mohammad Danesh Science and Technology University, Dinajpur, 5200, Bangladesh
5 Department of Field Crops, Faculty of Agriculture, Siirt University, Siirt, 56100, Turkey
6 Department of Agronomy, Faculty of Agriculture, Kafrelsheikh University, Kafrelsheikh, 33516, Egypt
7 Department of Research Centers, Montana State University, Bozeman, MT 59717, USA
* Corresponding Authors: Ayman El Sabagh. Email: ; Magdi T. Abdelhamid. Email:
Phyton-International Journal of Experimental Botany 2024, 93(7), 1623-1645. https://doi.org/10.32604/phyton.2024.054453
Received 29 November 2023; Accepted 18 April 2024; Issue published 30 July 2024
Abstract
While Egypt’s canola production per unit area has recently grown, productivity remains low, necessitating increased productivity. Hydrogels are water-absorbent polymer compounds that can optimize irrigation schedules by increasing the soil’s ability to retain water. Accordingly, two field experiments were conducted to examine hydrogel application to sandy soil on canola growth, biochemical aspects, yield, yield traits, and nutritional quality of yielded seeds grown under water deficit stress conditions. The experiments were conducted by arranging a split-plot layout in a randomized complete block design (RCBD) with three times replications of each treatment. While water stress at 75% or 50% of crop evapotranspiration (ETc) lowered chlorophyll a, chlorophyll b, carotenoids, and total pigments content, indole-3-acetic acid, plant development, seed yield, and oil and total carbohydrates of seed yield, hydrogel treatment enhanced all of the traits mentioned above. Furthermore, hydrogel enhanced to gather compatible solutes (proline, amino acids, total soluble sugars), phenolics content in leaves, seed protein, and crop water productivity, which increased while the plants were under water stress. The results revealed that the full irrigation (100%ETc) along with hydrogel compared to water-stressed (50%ETc) led to enhanced seed yield (kg ha), Oil (%), and Total carbohydrates (%) of rapeseed by 57.1%, 11.1% and 15.7%, respectively. Likewise, under water-stressed plots with hydrogel exhibited enhancement by 10.0%, 3.2% and 5.1% in seed yield (kg ha), oil (%), and total carbohydrates (%) of rapeseed by 57.1%, 11.1% and 15.7%, respectively compared to control. As a result, the use of hydrogel polymer will be a viable and practical solution for increasing agricultural output under water deficit stress situations.Keywords
Brassica napus (L.), commonly known as rapeseed or canola, belongs to the Brassicaceae family, and is renowned as one of the world’s foremost vegetable oil crops [1–3]. Canola is among the top five major oil crops in the world, alongside soybean, sunflower, cotton seeds, and palm oil. After the soybean and oil palm, it is considered the third most crucial oil seed crop, producing 70.91 million metric tons in the 2018/19 season [4]. Canola seeds boast a rich oil content of 40%–48% and a protein content of 18%–25% [5], with saturated fatty acids accounting for roughly 7% and unsaturated fatty acids making up the remaining 95% [6]. Canola serves as a versatile component in intercrops and as a green manuring crop in organic agriculture [7,8].
In Egypt, there is a shortage in vegetable oil production due to a notable increase in annual vegetable oil consumption. As a result, it is crucial to extend canola production outside of the Nile Valley, including on sandy soil [9–12]. Introducing canola production in Egypt provides an opportunity to mitigate the country’s deficiency in edible oil output. Hence, one of Egypt’s main goals is to boost crop yield by cultivating it in previously untapped territories with sandy soil.
Sandy soil is susceptible to damage from a variety of environmental factors, including water scarcity and temperature swings between day and night [12]. Agricultural water consumption accounts for more than 85% of worldwide water use. Water deficits in plants can lead to various morpho-physiological problems, including decreased nutrient absorption and hindered active transport, as well as a reduction in photosynthesis and transpiration [13–20]. Even short-term water deficits during reproductive stages can impair seed yield production [21–27]. Poor water quality reduces agricultural crop production to variable degrees worldwide [28,29], particularly in tropical and sub-tropical counties [30]. Dry and semiarid areas worldwide experience water scarcity as a severe environmental challenge due to lower amounts of precipitation and erratic spatial and temporal distributions, limiting overall plant development [31,32]. The development of modern irrigation technologies that conserve water is considered crucial for maintaining optimum soil moisture levels, enhancing water use efficiency (WUE), and minimizing the losses of crop productivity and quality [33,34]. Contemporary micro-irrigation tools, like micro-sprinklers and drip irrigation systems accompanied by ideal irrigation scheduling and artificial mulching (plastic mulch), have significantly reduced water consumption and increased WUE in crop plants [35–37]. These improved tools, however, are typically utilized in crops requiring substantial investments, ongoing operating costs, and specialized knowledge from growers. Exploring marginal irrigation technology utilizing highly expandable polymer materials to ensure sufficient soil moisture for productivity enhancement is another approach to address the pressing water-related issues [38]. Hydrogel polymer technology, due to its numerous benefits including high water absorption and retention, has recently gained widespread use in agriculture as a soil conditioner. By preventing water loss through evaporation, percolation, and leaching in arid and semiarid conditions worldwide, hydrogel polymer technology improves the growth and productivity of crops. Additionally, it maintains adequate soil moisture levels during water scarcity situations [39–41].
Hydrophilic materials play a crucial role in mitigating the impact of water deficits on plant productivity by increasing their ability to absorb large amounts of water, thereby alleviating the negative effects of water deficit stress on crops [42]. Hydrogel, also known as raindrop in India, is an irrigation water-binding clustered organic cross-linked co-polymer. Its use as a soil conditioner has been proven to reduce soil water loss and boost fruit yield [39]. In its dry formula, hydrogel polymer appears as white crystalline granules, specifically designed for fruit plants and orchards [43]. Often referred to as “root watering crystals,” hydrogels expand upon contact with irrigation water, multiplying their volume and increasing soil water holding capacity of soil while reducing the need for frequent irrigation [44]. Studies have demonstrated that incorporating hydrogel alongside irrigation can boost seedling growth. In sandy soil, hydrogel treatment increases water retention capacity and plant water potential [45]. Moreover, the application of hydrogel caused increases in water holding capacity as well as water potential in sandy soil. As stated by [44], hydrogel treatment significantly reduces irrigation frequency, especially for coarse-textured fields. Hydrogels are also claimed to reduce nutrient leaching (NPK). As a fruit tree soil conditioner, hydrogel polymer effectively improves water deficit stress tolerance and promotes the growth of fruit trees under sandy soil or lightweight gravel substrate-containing soil.
In comparison to the control group, the application of stockosorb @ 100 g/tree considerably enhanced the growth and productivity of Citrus limon [46]. Similarly, studies have shown a substantial increase in soybean seed production (6%–25% and wheat grain yield (3%–15%) with hydrogel application compared to no-hydrogel (control) plots [47]. In addition, hydrogel soil application has been found to improve water usage efficiency and enhance the growth of maize compared to the control group [48].
Under Egyptian conditions, several reports have highlighted the effectiveness of hydrogel in decreasing irrigation water usage to 50% or 75% of the recommended irrigation water requirements in several crops. For example, studies involving rice and barley cultivated in sandy soil treated with hydrogel at 8 g/m2 demonstrated a reduction in irrigation water needs by 25% while maintaining high yield levels. Another study focusing on sugar beet [49] revealed that hydrogel application improved the nutrient use efficiency of N, P, and K while decreasing irrigation water requirements for obtaining a higher yield of sugar beet.
Therefore, the main purpose of this study is to examine the effects of hydrogel application to the sandy soil on the growth, various biochemical aspects, yield, yield traits, and nutritional quality of yielded seeds of canola plants under different irrigation water requirements.
To find out the effects of hydrogel on growth, physio-chemical traits, yield, and quality of canola plants grown in sandy soil under water deficiency stress conditions, two studies were conducted at the Research and Production Station, National Research Centre, Nubaria, Egypt during two successive winter seasons 2019/20 and 2020/21. The site is located at 30°30′1.4′′N latitude, and 30°19′10.9′′E longitude, with an elevation of 21 m above sea level. The data of average temperature (minimum and maximum) and relative humidity during the 2019/20 and 2020/21 growing seasons at the experimental site were received at the weather station, National Research Centre, Nubaria region, which are shown in Fig. 1.
Figure 1: The data of average minimum and maximum temperature, and relative humidity at the Experimental Station of the National Research Centre, Nubaria region in seasons 2019/20 and 2020/21
Soil samples from two depths (0–30 cm and 30–60 cm from the soil surface) before canola planting were taken with an “auger” for soil analysis. Physical (Texture) and chemical analyses of the soil at the experimental site are presented in Tables 1 and 2. Please clarify that these analyses were made before treatment imposition.
Canola (Brassica napus L.) seeds variety “Serw 4” was provided by the oil crops Research Department, Agriculture Research Center, Giza, Egypt. Before sowing, the seeds were sterilized with 10% sodium hypochlorite solution for 15 min, then washed with distilled water and sun-dried. The dimensions of each experimental plot were 3.5 m by 3.0 m, with a gap of 60 cm between adjacent plots. Seeds were sown at a rate of 7.00 kg ha−1 on 29th November in the 2019 and 2020 growing seasons and harvested on 12 April 2020 and 13 April 2021, respectively. A combined driller was used for land preparation, as well as fertilizer application and seed sowing.
The experiments were conducted using a 3 × 2 split-plot layout according to a randomized complete block design (RCBD) having three repeats for each treatment. Irrigation treatments were allotted to the main plots, each main plot was separated into two sub-plots (split plots) wherein hydrogel at 40 kg ha−1 was placed at the first sub-plot and untreated control at the second sub-plot. The spacing between the main plots in the experimental layout was 1.5 m.
The main plots included three irrigation regimes, namely, (i) 100% of crop evapotranspiration (ETc) during the growing season i.e., sufficient moisture condition (I100), (ii) 75% of ETc during the growing season i.e., mild water deficit stress (I75), and (iii) 50% of ETc during the growing season i.e., water deficit stress (I50). The experiments consisted of six treatments of combined irrigation regimes and application of hydrogel viz., T1, 100% ETc without hydrogel; T2, 100% ETc + hydrogel; T3, 75% ETc without hydrogel; T4, 75% ETc + hydrogel; T5, 50% ETc without hydrogel; and T6, 50% ETc + hydrogel. Hydrogels are polymer materials having a three-dimensional (3D) network. These materials are formed synthetically from natural sources which are stable and hydrophilic in nature. However, the main properties of the used superabsorbent hydrogels are presented in Table 3.
The land was fertilized with calcium super-phosphate (15.5% P2O5) @ 150 kg ha−1 as basal dose, ammonium nitrate (33.5% N) @ 180 kg ha−1 after emergence at five equal doses before the 1st, 2nd, 3rd, 4th, and 5th irrigation, and potassium sulfate (48.52% K2O) @ 120 kg ha−1 at two equal doses of before the 1st and 3rd irrigations. The crop was grown following the recommendation of the Agriculture and Land Reclamation Ministry, Egypt.
2.2 Irrigation Water Requirements
Irrigation was done by using a drip irrigation system as per the requirement of the crop. The three irrigation (I) regimes, viz., 100%, 75%, and 50% of crop evapotranspiration (ETc), were used based on the difference between ETc and rainfall. ETc under standard conditions was measured according to Allen et al. [50]:
ETc (mm/day) = ETo * Kc
where ETo is the reference crop evapotranspiration (mm/day), and Kc is the crop coefficient.
The penman-Monteith equation was used to measure the ETo following Allen et al. [50], and the amount of water at every irrigation level throughout the 2019/20 and 2020/21 seasons is shown in Table 4. The soil moisture content as a percentage was measured gravimetrically.
ETo was calculated using data from a weather station placed in the facilities at the Research and Production Station of the National Research Centre, Nubaria, Egypt. These irrigation levels were applied after the uniform seedlings were established and continued up to the harvesting of the crop.
Irrigated treatments are applied on the same day. Furthermore, rainfall amounts were recorded throughout the experimental period. In each experimental plot, flow meters were installed to determine the volume of irrigation water applied. An irrigation channel was developed near the experimental area as a water source and measured the EC (0.41 dS m−1), SAR (2.8%), and pH (7.35) of irrigation water. The gravimetric method was to measure the soil moisture content on the preceding and succeeding days of each irrigation at each depth of 0–15, 15–30, 30–45, and 45–60 cm to determine water depletion from the root zone.
Ten plants were collected for measurement of plant height and dry weight at 60 days after sowing (DAS) during the vegetative stage. Fresh leaf samples were used to determine photosynthetic apparatus (chlorophyll a, chlorophyll b, carotenoids, and total pigments), indole-3-acetic acid (IAA), total phenolics (TP), osmolytes (proline; Pro, amino acids; AA, total soluble sugar; TSS). At the harvest date, one square meter from the center of each plot treatment was taken to determine seed yield per plant (SYP), thousand kernel weight (TKW), and seed yield per hectare (SYH). Crop water productivity (kg mm−1 ha−1) was measured by dividing seed yield (kg ha−1) and the total amount of supplied water (mm−1 ha−1) [51]. Photosynthetic pigments (Chlorophyll a, chlorophyll b, carotenoids, and total pigments) were estimated [52]. Indole acetic acid was determined according to the method reported by Larsen et al. [53]. Total phenolic compounds were determined according to the method described by Zheng et al. [54]. Total soluble sugars were determined according to Dubois et al. [55]. Proline was extracted following the method described by Vartanian et al. [56] and assayed according to the procedure outlined by Bates et al. [57]. Free amino acids were measured by following the method described by Yemm et al. [58]. Oil content in seed was measured by using the Soxhlet apparatus and petroleum ether (40°C–60°C) [59]. The protein content was determined by the micro-Kjeldahl method [59]. Total carbohydrates were determined according to Dubois et al. [55].
A test of normality distribution was done by using a method described by Shapiro et al. [60]. Collected data were tested for the validation of assumptions underlying the combined analysis of variance by a separate analysis of each season, and a combined analysis across the two seasons was then performed if the homogeneity of individual error variances examined by the Levene test [61] was insignificant. The collected data were subjected to a combined analysis of variance (ANOVA) for a split-plot layout with two factors in a randomized complete block design [62]. Statistically significant differences between means were compared at p ≤ 0.05 using Tukey’s honestly significant difference (HSD) test. The statistical analysis used GenStat 19th Edition (VSN International Ltd., Hemel Hempstead, UK). GenStat 19th Edition was used to perform principal component analysis (PCA). The treatments and variables were grouped using the first two PC scores, PC1 and PC2, which accounted for the most variability of the parameters examined.
3.1 Changes in Photosynthetic Pigments Content of Canola Plants
The results revealed that in Table 5, the addition of hydrogel to soil improved the chlorophyll a, chlorophyll b, carotenoids, and total pigments not only in plants grown under 100% ETc but also under 75% and 50% ETc over control plants. Water stress (75% or 50% ETc) statistically (P ≤ 0.05) decreased photosynthetic pigments constituents of canola leaves (chlorophyll a, chlorophyll b, carotenoids, and total pigments) contrasted to unstressed plants which grow under 100% ETc (Table 5). The addition of hydrogel with 40 kg ha−1 increased different photosynthetic pigment constituents in comparison with those plants produced without hydrogel addition to soil. Moreover, the interaction between the treatment of irrigation regimes and hydrogel treatments on chlorophyll a, chlorophyll b, carotenoids, and total pigments content was significant. However, the maximum values of chlorophyll a, chlorophyll b, carotenoids, and total pigments were observed under 100% ETc and hydrogel treatment combination, followed by irrigation at 75% or 50% ETc.
3.2 Changes in Indole Acetic Acid (IAA) and Total Phenolics (TP) Contents
Table 6 shows the effect of irrigation regimes and hydrogel soil addition on the canola plant’s endogenous IAA contents. Moderate and severe water stress (75% or 50% ETc) decreased endogenous IAA, increasing the phenolic contents of canola plants compared with control plants (100% ETc). Water stress at 75% and 50% ETc reduced IAA contents by 25.2% and 33.3% compared with those plants grown under 100% ETc, while increased phenolic contents by 40.5% and 83.9% at 75% and 50% ETc, respectively. On the other hand, hydrogel amended to soil treatment considerably augmented the IAA and phenolic contents in plants over the hydrogel untreated plants. The findings from Table 6 reveal that the highest concentration of indole-3-acetic acid (IAA) was observed when the irrigation regime was set at 100% of crop evapotranspiration (ETc) along with the addition of hydrogel to the soil. This was followed by lower concentrations of IAA with irrigation levels at 75% ETc and 50% ETc, which showed reductions of 23.19% and 37.26%, respectively. Concurrently, there was a decrease in phenolic contents across the different irrigation regimes and hydrogel applications.
3.3 Changes in Compatible Solute Accumulation
Water stress conditions represented by 75% and 50% of ETc resulted in a significant (p ≤ 0.05) accumulation of compatible solutes including proline (Pro), amino acids (AA), total soluble sugar (TSS) compared with control plants grown under 100% ETc. Moreover, the addition of hydrogel to soil increased the osmolytes compared to plants grown without hydrogel at different levels of water stress. Regarding the interaction of hydrogel application and different water irrigation levels, Table 7 shows the promoting role of hydrogel on the mentioned parameters compared to plants grown without hydrogel across different water irrigation requirements.
3.4 Changes in the Growth of Canola Plants
Compared to plants grown at 100% ETc, water deficit (75% and 50% ETc) resulted in significant declines in plant growth attributes expressed as plant height (cm) and dry weight (g) (p ≤ 0.05) (Table 8). Table 8 also illustrates the promotive consequence of hydrogel on the plant height and dry weight of canola plants under various irrigation water requirements (ETc). Meanwhile, the addition of hydrogel to sandy soil, both under well-watered and water deficit conditions (75% and 50% ETc) significantly increased the growth parameters over control. Concerning the interaction effect of hydrogel (with and without) on plant height and dry weight under different irrigation water requirements (100%, 75%, and 50% ETc), the highest values for plant height and dry weight were recorded when the hydrogel was applied with 100% ETc.
3.5 Changes in Yield and the Attributes of Canola Plants
A reduction in water availability, transitioning from 100% to 75% or 50% of ETc led to a remarkable decrease (p ≤ 0.05) in yield contributing characteristics including thousand kernel weight (TKW) and seed yield per plant (SYP), as well as seed yield per hectare (SYH) (Table 9). However, water productivity (WP) showed an increase with decreasing irrigation levels down to 50% ETc. In contrast, the addition of hydrogel significantly enhanced yield, its contributing attributes, and characteristics of WP (Table 9) compared to plants grown without hydrogel addition. Moreover, significant interactions were observed between different irrigation levels (ETc) and hydrogel addition to soil concerning yield and its attributes. The application of hydrogel to soil not only significantly increased the yield attributes, yield, and WP in plants grown under full irrigation conditions (100% ETc) but also in plants subjected to water stress conditions (75% or 50% ETc) as compared with control plants without hydrogel.
3.6 Changes in the Nutritional Value of Canola Plants
Moderate water stress (75% ETc) and severe water stress conditions (50% ETc) significantly decreased the oil and total carbohydrate contents of canola seeds paralleled to control plants (fully irrigated plants), as depicted in Table 10. Meanwhile, 75% or 50% ETc led to a significant increase in protein contents compared to 100% ETc. On the other hand, the application of hydrogel to the soil under all irrigation regimes significantly increased the oil, protein, and total carbohydrate contents in seeds relative to seeds under controlled plants (Table 10). Significant interaction effects between irrigation regimes and hydrogel treatment were observed for all studied traits, namely oil, protein, and total carbohydrates. Notably, the interaction effect of water stress and hydrogel significantly improved seed protein content (Table 10).
3.7 Principal Component Analysis (PCA) of Canola Plants under Irrigation Regimes
The PCA biplot of the PC1 (1st principal component) and PC2 (2nd principal component) was conducted to classify the 18 variables of canola plants measured under a combination of six treatments of soil application with hydrogel under three different levels of irrigation water requirements. The treatments are labeled as follows: T1, 100% ETc without hydrogel; T2, 100% ETc + hydrogel; T3, 75% ETc without hydrogel; T4, 75% ETc + hydrogel; T5, 50% ETc without hydrogel; and T6, 50% ETc + hydrogel). The analysis revealed that PC2 effectively sorted the parameters (Chl a, chlorophyll a; Chl b, chlorophyll b; Car, carotenoids; TPP, total photosynthetic pigments; IAA, indole acetic acid; TP, total phenolics; Pro, proline; AA, amino acids; TSS, total soluble sugars; PH, plant height; SDW, shoot dry weight; SYP, seed yield per plant; TKW, thousand kernel weight; SYH, seed yield per hectare; WP, water productivity; Oil, oil; Prot, protein; TC, total carbohydrates) observed across different treatments (Fig. 2). The first two factors accounted for 97.28% of the initial variability of the data, while the number of “useful” dimensions was automatically detected as five. In the biplots presented in Fig. 2, treatments that cluster together exhibit comparable behavior across variables, whereas clustered variables have similar effects on the treatments. The angle between variable vectors indicates the degree of correlation between them, with an acute angle representing a positive association (r close to 1), a right angle indicating no association (r close to 0), and an obtuse angle signifying a negative association (r close to -1). Distinct behavior was observed for treatments T2 and T4 compared to the other treatments. Furthermore, the variables Pro, AA, TSS, Prot, WP, and TP were found to be primarily correlated, while the other 12 variables exhibited associations among themselves.
Figure 2: PCA biplot for the PC1 vs. PC2 regarding the classification of 18 variables of canola plants measured under a combination of 6 treatments of soil application with hydrogel under 3 different levels of irrigation water requirements. T1, 100% ETc without hydrogel; T2, 100% ETc + hydrogel; T3, 75% ETc without hydrogel; T4, 75% ETc + hydrogel; T5, 50% ETc without hydrogel; and T6, 50% ETc + hydrogel. Chl a chlorophyll a; Chl b, chlorophyll b; Car, carotenoids; TPP, total photosynthetic pigments; IAA, indole acetic acid; TP, total phenolics; Pro, proline; AA, amino acids; TSS, total soluble sugars; PH, plant height; SDW, shoot dry weight; SYP, seed yield per plant; TKW, thousand kernel weight; SYH, seed yield per hectare; WP, water productivity; Oil, oil; Prot, protein; TC, total carbohydrates
Water stress poses a significant challenge in agriculture, leading to substantial impacts on crop yield by inhibiting plant growth and development [63–68]. The observed reduction in plant height and fresh weight due to water deficiency, as demonstrated in this study (Table 8), mirrors findings reported by [69–72], tthese adverse effects may be attributed to declines in cell enlargement and turgor pressure.
Furthermore, water deficiency can result in reduced water absorption, inadequate water potential, increased cell ion concentrations, and decreased leaf stomatal conductance [73]. It has been reported that water deficit stress exacerbates oxidative stress, disrupts nutritional balance, and causes hormonal abnormalities, ultimately leading to a decline in protein levels and enzyme activity [74–76].
Oilseed crops such as canola, benefit from increased soil moisture and optimized nutrient and photosynthate transport under low irrigation and rainfed conditions, facilitated by the application of hydrogel [77,78]. Hydrogel interacts with irrigation regimes to produce water-laden gel “chunks,” which aid in early plant establishment and promote superior plant growth. A comparable pattern for the release of water from hydrogel in pearl millet was also noted during water deprivation conditions [79]. Singh et al. [80] explained that the plant can more effectively utilize root zone hydration under irregular irrigation conditions due to increased soil moisture retaining capacity conferred by hydrogel treatment, along with its subsequent progressive release for extended periods. The hydrogel application, particularly in moisture-scarce conditions, encourages plant life [81], increases dry matter generation, and lengthens the stay-green quality [82]. This leads to improved growth and yield characteristics due to the increased nutrient availability and prolonged water uptake [77,83]. According to the current study, the application of hydrogels increased canola seed production by 10% compared to untreated plants (Table 9). Jat et al. [84] reported a similar increase in mustard seed yield by 8.7% under similar soil moisture conditions, although the specific causes differed owing to variations in soil types and weather conditions. In conditions of limited moisture, hydrogel treatment discharges roughly four times more soil moisture when the moisture tension rises from 10 to 100 kPa [85]. This demonstrates the capacity of hydrogel to efficiently retain and gradually release water, thus enhancing soil moisture availability for plant uptake. The addition of hydrogel has been found to enhance several key aspects of soybean growth, development, and yield by improving plant canopy structure, more fantastic chlorophyll content, and the number of branches [78].
During the siliqua forming and development stage, moisture deprivation drastically decreased the siliqua length, affecting the critical stages of plant growth. However, hydrogel application ensured the availability of substantial soil moisture and nutrients near the rhizosphere zone, aligning with the plant’s requirements and mitigating the decrease in siliqua length [86]. The hydrogel helps plants to draw water and nutrients from broader and deeper soil depths, which boosts the intake of nitrogen, phosphate, potassium, calcium, and magnesium leading to improved growth and yield characteristics [87]. The decline in seed production observed with increasing water deficit levels underscores the greater benefit of hydrogel use in moisture-stressed conditions (Table 9). A higher yield can be attained by improving the soil’s water-retention capacity and cluster structures by applying hydrogel, which promotes improved root development and plant growth control [88]. Similar findings of increased nutrient absorption and yield advantage have been documented in mustard [77], pearl millet [80], and sorghum [89].
The positive effect of adding hydrogel to soil aligns with previous research findings which have demonstrated a similar effect on the growth of olive [90] and apple mint plants [91]. Hydrogels possess a copolymeric nature that enables them to retain significant amounts of moisture and nutrients in the soil. This unique property allows hydrogels to provide moisture and nutrients to plants, even in conditions of water and nutritional deficits, thereby enhancing photosynthesis and promoting overall plant vigor. Hydrogels have an impressive capacity to absorb moisture, with the ability to expand up to 150 times their original volume and retain up to 980 mL of water [91]. In addition to their role in water retention, hydrogels also improve soil aeration, which leads to enhanced plant growth and production.
Compared to unstressed plants, considerable reductions in photosynthetic pigment components were observed to 50% or 75% water deficiencies (Table 5). These reductions may be attributed to the instability and degradation of pigment-protein complexes, a common consequence of drought-induced stress [92,93]. Furthermore, the decline in leaf photosynthetic pigments could be attributed to a defense mechanism against damage induced by reactive oxygen species (ROS), as suggested by Harbinger et al. [94]. In contrast, hydrogel soil amendments significantly augmented the photosynthetic pigments. This promotion of photosynthetic pigment by hydrogel under stressful circumstances has been demonstrated previously in various crops including sunflower [51], corn [95], and tomato [96]. Moreover, hydrogel promotes plant growth by gradually supplying water to the plant, thereby reducing water stress, and it is one of the most widely used agricultural treatments for this purpose [51]. The enhanced uptake of micro-and macro-nutrients, particularly nitrogen and potassium, also contributed to increased dry weight with hydrogel application, consistent with the results of M’barki et al. [90].
Water stress resulted in lower levels of IAA in canola leaves compared to control plants (Table 6). This reduction may be attributed to increased IAA degradation or enhanced IAA oxidase activity. Bano et al. [97] highlighted that plants respond to water stress by producing significant quantities of osmoprotectants. These osmoprotectants play a crucial role in safeguarding plants against stress by stabilizing the tertiary structure of various cellular components such as membranes, enzymes, and proteins. This stabilization helps maintain the functionality and integrity of these vital cellular elements even under conditions of water stress, thereby enhancing the plant’s resilience to adverse environmental conditions [98]. Phenols, which are antioxidants produced from various secondary metabolites in the shikimic acid cycle, play a role in cellular signaling activities under abiotic stress [99,100]. While water stress increased phenols content, hydrogel application further raised phenols contents (Table 6). In comparison to the control, tomato plants treated with super water absorbance hydrogel polymer exhibited elevated levels of total phenol concentration, an antioxidant marker, suggesting heightened antioxidative potential [96,101].
Water deficit increased the proline content of leaves compared to control plants (Table 7). This increase could be due to reduced proline oxidase and proline catabolic enzymes [102]. Furthermore, proline works as a stabilizer of membranes and specific macromolecules and a scavenger of free radicals. It is known as a carbon and nitrogen source via quick stress recovery [103]. The increase in proline levels in response to water shortage and hydrogel treatment is consistent with prior research findings [104]. Notably, there appears to be no direct link between osmotic potential and relative water content, indicating proline’s osmotic properties may not fully explain the water potential observed in hydrogel-treated plants [105]. Additionally, proline serves as a metal chelator, antioxidant, and osmoprotectant [106].
Soluble sugars increased in several species under water stress due to a water deficit. Water stress causes a considerable rise in sugar content [103], which may have a function in osmotic control, increasing the plant’s tolerance to water deficits [107]. The positive impact of hydrogel treatment on osmotic protector content may be linked to its role as a soil reservoir. This enhances crop water absorption capacity, and water use efficiency, prevents nutrient leaching, improves fertilizer use efficiency, and reduces nutrient loss from the root zone, particularly on sandy soils [90].
Water stress decreased yield components as well as the yield of the canola. At the same time, hydrogel application to soil increased yield components and yield of plants grown under water deficit stress and non-stress conditions (Table 9). Water stress adversely affects water status in plants, reduces cell water content, induces osmotic stress, and inhibits cell enlargement, cell division, and overall plant growth [92,108]. It is worth mentioning that the availability of water in plants at various growth stages negatively affects crop productivity and the chemical components of produced seeds. These decreases are mainly due to reduced growth parameters (Table 8) and photosynthetic pigments (Table 5). Hydrogel’s superiority in enhancing yield and yield characteristics may be related to its action as a soil reservoir, which maximizes plant water uptake efficiency while increasing soil water-holding, water use efficiency, nutrient leaching prevention, and fertilizer use efficiency. This reduces nutrient loss from the root zone in sandy soil [44]. The superiority effect of hydrogel on raising yield and yield attributes in several crops under Egyptian settings has been reported [109].
Furthermore, it is noteworthy that while water stress increased protein, it decreased seed yield and carbohydrate content (Tables 9 and 10). Reduced crop seed yields under water stress conditions can be attributed to lower photosynthetic pigment levels [92] and reduced activity of enzymes in the Calvin cycle [110]. Decreasing water availability during plant growth affects diverse enzyme activities, leading to changes in various metabolic activities and subsequent alterations in metabolite translocation to the grain [111]. Lipids (peroxidation of unsaturated fatty acids in membranes), proteins (denaturation), carbohydrates, and nucleic acids are primary components of cells vulnerable to damage under water stress conditions [112]. Reduced oil content during drought may be attributed to the oxidation of polyunsaturated fatty acids [113]. Total carbohydrate alterations are especially significant since they are linked to physiological functions like photosynthesis, translocation, and respiration. Water stress lowers the amounts of pigments in the leaves, which inhibits photosynthetic activity, resulting in less carbohydrate build-up in mature leaves and, in turn, a slower rate of glucose transport from source to seed (from leaves to developing seeds) [114]. The oxidative stability of polyunsaturated fatty acids in the oil and the expression of antioxidant activity depend on the total phenol content of oilseeds [111,115,116]. Moreover, the increase in photosynthetic pigment and total protein levels can be attributed to higher nutrition accumulation [117].
While water stress with 75% or 50% crop evapotranspiration, reduced photosynthetic pigments, indole acetic acid, plant growth, seed yield per plant, thousand kernel weight, seed yield per hectare, and nutritional values of yielded seed of oil, and total carbohydrates, application of hydrogel with 40 kg ha−1 in sandy soil improved all traits mentioned above. In addition, hydrogel also promoted compatible solute accumulation in leaves (proline, amino acids, total soluble sugar), total phenolics, seed protein, and water productivity, which were mainly increased under water stress. Thus, the use of hydrogels significantly reduced water requirements in plants. With hydrogel, the limited available irrigation water could be used effectively to reduce moisture stress in the canola crop when irrigation was insufficient. However, before promoting hydrogel, it is essential to thoroughly investigate the water retention and transmission properties under various soil types and textures. Researchers still have difficulties to overcome, including determining the gel’s compatibility with different conditioners and lowering the gel’s price.
Acknowledgement: The authors extend their appreciation to Researchers Supporting Project No. (RSP2024R298), King Saud University, Riyadh, Saudi Arabia.
Funding Statement: This research was funded by the Researchers Supporting Project No. (RSP2024R298), King Saud University, Riyadh, Saudi Arabia.
Author Contributions: The authors confirm their contribution to the paper as follows: study conception and design: Elham A. Badr and Gehan Sh. Bakhoum; data collection and analysis: Mervat Sh. Sadak; draft manuscript preparation: Magdi T. Abdelhamid. writing-review and editing, Mohammad Sohidul Islam, Ibrahim Al-Ashkar and Ayman El Sabagh. All authors reviewed the results and approved the final version of the manuscript.
Availability of Data and Materials: Not applicable.
Ethics Approval: All the data supporting the findings of this study are included in this article.
Conflicts of Interest: All the authors mentioned above have reviewed and endorsed the submission and declare that there are no competing financial or non-financial interests.
References
1. Gacek K, Bartkowiak-Broda I, Batley J. Genetic and molecular regulation of seed storage proteins (SSPs) to improve protein nutritional value of oilseed rape (Brassica napus L.) seeds. Front Plant Sci. 2018;9:890. doi:10.3389/fpls.2018.00890. [Google Scholar] [PubMed] [CrossRef]
2. Raboanatahiry N, Li H, Yu L, Li M. Rapeseed (Brassica napusprocessing, utilization, and genetic improvement. Agronomy. 2021;11(9):1776. doi:10.3390/agronomy11091776. [Google Scholar] [CrossRef]
3. Borges CE, von dos Santos Veloso R, da Conceição CA, Mendes DS, Ramirez-Cabral NYZ, Shabani F, et al. Forecasting Brassica napus production under climate change with a mechanistic species distribution model. Sci Rep. 2023;13:12656. doi:10.1038/s41598-023-38910-3. [Google Scholar] [PubMed] [CrossRef]
4. Adeleke BS, Babalola OO. Oilseed crop sunflower (Helianthus annuus) as a source of food: nutritional and health benefits. Food Sci Nutr. 2020;8(9):4666–84. doi:10.1002/fsn3.1783. [Google Scholar] [PubMed] [CrossRef]
5. Marinković R, Marjanović-Jeromela A, Mitrović P. Specifities of winter rapeseed (Brassica napus L.) production. Zbornik radova Instituta za ratarstvo i povrtarstvo. 2009;46(1):33–43 (In Latin). [Google Scholar]
6. Bukhari MA, Sharif MS, Ahmad Z, Barutçular C, Afzal M, Hossain A. El Sabagh A silicon mitigates the adverse effect of drought in Canola (Brassica napus L.) through promoting the physiological and antioxidants activity. Silicon. 2021;13:3817–26. doi:10.1007/s12633-020-00685-x. [Google Scholar] [CrossRef]
7. Antanasović S, Ćupina B, Krstić Đ., Manojlović M, Čabilovski R, Marjanović Jeromela A, et al. Potential of autumn-sown rapeseed (Brassica napus) as a green manure crop. Cruciferae Newsletter. 2012;31:26–8. [Google Scholar]
8. Mikic A, Antanasović S, Ćupina B, Marjanović Jeromela A, Erić P, Jakšić S. Intercropping autumn-sown brassicas with annual legumes for green manure. Cruciferae Newsletter. 2015;34:11–3. [Google Scholar]
9. Gao L, Caldwell CD, Jiang Y. Photosynthesis and growth of camelina and canola in response to water deficit and applied nitrogen. Crop Sci. 2018;58(1):393–401. [Google Scholar]
10. Marjanović-Jeromela A, Marinković R, Jocković M, Mitrović P, Milovac Ž., Hristov N, et al. Evaluation of genetic variance components for some quantitative traits in rapeseed (Brassica napus L.). Genetika. 2014;46(1):179–85. doi:10.2298/GENSR1401179M. [Google Scholar] [CrossRef]
11. Seyis F, Friedt W, Voss A, Luhs W. Identification of individual Brassica oleracea plants with low erucic acid content. Asian J Plant Sci. 2004;3(5):593–6. doi:10.3923/ajps.2004.593.596. [Google Scholar] [CrossRef]
12. Nuttall WF. The effect of seeding depth, soil moisture regime, and crust strength on emergence of rape cultivars. Agron J. 1982;74(6):1018–22. doi:10.2134/agronj1982.00021962007400060020x. [Google Scholar] [CrossRef]
13. Ahluwalia O, Singh PC, Bhatia R. A review on drought stress in plants: implications, mitigation and the role of plant growth promoting rhizobacteria. Resour Environ Sustain. 2021;5:100032. doi:10.1016/j.resenv.2021.100032. [Google Scholar] [CrossRef]
14. Muhammad M, Waheed A, Wahab A, Majeed M, Nazim M, Liu YH, et al. Soil salinity and drought tolerance: an evaluation of plant growth, productivity, microbial diversity, and amelioration strategies. Plant Stress. 2024;11:100319. doi:10.1016/j.stress.2023.100319. [Google Scholar] [CrossRef]
15. Chauhan J, Srivastava JP, Singhal RK, Soufan W, Mishra UN, Anuragi H, et al. Alterations of oxidative stress indicators, antioxidant enzymes, soluble sugars, and amino acids in mustard [Brassica juncea (L.) Czern and Coss.] in response to varying sowing time, and field temperature. Front Plant Sci. 2022;13:875009. doi:10.3389/fpls.2022.875009. [Google Scholar] [PubMed] [CrossRef]
16. El Sabagh A, Javeed HM, Ali M, Skalicky M, Nawaz F, Qamar R, et al. Lipoic acid combined with melatonin mitigates oxidative stress and promotes root formation and growth in salt-stressed canola seedlings (Brassica napus L.). Molecules. 2021;26(11):3147. doi:10.3390/molecules26113147. [Google Scholar] [PubMed] [CrossRef]
17. Ahmad Z, Barutçular C, Zia Ur Rehman M, Sabir Tariq RM, Afzal M, Waraich EA, et al. Pod shattering in canola reduced by mitigating drought stress through silicon application and molecular approaches—a review. J Plant Nutr. 2022;46(1):101–28. [Google Scholar]
18. El Sabagh A, Hossain A, Barutçular C, Gormus O, Ahmad Z, Hussain S, et al. Effects of drought stress on the quality of major oilseed crops: implications and possible mitigation strategies–a review. Appl Ecol Environ Res. 2019b;17(2):4019–43. doi:10.15666/aeer/1702_40194043. [Google Scholar] [CrossRef]
19. Awan SA, Khan I, Rizwan M, Brestic M, Wang X, Zhang X, et al. Exogenous melatonin regulates the expression pattern of antioxidant-responsive genes, antioxidant enzyme activities, and physio-chemical traits in pearl millet under drought stress. J Plant Growth Regul. 2023;43:1–15. doi:10.1007/s00344-023-11159-w. [Google Scholar] [CrossRef]
20. El Sabagh A, Hossain A, Islam MS, Barutcular C, Hussain S, Hasanuzzaman M, et al. Drought and salinity stresses in barley: consequences and mitigation strategies. AJCS. 2019d;13(6):810–20. doi:10.21475/ajcs.19.13.06.p1286. [Google Scholar] [CrossRef]
21. Ghobadi M, Bakhshandeh M, Fathi G, Gharineh MH, Alami-Said K, Naderi A, et al. Short and long periods of water stress during different growth stages of canola (Brassica napus L.effect on yield, yield components, seed oil and protein contents. J Agron. 2006;5:336–41. doi:10.3923/ja.2006.336.341. [Google Scholar] [CrossRef]
22. Germchi S, Shekari F, Hassanpooraghdam MB, Benam MBK, Shekari F. Water deficit stress affects growth and some biochemical characteristics of rapeseed (Brassica napus L.). J Food, Agric Environ. 2010;8(3&4):1126–9. [Google Scholar]
23. BirunAra A, Shekari F, Hassanpouraghdam MB, Khorshidi MB, Esfandyari E. Effects of water deficit stress on yield, yield components and phenology of canola (Brassica napus L.) at different growth stages. J Food, Agric Environ. 2011;9(3&4):506–9. [Google Scholar]
24. Pavlista AD, Isbell TA, Baltensperger DD, Hergert GW. Planting date and development of spring-seeded irrigated canola, brown mustard and Camelina. Ind Crop Prod. 2011;33(2):451–6. doi:10.1016/j.indcrop.2010.10.029. [Google Scholar] [CrossRef]
25. Ahmad M, Waraich EA, Hafeez MB, Zulfiqar U, Ahmad Z, Iqbal MA, et al. Changing climate scenario: perspectives of Camelina sativa as low-input biofuel and oilseed crop. Glob Agric Prod: Resilience Clim Change. 2023;2:197–236. [Google Scholar]
26. Ahmad Z, Warraich EA, Iqbal MA, Barutcular C, Alharby H, Bamagoos A, et al. Foliage applied silicon ameliorates drought stress through physio-morphological traits, osmoprotectants and antioxidant metabolism of camelina (Camelina sativa L.) genotypes. Acta Scientiarum Polonorum Hortorum Cultus. 2021;20(4):43–57. [Google Scholar]
27. Waraich EA, Ahmed Z, Zahoor A, Rashid A, Erman M, Cig F, et al. Alterations in growth and yield of camelina induced by different planting densities under water deficit stress. Phyton-Int J Exp Bot. 2020;89(3):587. doi:10.32604/phyton.2020.08734. [Google Scholar] [CrossRef]
28. Ahmadi M, Bahrani M. Yield and yield components of rapeseed as influenced by water stress at different growth stages and nitrogen levels. Am-Eurasian J Agric Environ Sci. 2009;5(6):755–61. [Google Scholar]
29. Okorogbona AOM, Denner FDN, Managa LR, Khosa TB, Maduwa K, Adebola PO, et al. Water quality impacts on agricultural productivity and environment. In: Lichtfouse E, editor. Sustainable agriculture reviews. Cham: Springer; 2018. vol. 27, p. 1–35. doi:10.1007/978-3-319-75190-0_1. [Google Scholar] [CrossRef]
30. Yarnia M, Arabifard N, Khoei FR, Zandi P. Evaluation of drought tolerance indices among some winter rapeseed cultivars. Afr J Biotechnol. 2011;10(53):10914–22. doi:10.5897/AJB11.1748. [Google Scholar] [CrossRef]
31. Ferrari E, da Paz A, da Silva AC. Déficit hídrico no metabolismo da soja em semeaduras antecipadas no Mato Grosso. Nativa. 2015;3:67–77. doi:10.14583/2318-7670.v03n01a12. [Google Scholar] [CrossRef]
32. Pereira JWL, Filho PAM, Albuquerque MB, Nogueira RJMC, Santos RC. Biochemical changes in peanut genotypes submitted to moderate water stress. Revista Ciência Agronômica. 2012;43(4):766–73 (In Portuguese). doi:10.1590/S1806-66902012000400019. [Google Scholar] [CrossRef]
33. Panigrahi P, Sahu NN, Pradhan S. Evaluating partial root-zone irrigation and mulching in okra (Abelmoschus esculentus L.) under a sub-humid tropical climate. J Agric Rural Dev Trop Subtrop (JARTS). 2011;112(2):169–75. [Google Scholar]
34. Pereira LS, Oweis T, Zairi A. Irrigation management under water scarcity. Agric Water Manage. 2002;57(3):175–206. doi:10.1016/S0378-3774(02)00075-6. [Google Scholar] [CrossRef]
35. Abd El-Wahed MH, Ali EA. Effect of irrigation systems, amounts of irrigation water and mulching on corn yield, water use efficiency and net profit. Agric Water Manage. 2013;120(1):64–71. doi:10.1016/j.agwat.2012.06.017. [Google Scholar] [CrossRef]
36. Brahma S, Phookan D, Kachari M, Hazarika TK, Das K. Growth, yield and economics of broccoli under different levels of nitrogen fertigation. Indian J Hortic. 2010;67(4):279–82. [Google Scholar]
37. Deshmukh G, Hardaha MK. Effect of irrigation and fertigation scheduling under drip irrigation in Papaya. J Agric Search. 2014;1(4):216–20. [Google Scholar]
38. Keivanfar S, Ghazvini RF, Ghasemnezhad M, Mousavi A, Khaledian MR. Effects of regulated deficit irrigation and superabsorbent polymer on fruit yield and quality of’granny smith’apple. Agricultur Conspect Scientifi. 2019;84(4):383–9. [Google Scholar]
39. Abobatta W. Impact of hydrogel polymer in agricultural sector. Adv Agric Environ Sci Open Access. 2018;1(2):59–64. doi:10.30881/aaeoa.00011. [Google Scholar] [CrossRef]
40. Bedi K, Sohrab F. Evaluation of super absorbent polymer application on water holding capacity and potential in three soil type. J Sci Polym Technol. 2004;3:163–73. [Google Scholar]
41. Saini AK, Patel M, Saini LH, Malve SH. Growth, phenology and yield of summer Pearl millet (Pennisetum glaucum L.) as affected by varied application of water, nutrients and hydrogel. Int J Ecol Environ Sci. 2016;2(3):248–52. [Google Scholar]
42. Olszewski M, Goldsmith RS, Guthrie EK, Young CA. Use of sieved compost plus hydrogel for solid matrix priming of carrot seeds. Compost Sci Util. 2012;20(1):5–10. doi:10.1080/1065657X.2012.10737015. [Google Scholar] [CrossRef]
43. Green C, Foster C, Cardon GE, Butters GL, Brick M, Ogg B. Water release from cross-linked polyacrylamide. Collins, CO, USA: Colorado State University; 2004. p. 252–60. [Google Scholar]
44. Koupai JA, Eslamian SS, Kazemi JA. Enhancing the available water content in unsaturated soil zone using hydrogel, to improve plant growth indices. Ecohydrol Hydrobiol. 2008;8(1):67–75. doi:10.2478/v10104-009-0005-0. [Google Scholar] [CrossRef]
45. Viero P, Little K, Oscroft D. The effect of a soil-amended hydrogel on the establishment of a Eucalyptus grandis x E. camaldulensis clone grown on the sandy soils of Zululand. South Afr For J. 2000;188(1):21–8. doi:10.1080/10295925.2000.9631266. [Google Scholar] [CrossRef]
46. Pattanaaik SK, Wangchu L, Singh B, Hazarika BN, Singh SM, Pandey AK. Effect of hydrogel on water and nutrient management of Citrus reticulata. Res Crops. 2015;16(1):98–103. doi:10.5958/2348-7542.2015.00015.7. [Google Scholar] [CrossRef]
47. Rajanna GA, Singh SMA, Singh SBVK, Dass A, Chakraborty D, Patanjali N, et al. Biopolymeric superabsorbent hydrogels enhance crop and water productivity of soybean-wheat system in indo-gangetic plains of India. Sci Rep. 2022;12:11955. doi:10.1038/s41598-022-16049-x. [Google Scholar] [PubMed] [CrossRef]
48. Albalasmeh AA, Mohawesh O, Gharaibeh MA, Alghamdi AG, Alajlouni MA, Alqudah AM. Effect of hydrogel on corn growth, water use efficiency, and soil properties in a semi-arid region. J Saudi Soc Agric Sci. 2022;21(8):518–24. [Google Scholar]
49. El-Karamany M, Waly A, Shaaban AM, Alhady OA, Bakry AB. Utilization of hydrogel for reducing water irrigation under sandy soil condition. 3-effect of hydrogel on yield and yield components of sugar beet under sandy soil conditions. Res J Pharm, Biol Chem Sci. 2015;6(2):1025–32. [Google Scholar]
50. Allen RG, Pereira LS, Raes D, Smith MCE. Guidelines for computing crop water requirements. In: FAO Irrigation and Drainage Paper 56. Food and Agriculture Organization of the United Nations; 1998; Rome, Italy. p. 300. [Google Scholar]
51. Nazarli H, Zardashti MR, Darvishzadeh R, Najafi S. The effect of water stress and polymer on water use efficiency, yield and several morphological traits of sunflower under greenhouse condition. Notulae Sci Biologic. 2010;2(4):53–8. doi:10.15835/nsb.2.4.4823. [Google Scholar] [CrossRef]
52. Lichtenthaler HK, Buschmann C. Chlorophylls and carotenoids: measurement and characterization by UV-VIS spectroscopy, in current protocols in food analytical chemistry. John Wiley and Sons; 2001. [Google Scholar]
53. Larsen P, Harbo A, Klungsöyr S, Aasheim T. On the biogenesis of some indole compounds in Acetobacter xylinum. Physiol Plant. 1962;15(3):552–65. doi:10.1111/j.1399-3054.1962.tb08058.x. [Google Scholar] [CrossRef]
54. Zheng W, Wang SY. Antioxidant activity and phenolic compounds in selected herbs. J Agric Food Chem. 2001;49(11):5165–70. doi:10.1021/jf010697n. [Google Scholar] [PubMed] [CrossRef]
55. Dubois M, Gilles KA, Hamilton JK, Rebers PT, Smith F, et al. Colorimetric method for determination of sugars and related substances. Anal Chem. 1956;28(3):350–6. [Google Scholar]
56. Vartanian N, Hervochon P, Marcolte L, Larher F. Proline accumulation during drought rhizogenesis in Brassica napus var. Oleifera. J Plant Physiol. 1992;140(5):623–8. doi:10.1016/S0176-1617(11)80799-6. [Google Scholar] [CrossRef]
57. Bates LS, Waldren RP, Teare I. Rapid determination of free proline for water-stress studies. Plant Soil. 1973;39(1):205–7. doi:10.1007/BF00018060. [Google Scholar] [CrossRef]
58. Yemm E, Cocking E, Ricketts R. The determination of amino-acids with ninhydrin. Analyst. 1955;80(948):209–14. doi:10.1039/AN9558000209. [Google Scholar] [CrossRef]
59. AOAC. Official methods of analysis. In: Changes in Official Methods of Analysis Made at the Annual Meeting; 1990; VA, USA. vol. 15. [Google Scholar]
60. Shapiro SS, Wilk MB. An analysis of variance test for normality (complete samples). Biometrika. 1965;52(3/4):591–611. [Google Scholar]
61. Levene H. Robust tests for equality of variances, in contributions to probability and statistics. In: Essays in honor of Harold Hotelling. Palo Alto: Stanford University Press; 1961. p. 279–92. [Google Scholar]
62. Gomez KA, Gomez AA. Statistical procedures for agricultural research. NY, USA: John Wiley & Sons; 1984. [Google Scholar]
63. Tesfamariam EH, Annandale JG, Steyn JM. Water stress effects on winter canola growth and yield. Agron J. 2010;102(2):658–66. doi:10.2134/agronj2008.0043. [Google Scholar] [CrossRef]
64. Hu Y, Javed HH, Asghar MA, Peng X, Brestic M, Skalicktfytf M, et al. Enhancement of lodging resistance and lignin content by application of organic carbon and silicon fertilization in Brassica napus L. Front Plant Sci. 2022;13:807048. doi:10.3389/fpls.2022.807048. [Google Scholar] [PubMed] [CrossRef]
65. Sadras VO, Villalobos FJ, Orgaz F, Fereres E. Effects of water stress on crop production. In: Villalobos F, Fereres E, editors. Principles of agronomy for sustainable agriculture. Cham: Springer; 2016. p. 189–204. doi:10.1007/978-3-319-46116-8_14. [Google Scholar] [CrossRef]
66. El Sabagh A, Hossain A, Barutçular C, Islam MS, Ahmad Z, Wasaya A, et al. Adverse effect of drought on quality of major cereal crops: implications and their possible mitigation strategies. In: Hasanuzzaman M, editor. Agronomic crops: stress responses and tolerance. Germany: Springer; 2020a. vol. 3, p. 635–58. [Google Scholar]
67. El Sabagh A, Hossain A, Islam MS, Barutcular C, Ratnasekera D, Gormus O, et al. Drought and heat stress in cotton (Gossypium hirsutum L.consequences and their possible mitigation strategies. In: Agronomic crops: stress responses and tolerance. Germany: Springer; 2020b. vol. 3, p. 613–34. [Google Scholar]
68. Waraich EA, Ahmed Z, Ahmad R, Shabbir RN. Modulating the phenology and yield of Camelina sativa L. by varying sowing dates under water deficit stress conditions. Soil Environ. 2017;36:84–92. [Google Scholar]
69. Sharghi Y, Shirani rad AH, Ayene band A, Mohammadi GN, Zahedi HH, et al. Yield and yield components of six canola (Brassica napus L.) cultivars affected by planting date and water deficit stress. Afr J Biotechnol. 2011;10:9309–13. [Google Scholar]
70. Din J, Khan SU, Ali I, Gurmani AR. Physiological and agronomic response of Rapeseed varieties to drought stress. J Anim Plant Sci. 2011;21:78–82. [Google Scholar]
71. Wasaya A, Affan M, Ahmad Yasir T, Rehman A, Mubeen K, Rehman HU, et al. Foliar potassium sulfate application improved photosynthetic characteristics, water relations and seedling growth of drought-stressed maize. Atmosphere. 2021;12:663. doi:10.3390/atmos12060663. [Google Scholar] [CrossRef]
72. Islam MR, Sarker BC, Alam MA, Javed T, Alam MJ, Zaman MSU, et al. Yield stability and genotype environment interaction of water deficit stress tolerant mung bean (Vigna radiata L. Wilczak) genotypes of Bangladesh. Agronomy. 2021;11:2136. doi:10.3390/agronomy11112136. [Google Scholar] [CrossRef]
73. Arab MM, Askari H, Aliniaeifard S, Mokhtassi-Bidgoli A, Estaji A, Sadat-Hosseini M, et al. Natural variation in photosynthesis and water use efficiency of locally adapted Persian walnut populations under drought stress and recovery. Plant Physiol Biochem. 2023;201:107859. doi:10.1016/j.plaphy.2023.107859. [Google Scholar] [PubMed] [CrossRef]
74. Jabeen M, Akram NA, Ashraf M, Alyemeni MN, Ahmad P. Thiamin stimulates growth and secondary metabolites in turnip (Brassica rapa L.) leaf and root under drought stress. Physiol Plant. 2021;172(2):1399–411. doi:10.1111/ppl.13215. [Google Scholar] [PubMed] [CrossRef]
75. Islam MR, Kamal MM, Hossain MF, Hossain J, Azam MG, Akhter MM, et al. Drought tolerance in mung bean is associated with the genotypic divergence, regulation of proline, photosynthetic pigment and water relation. Phyton-Int J Exp Bot. 2022;92(3):955–81. doi:10.32604/phyton.2023.025138. [Google Scholar] [CrossRef]
76. Oksana S, Marek K, Marian B, Marek Z. Cultivar-dependent and drought-induced modulation of secondary metabolites, adaptative defense in Fagopyrum esculentum L. Physiol Mol Biol Plants. 2023;29(10):1605–18. doi:10.1007/s12298-023-01376-8. [Google Scholar] [PubMed] [CrossRef]
77. Rathore SS, Shekhawat K, Dass A, Premi OP, Rathore BS, Singh VK. Deficit irrigation scheduling and superabsorbent polymer-hydrogel enhance seed yield, water productivity and economics of Indian mustard under semi-arid ecologies. Irrig Drain. 2019;68(3):531–41. doi:10.1002/ird.2322. [Google Scholar] [CrossRef]
78. Sivapalan S. Effect of a polymer on growth and yield of soybeans (Glycine max) grown in a coarse textured soil. In: Irrigation 2001 Regional Conference; 2001; Toowoomba, Queensland, Australia. [Google Scholar]
79. Singh H. Effect of hydrogel on growth, yield and water use efficiency in pearl millet (Pennisetum glaucum) production. Forage Res. 2012;38(1):27–8. [Google Scholar]
80. Singh A, Singh AK, Aswin C. Effect of hydrogel and thiourea on yield, quality and nutrient uptake of Indian mustard under moisture stress condition. Res Crops. 2017;18(1):42. doi:10.5958/2348-7542.2017.00008.0. [Google Scholar] [CrossRef]
81. Woodhouse J, Johnson M. Effect of superabsorbent polymers on survival and growth of crop seedlings. Agric Water Manage. 1991;20(1):63–70. doi:10.1016/0378-3774(91)90035-H. [Google Scholar] [CrossRef]
82. Asayesh ZM, Vahdati K, Aliniaeifard S. Investigation of physiological components involved in low water conservation capacity of in vitro walnut plants. Sci Hortic. 2017;224:1–7. doi:10.1016/j.scienta.2017.04.023. [Google Scholar] [CrossRef]
83. Chen SL, Zommorodi M, Fritz E, Wang S, Hüttermann A. Hydrogel modified uptake of salt ions and calcium in Populus euphratica under saline conditions. Trees. 2004;18(2):175–83. doi:10.1007/s00468-003-0267-x. [Google Scholar] [CrossRef]
84. Jat AL, Rathore BS, Desai AG, Shah S. Production potential, water productivity and economic feasibility of Indian mustard (Brassica juncea) under deficit and adequate irrigation scheduling with hydrogel. Indian J Agril Sci. 2018;88(2):212–5. doi:10.56093/ijas.v88i2.79170. [Google Scholar] [CrossRef]
85. Narjary B, Aggrawal P, Chikara A, Chakraborty D, Singh R. Water availability in different soils in relation to hydrogel application. Geoderma. 2012;187:94–101. doi:10.1016/j.geoderma.2012.03.002. [Google Scholar] [CrossRef]
86. Bhardwaj AK, Shainberg I, Goldstein D, Warrington D, Levy GJ. Water retention and hydraulic conductivity of cross-linked polyacrylamides in sandy soils. Soil Sci Soc Am J. 2007;71(2):406–12. doi:10.2136/sssaj2006.0138. [Google Scholar] [CrossRef]
87. Mandal UK, Sharma KL, Venkanna K, Korwar GR, Reddy KS, Pushpanjali, et al. Evaluating hydrogel application on soil water availability and crop productivity in semiarid tropical red soil. Indian J Dryland Agril Res Dev. 2015;30(2):1–10. doi:10.5958/2231-6701.2015.00018.4. [Google Scholar] [CrossRef]
88. Akelah A. Functionalized polymeric materials in agriculture and the food industry. New York, NY, USA: Springer; 2013. doi:10.1007/978-1-4614-7061-8. [Google Scholar] [CrossRef]
89. Dai L, Wang B, An X, Zhang L, Khan A, Ni Y. Oil/water interfaces of guar gum-based biopolymer hydrogels and application to their separation. Carbohydr Polym. 2017;169:9–15. doi:10.1016/j.carbpol.2017.03.096. [Google Scholar] [PubMed] [CrossRef]
90. M’barki N, Aissaoui F, Chehab H, Dabbaghi O, del Giudice T, Boujnah D, et al. Cultivar dependent impact of soil amendment with water retaining polymer on olive (Olea europaea L.) under two water regimes. Agric Water Manage. 2019;216:70–5. doi:10.1016/j.agwat.2019.01.016. [Google Scholar] [CrossRef]
91. Xu H, Yeum KJ, Yoon YH, Ju JH. Effect of hydrogels in three substrates on growth and ornamental quality of apple mint (Mentha suaveolens) in unirrigated green roofs. J Hortic. 2019;6(3):260. doi:10.35248/2376-0354.19.06.260. [Google Scholar] [CrossRef]
92. Anjum SA, Xie X, Wang L, Saleem MF, Man C, Lei W. Morphological, physiological and biochemical responses of plants to drought stress. Afr J Agric Res. 2011;6(9):2026–32. doi:10.5897/AJAR10.027. [Google Scholar] [CrossRef]
93. Sehgal A, Sita K, Siddique KH, Kumar R, Bhogireddy S, Varshney RK, et al. Drought or/and heatstress effects on seed filling in food crops: impacts on functional biochemistry, seed yields, and nutritional quality, 2019. Front Plant Sci. 2018;9:1226. doi:10.3389/fpls.2018.01705. [Google Scholar] [PubMed] [CrossRef]
94. Herbinger K, Tausz M, Wonisch A, Soja G, Sorger A, Grill D. Complex interactive effects of drought and ozone stress on the antioxidant defence systems of two wheat cultivars. Plant Physiol Biochem. 2002;40(6-8):691–6. doi:10.1016/S0981-9428(02)01410-9. [Google Scholar] [CrossRef]
95. Khadem SA, Galavi M, Ramrodi M, Mousavi SR, Rousta MJ, Moghaddam PR. Effect of animal manure and superabsorbent polymer on corn leaf relative water content, cell membrane stability and leaf chlorophyll content under dry condition. Aust J Crop Sci. 2010;4(8):642–7. [Google Scholar]
96. Ahmed SS, Fahmy AH. Applications of natural polysaccharide polymers to overcome water scarcity on the yield and quality of tomato fruits. J Soil Sci Agric Eng, Mansoura Univ. 2019;10(4):199–208. doi:10.21608/jssae.2019.36727. [Google Scholar] [CrossRef]
97. Bano A, Yasmeen S. Role of phytohormones under induced drought stress in wheat. Pak J Bot. 2010;42(4):2579–87. [Google Scholar]
98. Ashraf M, Foolad MR. Roles of glycine betaine and proline in improving plant abiotic stress resistance. Environ Exp Bot. 2007;59(2):206–16. doi:10.1016/j.envexpbot.2005.12.006. [Google Scholar] [CrossRef]
99. Mansori M, Chernane H, Latique S, Benaliat A, Hsissou D, El Kaoua M. Seaweed extract effect on water deficit and antioxidative mechanisms in bean plants (Phaseolus vulgaris L.). J Appl Phycol. 2015;27(4):1689–98. doi:10.1007/s10811-014-0455-7. [Google Scholar] [CrossRef]
100. Michalak A. Phenolic compounds and their antioxidant activity in plants growing under heavy metal stress. Pol J Environ Stud. 2006;15(4):523–30. [Google Scholar]
101. Sultana S, Shariff MA, Hossain MA, Khatun MA, Haque R. Effect of super water absorbent (SWA) hydrogel on productivity and quality of tomato. Arch Appl Sci Res. 2016;8(10):5–9. [Google Scholar]
102. Arab MM, Brown PJ, Abdollahi-Arpanahi R, Sohrabi SS, Askari H, Aliniaeifard S, et al. Genome-wide association analysis and pathway enrichment provide insights into the genetic basis of photosynthetic responses to drought stress in Persian walnut. Hortic Res. 2022;9:1–38. doi:10.1093/hr/uhac124. [Google Scholar] [PubMed] [CrossRef]
103. Mousavi EA, Kalantari KM, Jafari S. Change of some osmolytes accumulation in water-stressed colza (Brassica napus L.) as affected by 24-epibrassinolide. Iran J Sci Technol. 2009;33:1–11. [Google Scholar]
104. Hussain M, Malik M, Farooq M, Khan MB, Akram M, Saleem MF. Exogenous glycinebetaine and salicylic acid application improves water relations, allometry and quality of hybrid sunflower under water deficit conditions. J Agron Crop Sci. 2009;195(2):98–109. doi:10.1111/j.1439-037X.2008.00354.x. [Google Scholar] [CrossRef]
105. Yang W, Li P, Guo S, Song R, Yu J. Co-application of soil superabsorbent polymer and foliar fulvic acid to increase tolerance to water deficit maize: photosynthesis, water parameters, and proline. Chil J Agric Res. 2019;79(3):435–46. doi:10.4067/S0718-58392019000300435. [Google Scholar] [CrossRef]
106. Hayat S, Hayat Q, Alyemeni MN, Wani AS, Pichtel J, Ahmad A. Role of proline under changing environments: a review. Plant Signal Behav. 2012;7(11):1456–66. doi:10.4161/psb.21949. [Google Scholar] [PubMed] [CrossRef]
107. Keyvan S. The effects of drought stress on yield, relative water content, proline, soluble carbohydrates and chlorophyll of bread wheat cultivars. J Anim Plant Sci. 2010;8(3):1051–60. [Google Scholar]
108. Anjum F, Yaseen M, Rasool E, Wahid A, Anjum S. Water stress in barley (Hordeum vulgare L.). II. Effect on chemical composition and chlorophyll contents. Pak J Agric Sci. 2003;40(1–2):45–9. [Google Scholar]
109. Waly A, El-Karamany MF, Shaban AM, Bakry AB, Elewa TA. Utilization of hydrogel for reducing water irrigation under sandy soil condition. 1-preliminary study on the effect of hydrogel on yield and yield components of sunflower and wheat under newly reclaimed sandy soil. Res J Pharm, Biol Chem Sci. 2015;6(2):1033–9. [Google Scholar]
110. Ashraf M, Shahbaz M, Ali Q. Drought-induced modulation in growth and mineral nutrients in canola (Brassica napus L.). Pak J Bot. 2013;45(1):93–8. [Google Scholar]
111. Ali Q, Ashraf M, Anwar F. Seed composition and seed oil antioxidant activity of maize under water stress. J Am Oil Chem’ Soc. 2010;87(10):1179–87. doi:10.1007/s11746-010-1599-5. [Google Scholar] [CrossRef]
112. Ali AA, Alqurainy F. Activities of antioxidants in plants under environmental stress. Lutein-Prevent Treat Dis. Trivandrum: Transworld Research Network; 2006; 187–256. [Google Scholar]
113. Singh S, Sinha S. Accumulation of metals and its effects in Brassica juncea (L.) Czern. (cv. Rohini) grown on various amendments of tannery waste. Ecotoxicol Environ Saf. 2005;62(1):118–27. doi:10.1016/j.ecoenv.2004.12.026. [Google Scholar] [PubMed] [CrossRef]
114. Nascimento V, Rauber WA, Silva GS, Siebeneichler SC, Rodrigo R. Fidelis hydrogel effects in biochemical composition of soybean grains cultivated under water deficit in Brazilian Cerrado. Commun Plant Sci. 2019;9:13–9. doi:10.26814/cps2019003. [Google Scholar] [CrossRef]
115. Karimizadeh R, Mohammadi M, Sabaghni N, Mamoodi AA, Roustami B, Sayyedi F, et al. GGE biplot analysis of yield stability in multi-environment trials of lentil genotypes under rainfed condition. Notulae Sci Biologic. 2013;5(2):256–62. doi:10.15835/nsb529067. [Google Scholar] [CrossRef]
116. Rice-Evans C, Miller N, Paganga G. Antioxidant properties of phenolic compounds. Trends Plant Sci. 1997;2(4):152–9. doi:10.1016/S1360-1385(97)01018-2. [Google Scholar] [CrossRef]
117. Canellas LP, Olivares FL, Aguiar NO, Jones DL, Nebbioso A, Mazzei P, et al. Humic and fulvic acids as biostimulants in horticulture. Sci Hortic. 2015;196:15–27. doi:10.1016/j.scienta.2015.09.013. [Google Scholar] [CrossRef]
Cite This Article
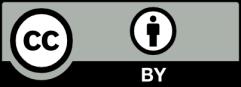
This work is licensed under a Creative Commons Attribution 4.0 International License , which permits unrestricted use, distribution, and reproduction in any medium, provided the original work is properly cited.