Open Access
ARTICLE
Experimental Exclusion of Guanaco Grazing Increases Cover, Diversity, Land Function and Plant Recruitment in Patagonia
1 INTA Instituto Nacional de Tecnología Agropecuaria, Santa Cruz, 9400, Rio Gallegos, Argentina
2 Universidad Nacional de la Patagonia Austral, Santa Cruz, 9400, Rio Gallegos, Argentina
* Corresponding Author: Carla Cepeda. Email:
(This article belongs to the Special Issue: Ecology of Rangelands in Argentina)
Phyton-International Journal of Experimental Botany 2024, 93(7), 1383-1401. https://doi.org/10.32604/phyton.2024.052534
Received 04 April 2024; Accepted 30 May 2024; Issue published 30 July 2024
Abstract
Semiarid Patagonia represents 25% of the rangeland area in Argentina, and sheep overgrazing has degraded about a third of it in the past. In this century, depleted domestic stocks have mostly stabilized, but Guanaco populations have grown. These native camelids share habitat and diets with sheep, but their effect on vegetation is poorly understood and has long been debated. We set up an exclusion experiment in Monte León and Cañadón Vaca, a semiarid shrubland grassland in southern Patagonia, currently grazed only by guanacos. Vegetation baselines were studied in 2016 in twelve plots, and half of them were protected from guanaco grazing. Thirty-six plots were cleared to study revegetation. Vegetation was reassessed in 2021, and changes were evaluated using a paired t-test. Within protected plots, vegetation cover increased, bare soil diminished, and vegetated patches grew in size, but the density of the patch-interpatch arrangement did not change. Biodiversity, measured by richness and the Shannon-Wiener (SW) index, grew significantly. Nutrient recycling increased, as did the Stability and Infiltration Land Function indexes, although these last differences were not significant. Vegetation cover under guanaco grazing also increased, mainly due to the growth of dwarf shrubs, a typical tendency in sheep grazing-induced transitions in the region. Vegetated patches under grazing fragmented, resulting in smaller patches and denser patch structures, while diversity and land function indexes remained unchanged. Young plants established in cleared plots without guanacos showed higher cover density and individual size. These effects are similar to those observed in numerous experimental sheep exclosures. Guanaco grazing may thus prevent restoration and contribute to the generalized land degradation processes that overgrazing has been causing in Patagonia for over a century.Keywords
Patagonia is a ≈1 M km2 region of woodlands and natural rangelands in southern South America. The semi-arid steppe area occupies close to 700,000 km2, mostly in Argentina east of the Andes, and represents about a quarter of the total rangeland area of the country. The domestic stock was introduced there at the end of the XIX century in large ‘estancias’, and the area endured subsequently a prolonged period of overgrazing [1] with stocks that reached 20 M sheep in 1952. A third of Patagonia rangelands were severely degraded due to high grazing pressure by the end of the last century [2], when low reproductive outputs and high sheep mortality forced producers to reduce flocks or abandon the land. Two-thirds of the sheep were lost, and only partially replaced by cattle.
The effects of high levels of sheep grazing on vegetation have been well documented in Patagonia, a region that was included in the Milchunas et al. [3] model as an example of semiarid areas with a short evolutionary history of herbivory. As predicted in this model, most sheep stocking rate and exclosure experiments in the region have found a neutral or negative effect of grazing on biodiversity [4]. High levels of grazing have also been found to reduce fecundity, reproductive effort, and plant recruitment [5]. Another effect of grazing is to modify the relative abundance of plant functional types (PFT) [6] including a global trend of increasing shrub dominance at higher grazing pressures. In Patagonia, the dominant trend under sheep grazing is an increase dwarf shrub cover, and grazing protection experiments show increases in herbaceous cover, especially in the most palatable short grasses [4]. Sheep grazing also modifies the structure of vegetation, increasing the number and size of bare soil interpatches and subdividing vegetated patches [7].
Guanaco (Lama guanicoe) is a South American native wild camelid ungulate; its populations have been impaired after sheep introduction, with great reductions in the last century; but its populations have grown in the last two decades in a context of vacant land resources and reduced hunting pressure. Total guanacos in Patagonia increased from about 500,000 in 2000 [8] to about 2 M in 2015 [9]. In this way, even when total domestic stock numbers in the last two decades have stabilized and may be close to the regional carrying capacity [10], the sustainability of the Patagonia condition characterized by depleted sheep flocks combined with increased wildlife populations remains uncertain. The situation in the southern province of Santa Cruz is particularly challenging. Provincial sheep flocks reached 7.5 M in 1937 and remained over 6 M until 1978 when two-thirds of this stock was lost in an economic, social, and natural resource crisis. The number stabilized in the present century at about 2.2 M are now competing there with a growing number of guanacos that was estimated at 1.1 M in 2015 [11] and may still be growing.
A number of studies reviewed by Shroederer et al. [12] have described vegetation traits under guanaco grazing and in general report low impacts, but they are mostly based on the description of areas under guanaco or sheep grazing [12,13], or analyze the long-term evolution of vegetation in areas used only by guanacos [14]. Stocking rate experiments such as those conducted with sheep [15,16] are, on the other hand, not possible with a highly mobile species with a large home range such as the guanaco. Experiments comparing the state and evolution of vegetation of protected vs. grazed areas are nevertheless possible, and have long been used to interpret the effects of sheep grazing in Patagonian literature [4,17] and elsewhere [18,19]. We applied the latter kind of experiments in this study, based on the construction of exclosures in areas that were subject to guanaco grazing, and monitored the subsequent changes in protected and grazed vegetation. We also included an experiment in which all the aerial vegetation is removed. Cleared, bare soil surfaces are vulnerable to erosion in Patagonia, and sexual revegetation is limited because the soil seed banks of the main perennial species are small and transient [5]. Seed must be produced and dispersed from nearby plants, find safe sites, germinate, and survive and any of these stages may be obstructed by guanaco grazing [20]. Given that the impact of grazing depends not only on the type of herbivore but also on the density of animals in relation to the productivity of the rangelands [21], this experiment included conditions with different densities of guanacos and carrying capacity of the rangelands.
Under the hypothesis that anatomical, physiological, or behavioral population regulation processes limit the impact of guanacos on vegetation, no significant differences should be expected in the evolution of vegetation in protected and grazed areas. On the contrary, if they exert a similar effect on vegetation as sheep, and based on the described effects grazing in Patagonia, protected areas should show an increase in vegetation cover, diversity, and cover of grasses and herbaceous dicots. Bare soil patches should reduce in size and land function indicators such as Recycling, Infiltration, and Stability [22] should increase. Grazed areas would be expected, on the contrary, to lose cover of grasses and herbs and gain woody dwarf-shrub cover, land function indexes should fall, bare soil patches increase, and vegetation patches should shrink and subdivide. More seedling recruits that attain higher total cover and higher size of the young plants should be expected in experimental plots of vegetation removal within guanaco exclosures about those that remain grazed.
The experimental area (Fig. 1) was located in Monte León National Park (S50 20 34.97; W68 52 47.94) and the neighboring estancia Cañadón de las Vacas (S50 32 41.37; W69 14 34.42), both in the Mata Negra biozone [23]. Climate is semiarid with mean annual temperature of 6.5°C. Rainfall records (1963–23) at Cañadón de las Vacas show a mean of 291 mm, with a coefficient of variation of 36%, and a winter maximum of 32 mm rainfall in July and a spring minimum of 15 mm in September. The landscape consists in high 400 m.a.s.l. sedimentary plateaus with Molisol type soils, of Sandy-loam texture and abundant pebbles. Vegetation in this biozone consists of “Mata negra” (Mulguraea tridens) shrublands, with patches of grass steppe of “Coirón blanco” (Festuca pallescens) and areas dominated by dwarf shrublands of “Colapiche” (Nassauvia glomerulosa). All this area was heavily grazed in the last century: maximum stocks were probably reached in 1932 in Monte León that tended about 33,400 in 62,169 ha (0.53 sheep/ha), while the 48,000 ha Cañadón de las Vacas held 17,900 sheep in 1953 (0.37 sheep/ha). In 2004 Monte León was declared a National Park, and all domestic stock was removed. Estancia Cañadón de las Vacas also removed completely sheep from the northern paddocks in 2000, so that experimental sites and have only been grazed by guanacos since.
Figure 1: Vegetation map of the study area in the Santa Cruz Province, Patagonia, Argentina, including estancia Cañadón de las Vacas in the south, and Monte León National Park in the north. Red squares identify location of experimental fences for guanaco grazing exclusion
Previous surveys and interviews with land managers indicated that guanacos had preference for grass steppes and low shrub steppes, so we selected six experimental plots in a mixed Mulguraea shrubland-grassland in northern UNO paddock in Cañadón de las Vacas and six in a dwarf shrubland-grassland in southern Jack paddock of Monte León (Fig. 1). In March 2016, in each of those two working areas, three pairs of experimental 30 m × 75 m plots were selected (total n = 12 plots) (Fig. 2).
Figure 2: Diagram of the setting of the six pairs of experimental plots with MARAS monitoring transects that include point intercept, soil and land function analysis using line transects in Cañadón de las Vacas and Monte León National Park; one plot in each pair was surrounded by a guanaco-proof fence after the initial assessment. Vegetation was completely removed in three 1 m × 2 m plots inside and outside of these exclosures in order to study natural recruitment of plants
2.1 Changes in Soil and Vegetation Variables
In the twelve selected areas, a MARAS monitoring plot [24] was installed. This methodology includes (Photo 1): (1) point intercept lines, with 500 sequential point intercepts at 20-cm intervals along two 50 m graduated lines; a needle was set in each point and perennial vascular plants were identified; (2) one 50 m gap-intercept line that record patch structure, where the length of successive vegetated patches (run on sink areas with a minimum length of 10 cm) and bare soil interpatches (runoff areas at least 5 cm long) was recorded; and (3) one 50-m gap-intercept line where soil surface condition was assessed using the LFA [22] methodology in ten interpatches. At each sampling point eleven indicators of soil surface conditions were visually estimated: (1) aerial cover for rain interception, (2) basal cover of patches, (3) litter cover, origin and degree of incorporation, (4) cryptogram cover, (5) erosion features (hummocks, desert pavements, microrills or rills), (6) deposited materials, (7) microtopography, (8) soil crust type and degree to which it is disturbed, (9) surface crust resistance, (10) slake test (time that soil aggregates retain integrity in water), and (11) texture. Three LFA indexes were estimated based on combinations of these indicators: Stability, Infiltration and Nutrient Cycling, which were calculated following [22], with adaptations to the soil and vegetation characteristics of Patagonia. See [24] to obtain further descriptions of these LFA indexes and an estimate of minimum samples and precision of Stability index.
Photo1: MARAS plots. Top: Jack paddock of Monte León National Park, a grass steppe dominated by Festuca pallescens tussock grasses, with a guanaco exclosure in the foreground. Bottom: UNO paddock of the Estancia “Cañadón de las Vacas”, a shrub steppe dominated by Mulguraea tridens shrubs and a number of dwarf shrubs
After the initial assessment of vegetation and soil using MARAS monitors one of each of the paired 30 m × 75 m plots was randomly selected, and surrounded with a 1.8 m high fence. This was an effective barrier for guanacos, but not for hares (Lepus capensis), rheas (Pterocnemia pennata) and armadillos (Zaedyus pichiy), that could graze freely within the protected plots. The impact of these lesser herbivores was not important.
2.2 Carrying Capacity Estimation (CC)
Carrying Capacity of the rangelands has been defined as the maximum density that an area can sustain without damage to natural resources [25] and to estimate it we used a procedure described in Travaini et al. 2019 [11]. Briefly, it is based on mean annual net primary production (NPP) obtained from MOD17/A3 model 2000–2015 images. Pixels 1 km × 1 km in size corresponding to the UNO and Jack paddocks were converted to Aerial Net Primary Productivity (ANPP) by means of a linear coefficient. Harvest index (the proportion of ANPP that can be sustainably consumed) was estimated using the equation HI (%) = −5.71 + 0.72 ANPP0.5, following Golluscio 2009 [26]. Consumable forage was estimated as CF = ANPP × HI. Estimated consumption for each herbivore was 500 kg DM·head-1·yr-1 (sheep) and 750 kg DM·head-1·yr-1 (guanacos) [23]. Carrying Capacity (CC) was estimated as the density of guanacos that would demand 100% of the consumable forage. Density and CC are shown as guanaco equivalents/km2 (EqG/km2). One EqG equals 1.46 Ewe equivalents in south Patagonia and in New Zealand, 2.25 dry sheep units in Australia [27], and 0.17 animal units AU in the United States [28].
2.3 Guanaco Density and Abundance Estimation
The area was sampled using line transect using DISTANCE methodology [29]. Three land transects (6 km) in the UNO paddock (2560 ha) of Cañadón de las Vacas and transects (21 km) in the Jack paddock (5900 ha) of Monte León were analyzed. Each transect was sampled three times between 2014 and 2022 in spring-summer, and five times in autumn-winter in “Cañadón de las Vacas”. In Monte León surveys were made between 2015 and 2020, three times in spring-summer and three in autumn-winter. Each guanaco group detected was registered including 1) GPS position of vehicle 2) azimuth 3) angle with respect to the transect 4) radial distance using Bushnell 10 × 25 1000 laser telemeter. Each transect was analyzed separately and all the dates were combined. Guanaco density and abundance were estimated using software Distance Sampling 7.5. Detection functions used were Half-Normal with cosine expansion for Cañadón de las Vacas, and Uniform with cosine expansion for Monte León.
In order to compare guanaco and sheep grazing pressures, Guanaco equivalents was estimated using a 100-kg guanaco that is expected to consume about 750-kg DM of forage/year [30], while a 50-kg sheep ewe would consume about 500 kg DM/year [23] so that 1 guanaco is equivalent to 1, 5 sheep.
In order to record recruitment, colonization and species establishment from seed, either from soil seed banks or from seed rain from adjacent plants, three 1 m × 2 m plots were marked within the guanaco exclosures and vegetation cover was completely removed by means of a rotocultivator (Photo 2a). The procedure was also performed in three similar plots outside the exclosures that were grazed by guanacos (Fig. 2). Plots were put in place in September 2016 and assessed in March 2021. Plant recruits were identified with colored plastic markers as grasses (including graminoids such as Carex sp), dwarf shrubs, and herbaceous dicots. Plots were photographed with a Nikon 3100, 3264 × 2448 resolution, and 35 mm lens camera. Images were rectified using Perspective Image Correction 2.0.0.8–64 Bit (GNU General Public License version 3.0 GPLv3). Young plants were digitized, and number, size and total cover was estimated for each plot (Photos 2c,d) using Quantum Gis 3.28 software [31].
Photo 2: Top: vegetation removal plots in (a) September 2016 and (b) reassessment in 2021. Down: Vegetation removal plots photographed in 2021 in conditions of (c) grazing exclusion (d) guanaco grazing. Plant recruits were identified in the field with plastic markers and their location and type (grasses, dwarf shrubs and herbaceous dicots) were marked and later digitalized to evaluate their perimeter and total cover
Plant species were classified as herbaceous/woody, and further grouped into four functional groups: shrubs, dwarf shrubs, tussocks (medium-sized, caespitose, hummock-forming grasses of genera Festuca and Stipa with low quality forage), short grasses (a variety of grasses and graminoid species mostly of good quality forage that constitute over 70% of sheep and guanaco diet), and herbs (a variety of species of herbaceous dicots).
In order to statistically analyze variables, vegetation and LFA values were expressed as percentages and were normalized using the arc sin transformation. Changes in vegetation after five years were tested with an ANOVA and given that no significant interaction was recorded in response variables between location (Monte Leon or Cañadón Vacas) and guanaco grazing, changes are shown as mean values for both locations. Initial and final values of the main variables for each plot in grazed and ungrazed areas (n = 6 in each case) were compared using the t-test for paired samples included in INFOSTAT [32], in order to check for possible significant of changes between 2016–2022. Patch and interpatch size were normalized using a log transformation and analyzed in a similar way. Mean number, size of plants, and total cover of vegetation removal plots was analyzed using 3-way ANOVA with site, pair, and guanacos as factors.
The experiment was conducted in a relatively favorable period, with a 2016–2022 mean annual rainfall of 254 mm, slightly lower than the 1963–2015 mean of 276 mm (Fig. 3), with a good starting year of 460 mm in 2017 (a factor that may have influenced seed recruitment and vegetation cover). Subsequent 2018–2020 rains were close to the historic mean and the only dry year was 2021. The previous 2006–2015 decade was drier (239 mm) than the annual average and lacked any exceptionally good year, with most rainfalls below or barely reaching the historic annual mean.
Figure 3: Rainfall in the study area between 1990 and 2023 (mm). Dotted line is the historic 1963–2015 mean (276 mm). The experimental period is marked in a box. Source: Ea Cañadón de las Vacas rain gauge
Initial vegetation cover was over 55% in both sites (Table 1), with higher litter accumulation and lower bare soil in Monte León. With a mean richness of 24 species per monitoring plot, the community was highly diverse within the context of Patagonian rangelands, that have a mean species richness of 15 [24]. Cañadón de las Vacas showed higher woody vegetation cover close to 20%, while Monte León was mostly herbaceous. Short grasses were the dominant PFT in both sites, followed by Shrubs PFT in Cañadón de las Vacas, and by Tussock grass PFT in Monte León. Landscape Function Indexes of Stability, Infiltration and Recycling were similar in both sites and close to the mean values for Patagonia of 47, 47 and 32, respectively [24]. Dwarf shrubs and herbaceous dicots cover were similar in both sites. Vegetation was structured with a great number (17 patches/10 m) of small-60 cm long patches, and also small-40 cm long interpatches. This is a fine landscape grain considering that mean density in Patagonia is 6 patches/10 m, with patches and interpatches of mean lengths of about 90 and 150 cm, respectively [24].
Vegetation cover increased and bare soil decreased significantly both in grazed and ungrazed areas but changes were more pronounced in the latter (Fig. 4). Diversity, measured by Species richness and Shannon Wiener index increased significantly only within the exclosures. With protection from grazing, herbaceous cover increased significantly, driven by two PFT: herbs (herbaceous dicots) and tussock grasses. Grazed areas gained cover of woody plants, specially dwarf shrubs, but the rest of PFT and did not change significantly.
Figure 4: The 2016 and 2021 (Initial–Final) change registered in the two experimental areas combined: Total vegetation cover (%), Bare soil (%), Species richness (N° species), Total cover of herbaceous and woody plants (%), and cover of four Plant Functional Types (%). Blue bars represent guanaco exclusion areas, and red bars are the areas that remained under guanaco grazing. Lines are standard errors of the mean, and asterisks show a statistically significant change between Initial and Final values (paired t-test, p < 0.05)
Land function indexes had a positive tendency in excluded areas, but this increase was only significant in the Recycling index (Fig. 5). Grazed areas showed non-significant changes with a neutral or negative tendency.
Figure 5: Changes between 2016 and 2021 (Initial–Final) registered in LFA (Land Function Analysis) Indexes of Stability, Nutrient Recycling and Infiltration. Density of patches (N° of patches in 10 m transect), Degree of fragmentation of patches (a measure of the degree of subdivision of vegetation cover with 100% = all cover is concentrated in one patch), and Patch and Interpatch length (cm). Blue bars represent guanaco exclusion areas and those in red areas that remain under guanaco grazing. Lines are standard error of the mean and asterisks show significant changes between initial and final values (paired t-test p < 0.05)
The density of patches (and also of interpatches) increased in the grazed area, where a larger number of smaller patches were recorded. Patch area had a higher degree of fragmentation, and interpatch length was also smaller outside the exclosures, because a number of new, small interpatches were created by fragmentation of the vegetated patch area. Within the exclosures patch density did not change and the size of patches increased with a simultaneous decrease in interpatch size and a relative decrease in patch fragmentation.
3.2 Carrying Capacity vs. Density and Abundance of Guanacos
Dwarf shrub communities in the UNO paddock of Cañadón de las Vacas showed significantly lower ANPP than those of the grass steppes of the Jack paddock of Monte León. With only around 100 g·m−2.yr−1 of net aerial productivity, Harvest Indexes were low, and guanaco Carrying Capacity estimates were 16 and 23 GEq/km2 for Cañadón de las Vacas and Monte León, respectively. Guanaco densities in Cañadón de las Vacas were also low in relation to those of Monte León, although differences were not significant. Estimated densities (guanacos/km2) were in both areas more than twice the Carrying Capacity (GEq/km2) of the land (Table 2).
Recruitment in 2021, five years after total removal of the aerial vegetation in the plots, was 242 ± 16 plants/m2 within the exclosures, significantly higher than the 182 ± 8 plants/m2 grazed areas. Three quarters of these recruits were grasses, which reached higher density, total cover and individual size in the protected plots as compared to the excluded plots (Fig. 6). Recruitment of dwarf shrubs and herbs were similar in density in the exclosure and grazed plots, but herbs reached a significantly higher total cover and individual plant size without guanacos. After five years protected plots reached 28.4 ± 2.4% of total cover, a significantly higher compared to the 16.5 ± 1.6% of total cover reached by plant recruits in grazed areas.
Figure 6: Number, total cover (%) and individual plant size (cm2) of plant recruits recorded in March 2021 in plots that had all plants removed in 2016. Plots under guanaco grazing are shown in red, and those within exclosures in blue. Lines indicate standard error of the mean (n = 12) and letters indicate significant differences between grazed and ungrazed plots (ANOVA p < 0.05)
Our results show that vegetation is clearly impacted by guanaco grazing, and this leads to a renewed debate over the contribution of native grazing in the rangeland degradation. Some authors believe that, given that guanacos have occupied these steppes during the last 10,000 yrs of the Pleistocene, they must have developed adaptations that regulate their impact on vegetation. Possible differences may be: (a) the mouth anatomy and gut physiology that enable guanacos to use the vegetation more efficiently, and consume items of lesser forage quality such as tussock grasses and shrubs [33], and (b) the fact that they show soft pads instead of hoofs present in sheep, with a smaller impact on the soil surface [34] (this assumption has not been clearly demonstrated). Additionally, other researchers [35] postulate that guanacos should naturally limit the impact on vegetation because their reproductive system, composed of polygynyc groups that have a number of females with a dominant male that actively defends a territory, would naturally regulate densities below the carrying capacity of the rangelands [36].
MARAS monitoring plots indicate an increase in vegetation cover and the decrease in bare soil observed both with or without grazing protection, may be related to the extraordinary rainfall in 2017, and the relatively good precipitation in the years following the experiment setup. Higher responses were nevertheless recorded within the exclosures (Fig. 4) with about a 10% increase that is comparable to the results observed in sheep exclosures [6,15,37].
Different plant function types explained changes in cover in and out of the exclosures (Fig. 4). Under guanaco grazing, woody plant cover increased (+4%) in line with the general trend of grazed drylands [38]. This increase was not driven by the dominant shrubs (Mulgurarea tridens), that decreased 0.1% but rather by a number of short shrubs such as Nassauvia, Perezia and Colobanthus of the Dwarf Shrub PFT (that grew 3.4%). Guanacos prefer grasses and herbs [39] because dwarf shrubs have low digestibility and spiny defenses, and their grazing may check growth of these PFT in a similar way as sheep, and prevent them from outcompeting low lying vegetation. Increased dominance of dwarf shrubs is also common under sheep grazing, and takes place in all the transitions of State and Transition models for Patagonia in areas with less than 250 mm annual rainfall [40], an increase that is associated with soil erosion and loss of soil seed banks, probably because they fail to protect the soil as efficiently as grass tillers with a caespitose growth.
Total herbaceous cover increased within exclosures (Fig. 4) as did plots that were protected from sheep grazing in the nearby Magellan Steppe [15], but with an important difference. While sheep exclosure in the Magellan Festuca grasslands lead to 25% increases in Short Grass PFT in 5 years, Tussock PFT (Festuca gracillima in this case) lost 10% cover. Sheep graze heavily on the palatable Short Grass PFT if it is available, and tend to avoid tussocks [41], so that exclusions in the Magellan Steppe experiment may have liberated this PFT that, in turn, outcompeted tussocks [15]. Guanacos also select positively short grasses, but they rely more than sheep on the coarse tussock forage [33,41], so that they may induce tussock replacement by short grasses or dwarf shrubs.
Plots protected from guanaco grazing incorporated 1–4 species (+3 sp mean) and increased in Shannon Wiener index (Fig. 4), while grazed areas remained unchanged. A revision of grazing experiments around the world [42] found that, regardless of the type of herbivores, protected plots gained about 5 species in relation to heavily grazed ones, with the most positive responses recorded in arid, low productivity systems. While herbivory may increase richness in productive habitats, the opposite effect is frequent in low-productivity habitats where water or nutrients are limiting and competition for space and light is minimal [43]. Herbivory may reduce species richness directly consuming highly palatable species or indirectly by increasing resource limitation of a few grazing-tolerant species [3]. In these conditions grazing exclusion may allow recovery and recolonization of plants, resulting in increased species richness. The impact of guanaco grazing on diversity was similar to the effect of sheep: Cesa et al. [4] studied 29 sheep exclosures in shrub-grass communities of central Patagonia and found that nearly all of them incorporated 1–10 species in relation to grazed areas, and Oliva et al. [15] found that sheep exclosures in the Magellan Steppe gained 5 species in a period of 5 years. Land function indexes (Fig. 5) increased in the exclosures, and the Nutrient Recycling index (that estimates the degree of organic matter recycling did so in a significant way (+7%). Similar, but non-significant, increases were found for Stability index (+7%) that evaluates the ability of soil to resist erosion, and also for the Infiltration index (+4%) that estimates the proportion of rainfall that penetrates the soil instead of being lost to runoff. The minimum detectable change in paired t-test for the Stability index for a 5-plot MARAS set was estimated at 14% [24] so that more time may be necessary in order to detect the changes in these indexes. Gaitán et al. [44] found similar results in mixed grass- shrubs in Northern Patagonia, where the Nutrient Recycling index in sheep exclosures was 15 points higher, and similar non-significant increases in the other two LFA indexes.
Patches in protected plots grew in size and the density of the patch-interpatch arrangement did not change (Fig. 6). Guanaco grazing on the contrary induced fragmentation of vegetated patches (+10% in Fragmentation index), that became smaller and increased in density. Fragmentation of patches is a general effect of grazing in drylands: smaller shrub patches under sheep grazing was found by Golodets and Boeken in the Negev [45] and by Bisigato and Bertiller in Patagonia [7]. Sheep grazing also reduced grass patch size in other examples in Patagonia: Oñatibia [46]. Bare soil interpatches grew significantly smaller in the exclosures, but also outside. This last result may be explained because grazing induces fragmentation of patches and generates a number of new small interpatches that are included in the computation of the mean length.
Protected plots showed a number of significant differences in relation to those grazed by guanacos: they recruited 33% more plants than those under grazing, and they showed young plants that were 58% bigger and a total plant cover that was 72% higher (Fig. 5). Given that the protected plots were cleared simultaneously with the fence establishment, the initial seed banks were similar, and differences in recruitment should be explained either by reduced seed rain in the subsequent years or by the increased seedling mortality. Mortality by direct trampling is not common under sheep grazing, even at high stocking rates [20], and has been recorded only in high intensity-short duration grazing systems [47]. Smaller seed input may, on the other hand, arise if guanacos graze culms and reproductive tissues. Reduced fecundity and soil seed bank replenishment under sheep grazing was recorded in various studies in Patagonia: the Festuca pallescens grassland [48,49] the Larrea Monte Austral [50], in Stipa grasslands [51] and the Festuca gracillima grasslands in the Magellan Steppe. Pecile [52] carried out an exclosure experiment to compare guanaco vs combined guanaco-sheep grazing in the Monte Austral of Patagonia and found similar soil seed banks but increased recruitment within exclosures independently of the type of grazing. Recolonization of cleared plots in our study was slow, and after five years the total cover was half the initial value in protected areas, and only a quarter in those subject to guanaco grazing and trampling. Timing of this cover reconstruction is crucial because disturbed areas are subject to intense wind erosion.
Ground transects show a density of around 38–51 guanacos/km2 in the paddocks that include the two experimental sites (Table 1), that seem to be particularly attractive to guanacos, as the mean density in the total area of Cañadón de las Vacas was 25 g/km2 [53] and 24 g/km2 in Monte León (Cepeda, in press). The experimental areas should be considered overgrazed, as densities are high in relation to the carrying capacity of the rangelands (16 and 23 g/km2, respectively). The grazing pressure that these guanaco densities exert are also high in relation to the historical maximum sheep stocking rates of Cañadón de las Vacas that reached the equivalent to 25 g/km2 in 1953 and Monte León, that beared 35 g/km2 in 1932. These densities are similar to those used in exclosure experiments comparisons in the Magellan Steppe by Oliva et al. [15], that used rates equivalent up to 42 g/km2 and to the 24 plots of shrub grass steppes analyzed by Cesa et al. [4] in North Patagonia that reported highest sheep rates equivalent to 40 g/km2. They were nevertheless higher than the combined sheep-guanaco densities used in the seed bank and regeneration experiments of Pecile [52] in the shrub-steppes of the Peninsula Valdez that reached about 26 g/km2. Sheep are limited by fences and guanacos are not and this may introduce a qualitative difference in their type of grazing, but this exclusion experiment indicates that grazing pressure in the tussock and dwarf shrub steppes grasslands is very high and rangelands are prone to transitions to even more degraded States.
Previous vegetation studies have considered that the impact of guanaco, even at high densities, was not important [12]. Some of these studies such as the example of Reserva San Pablo Valdez are long-term sensitive monitors similar to the MARAS system used in this study [14] but they lack of exclosures, so that slow and rainfall-dependant changes of vegetation are described without a proper control. Furthermore, a careful analysis of the data presented in this papershows that grass steppes under guanaco grazing loose 40% of cover, mostly due to loss of grasses. Other communities such as shrub-steppes show less changes, but they may be degraded states that do not change unless a grazing is relieved. Comparison with neighboring areas under different types of grazing such as in the Cabo dos Bahias site [12] are natural experiments that lack proper replications [54], and are complicated by heterogeneity in soil, topography and vegetation patch structure at different scales.
About half of the variables that we recorded including shrub and shortgrass cover, or infiltration and stability indexes failed to show significant changes in the ungrazed plots at the end of the experiment. Three possible causes of this lack of response could be 1) After a century of overgrazing, communities have lost resilience, cannot recuperate when disturbance is eased and remain in stable, degraded states 2) Five years may be a short period in order to reestablish grazing intolerant species, mostly long- lived perennials that have poor seed banks and regenerate mostly by vegetative growth 3) Guanaco grazing may not affect this particular PFT or soil traits. Further observations of these exclosures may clarify these points.
In summary, in this first experimental exclusion of guanaco, and in line with our hypothesis, we registered an increase in vegetation cover, with a special growth in tussock grasses and herbaceous dicots. Plant biodiversity also increased as rare species were reestablished, probably from soil seed banks or protected relics. These results show that rangelands are resilient and that grazing relief may start transitions to better-conserved states in the Mata Negra Shrubland, which is mostly dominated by tussock grass and shrub-tussock grass communities.
Contrary to our hypothesis, vegetation under guanaco grazing also increased, and bare soil decreased, but these changes are almost entirely explained by the growth of dwarf shrubs, a typical tendency in degradation paths of sheep grazing-induced transitions in the region. Vegetated patches constitute sink areas that retain resources and they become fragmented under grazing, giving way to a canopy with a denser structure of small patches and several new interpatches prone to erosion. Expected reductions of herbaceous vegetation, diversity, and land function indexes were not observed, and grazed areas remained mostly without changes.
After a century of heavy sheep grazing, communities in Cañadón de las Vacas and Monte León such as those in Photo 1 may be regarded as degraded stable states that are common in semiarid areas [55], and have been described elsewhere in Patagonia [15,40] Guanaco replaced sheep grazing but it seems to be preventing restoration, and guanaco-grazed communities in this study accumulated slow changes such as the observed increases in dwarf shrub cover, and increased patch fragmentation that creates new erosion sources and increments “leakiness” of landscapes that are less able to retain resources and are also typical of degraded areas [56].
Guanaco is abundant in south Patagonia but its populations have declined elsewhere in its distribution in South America. The debate about its management displays two contrasting attitudes: some stakeholders think that sheep are to blame for range degradation, not guanacos, and that their populations should be left to increase freely, ensuring the conservation of a charismatic species that should eventually establish a density in equilibrium with its resources. We have shown nevertheless that the densities that guanaco populations reach, even in the absence of sheep and in the presence of its main predator, the Puma (Felis concolor), may prevent restoration and keep rangelands in degraded states with slow, pervasive, land degradation typical of overgrazing. The main concern should not however be conservation areas or farms that have been forced to stop production such as those where our experiments took place: the real challenge is to manage guanaco populations in productive landscapes, where they share the range with sheep and where pumas are mostly absent. Joint grazing of domestic stock and native herbivores in these areas has the potential to trigger renewed degradation processes that should be avoided in Patagonia, a region that still encloses some of the finest world’s remaining natural rangelands.
Acknowledgement: Thanks to Robert and Michelle Lemaire, owners of “Cañadón de las Vacas ” estancia and their daughter Claire Lemaire that provided help and lodging in a number of opportunities. The manager of Monte León National Park, Grisel Roskos, and a number of park rangers of provided also field help, logistics and data of guanaco field surveys. Gervasio Humano, Paula Paredes, Cinthia Schenkel and Eugenia Vivar helped in the MARAS plots, and Juan Carlos Kofalt and Daniel Barría helped in fence maintenance and revegetation plots clearing. Jorge Rabinovich performed a thorough revision of this manuscript and suggested a number of changes in order to improve it.
Funding Statement: Resources for this study were obtained from INTA Projects.
Author Contributions: The authors confirm contribution to the paper as follows: study conception and design: Carla Cepeda and Gabriel Oliva; data collection: Carla Cepeda, Gabriel Oliva and Daniela Ferrante; analysis and interpretation of results: Carla Cepeda, Gabriel Oliva and Daniela Ferrante; draft manuscript preparation: Gabriel Oliva. All authors reviewed the results and approved the final version of the manuscript.
Availability of Data and Materials: Data are available upon request to the authors.
Ethics Approval: Not applicable.
Conflicts of Interest: The authors declare that they have no conflicts of interest to report regarding the present study.
References
1. Oñatibia GR. Grazing management and provision of ecosystem services in Patagonian arid rangelands. In: Ecosystem services in Patagonia: A multi-criteria approach for an integrated assessment. Springer; 2021. p. 47–74. [Google Scholar]
2. Navone SM. Desertification in Argentina: The causes and effects on human beings. In: Global degradation of soil and water resources: Regional assessment and strategies. Springer; 2022. p. 131–48. [Google Scholar]
3. Milchunas DG, Sala OE, Lauenroth WK. A generalized model of the effects of grazing by large herbivores on grassland community structure. Am Nat. 1988;132(1):87–106. doi:10.1086/284839. [Google Scholar] [CrossRef]
4. Cesa A, Paruelo JM. Changes in vegetation structure induced by domestic grazing in Patagonia (Southern Argentina). J Arid Environ. 2011;75(11):1129–35. doi:10.1016/j.jaridenv.2011.04.003. [Google Scholar] [CrossRef]
5. Funk FA, Loydi A, Peter G, Distel RA. Effect of grazing and drought on seed bank in semiarid patchy rangelands of northern Patagonia. Argentina Int J Plant Sci. 2019;180(4):337–44. doi:10.1086/702661. [Google Scholar] [CrossRef]
6. Vogel B, Rostagno CM, Molina L, Antilef M, La Manna L. Cushion shrubs encroach subhumid rangelands and form fertility islands along a grazing gradient in Patagonia. Plant Soil. 2022;475(1):623–43. doi:10.1007/s11104-022-05398-1. [Google Scholar] [CrossRef]
7. Bisigato AJ, Bertiller M. Grazing effects on patchy dryland vegetation in northern Patagonia. J Arid Environ. 1997;36(4):639–53. doi:10.1006/jare.1996.0247. [Google Scholar] [CrossRef]
8. Amaya J, von Tüngen J, de Lamo D. Informe preliminar del Proyecto de Relevamiento y Distribución de guanacos en la Patagonia. Resultados sobre la densidad de guanacos en las diferentes provincias patagónicas. In: INTA-GTZ-TÖB. Bariloche: Area de Recursos Naturales, INTA EEA; 2001. p. 13 (INTA IN de TA, editor. COMUNICACION TECNICA No 107). [Google Scholar]
9. Bay Gavuzzo A, Gaspero P, Bernardos J, Pedrana J, de Lamo D, von Thungen J. Distribution and density of guanacos (Lama guanicoe) in Patagonia. In: Report survey report 2014–2015. Bariloche: Ediciones INTA; 2015. p. 40 (In Spanish). Available from: https://www.researchgate.net/publication/308416690_Distribucion_y_densidad_de_guanacos_Lama_guanicoe_en_la_Patagonia_INFORME_RELEVAMIENTO_2014-_2015. [Accessed 2024]. [Google Scholar]
10. Oliva G, Paredes P, Ferrante D, Cepeda C, Rabinovich J. Remotely sensed primary productivity shows that domestic and native herbivores combined are overgrazing Patagonia. J Appl Ecol. 2019;56(7):1575–84. doi:10.1111/1365-2664.13408. [Google Scholar] [CrossRef]
11. Travaini A, Zapata SC, Bustamante J, Pedrana J, Zanón JI, Rodríguez A. Guanaco abundance and monitoring in Southern Patagonia: distance sampling reveals substantially greater numbers than previously reported. Zool Stud. 2015;54(1):23. [Google Scholar]
12. Schroeder NM, Rodríguez V, Marino A, Panebianco A, Peña F. Interspecific competition between guanacos and livestock and their relative impact on Patagonian rangelands: evidence, knowledge gaps, and future directions. In: Guanacos and people in Patagonia: a social-ecological approach to a relationship of conflicts and opportunities. Springer; 2022. p. 47–84. [Google Scholar]
13. Burgi MV, Marino A, Rodríguez MV, Pazos G, Baldi R. Response of guanacos Lama guanicoe to changes in land management in Península Valdés, Argentine Patagonia: conservation implications. Oryx. 2012;46(1):99–105. [Google Scholar]
14. Pazos GE, Rodriguez MV, Blanco PD. Terrestrial vegetation: description, monitoring and relation with climate and herbivores. In: Reserva de San Pablo Valdés: 10 years. Buenos Aires: Fundacion Vida Silvestre Argentina; 2017. p. 72–93, Available from: https://wwfar.awsassets.panda.org/downloads/Libro_RSPV_final. [Accessed 2024]. [Google Scholar]
15. Oliva G, Cibils A, Borrelli P, Humano G. Stable states in relation to grazing in Patagonia: a 10-year experimental trial. J Arid Environ. 1998;40:113–31. [Google Scholar]
16. Fariña CM, Cibils A, Siffredi GL, Oesterheld M, Willems PM. Efecto del pastoreo intensivo aplicado en fenofases contrastantes sobre una estepa semiárida de Patagonia Norte. Revista de Divulgación Técnica Agropecuaria, Agroindustrial y Ambiental Facultad de Ciencias Agrarias. UNLZ. 2016;3(4):2016. [Google Scholar]
17. Soriano A, Sala O, Leon R. Vegetación actual y vegetación potencial en el pastizal de coirón amargo (Stipa spp) del SW del Chubut. Bol Soc Argent Botánica. 1980;19:309–14. [Google Scholar]
18. James MB, Joe F, Mark JS, Jonathan S, Sarah JC, Steve JG, et al. A plant trait analysis of responses to grazing in a long-term experiment. J Appl Ecol. 2001;38(2):253–67. doi:10.1046/j.1365-2664.2001.00599.x. [Google Scholar] [CrossRef]
19. Yeo JJ. Effects of grazing exclusion on rangeland vegetation and soils, East Central Idaho. West North Am Nat. 2005;65(1):91–102. [Google Scholar]
20. Oliva G, Collantes M, Humano G. Reproductive effort and seed establishment in Grazed Tussock grass populations of Patagonia. Rangel Ecol Manag. 2013;66(2):164–73. doi:10.2111/REM-D-11-00121.1. [Google Scholar] [CrossRef]
21. Cingolani A, Imanual NM, Renison D, ganadería extensiva M. La ganadería extensiva, ¿es compatible con la conservación de la biodiversidad y de los suelos? Ecol Austral. 2008;18:253–71. [Google Scholar]
22. Tongway D. Rangeland soil condition assessment manual. Canberra: CSIRO, Division of Wildlife and Ecology; 1994. p. 69. [Google Scholar]
23. Behr SJ, Bottaro H, Buduba CG, Buono GG, Cesa A, Ciari G, et al. Rangeland evaluation methods in South Patagonia.Sur. Ediciones INTA. Report No.:9878333485; 2020 (In Spanish). [Google Scholar]
24. Oliva G, Dos Santos E, Sofía O, Umaña F, Massara V, García Martínez G, et al. The MARAS dataset, vegetation and soil characteristics of dryland rangelands across Patagonia. Sci Data. 2020;7(1):327. [Google Scholar] [PubMed]
25. Merrill JL. Determining carrying capacity on Rangeland to set stocking rates that will be most productive. In: Stud managers’ handbook. Boca Raton: CRC Press; 2019. vol. 18, p. 314–23. [Google Scholar]
26. Golluscio R. Receptividad ganadera: marco teórico y aplicaciones prácticas. Ecol Austral. 2009;19(3):215–32. [Google Scholar]
27. McLaren CA. Dry sheep equivalents for comparing different classes of livestock. State of Victoria, Department of Primary Industries; 1997. p. 4. [Google Scholar]
28. SRM GRSC. A Glossary of terms used in range management: a definition of terms commonly used in range management. Denver: Edison Press, Society for Range Management; 1989. p. 20. [Google Scholar]
29. Andersen EM, Steidl RJ. Power to detect trends in abundance within a distance sampling framework. J Appl Ecol. 2020;57(2):344–53. [Google Scholar]
30. Sarasqueta DV. Guanaco (Lama guanicoe) breeding and reproduction in cautivity. S.C. de Bariloche: Provincia de Río Negro, Argentina: Centro Regional Patagonia Norte INTA, EEA Bariloche; 2001. p. 78 (Comunicación Técnica N° 110 Recursos naturales-Fauna) (In Spanish). [Google Scholar]
31. Flenniken JM, Stuglik S, Iannone BV. Quantum GIS (QGISan introduction to a free alternative to more costly GIS platforms: FOR359/FR428, 2/2020. EDIS. 2020;2020(2):7. [Google Scholar]
32. di Rienzo J, Casanoves F, Balzarini M, Gonzalez L, Tablada M, Robledo C. InfoStat versión 2020. Argentina: Centro de Transferencia InfoStat, FCA, Universidad Nacional de Córdoba; 2020. [Google Scholar]
33. San Martin F, Bryant FC. Nutrition of domesticated South American Llamas and Alpacas. Small Rumin Res. 1989;2:191–216. [Google Scholar]
34. Clemente CJ, Dick TJ, Glen CL, Panagiotopoulou O. Biomechanical insights into the role of foot pads during locomotion in camelid species. Sci Rep. 2020;10(1):3856. doi:10.1038/s41598-020-60795-9. [Google Scholar] [PubMed] [CrossRef]
35. Marino A, Rodríguez V. Competitive exclusion and herbivore management in a context of livestock-wildlife conflict. Austral Ecol. 2022;47(6):1208–21. doi:10.1111/aec.13210. [Google Scholar] [CrossRef]
36. Marino A, Rodriguez M, Schroeder N. Wild guanacos as scapegoat for continued overgrazing by livestock across southern Patagonia. J Appl Ecol. 2020;57(12):2393–98. [Google Scholar]
37. Funk FA, Peter G, Loydi A, Kröpfl AI, Distel RA. Structural and functional recovery of interspaces between shrubs after 10 years of grazing exclusion in a semiarid steppe of northwest Patagonia. Ecol Austral. 2012;22(3):195–202 (In Spanish). [Google Scholar]
38. Liu YX, Cui X, Chen J, Chen X. Mechanisms, monitoring and modeling of shrub encroachment into grassland: a review. Int J Digit Earth. 2019;12(6):625–41. doi:10.1080/17538947.2018.1478004. [Google Scholar] [CrossRef]
39. Baldi R, Pelliza-Sbriller A, Elston D, Albon S. High potential for competition between guanacos and sheep in Patagonia. J Wildl Manag. 2004;68(4):924–38. doi:10.2193/0022-541X(2004)068[0924:HPFCBG]2.0.CO;2. [Google Scholar] [CrossRef]
40. Bertiller MB, Bisigato A. Vegetation dynamics under grazing disturbance. The state-and-transition model for the Patagonian steppes. Ecol Austral. 1998;8(2):191–9. [Google Scholar]
41. Bonino N, Pelliza Sbriller A. Comparación de las dietas del guanaco, ovino y bovino en Tierra del Fuego. Argentina Turrialba. 1991;41:452–2. [Google Scholar]
42. Herrero-Jáuregui C, Oesterheld M. Effects of grazing intensity on plant richness and diversity: a meta-analysis. Oikos. 2018;127(6):757–66. [Google Scholar]
43. Johnson CA, Bronstein JL. Coexistence and competitive exclusion in mutualism. Ecology. 2019;100(6):1–8. [Google Scholar]
44. Gaitán JJ, López CR, Bran DE. Efectos del pastoreo sobre el suelo y la vegetación en la estepa patagónica. Cienc Suelo. 2009;27(2):261–70. [Google Scholar]
45. Golodets C, Boeken B. Moderate sheep grazing in semiarid shrubland alters small-scale soil surface structure and patch properties. CATENA. 2006;65(3):285–91. [Google Scholar]
46. Oñatibia GR, Boyero L, Aguiar MR. Regional productivity mediates the effects of grazing disturbance on plant cover and patch-size distribution in arid and semi-arid communities. Oikos. 2018;127(8):1205–15. [Google Scholar]
47. Salihi D, Norton B. Survival of perennial grass seedlings under intensive grazing in semi-arid rangelands. J Appl Ecol. 1987;24:145–51. [Google Scholar]
48. Bertiller M. Seasonal variation in the seed bank of a Patagonian grassland in relation to grazing and topography. J Veg Sci. 1992;3:47–54. [Google Scholar]
49. Bertiller MB. Grazing effects on sustainable semiarid rangeiands in Patagonia: the state and dynamics of the soil seed bank. Environ Manage. 1996;20(1):123–32. [Google Scholar]
50. Bertiller MB, Ares JO. Does sheep selectivity along grazing paths negatively affect biological crusts and soil seed banks in arid shrublands? A case study in the Patagonian Monte. Argentina J Environ Manage. 2011;92(8):2091–6. [Google Scholar] [PubMed]
51. Ghermandi L. Seasonal patterns in the seed bank of a grassland in north-western Patagonia. J Arid Environ. 1997;35(2):215–24. [Google Scholar]
52. Pecile MV. Efectos del pastoreo de animales silvestres y domésticos sobre la regeneración de pastizales de la estepa patagónica (Ph.D. Thesis). Facultad de Agronomía, Universidad de Buenos Aires: Argentina; 2019. [Google Scholar]
53. Oliva G, Paredes P, Ferrante D, Cepeda C, Rabinovich J. Are Patagonia grasslands being overgrazed? A response to Marino et al. (2020). J Appl Ecol. 2020;57(12):2399–405. [Google Scholar]
54. Hurlbert SH. Pseudoreplication and the design of ecological field experiments. Ecol Monogr. 1984;54:187–211. [Google Scholar]
55. Laycock W. Stable states and thresholds of range condition on North American rangelands: a viewpoint. J Range Manag. 1991;44(5):427–33. [Google Scholar]
56. Bastin GN, Ludwig JA, Eager RW, Chewings VH, Liedloff AC. Indicators of landscape function: comparing patchiness metrics using remotely-sensed data from rangelands. Ecol Indic. 2002;1(4):247–60. doi:10.1016/S1470-160X(02)00009-2. [Google Scholar] [CrossRef]
Cite This Article
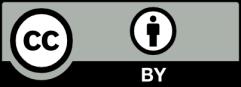
This work is licensed under a Creative Commons Attribution 4.0 International License , which permits unrestricted use, distribution, and reproduction in any medium, provided the original work is properly cited.