Open Access
ARTICLE
Physiological and Biochemical Responses of Perennial Ryegrass Mixed Planting with Legumes under Heavy Metal Pollution
College of Animal Science, Guizhou University, Guiyang, 550025, China
* Corresponding Author: Chao Chen. Email:
(This article belongs to the Special Issue: Abiotic Stress Impacts on Plant Physiology and Their Alleviation)
Phyton-International Journal of Experimental Botany 2024, 93(7), 1749-1765. https://doi.org/10.32604/phyton.2024.051793
Received 15 March 2024; Accepted 17 June 2024; Issue published 30 July 2024
Abstract
In artificially controlled pot experiments, perennial ryegrass was mixed with other leguminous plants (white clover and alfalfa) and treated with lead, zinc and cadmium (337 mg·kg, 648 mg·kg, and 9 mg·kg, respectively) to simulate compound pollution conditions. The results showed that the concentrations of heavy metals, transport factors, and bioconcentration factors in mixed planting of ryegrass decreased compared with those in monoculture. Regardless of whether heavy metal pollution was introduced, mixed planting increased the aboveground and underground biomasses of ryegrass. The different mixed planting treatments had no significant impact on the chlorophyll concentration of ryegrass. The mowing time, mixed planting treatment, and heavy metal treatment had impacts on antioxidant and osmotic adjustment substances, and there were some interactions. The mixed planting treatment did not significantly affect glutathione concentration, cysteine concentration, or nonprotein thiol. Mixed planting generally increased the nitrogen and phosphorus concentrations of ryegrass while reducing the stoichiometric ratio of carbon, nitrogen, and phosphorus. These results suggest that the mixed planting of ryegrass with legumes promotes the growth of ryegrass in the presence of high concentrations of heavy metal pollution. However, it does not enhance the ability of ryegrass to remediate heavy metal pollution in the soil.Keywords
The rapid development of industry, agriculture, and transportation has been highly convenient for meeting human living standards but has also caused environmental pollution [1]. The discharge of three types of wastes (waste gas, wastewater, and industrial residue), the development and utilization of mineral resources, the use of chemical fertilizers and pesticides containing heavy metals, or the unreasonable application of fertilizers can lead to severe air, soil, and water pollution, leading to environmental degradation and harm to society, the economy and human beings. For soil heavy metal pollution, once excess heavy metals enter the soil, they are difficult to exclude. There are many kinds of pollutants in the soil, including inorganic pollutants such as Cd, Hg, As, Pb, Cr, Cu, Zn, Ni, Mn, Co, Se, V, Sb, Ti, and Mo [2].
Currently, the remediation methods for heavy metal pollution in soils mainly include microbial remediation and phytoremediation, as well as joint remediation [3]. However, due to the nature of the microorganisms themselves, the use of this technology is limited. First, microbial genetic stability is poor, microbes can easily change, and microbes have difficulty removing all pollutants [4]. Second, although microorganisms can adsorb and enrich heavy metal ions, they compete with indigenous microbial strains and may be eliminated due to competitive exclusion [5]. Third, microbial remediation technology is vulnerable to the external environment [6]. Phytoremediation is a green remediation technology in which green plants are used to transfer, accommodate or transform contaminants [7]. It has the advantages of being low cost and can easily control secondary pollution. After vegetation forms, it can protect the soil, reduce erosion and soil loss, and be applied in mine reclamation, vegetation restoration, and landscape restoration at heavy metal polluted sites; however, it also has several limitations. There are few hyperaccumulators in nature. The hyperaccumulators that are screened from the wild generally have low biomass, short stature, and slow growth and are affected by climate, soil, and other factors, resulting in low effectiveness and long cycles, which restrict their large-scale application [8].
Herbage plants are highly resistant to heavy metals, and their biomass is very high. Although these plants are not classified as hyperaccumulators, they are often used to remediate heavy metal-contaminated soil [9]. Perennial ryegrass (Lolium perenne L.), which belongs to the Poaceae family, has multiple uses. It serves as a valuable plant for both pasture and forage and is also commonly used to create attractive lawns in landscapes and sports fields [10]. Additionally, perennial ryegrass is widely utilized in pollution remediation and exhibits strong tolerance to barren land, organic matter, sewage, etc. Perennial ryegrass can also survive or even grow normally in areas with poor habitats. Through repeated trimming of perennial ryegrass, the heavy metal ion concentration in the soil can be gradually reduced, facilitating the remediation of contaminated soil [11].
The intercropping or mixed planting of Fabaceae and Poaceae plants is a traditional farming method. Many studies have shown that intercropping plants of these two families allow the plants to make full use of light, heat, water, gas and other resources [12]. Therefore, intercropping or mixed planting can improve the land utilization rate and promote high yields of plants through the use of the physiological characteristics of different plants [13]. Intercropping has been proposed as an alternative to phytostabilization for heavy metal-contaminated soil and has many advantages [14,15]. It has been reported that intercropping cannot only increase plant biomass but also change the accumulation of heavy metals in plants [16]. Intercropping alleviates the inhibitory effect of heavy metals on plant growth and increases the nitrogen and chlorophyll concentrations in the shoots and roots. Moreover, in a previous study, the Pb concentrations in the shoots and roots of ryegrass and alfalfa in the intercropping systems were significantly lower than those in monocultures [17]. Intercropping can increase the resistance of plants to heavy metals by reducing plant oxidative damage and increasing antioxidant activity. In this study, ryegrass was mixed with white clover (Trifolium repens L.) and alfalfa (Medicago sativa L.) in different treatments in soil contaminated with lead, zinc and cadmium to explore whether the mixed planting of ryegrass and Fabaceae could improve the ability of these plants to remediate highly heavy metal contaminated soil.
2.1 Plant Material and Growth Conditions
The experimental seeds for perennial ryegrass cv.Yaqing, white clover cv. Haifa, and alfalfa cv. Suntory were purchased from Zhongzhiheng Ecological Technology Co., Ltd. in Guiyang, Guizhou, China. The test soil was collected from the test site at the West Campus of Guizhou University. Topsoil from a depth of less than 20 cm was collected, mixed and air-dried for further analysis (Table 1). Tap water was used for irrigation. The heavy metal additives used were analytically pure Pb(NO3)2, Zn(NO3)2·6H2O and Cd(NO3)2·4H2O, which were purchased from Sinopharm Chemical Reagent Co., Ltd., Ningbo, China.
In October 2021, the natural air-dried soil was placed in rectangular plastic pots with dimensions of 48 cm × 33 cm × 26 cm (length × width × height), and each pot was filled with 21 kg of soil. Pb(NO3)2, Zn(NO3) 2·6H2O and Cd(NO3)2·4H2O were added to the soil, and the Pb, Zn and Cd concentrations were 337 mg·kg−1, 648 mg·kg−1 and 9 mg·kg−1, respectively. The Pb, Zn, and Cd pollution concentrations were set based on the heavy metal concentration in the soil of Xinguanzhai (a gathering place for local indigenous zinc smelting), Hezhang County, Guizhou Province, China [18]. At the same time, 50 g·m−2 compound fertilizer (total nutrient content ≥ 64%, the ratio of N, P2O5 and K2O was 18:46:0) was added as the base fertilizer. The mixed potted soil was placed in a greenhouse for 5 months and mixed periodically to ensure thorough and even mixing.
Eight different treatments were carried out (Table 2), with three replicates for each treatment. For the monoculture and the mixed planting of two kinds of plants, there were four rows of plants per pot. For the mixed planting of three kinds of plants, there were three rows of plants per pot. There were 30 seedlings in each row.
Seeds were sown on 26 March, 2022. The seeds Disinfected with 10% H2O2, rinsed with water, and then sown. After the seeds emerged, 30 plants were retained in each row. The distance between the pots was 20 cm. The pots were placed outdoors in a completely randomly arrangement, and the positions of the pots were periodically changed to reduce edge effects. Irregular watering was used to maintain the field water capacity of the soil at approximately 60%. After 2 months of plant growth (when the plants were approximately 20 cm high), the first mowing was carried out. The leaves were then cut once every other month for a total of three times (26th May, 26th June, 26th July) during the experiment, and the physiological indices of the fresh leaves were measured during the third mowing. The leaf C, N, and P concentrations; plant root parameters; and heavy metal concentrations were determined.
2.3 Determination Indices and Methods
Plant samples were separated into aboveground and underground parts. The soil attached to the plant samples was rinsed off with tap water, and then the plants were rinsed with deionized water two or three times. An EPSON V800 scanner was used to scan the root system, and a Win RHIZO 2007 root analysis system was used to analyze the total length, total surface area, total volume, average root diameter and total amount of root tips. Some of the fresh samples were stored in an ultralow temperature refrigerator for measurement of physiological indices. Some of the samples were de-enzymed at 105°C for 30 min and then dried to constant weight at 70°C. The oven-dried plant samples were weighed, crushed, and sieved (100 mesh nylon sieve) for later testing.
The activities of superoxide dismutase (SOD), peroxidase (POD), and catalase (CAT) and the concentrations of malondialdehyde (MDA), proline (Pro), soluble sugar (SS), soluble protein (SP), glutathione (GSH), cysteine (Cys), and nonprotein thiol (NPT) were determined using commercial kits purchased from Beijing Solabao Technology Co., Ltd., Beijing, China.
The chlorophyll concentrations were determined using the methods of the mixed solvents of ethanol and acetone [19,20].
The plant total carbon (C), nitrogen (N) and phosphorus (P) concentrations were determined by using the potassium dichromate-sulfuric acid oxidation method [21], the semi-micro Kjeldahl method [22] and molybdenum antimony resistance colorimetry [23], respectively.
The oven-dried plant samples were digested using HNO3-HClO4 (4:1, v/v) to analyze the concentrations of metals. The heavy metal concentration was determined using an atomic absorption spectrophotometer [24].
The transport factor (TF) is used to calculate the ability of plants to transfer heavy metals from their roots to aboveground parts. The TF of heavy metals was calculated according to shoot/root heavy metal concentration ratios in plants. The bioconcentration factor (BCF) is defined as the ratio of the metal concentration in plants to the metal concentration in soil and is also known as the enrichment factor. This parameter provides information on the absorption of metals by plants. The BCF was calculated as the ratio between the metal concentration in plant tissues and that in nutrient solutions [25].
All statistical analyses were carried out in SPSS 20.0 (SPSS Inc., Chicago, IL, USA). The differences in parameters among different treatments were analyzed using one-way ANOVA and multivariate analysis of variance with the least significant difference (LSD) test at the 0.05 significance level. All bar graphs were prepared in Origin 2023 (Origin Lab, Hampton, USA).
3.1 Accumulation Characteristics of Heavy Metals in Perennial Ryegrass
The mowing time and mixed planting treatment significantly affected the concentration of heavy metals in ryegrass, but there was no interaction between the mowing time and mixed planting treatment (Fig. 1). Compared with that in the monoculture treatment, the concentration of heavy metals in the mixed planting treatment decreased (Fig. 2). Mixed planting with white clover or alfalfa significantly reduced the concentrations of Pb, Zn, and Cd in the shoots of ryegrass.
Figure 1: The heavy metal concentrations in perennial ryegrass shoot of different mowing times
Note: *p < 0.05, **p < 0.01, ***p < 0.001”, NS: not significant. “PL”, “PLT”, “PLM” and “PLTM” represent “perennial ryegrass monoculture”, “mixed-planting perennial ryegrass and white clover”, “mixed-planting perennial ryegrass and alfalfa”, “mixed-planting perennial ryegrass, white clover, and alfalfa” under heavy metal pollution treatments, respectively. Different capital letters above the histograms indicate significant difference between mowing time, different lowercase letters above the histograms indicate significant difference between planting treatments (p < 0.05).
Figure 2: The heavy metal concentrations in perennial ryegrass
Note: Different lowercase letters in the figure indicate significant differences between different treatments (p < 0.05). “PL”, “PLT”, “PLM” and “PLTM” represents “perennial ryegrass monoculture”, “mixed-planting perennial ryegrass and white clover”, “mixed-planting perennial ryegrass and alfalfa”, “mixed-planting perennial ryegrass, white clover, and alfalfa” under heavy metal pollution treatments, respectively.
There were differences in the heavy metal transport factors of ryegrass under the different planting treatments (Table 3). The Pb and Cd transport factors were less than one, while the Zn transport factor was generally greater than one (except for mixed planting with alfalfa). Compared with monoculture, mixed planting reduced the heavy metal transport factors of ryegrass. When ryegrass and alfalfa were mixed, the transport factors of the three heavy metals were the lowest. When the three plants were mixed, the transport factors of the three heavy metals were the highest (Table 3).
The concentrations of heavy metals in the shoots of ryegrass differed among the different mixed planting treatments, but the difference in heavy metal concentrations in the roots was not significant (Table 4). Compared with monoculture, mixed planting reduced the heavy metal concentration factor in ryegrass. When mixed with white clover or alfalfa, the shoot bioconcentration factor of ryegrass decreased significantly (Table 4).
3.2 Toxic Effects of Heavy Metals on Perennial Ryegrass
3.2.1 Biomass and Roots of Perennial Ryegrass under Different Planting Treatments
Regardless of whether heavy metal pollution was present, the mixed planting increased the aboveground and underground biomasses of ryegrass (Fig. 3). When the three plants were mixed, the biomass was the highest among all the treatments, and the biomass of ryegrass was 1.44 times greater (uncontaminated) and 0.88 times greater (contaminated) than that of monoculture plants. Among the uncontaminated treatments, ryegrass plants had the highest root-to-shoot ratio. Under contaminated treatments, the root-to-shoot ratio was greatest for ryegrass mixed with white clover.
Figure 3: Biomass and root-to-shoot ratio of perennial ryegrass plants under the different planting treatments
Note: Different lowercase letters in the figure indicate significant differences between different treatments (p < 0.05). “L”, “LT”, “LM” and “LTM” represents “perennial ryegrass monoculture”, “mixed-planting perennial ryegrass and white clover”, “mixed-planting perennial ryegrass and alfalfa”, “mixed-planting perennial ryegrass, white clover, and alfalfa” without heavy metal pollution treatments, respectively. “PL”, “PLT”, “PLM” and “PLTM” represents “perennial ryegrass monoculture”, “mixed-planting perennial ryegrass and white clover”, “mixed-planting perennial ryegrass and alfalfa”, “mixed-planting perennial ryegrass, white clover, and alfalfa” under heavy metal pollution treatments, respectively.
The plants grown in uncontaminated soil, the maximum average root diameter, total root surface area, total root volume, and total root tip number occurred in the mixed planting system with ryegrass and alfalfa. Under the contaminated treatment, mixed planting increased the average root diameter of ryegrass compared with monoculture. In the same type of planting, the average root diameter under contaminated treatment was greater than that under uncontaminated treatment, but the total number of root tips under contaminated treatment was lower than that under uncontaminated treatment (Fig. 4).
Figure 4: Root characteristics of perennial ryegrass plants under different planting treatments
Note: Different lowercase letters in the figure indicate significant differences between different treatments (p < 0.05). “L”, “LT”, “LM” and “LTM” represents “perennial ryegrass monoculture”, “mixed-planting perennial ryegrass and white clover”, “mixed-planting perennial ryegrass and alfalfa”, “mixed-planting perennial ryegrass, white clover, and alfalfa” without heavy metal pollution treatments, respectively. “PL”, “PLT”, “PLM” and “PLTM” represents “perennial ryegrass monoculture”, “mixed-planting perennial ryegrass and white clover”, “mixed-planting perennial ryegrass and alfalfa”, “mixed-planting perennial ryegrass, white clover, and alfalfa” under heavy metal pollution treatments, respectively.
3.2.2 Physiological Indices of Perennial Ryegrass Plants under Different Planting Treatments
Mowing time and heavy metal treatment significantly affected the chlorophyll concentration of ryegrass, while the different mixed planting treatments had no significant impact on the chlorophyll concentration. However, mowing time and mixed planting treatment had an interaction on chlorophyll concentration (Fig. 5).
Figure 5: The chlorophyll concentration in perennial ryegrass under different treatments
Note: *p < 0.05, **p < 0.01, ***p < 0.001, and “NS” represents “ not significant”. “L”, “LT”, “LM” and “LTM” represents “perennial ryegrass monoculture”, “mixed-planting perennial ryegrass and white clover”, “mixed-planting perennial ryegrass and alfalfa”, “mixed-planting perennial ryegrass, white clover, and alfalfa” without heavy metal pollution treatments, respectively. “PL”, “PLT”, “PLM” and “PLTM” represent “perennial ryegrass monoculture”, “mixed-planting perennial ryegrass and white clover”, “mixed-planting perennial ryegrass and alfalfa”, “mixed-planting perennial ryegrass, white clover, and alfalfa” under heavy metal pollution treatments, respectively. Different capital letters above the histograms indicate significant difference between mowing time, different lowercase letters above the histograms indicate significant difference between planting treatments (p < 0.05).
Mowing time, mixed planting treatment, and heavy metal treatment had impacts on antioxidant substances (Fig. 6) and osmotic adjustment substances (Fig. 7), and there were some interactions. Mowing time significantly affected all indices. The mixed planting treatment did not significantly affect the MDA or soluble sugar concentration. Heavy metal treatment did not significantly affect CAT activity.
Figure 6: The antioxidant substances in perennial ryegrass under different treatments
Note: *p < 0.05, **p < 0.01, ***p < 0.001, NS: not significant. “L”, “LT”, “LM” and “LTM” represents “perennial ryegrass monoculture”, “mixed-planting perennial ryegrass and white clover”, “mixed-planting perennial ryegrass and alfalfa”, “mixed-planting perennial ryegrass, white clover, and alfalfa” without heavy metal pollution treatments, respectively. “PL”, “PLT”, “PLM” and “PLTM” represent “perennial ryegrass monoculture”, “mixed-planting perennial ryegrass and white clover”, “mixed-planting perennial ryegrass and alfalfa”, “mixed-planting perennial ryegrass, white clover, and alfalfa” under heavy metal pollution treatments, respectively. Different capital letters above the histograms indicate significant difference between mowing time, different lowercase letters above the histograms indicate significant difference between planting treatments (p < 0.05).
Figure 7: The osmotic adjustment substances in perennial ryegrass under different treatments
Note: *p < 0.05, **p < 0.01, ***p < 0.001”, and “NS” represents “not significant”. “L”, “LT”, “LM” and “LTM” represents “perennial ryegrass monoculture”, “mixed-planting perennial ryegrass and white clover”, “mixed-planting perennial ryegrass and alfalfa”, “mixed-planting perennial ryegrass, white clover, and alfalfa” without heavy metal pollution treatments, respectively. “PL”, “PLT”, “PLM” and “PLTM” represent “perennial ryegrass monoculture”, “mixed-planting perennial ryegrass and white clover”, “mixed-planting perennial ryegrass and alfalfa”, “mixed-planting perennial ryegrass, white clover, and alfalfa” under heavy metal pollution treatments, respectively. Different capital letters above the histograms indicate significant difference between mowing time, different lowercase letters above the histograms indicate significant difference between planting treatments (p < 0.05).
3.3 Effect of Heavy Metal Detoxification on Perennial Ryegrass
Mowing time significantly affected GSH, Cys, and NPT. The mixed planting treatment did not significantly affect GSH, Cys, or NPT. Heavy metal treatment significantly affected Cys and the NPT. Mowing time and heavy metal treatment had interactive effects on Cys and NPT (Fig. 8).
Figure 8: The heavy metal detoxification indices in perennial ryegrass under different treatments
Note: *p < 0.05, **p < 0.01, ***p < 0.001”, NS: not significant. “L”, “LT”, “LM” and “LTM” represents “perennial ryegrass monoculture”, “mixed-planting perennial ryegrass and white clover”, “mixed-planting perennial ryegrass and alfalfa”, “mixed-planting perennial ryegrass, white clover, and alfalfa” without heavy metal pollution treatments, respectively. “PL”, “PLT”, “PLM” and “PLTM” represent “perennial ryegrass monoculture”, “mixed-planting perennial ryegrass and white clover”, “mixed-planting perennial ryegrass and alfalfa”, “mixed-planting perennial ryegrass, white clover, and alfalfa” under heavy metal pollution treatments, respectively. Different capital letters above the histograms indicate significant difference between mowing time, different lowercase letters above the histograms indicate significant difference between planting treatments (p < 0.05).
3.4 Elemental Distribution of Perennial Ryegrass under Different Planting Treatments
The C, N, and P concentrations in the aboveground parts of the ryegrass were generally greater than those in the underground parts (Table 5). Under the different treatments, the C, N and P concentrations of ryegrass differed, but the change in P concentration was not significant. Under the uncontaminated treatments, mixed planting significantly increased the N concentration in the aboveground part of ryegrass (p < 0.05) compared with monoculture, and the mixed planting of the three plants significantly increased the C concentration in the underground part of ryegrass (p < 0.05). Under the contamination treatments, the C concentration in the aboveground part of ryegrass significantly decreased (p < 0.05). Under the same type of planting, the aboveground C concentration of the ryegrass grown in contaminated soil was lower than those grown in uncontaminated soil, and the aboveground N and P concentrations and underground C and N concentrations of the ryegrass grown in contaminated soil were greater than those grown in uncontaminated soil. The aboveground C:N concentration of ryegrass was positively correlated with the underground C:N concentration (p < 0.01). There was a significant negative correlation between underground C:N and underground N:P (p < 0.01).
4.1 Effects of Mixed Planting with Fabaceae Plants on the Heavy Metal Enrichment Ability of Perennial Ryegrass
Interplanting or mixed planting is a traditional agricultural practice. Different mixed planting treatments drive the diversity of soil biodiversity and ecological functions through different root characteristics, thus affecting the absorption and transport of heavy metals by plants. The interaction between the Zn hyperaccumulator Thlaspi caerulescens and the nonhyperaccumulator Thlaspi arvense in soil supplemented with ZnO or ZnS showed that intercropping significantly increased the Zn uptake of the hyperaccumulator and decreased the Zn uptake of the nonhyperaccumulator [26]. Wang et al. showed that when alfalfa was interplanted with ryegrass, interplanting increased the uptake of Pb, Zn and Cd, especially Cd and Zn, by ryegrass [27]. Cui et al. suggested that interplanting significantly reduced the Pb concentration in the shoots and roots of ryegrass and alfalfa compared with monocultures [17]. The results of this study showed that, in terms of simulated Pb-Zn-Cd pollution, compared with monocultures ryegrass, mixed planting resulted in lower Pb, Zn and Cd concentrations in the shoots of ryegrass, and the Pb, Zn and Cd concentrations were the highest among the three mixed planting treatments in the shoots of ryegrass.
4.2 Effects of Heavy Metal Pollution on Plant Growth and Physiological Characteristics
When plants are under stressed due to heavy metals pollution, their growth status can directly reflect their resistance to heavy metals and degree of stress. Usually, under adverse stress conditions, the size of underground roots increases to improve plant water absorption capacity, reduce the quantity of dry matter of aboveground stems and leaves, reduce transpiration, and subsequently reduce water loss to adapt to adverse conditions [28]. In this study, when ryegrass was mixed with white clover and alfalfa, white clover and alfalfa met their needs for nitrogen through biological nitrogen fixation. Perennial ryegrass grows quickly, which is advantageous for competing for nutrients, and the biomass of mixed-planting ryegrass is high. The root-shoot ratio of ryegrass plants under mixed planting (except mixed planting with white clover) was lower than that under monoculture. The mixed planting of leguminous plants and gramineous plants can not only improve the utilization of light, temperature, water and nutrients but also improve the soil structure, soil biodiversity and nutrient availability, ultimately promoting the growth of plants and increasing total plant output [29]. In this study, the aboveground and underground biomass of ryegrass increased in response to the mixed planting of ryegrass, white clover and alfalfa. The reason may be that white clover and alfalfa meet their needs for nitrogen through biological nitrogen fixation, and the fixed nitrogen was transferred to adjacent ryegrass plants.
Photosynthesis is an important way for plants to obtain energy, and there is a very close relationship between plant energy content and photosynthesis. Previous studies have shown that under Pb2+ stress, the chlorophyll concentration of ryegrass was significantly greater than that in the control group, while under Cd2+ stress, the chlorophyll concentration in ryegrass significantly decreased [30]. Under Pb, Zn, Cd, Pb-Zn and Cu-Cd stress, the chlorophyll concentration of perennial ryegrass and tall fescue decreased significantly, while under Cu stress alone, the chlorophyll concentration of perennial ryegrass and tall fescue increased [31]. In this study, the chlorophyll a and b and total chlorophyll concentrations in the mixed planting treatment group were obviously greater than those in the monoculture treatment group at the first mowing. The increase in the chlorophyll concentration may be due to a change in the light requirements of ryegrass plants after mixed planting, which improved the full utilisation of light by ryegrass plants. However, at the third mowing, the chlorophyll concentration of ryegrass generally decreased under contaminated treatment. The decrease in the chlorophyll concentration in ryegrass may be due to the accumulation of heavy metals at certain concentrations of Pb, Zn and Cd, which disrupts the synthesis of chlorophyll and affects the activity of chlorophyllase, thus inhibiting the biosynthesis of chlorophyll and causing a decrease in chlorophyll concentration.
Oxidative damage is an important mechanism in plants under heavy metal stress. Under heavy metal stress, reactive oxygen species (ROS) in plants are overproduced and accumulate [32]. High levels of ROS generally destroy auxiliary cellular components, such as the cell membrane, nucleic acids and chlorophyll [33], causing oxidative damage and affecting the normal growth and development of plants. Low concentrations of heavy metals can activate the antioxidant management system of plants; increase the activity of the antioxidant proteases SOD, POD and CAT; and remove active oxygen species in plants [34]. The induction of antioxidant enzymes is an important protective mechanism for reducing oxidative discolouration damage; SOD increases during induction, catalyzing the conversion of superoxide anions to H2 and O2; H2O2 is also a free radical that can also cause oxidative damage to cell membranes. POD and CAT can catalyze the production of nontoxic water and oxygen molecules from hydrogen peroxide, maintain free radicals in plants at a low level, and promote the avoidance of oxidative damage caused by free radicals in plants. These enzymes are key protective enzymes for plant metabolism under adverse conditions [35]. In this study, during the first mowing, mixed planting increased the activities of SOD, POD and CAT in ryegrass plants under the uncontaminated treatment. Under the contamination treatment, the activities of SOD and CAT in ryegrass decreased, while the activity of POD increased. During the third mowing, mixed planting had no significant effect on the SOD, POD or CAT activity in ryegrass under the uncontaminated treatment. Under the contamination treatments, mixed planting decreased the SOD activity of ryegrass. Except for the three kinds of mixed plantings, the changes in the other mixed plantings were not obvious, and the CAT activity generally increased (except for the mixed plantings with white clover). The decrease in SOD activity may be attributed to the toxic effects of heavy metals on ryegrass after mixed planting. In addition, the interaction of different plant root exudates among mixed plants leads to the destruction of various functional enzymes and enzyme systems in plant tissues and cells, resulting in decreased accumulation of active oxygen and free radicals and decreased enzyme synthesis. The increase in POD activity may be related to the concentration of the substrate for the POD enzyme; that is, when heavy metal ions enter plants, the amount of toxic substances produced in plants increases rapidly, which increases the substrate concentration of the POD enzyme and may also be related to the expression of specific genes and cell aging. The decrease in CAT activity during the first mowing of ryegrass may have occurred due to the toxicity of heavy metals to plants under the given conditions, but the toxicity of heavy metals to the roots was lower. It is also possible that CAT and POD were mainly used to scavenge H2O2 produced during oxygen metabolism and the organic peroxide ROOH produced from it. Combined with the results showing a change in POD activity, these results showed that CAT and POD in ryegrass play complementary roles in catalyzing H2O2 to form H2O under Pb-Zn-Cd stress. MDA is the final product of membrane lipid peroxidation. Under the stimulation of the external environment, plants imbalance the degree of membrane lipid peroxidation and produce many reactive oxygen species that are not conducive to plant growth [36].
Pro is an important osmoregulatory substance. Under stress, the synthesis of free amino acids is strengthened, and free proline regulates plant cells through osmosis, thus improving the ability of plants to resist stress and protecting their own mechanisms from maximum damage [37]. Under heavy metal stress, due to the presence of heavy metal ions, the external osmotic potential decreases, and the water in plant cells is lost to the outside with low osmotic potential, resulting in plant water shortages and common plant damage [38]. An increase in the Pro concentration can reduce the osmotic potential in plant cells and improve the water-holding capacity of plants, thus preventing water shortage. One mechanism by which many plants and algae respond to toxic heavy metals and detoxify plants is the synthesis of Pro. In this study, the Pro concentration in the mixed planting treatment did not change obviously compared with that in the monoculture under the uncontaminated treatment, but the Pro concentration in the mixed planting treatment decreased under the contaminated treatment. The main reason for the decrease in the Pro concentration was that mixed planting increased the stress from heavy metals on plants, and mixed planting increased the damage to the plant cell membrane system; thus, the amount of osmotic adjustment substances that stimulate plant production decreased.
Soluble proteins can participate in the osmotic adjustment in plant cells, thus improving the stress resistance of plants. Generally, under unfavorable conditions, due to the acceleration of protein decomposition, protein synthesis is inhibited, and the concentration and quality of proteins in plants decrease [39]. In this study, during the first mowing, the soluble protein concentration of ryegrass decreased with mixed planting, regardless of whether heavy metal contaminants were present. At the third mowing, the soluble protein concentration did not change obviously under the uncontaminated treatment, but compared with that under the monoculture, the soluble protein concentration under the mixed planting decreased under the contaminated treatment. The decrease in the soluble protein concentration under the contamination treatment may have occurred due to the chelation reaction of soluble protein caused by excessive heavy metals in plant cells. The chelation of soluble proteins and heavy metals reduces the heavy metal concentration in plants, which leads to damage caused by heavy metals. This is a self-protection mechanism of cells.
Soluble sugars are small molecules that regulate cell osmotic pressure. Plants can increase the concentrations of soluble sugars and other organic substances under adverse conditions so that the osmotic pressure of cells can be adjusted, and the ability of plants to adapt to adverse conditions can be improved [40]. In this study, under the contaminated treatment, the soluble sugar concentration decreased at the first mowing of ryegrass but increased at the third mowing. The main reason for the decrease in soluble sugar concentration was that mixed planting increased the stress from heavy metals on plants, and mixed planting increase the damage to the plant cell membrane system, thus reducing the amount of osmotic regulatory substances that stimulate plant production. The increased in soluble sugar concentration may have occurred due to the water deficit in plants under lead and zinc stress, which results in increased starch hydrolysis in leaves, a decreased output of photosynthetic products and an accumulation of sugars.
4.3 Effect of Heavy Metal Pollution on Plant Sulfhydryl Compounds
Cys is the initial product of sulfate assimilation and the rate-limiting factor of glutathione synthesis. Cys synthesis may be involved in the detoxification of Cd in plants [41]. In this study, the Cys concentration after the first mowing of ryegrass was greater than that after the third mowing. Compared with that in ryegrass monoculture, the concentration of Cys in ryegrass increased in response to mixed planting under the uncontaminated treatment, and the concentration of Cys was lower under the contaminated treatment.
Sulfhydryl compounds play an important role in plant resistance to metal stress. These compounds can promote the synthesis of NPT induced by various metals and metalloids in cells, and their defense against metal toxicity is mainly achieved by the chelation of metal ions [41]. In this study, the NPT concentration of ryegrass in the third mowing was lower than that in the first mowing. The concentration of NPT was generally greater under contaminated treatment than under uncontaminated treatment. An increase in the NPT concentration promotes the synthesis of low-molecular-weight sulfhydryl compounds, such as GSH and phytocomplexin (PC), which participate in the detoxification of heavy metals in the form of metal mercaptans.
GSH can protect plants from oxidation and inactivation. These compounds have antioxidative, integrative and detoxifying effects and participate in the elimination of plant toxins [41]. In this study, the GSH concentration of ryegrass after the third mowing was lower than that after the first mowing. Under the same mixed planting pattern, the GSH concentration of ryegrass under the contaminated conditions increased or decreased but did not reach a significant level. Studies have shown that heavy metal stress can cause two effects on GSH: one is to stimulate the synthesis of GSH, and the other is to stimulate phytochelatin (PC) synthesis under heavy metal stress, which leads to the consumption of GSH [42,43]. Therefore, the decrease in the GSH concentration may be caused by the rapid synthesis of PCs under heavy metal stress, which temporarily downregulates GSH in plants.
4.4 Effect of Heavy Metal Pollution on Phytochemical Parameters
C, N and P are essential nutrient elements for plant growth and play important roles in the regulation of plant growth and physiological mechanisms [44]. In this study, compared with those in the monoculture treatment, the C concentration in the aboveground part of the ryegrass mixed planting system decreased, but the N and P concentrations in the aboveground part and the C, N, and P concentrations in the underground part increased. Under the Pb-Zn-Cd pollution treatment, the C concentration in the aboveground part and the N and P concentrations in the underground part of ryegrass in mixed plantings decreased, but the C and P concentrations in the underground part increased except when ryegrass was mixed planting with white clover. The decrease in the C concentration may have occurred due to the competition between the plants in mixed plantings for light, which led to a lower accumulation of C during photosynthesis than in monoculture. White clover and alfalfa, as leguminous plants, have a good nitrogen fixation abilities and can provide N for other plants. An increase in the P concentration may increase plant resistance and promote growth. Under mixed planting and heavy metal stress, plants compete for nutrients to maintain normal growth. Moreover, plants with well-developed roots exhibit strong competitiveness under heavy metal stress [45].
C is a structural element of plants and generally does not restrict plant growth, while N and P easily become limiting factors in plant growth. C:N and C:P can reflect the growth rate of plants and the rate of nutrient utilization, respectively [46]. The N:P ratio can reflect the relative utilization rate of N and P by plants and subsequently predict deficits in N and P. Higher C:N and C:P ratios indicate that plants have greater utilization efficiency of N and P. In this study, mixed planting generally increased the C:P and N:P ratios in the shoots and the C:N and N:P ratios in the shoots of ryegrass but decreased the C:N ratio in the shoots compared with the monoculture under uncontaminated treatment. Compared with those in the monoculture, the C:N and N:P ratios in the shoots in the mixed planting treatment decreased under the contaminated treatment. When N:P < 14, plant growth is limited by N; when N:P > 16, plant growth is limited by P; and when 14 < N:P < 16, plant growth is or is not limited by N and P together [47].
The ability of plants to absorb and transport heavy metals varies with species and planting area. This is mainly because the genetic structure of a plant itself determines its ability to absorb or transport metal elements, but this ability strengthens or weakens with changes in the environment. In this study, the total biomass of ryegrass was greater in the mixed planting treatment than in the monoculture treatment under simulated Pb-Zn-Cd pollution. Mixed planting reduced the concentrations of Pb, Zn and Cd, transport factors and bioconcentration factors of Pb, Zn and Cd in the aboveground and underground parts of ryegrass, which indicated that under high concentrations of heavy metal pollution, the mixed planting of ryegrass with legumes promoted the growth of ryegrass but did not improve its ability to remediate heavy metal pollution in the soil.
Acknowledgement: We would like to thank Lili Zhao for her technical assistance during the experiments.
Funding Statement: This work was funded through projects of the National Key Research and Development Program of China (2023YFD1301401). Cheng Wei received the grant. Ministry of Science and Technology of the People’s Republic of China (https://www.most.gov.cn/index.html, accessed on 19/03/2024). And the Guizhou Provincial Science and Technology Projects (QKHPTRC-CXTD[2022]1011). Chao Chen received the grant. Guizhou Provincial Department of Science and Technology (https://kjt.guizhou.gov.cn/, accessed on 19/03/2024).
Author Contributions: The authors confirm contribution to the paper as follows: study conception and design: Yi Xi, Yanhong Xu, Chao Chen data collection: Yanhong Xu, Li Zhang analysis and interpretation of results: Li Zhang, Yi Xi, Yanhong Xu, Wei Cheng, Chao Chen, draft manuscript preparation: Li Zhang, Yi Xi, Wei Cheng, Chao Chen. All authors reviewed the results and approved the final version of the manuscript.
Availability of Data and Materials: All data were uploaded as supporting information files accompanying the manuscript.
Ethics Approval: Not applicable.
Conflicts of Interest: The authors declare that they have no conflicts of interest to report regarding the present study.
References
1. Yu H, Zahidi I. Environmental hazards posed by mine dust, and monitoring method of mine dust pollution using remote sensing technologies: an overview. Sci Total Environ. 2023;864:161135. [Google Scholar] [PubMed]
2. Wang X, Xiang X. Environmental risks for application of magnesium slag to soils in China. Agric Sci China. 2020;19(7):9. [Google Scholar]
3. Wu Y, Li X, Yu L, Wang T, Wang J, Liu T. Review of soil heavy metal pollution in China: spatial distribution, primary sources, and remediation alternatives. Resour Conserv Recy. 2022;181:106261. [Google Scholar]
4. Shi K, Cheng WM, Cheng DL, Xue JL, Qiao YL, Gao Y, et al. Stability improvement and the mechanism of a microbial electrolysis cell biocathode for treating wastewater containing sulfate by quorum sensing. Chem Eng J. 2023;455(1):140597. [Google Scholar]
5. Gao S, Zhang M, Liang J, Teng T, Huo X. Bioremediation strategies for petroleum removal and bacterial communities in loess under 10°C. Environ Eng Sci. 2019;36(9):1258–68. [Google Scholar]
6. Zhang G, Gao YF, Ren R, Guo X, Xing B, Li Y, et al. Enhanced biological stabilization of metal-chelant complexes in the chelator-washed soils by sulfate-reducing bacteria. J Soil Sediment. 2023;23(6):2457–72. [Google Scholar]
7. Wang JY, Delavar MA. Techno-economic analysis of phytoremediation: a strategic rethinking. Sci Total Environ. 2023;902:165949. [Google Scholar] [PubMed]
8. Xu W, Du Q, Yan S, Cao Y, Liu X, Guan DX, et al. Geographical distribution of As-hyperaccumulator Pteris vittata in China: environmental factors and climate changes. Sci Total Environ. 2022;803:149864. [Google Scholar] [PubMed]
9. Zhao J, Xia B, Meng Y, Yang Z, Zhang X. Transcriptome analysis to shed light on the molecular mechanisms of early responses to cadmium in roots and leaves of King Grass (Pennisetum americanum × P. purpureum). Int J Mol Sci. 2019;20(10):2532. [Google Scholar] [PubMed]
10. Safari S, Nazari F, Vafaee Y, Teixeira da Silva JA. Impact of rice husk biochar on drought stress tolerance in perennial ryegrass (Lolium perenne L.). J Plant Growth Regul. 2023;42(2):810–26. [Google Scholar]
11. Jiang N, Li Z, Yang J, Zu Y. Responses of antioxidant enzymes and key resistant substances in perennial ryegrass (Lolium perenne L.) to cadmium and arsenic stresses. BMC Plant Biol. 2022;22(1):1–15. [Google Scholar]
12. Wang D, Zhou W, He Y, Tang S, Yin L, Zhao X, et al. Intercropping and N application enhance soil dissolved organic carbon concentration with complicated chemical composition. Soil Till Res. 2021;210:104979. [Google Scholar]
13. Li QS, Chen J, Wu LK, Luo XM, Li N, Arafat Y, et al. Belowground interactions impact the soil bacterial community, soil fertility, and crop yield in maize/peanut intercropping systems. Int J Mol Sci. 2018;19(2):622. [Google Scholar] [PubMed]
14. Wan X, Lei M, Chen T, Intercropped Pteris vittata L, Morus alba L. Intercropped Pteris vittata L. and Morus alba L. presents a safe utilization mode for arsenic-contaminated soil-sciencedirect. Sci Total Environ. 2017;579:1467–75. [Google Scholar] [PubMed]
15. Zhan FD, Qin L, Guo XH, Tan JB, Liu NN, Zu YQ, et al. Cadmium and lead accumulation and low-molecular-weight organic acids secreted by roots in an intercropping of a cadmium accumulator Sonchus asper L. with Vicia faba L. Rsc Adv. 2016;6(40):33240–48. [Google Scholar]
16. Lin LY, Yan XL, Liao XY, Zhang YX, Ma X. Arsenic accumulation in Panax notoginseng monoculture and intercropping with Pteris vittata. Water Air Soil Pol. 2015;226(4):113. [Google Scholar]
17. Cui T, Fang L, Wang M, Shen G. Intercropping of gramineous pasture ryegrass (Lolium perenne L.) and leguminous forage alfalfa (Medicago sativa L.) increase the resistance of plants to heavy metals. J Chem. 2018;11:7803408. [Google Scholar]
18. Li Z, Feng X, Bi X, Sun G, Liu T. Present situation of soil heavy metals contamination in an artisanal zinc-smelting zone of Guizhou. China J Ecol. 2011;30(5):897–901. [Google Scholar]
19. Arnon DI. Copper enzymes in isolated chloroplasts: polyphenoloxidase in Beta vulgaris. Plant Physiol. 1949;24(1):1–15. [Google Scholar] [PubMed]
20. Zhang XZ. Determination of plant chlorophyll content: acetone-ethanol mixture method. Liaoning Agric Sci. 1986;3:26–8. [Google Scholar]
21. Cai YF, Peng C, Qiu S, Li YT, Gao YH. Dichromate digestion-spectrophotometric procedure for determination of soil microbial biomass carbon in association with fumigation-extraction. Commun Soil Sci Plan. 2011;42(22):2824–34. doi:10.1080/00103624.2011.623027. [Google Scholar] [CrossRef]
22. Menefee SG, Overman OR. A semimicro-kjeldahl method for the determination of total nitrogen in milk. J Dairy Sci. 1940;23(12):1177–85. doi:10.3168/jds.S0022-0302(40)92829-6. [Google Scholar] [CrossRef]
23. Li HJ. Comparative study on determination of phosphorus content in two kinds of plants. Mod Agric Sci Technol. 2012;11:16–7. [Google Scholar]
24. Danielson BG, Oberg PA. An integrating atomic absorption spectrophotometer for ultramicroanalysis of metals. Anal Biochem. 1974;62(2):327–40. doi:10.1016/0003-2697(74)90165-1. [Google Scholar] [PubMed] [CrossRef]
25. Nissim WG, Palm E, Mancuso S, Azzarello E. Trace element phytoextraction from contaminated soil: a case study under mediterranean climate. Environ Sci Pollut Res Int. 2018;25(9):9114–31. doi:10.1007/s11356-018-1197-x. [Google Scholar] [PubMed] [CrossRef]
26. Whiting SN, Leake JR, Mcgrath SP, Baker AJ. Hyperaccumulation of Zn by Thlaspi caerulescens can ameliorate Zn toxicity in the rhizosphere of cocropped Thlaspi arvense. Environ Sci Technol. 2001;35(15):3237–41. [Google Scholar] [PubMed]
27. Wang K, Huang H, Zhu Z, Li T, Alva A. Phytoextraction of metals and rhizoremediation of PAHs in co-contaminated soil by co-planting of Sedum alfredii with ryegrass (Lolium perenne) or castor (Ricinus communis). Int J Phytoremediat. 2013;15(3):283–98. [Google Scholar]
28. Roychoudhury A, Roy C, Sengupta DN. Transgenic tobacco plants over expressing the heterologous lea gene Rab16A from rice during high salt and water deficit display enhanced tolerance to salinity stress. Plant Cell Rep. 2007;26(10):1839–59. [Google Scholar] [PubMed]
29. Liu J, Ge X, Fan X, Liu H, Ren A. The inhibitory effect of endophyte-infected tall fescue on white clover can be alleviated by glomus mosseae instead of rhizobia. Microorganisms. 2021;9(1):109. [Google Scholar] [PubMed]
30. Howladar SM. A novel Moringa oleifera leaf extract can mitigate the stress effects of salinity and cadmium in bean (Phaseolus vulgaris L.) plants. Ecoto Environ Safe. 2014;100:69–75. [Google Scholar]
31. Lou Y, Zhao P, Wang D, Amombo E, Zhuge Y. Germination, physiological responses and gene expression of tall fescue (Festuca arundinacea Schreb.) growing under Pb and Cd. PLoS One. 2017;12(1):e0169495. [Google Scholar] [PubMed]
32. Schutzendubel A, Polle A. Plant responses to abiotic stresses: heavy metal-induced oxidative stress and protection by mycorrhization. J Exp Bot. 2002;53(372):1351–65. [Google Scholar] [PubMed]
33. Tewari RK, Kumar P, Sharma PN, Bisht SS. Modulation of oxidative stress responsive enzymes by excess cobalt. Plant Sci. 2002;162(3):381–8. [Google Scholar]
34. Srivastava S, TripathiI RD, Dwivedi UN. Synthesis of phytochelatins and modulation of antioxidants in response to cadmium stress in Cuscuta reflexa-an angiospermic parasite. J Plant Physiol. 2004;161(6):665–74. [Google Scholar] [PubMed]
35. Hu Z, Xie Y, Jin G, Fu J, Li H. Growth responses of two tall fescue cultivars to Pb stress and their metal accumulation characteristics. Ecotoxicology. 2015;24(3):563–72. [Google Scholar] [PubMed]
36. Catola S, Marino G, Emiliani G, Huseynova T, Musayev M, Akparov Z, et al. Physiological and metabolomic analysis of Punica granatum L. under drought stress. Planta. 2016;243(2):441–9. [Google Scholar] [PubMed]
37. Hessini K, Jeddi K, Siddique K, Chaieb M. Physiological and biochemical responses of Lawsonia inermis L. to heavy metal pollution in arid environments. S Afr J Bot. 2021;143:7–16. [Google Scholar]
38. Zhao H, Jun T, Zheng W. Effects of Cu2+ stress on the growth and some physiological characteristics of Kandelia candel seedlings. Mar Sci. 2016;40(4):65–72. [Google Scholar]
39. Zhang X, Yang H, Du T. Osmotic adjustment of tomato under mild soil salinity can enhance drought resistance. Environ Exp Bot. 2022;202:105004. [Google Scholar]
40. Wang L, Wang G, Cui J, Wang X, Li M, Qi X, et al. Transcriptomics, metabolomics, antioxidant enzymes activities and respiration rate analysis reveal the molecular responses of rice to Cd stress and/or elevated CO2 concentration. Plant Soil. 2022;485(1–2):259–80. [Google Scholar]
41. Yang X, Wang C, Huang Y, Liu B, Liu Z, Huang Y, et al. Foliar application of the sulfhydryl compound 2,3-dimercaptosuccinic acid inhibits cadmium, lead, and arsenic accumulation in rice grains by promoting heavy metal immobilization in flag leaves. Environ Pollut. 2021;285:117355. [Google Scholar] [PubMed]
42. Rola K, Latkowska E, Ogar W, Osyczka P. Towards understanding the effect of heavy metals on mycobiont physiological condition in a widespread metal-tolerant lichen Cladonia rei. Chemosphere. 2022;308(2):136365. [Google Scholar] [PubMed]
43. Zhang J, Li J, Lin Q, Huang Y, Chen D, Ma H, et al. Impact of coconut-fiber biochar on lead translocation, accumulation, and detoxification mechanisms in a soil-rice system under elevated lead stress. J Hazard Mater. 2024;469:133903. [Google Scholar] [PubMed]
44. Sardans J, Rivas-Ubach A, Penuelas J. The elemental stoichiometry of aquatic and terrestrial relationships with organismic lifestyle and ecosystem structure and function: a review and perspectives. Biogeochemistry. 2012;111:1–39. [Google Scholar]
45. Ma CJ, Ming H, Lin CH, Naidu R, Bolan N. Phytoextraction of heavy metal from tailing waste using Napier grass. CATENA. 2016;136:74–83. [Google Scholar]
46. Wang Y, Zhao Z, Jiang G. Effects of phosphate rock on absorption and accumulation of heavy metals of ryegrass in heavy metal contaminated soil. Appl Mec Mater. 2014;522:752–7. [Google Scholar]
47. Koerselman W, Meuleman AFM. The vegetation N:P ratio: a new tool to detect the nature of nutrient limitation. J Appl Ecol. 1996;33(6):1441–50. [Google Scholar]
Cite This Article
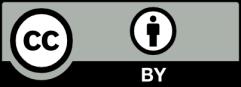
This work is licensed under a Creative Commons Attribution 4.0 International License , which permits unrestricted use, distribution, and reproduction in any medium, provided the original work is properly cited.