Open Access
REVIEW
Organ Abscission in Plants: With Special Emphasis on Bell Pepper
1 Life Sciences Division, Universidad de Guanajuato, Irapuato, 36500, México
2 School of Engineering and Sciences, Tecnologico de Monterrey, Querétaro, 76130, México
* Corresponding Author: Héctor G. Núñez-Palenius. Email:
(This article belongs to the Special Issue: Recent Research Trends in Genetics, Genomics, and Physiology of Crop Plants)
Phyton-International Journal of Experimental Botany 2024, 93(7), 1467-1484. https://doi.org/10.32604/phyton.2024.051644
Received 12 March 2024; Accepted 17 June 2024; Issue published 30 July 2024
Abstract
Bell pepper (Capsicum annuum L.), along with potato and tomato, is one of the three most cultivated vegetables in the world. Bell pepper is worldwide accepted due to its characteristics of color, smell, flavor, and texture. Bell pepper is also considered a nutritious food due to its vitamin and antioxidant contents. In Mexico, bell pepper production has a high value because it is grown for the international markets, particularly the United States of America. Nevertheless, the abscission of flowers and fruits is a factor that limits the yield, hinders the planning of activities, and causes a variation in the prices of peppers. Due to the importance of this process, in this work we did a bibliometric analysis and literature review of scientific advances for the understanding of the abscission process in plants, and in particular for the bell pepper. Finally, we introduce new perspectives that would help direct future research about organ abscission in plants. Likewise, the lack of research that would further clarify abscission process in the bell pepper plant is discussed.Keywords
Plant reproductive organ abscission is an essential biological process and is an evolutionary mechanism generated in response to stress or environmental changes, because of external and internal signal induction or growth stages [1]. Horticulture can benefit from both enhancing or blocking abscission depending on the grower’s needs [2].
Premature abscission (separation of plant organs) of flowers and fruits is a factor that limits the yield in horticultural crops [3]. Bell pepper crops, like other indeterminate greenhouse vegetable crops, present strong fluctuations in fruit harvesting patterns, in which periods of high yield alternate with periods of low yield [4,5]. The variability in the production and yield of bell peppers poses a challenge for the grower to plan activities throughout the production cycle. These yield fluctuations can also affect the price of bell peppers during the growing season [6].
Abscission in vegetable crops is related to factors including temperature, drought stress [7,8], reduced light conditions [9,10], and limited pollination [11]. However, bell pepper plants may also present abortion of flowers and young fruits in non-limiting conditions, probably due to internal competition for nutrients (macro and micronutrients) [5].
A precise search into the studies focused on plant organ abscission will allow us to examine the evolution and attention given to the subject over the years. Accordingly, we carried out a simple research to quantify published scientific articles in the Scopus® scientific platform using the keywords: organ abscission/plants (Fig. 1) and were able to determine that the publications on this issue increased considerably from 2005 to 2023.
Figure 1: Scientific publications on plant organ abscission by year, from 1972 to 2024 based on the Scopus® scientific platform according to the following keywords: organ abscission/plants
The parametrization was done of scientific studies related to abscission in plants, the network visualized can be attributed to the keywords that appear most frequently in each published article (Fig. 2). These keywords served to identify the focus of the bibliographic study, which helps in providing answers and solutions to research problems [12]. The characterization of global network mapping was established and depicted the bibliographic map of keyword concurrence frequency using the scientific platform Scopus®.
Figure 2: VOSviewer global network map about the bibliographic occurrence on abscission-related topics, considering at least three keyword concurrences in documents published from 1972 to 2024. Utilizing the scientific platform Scopus®, with reference to the keywords: organ abscission/plants. 257 keywords in 6 groups were taken into consideration; the largest group is made up of 68 words
An image for the keyword’s organ abscission/plants (Fig. 2) and another one for Abscission/Capsicum (Fig. 3) are portrayed.
Figure 3: VOSviewer frequency of co-occurrence keywords taking into account two as the minimum number of occurrences of a keyword, from 1972 to 2024. Based on the scientific platform Scopus®, with reference to the following keywords: abscission/capsicum. 73 keywords in 6 groups were taken into consideration; the largest group is made up of 42 elements
The main keywords associated with the abscission process were “Arabidopsis,” “abscission,” “flowers,” “metabolism,” and “gene expression regulation.” The findings from this search suggest that most of the studies about abscission were carried out in the model plant Arabidopsis and have focused on flower abscission. These results also indicated that this topic was extensively studied in tomato, citrus, sweet pepper, litchi, and Lupinus. Most of these studies were achieved on tomato crops followed by sweet peppers. Regarding plant growth regulators (PGRs), ethylene has the highest frequency, followed by auxins and abscisic acid (Fig. 2).
A similar search within the Capsicum genus, which included the keywords “Capsicum annuum”, “bell pepper”, “pepper”, “abscission”, and “ethylene”, indicated several studies on bell pepper, abscission, and ethylene, in the second and third most important, respectively; Capsicum frutescens was also present, although with less importance (Fig. 3).
This review article focuses on the abscission process in plants, integrating the advances carried out up to date to comprehend this phenomenon. The abscission of fruits and flowers of bell pepper, because of its great importance for the crop, is emphasized.
Organ abscission is a critical process during the different stages of a plant’s phenology. The abscission process ensures that undesired organs are separated from the main body of the plant [13,14]. Abscission is described as a genetically programmed phenomenon in plant growth that facilitates the detachment of unneeded, aging, or impaired body parts, including leaves, petals, whole flowers, inflorescences, fruits, and clusters, and occasionally even infected parts of the plants [15,16]. Therefore, abscission is the mechanism through which plant’s vegetative and reproductive organs detach due to hormonal, environmental, and developmental cues. The factors that lead to this process involve adverse environmental and internal conditions, like drought or limited nutrition [17]. The shedding of flower organs in Arabidopsis thaliana has been the primary model for comprehending abscission at the molecular and genetic levels. The combination of direct and reverse genetic methods has revealed a set of essential components for abscission. In addition, recent advancements in biochemistry have generated new insights into the molecular mechanisms at play. The abscission process can be segmented into four distinct stages. Initially, the abscission zone is formed, followed by the activation of abscission signaling. Subsequently, enzymatic hydrolysis of the middle lamella within the abscission zone occurs, leading finally to elongation and increased volume of the AZ cells. And fourth, the abscission protective layer differentiates and completely seals the separated cell layers [18,19].
In agriculture, the control of abscission is vital as it affects yield and crop quality [20]. In some crops, abscission can have relevant yield implications. For example, in litchi plants, abscission may occur in approximately 95% of flowers, causing less than 5% to reach fruit maturity. Similarly, in bell peppers, between 67% and 81% of fruitlets are aborted. Abscission also affects quality and post-harvest storage in some crops [21,22].
The abscission process occurs in a specific cell area, named the abscission zone (AZ). The AZ is often band-shaped and develops at the beginning of organ development [23]. The cells within the AZ typically exhibit a smaller size compared to the surrounding cells and contain densely packed cytoplasm. Additionally, within the AZ, the bonds of the cell wall are separated in the middle lamella (the pectin layer responsible for joining adjacent cells) [23]. Once the abscission process starts, cells in the AZ expand, and the middle lamella is dissolved by hydrolytic enzymes, which allows cell separation. Thus, a new protective epidermic layer may grow to protect exposed cells at the abscission location [24].
Recent advances in genetic, molecular, and biochemical methods have brought about a better understanding of separation process. Besides PGRs, additional molecules (IDA peptide, hydrogen peroxide) that play a crucial role in events during abscission were identified [25].
The initiation of the AZ development, which may eventually lead to abscission, commences during the early stages of organ development. Certain genetic aspects of floral organ AZ development in Arabidopsis have indicated the necessity of the BLADE-ON-PETIOLE 1/2 (BOP1/2) transcription factors for its formation [26]. The homeodomain transcription factor BREVIPEDICELLUS (BP) serves to prevent excessive enlargement of the AZ in floral organs of Arabidopsis [27], while the MYB transcription factor ASYMMETRIC LEAVES1 (AS1) is responsible for establishing the positioning of the AZ in floral organs [28]. The A. thaliana Homeobox Gene1 (ATH1), a BELL-type transcription factor, is essential for the location and development of the stamen AZ [29]. In tomato plants, a MADS-domain transcription factor, such as JOINTLESS, is necessary for the formation of the pedicel AZ [30,31]. Even though the MADS-domain transcription factors regulate the activation of abscission in Arabidopsis, it has not been demonstrated that they regulate the development of floral organ or leaf AZ [32,33].
The mechanisms governing the initiation of abscission signaling have been predominantly elucidated in the model plant, Arabidopsis. The signaling events during the activation phase of abscission commence with the expression of enzymes that modify the cell wall, leading to the dissolution of the pectin-rich middle lamella of the AZ. In Arabidopsis, the mutant of Inflorescence Deficient in Abscission (IDA) fails to undergo abscission, and its AZ cannot be differentiated [34]. However, it is probable that the signaling elements involved in the initiation stage of abscission also contribute to the ultimate formation of the protective layer within the AZ [34]. For example, the overexpression of IDA, a gene essential for abscission initiation, leads to excessive scar differentiation in the AZ.
The AZ in yellow lupine flowers (Lupinus luteus) has a particularly active transcriptional network at specific stages, as reflected by the expression profile of LIBOP [35]. Transcripts of LIBOP were detected in the cytoplasm and nucleus of the cell AZ. After abscission, cells AZ accumulate reactive oxidative species and are stimulated by an increasing quantity of uridine-rich small nuclear RNA, along with a heightened synthesis of poly(A) mRNA. LIBOP plays the role of a mobile key regulator for abscission.
2.2 Advances in Flower and Fruit Abscission
The research on the plant-abscission process has focused mainly on fruit crops, including litchi (Litchi chinensis), mango (Mangifera indica), citrus crops, grapes (Vitis vinifera), apple (Malus domestica) and peach (Prunus persica); fruiting vegetables, mainly tomato (Solanum lycopersicum); and the Arabidopsis plant (Arabidopsis thaliana). These studies have permitted the identification of genes, peptides, and PGRs involved in the entire process (Table 1) [36].
In woody plants, the existing knowledge on flower and fruit abscission has focused primarily on the effects of environmental stresses, which are related to this process [13]. Many of these environmental factors alter PGR balances and/or carbohydrate metabolism (Table 1), but the precise process are not fully understood. PGRs serve as the abscission effectors, of which the of which the equilibrium of ethylene and auxins is particularly important. The balance between photosynthesis and reserve mobilization is responsible for the intricate regulation of the carbohydrate equilibrium [6]. PGRs and carbohydrates are integral components of complex signal transduction systems, particularly during stress responses.
2.2.1 Primary and Secondary Abscission
The process of primary abscission in poinsettia (Euphorbia pulcherrima) inflorescences, as compared to the non-abscising wild-type pea mutant, reveals that in poinsettia, the primary abscission process initiates at the epidermis around the pedicel. As the AZ develops towards the center of the pedicel, the pedicel continues to grow through cell division and cell elongations of surrounding tissues. Subsequently, the AZ is pushed upward, resulting in the formation of a cone-shaped stump when the bud falls. This cone-shaped bud is a characteristic feature of floral organ abscission in poinsettia. The expression of gene sequence 90a (XM_011094475/AY188755) is required for abscission; both in pea and poinsettia. These findings could have broader implications for understanding abscission, given that pea and poinsettia diverged in evolution approximately 94–98 million years ago. Consequently, any shared gene or process is likely to be widespread in the plant kingdom [47].
2.2.2 Elucidated Causes or Factors in Plant Abscission
In mango, two coding genes like the INFLORESCENCE DEFICIENT IN ABSCISSION (IDA) were isolated, the MiIDA1, and MiIDA2 genes [41]. These MiIDA-LIKE genes were triggered by ethephon (Ethylene). The ectopic expression of both genes in Arabidopsis led to the promotion of floral organ abscission. This was accompanied by an early increase in the cytosolic pH of flower AZ cells, a phenomenon associated with abscission, and by the activation of cell separation in vestigial AZ [41]. The abscission ability was restored in an AtIDA mutant, with overexpression of both genes. These findings indicated that MiIDA1 and MiIDA2 play roles in influencing mango fruit abscission.
In litchi fruits, the external application of brassinosteroids (BRs) decreased ethylene-provoked fruit abscission by 53.75% [43]. This response was associated with reduced ethylene production and suppressed activation of the ethylene biosynthetic genes ACC-SYNTHASE LcACS1/4 and ACC-OXIDASE LcACO2/3 in the AZ. Two genes encoding signaling components of the BR nucleous, BRASSINAZOLE RESISTANT (BZR) transcription factors, LcBZR1 and LcBZR2, were described. LcBZR1/2 are in the nucleus and act as transcriptional repressors. These results suggest that BR suppresses abscission in ethylene-induced fruits via LcBZR1/2-regulated expression of genes involved in ethylene biosynthesis in litchi. The Arabidopsis BZR1-1D enhancement of function mutant also depicted tardy floral organ abscission at the same time with reduce expression of ACS/ACO genes and minimum ethylene production, so it can be inferred a conservation in Arabidopsis of mechanisms in the organ controlled by BZR transcriptional factors during abscission through the regulation of ethylene biosynthesis.
LcKNAT1, a cluster of ARABIDOPSIS THALIANA-LIKE PROTEIN1 (KNAT1), acted as a negative regulator, controlling fruit abscission by suppressing the expression of ethylene (ET) biosynthetic genes in litchi [39]. LcKNAT1 is capable of directly inhibiting the transcription of LcEIL2 and LcEIL3, two homologs of ETHYLENE INSENSITIVE 3-like (EIL) in litchi, and these homologs act as positive regulators in ethylene-activated fruit abscission by directly stimulating the expression of genes responsible for ethylene biosynthesis and cell wall degradation [39]. The LcKNAT1-LcEIL2/3 regulatory module may play a role in fruit abscission in litchi, and it is suggested that LcKNAT1 could suppress ethylene biosynthesis and signaling to regulate fruit abscission.
The suppression of genetic expression of BEL1-LIKE HOMEODOMAIN4 (SlBL4) induced the elongation and abscission of fruit pedicels in tomato plants (Solanum lycopersicum cv. Micro-TOM) [42]. The anatomical study revealed an increased number of epidermal cell layers and the absence of a AZ in the SlBL4 RNAi lines, as compared to the wild-type plants. RNA-seq analysis indicated that the control of abscission by SlBL4 was linked to the modified abundance of genes associated with meristems, auxin transporters, signaling components, and cell wall metabolism. Additionally, SlBL4 had a positive impact on the concentration of auxin in the AZ.
The AGAMOUS-LIKE 15 (AGL15) was recognized as a potential regulator of HAE expression and governs a portion of the mechanisms and regulatory networks involved in a receptor-mediated signaling pathway that manages of the floral organ abscission [54]. The overexpression of AGL15 led to reduced expression of HAE and a delayed abscission phenotype. Studies using chromatin immunoprecipitation indicated that AGL15 binds to the HAE promoter in floral receptacles. Furthermore, AGL15 undergoes differential phosphorylation during floral receptacle development in a manner dependent on mitogen-activated protein kinase-kinase 4/5. The phosphorylation of AGL15 by MAP kinase is crucial for the complete expression of HAE, thus completing a positive feedback loop controlling HAE expression [54].
The signaling mediated by the IDA peptide regulates the flower abscission induced by low light in tomato plants (Solanum lycopersicum) [10]. The patterns of expression for an IDA-like gene (SlIDL6) were examined using tandem mass spectrometry to detect and characterize the mature SlIDL6 peptide. Knockout lines of SlIDL6 exhibited flower drop, and the application of SlIDL6 peptide hastened abscission. Overexpression of SlIDL6 rescued the IDA mutant phenotype in Arabidopsis, indicating functional conservation across species. SlIDL6-mediated abscission occurred through an ethylene-independent pathway. Additionally, a regulatory module involving SlWRKY17 and SlIDL6, which operates under low light conditions, induced abscission by upregulating the expression of enzymes related to the remodeling and disassembly of cell walls.
In Nicotiana attenuate, the JASMONATE ZIM DOMAIN (NaJAZd) protein counteracts flower abscission [55]. The mutant that does not express NaJAZd (arJAZd) displays a higher rate of flower abscission, perhaps through regulation of jasmonic acid (JA), jasmonoyl-L-isoleucine tiers and/or expression of NaMYB305 gene in flowers. This insight into the role of JAZ proteins in flower and seed development underscores the diverse functions of jasmonates and JAZ proteins.
Naturally, drop flower rate increased in 63.1% in untreated ‘Thompson Seedless’ grapevine (Vitis vinifera), to 83% and 99% following the application of gibberellic acid (GAc) and shading treatments, respectively [33]. Both treatmets exerted a wide-ranging impact on the metabolic processes of the flower clusters. Distinct effects of shade encompassed the inhibition of photosynthesis, linked nutritional strain, imbalance in carbon/nitrogen levels, and suppression of cell division. Conversely, GAc application stimulated energetic metabolism alongside the initiation of nucleotide biosynthesis and carbon metabolism, revealing alternative pathways for abscission regulation.
In yellow lupine (Lupinus luteus), a water-holding capacity of 25% reduced the number of nodules in the root and stimulated floral abortion [40]. The response of the flower AZ to drought involves the increased expression of genes responsible for extensins, galactans, arabins, xylogalacturonan, and xyloglucans.
Soil water deficits leads to lipid peroxidation in yellow lupine and the increased expression of phospholipase D and lipoxygenase; concurrently, there is a substantial accumulation of JA in the AZ tissue [38]. Crucial processes in JA coupling and signaling under adverse conditions were clarified by assessing the levels and tissue-specific distribution of enzyme-providing JASMONATE RESISTANT1 (JAR1) derivatives and the CORONATINE INSENSITIVE1 (COI1) receptor. Simultaneously, the stimulation of AZ during flower abscission under drought conditions is intricately linked to lipid alterations, resulting in the production of JA, its conjugation, and the initiation of signaling pathways.
In ‘Hongro’ apple grafted onto ‘M9’ rootstocks under cold-stress conditions (4°C), the initiation of abscission was observed, accompanied by the increased expression of genes involved in abscisic acid biosynthesis (MdNCED1) and metabolism (MdCYP707A), as well as genes for ethylene biosynthesis (MdACS1) and receptor (MdETR2) in the pedicel [49]. For ‘Golden Delicious’ apple benzyladenine and metamitron use of application led to a 66% and 91% increase in total fruitlet abscission, respectively [50]. Additionally, the initiation of the abscission process at the cortex level is regulated by a complex network of interactions involving hormones (primarily abscisic acid and ethylene) and other signaling molecules.
In peach, the PpILR1 enzyme, an IAA-amido acid hydrolase, directly controls the expression of PpACS1, which is a crucial factor in ethylene biosynthesis during fruit abscission [51]. PpILR1 triggers the ethylene biosynthesis pathway through two mechanisms: firstly, by directly stimulating the expression of PpACS1 via its transcriptional induction activity, and secondly, by releasing active IAA from auxin-amino acid conjugates, thereby inducing the expression of PpACS1 through the auxin signal transduction pathway.
2.2.3 IDA/IDL-HAE/HSL2 Pathway
The LcIDLI gene of litchi, a homolog of IDA, was identified and it has a comparable function to AtIDA, which is controlling floral organ abscission in Arabidopsis [22]. A HAESA-LIKE homolog, LcHSL2, was isolated and is probably engaged in fruit abscission in litchi, as well [45]. Misplaced expression of LcHSL2 in wild-type Arabidopsis did not affect floral abscission. Nevertheless, the presence of LcHSL2 in the hae hsl2 mutant background completely restored the deficiency in floral organ abscission. LcHSL2 is situated in the cell membrane, and this gene is expressed at the pedicel AZ in litchi and the floral AZ in Arabidopsis. Real-time PCR analysis revealed an increase in LcHSL2 expression during ethephon-induced fruitlet abscission. These observations indicate that HSL2 homologs exhibit functional conservation in both Arabidopsis and litchi, with LcHSL2 potentially playing a crucial role in regulating fruit abscission in litchi.
Floral organ abscission in Arabidopsis is controlled by a signaling pathway that includes the peptide ligand IDA, the RECEPTOR-LIKE KINASES (RLKs) HAE and HSL2, and a downstream MAP KINASE (MAPK) cascade [46]. The SERK family RLKs act in a redundant way in controlling floral organ abscission downriver of IDA and upriver of MAPK cascade. IDA induces the formation of heterodimers between HAE/HSL2 and SERKs, leading to reciprocal transphosphorylation. Notably, the interaction of SERK3 residues with the immune receptor FLS2 is regulated, and the involvement of the brassinosteroid receptor BRI1 is essential for the IDA-regulated HAE/HSL2-SERK3 interaction. This suggests that SERKs act as co-receptors of HAE/HSL2 in sensing.
It was proposed that the IDA–HAE–HSL2 complex contributes to a pathway for abscission that is not dependent on ethylene [56], based on results of no abscise flower organs in the ida mutant ethylene-treated. IDA-HAE/HSL2 signaling is critical as a signal inducer for the conclude organ abscission and it is preserved in many species, but it is not participate in initiating the abscission processes of floral organs in the AZ and it controls later stages of the process, in particular the ethylene promotes the IDA gene in the AZ flower organs in Arabidopsis [57].
The initial differentiation of pedicel AZ in tomato plants occurs when the sepal differentiates from the floral primordium. The AZ cells are formed in the internal region of pedicel and gradually extend to the external tissues. Cells in the AZ surrounding the vascular tissue and cortex can divide, so that, the AZ has a capacity like meristems [58].
Normally, abscission occurs when ovule-fertilization unsuccessful, or after the fruit totally matures. To study the process of pedicel absorption, it is artificially induced with the ethylene-external application and by removing flowers. The initial phase of cell separation for abscission occurs in the cortex, specifically on the distal side of the AZ, when ethylene is applied to the pedicel. In the case of pedicel abscission induced by emasculation, first takes place in the epidermis AZ. The dissolution of the middle lamina is a common response to both types of abscission induction. Essential components such as cell wall hydrolysis enzymes and remodeling proteins, including polygalacturonase (TOMATO ABSCISSION-ASSOCIATED POLYGALACTURONASE; TAPG), endo-β-1,4-glucanase (CELLULASE; CEL), XYLOGLUCAN ENDOTRANSGLUCOSYLASE/HYDROLASE (XTH), and expansins, play a crucial role in the abscission process. Additionally, the enlargement of epidermal cells is stimulated to provide mechanical force and facilitate abscission. Furthermore, the formation of a thickened and lignified cell wall on the abscissa surface of the proximal side suggests the development of a protective layer to prevent pathogen invasion [31].
In this, the MADS-BOX JOINTLESS (j), MACROCALYX (MC) and SIMBP21 genes are co-expressed in vascular tissue for the development of the AZ [31].
When the AZ cells of the pedicel of tomato sense an abscission stimulation signal, their adhesion begins to deteriorate, since genes for cell wall hydrolysis enzymes (TAPG and Cel) are expressed and components that regulate adhesion specifically increase and intensify programmed cell death in AZ [59].
Transcriptomic analysis at the onset of abscission indicated that many genes may be responsible for abscission regulatory functions, such as genes for families of transcription factors ARF, Aux/IAA, AGL, KNOX, WRKY, HAT, AP2b, HLH, and NAC, genes for elements of signal transduction pathways like LRR-RLK, a Ser/Thr protein kinase, and a component gene of an RNA-induced silencing complex, AGO1 [60].
The tomato transcription factors, homologues of WUSCHEL (LeWUS), GOBLET (GOB), LATERAL SUPPRESSOR (Ls), and BLIND (Bl), play an important role in AZ, since they promote the maintenance of undifferentiated cells of the AZ [61].
The ERF transcription factor SlERF52 in tomato serves as a component of a signaling pathway for pedicel abscission and plays a essential role in stimulating the expression of responsible genes for cell wall hydrolysis [62]. Additionally, the transcription factor KD1, a member of the KNOTTED1-LIKE HOMEOBOX (KNOX) family, reguls abscission by controlling genes that modulate auxin levels in tomato [63].
The SlIDL6 gen, IDA-like, controls low light-provoked flower abscission in tomato plants [10]. The SlBL4 gen negatively regulates fruit pedicels abscission in tomato, due to positively exerted influence on the auxin concentration in the AZ [42].
The Capsicum genus encompasses fruits that produce carotenoids, flavonoids, alkaloids, and capsaicinoids. Carotenoids and flavonoids are responsible for the attractive colors of bell peppers ranging from green to red, and even blue and chocolate colors [64]. Capsaicinoids attribute a characteristic flavor known as pungency, which is not present in bell pepper fruits [65].
Bell peppers are globally recognized as a highly sought-after vegetable due to their rich nutritional profile, including the presence of essential vitamins (A, C, E, and K1) and antioxidants. Their vibrant color, aromatic fragrance, flavor, and crisp texture contribute significantly to their widespread popularity in various culinary preparations worldwide [66]. As a crop, the abscission of flowers and fruits of bell peppers limits its production, and make it difficult to manage, and causes variations in the pepper prices.
3.1 Flower and Fruit Abscission in Bell Pepper
In bell peppers, the floral bud, flower, and fruit abscission is considered a yield-restrictive aspect [67]. Bell peppers also exhibit variations in the fruit harvesting pattern, with periods of high production alternating with periods of reduced production [4,5]. The variations in fruit development and yield pose challenges for growers in scheduling activities and managing the production cycle, leading to price fluctuations of peppers within a given season [6].
The abscission of bell peppers is associated with periods of high yields alternating with low-production periods, failure of pollination, drought stress, reduced light intensity, PGR (ethylene, and auxins, primarily), and source-demand relations (Table 2).
The recognized causes that provoke the abortion of reproductive structures (flowers and fruits) in the cultivation of bell pepper, include; high temperatures, reduced light intensity, plant density, drought, salt stress, production alternation, pollination failure, PGR, source-demand relationships, and fruits with high growth rate. These factors are summarized in Fig. 4.
Figure 4: Causes of abscission of reproductive structures in bell pepper (Capsicum annuum L.)
Exposure to high temperatures (32°C and 40°C) triggered the rise of abortion rate of reproductive structures in bell pepper cv. ‘Maor’ [74]. High temperatures were related to an increased production of ethylene and a lower concentration of IAA, which are induced in the AZ of reproductive organs. Ethylene production was caused by the expression of genes that encode ACS, ACO, ETHYLENE RESPONSE (ETR, ERS), CONSTITUTIVE TRIPLE RESPONSE (CTR), and ETHYLENE INSENSITIVE3 (EIN3) [44]. Auxins are synthesized by the AUXIN RESPONSE FACTOR 1/2 (ARF1/2, 19) genes [77].
Floral abscission was also caused by reduced light intensity or leaf removal in bell peppers. These conditions decreased carbohydrate accumulation (mainly sucrose and reducing sugars) [72]. The light intensity threshold for daily carbohydrate accumulation in sink leaves was considerably lower than in flowers, resulting in higher daytime carbohydrate accumulation in the sink leaves compared to the adjacent floral buds under all light intensities. This differential accumulation indicates a competition for assimilates between these organs. Notably, bell pepper cv. ‘Maor’ and ‘899’ (sensitive to abscission) exhibited lower accumulation of soluble sugars and starch in shaded conditions compared to bell pepper cv. ‘Mazurka’ and paprika cv. ‘Lehava’ (less sensitive).
In sweet pepper cv. ‘Gialte’, far-red light (FR)-encouraged fruit abortion in non-decapitated plants (<1 fruit plant–1) [78]. Notably, the removal of the apical shoot led to an increase in the number of fruits per plant (6–7 fruit plant–1) under both light conditions, indicating that the presence of the apical shoot has a stimulating effect on fruit abortion. This observation suggests that the apical shoot mediates the fruit abortion stimulated by FR in pepper, as FR had no influence on fruit abortion in decapitated plants. Reduced sucrose accumulation and lower invertase activities in flowers were associated with FR-stimulated fruit abortion. Additionally, under additional far-red light conditions, increased auxin levels in shoot apices promote fruit abortion by increasing competition for assimilates between apices and flowers, thereby limiting assimilate import into flowers.
The signaling pathways of trehalose 6-phosphate (T6P) and sucrose nonfermenting-related (SnRK1) appear to play a role in adjusting metabolism to sugar starvation stress in flowers [37]. This adaptation involves the regulation of starch remobilization and interactions with 3-Indole Acetic Acid (IAA), Abscisic Acid (ABA), and ET. Continuous limitation of assimilable supply leads to starch depletion in flowers, potentially resulting in abscission.
Ethylene in bell pepper acts as an abscission promoter, whereas auxins play an inhibitory role [69]. Similarly, the best moment within the flowering stages for the application of commercial chemical products that inhibit the abscission caused by ET is when anthesis is at 45° of petals opening. The application of commercial inhibitors (IAA) has the best inhibitory effect of abscission process [71].
The auxin-exogenous application at concentrations of 50, 100, 150, and 200 µg ml–1 IAA helped to improve the characteristics of growth and fruiting under high-temperature conditions in the hybrid ‘Indra’ [21]. IAA applications enhanced chlorophyll, carotenoids, carbohydrates, protein, and proline contents, reduced lipid peroxidation, and decreased cellulase activity in the pedicel of flowers of treated plants (17.15% less with 100 µg ml–1 of IAA). These effects lead to a reduction in floral abortion. Similarly, there was a greater pollen viability and percentage of fruit production at high temperatures, which is equivalent to a higher yield (1.6 kg plant with 100 µg ml–1 of IAA) compared to control.
The PGR successfully tested in the cultivation of sweet pepper is 4-chlorophenoxyacetic acid (4-CPA), a synthetic pesticide alike to auxin [75]. The application of 2000 ppm resulted in a higher quantity of flowers and fruits, representing a 24.50% increase in the specific cultivar ‘BARI Misti morich-1’ and 26.02% in the cultivar ‘BARI Misti morich-1’ Lamuyo, in comparison to control plants that only exhibited a 18%.
Differences, between cultivars (‘McIlhenny Select’ and ‘HP’) of Capsicum frutescens, regarding abscission time of deflowered pedicel, with (complete prevention of abscission for the ‘HP’ cultivar and only 6% for the ‘McIlhenny Select’ cultivar) and without (abscission at 84 h for ‘McIlhenny Select’ and 48 h for ‘HP’) the application of auxins (1-naphthaleneacetic acid) in the pedicel AZ, were observed [79].
Abscission of bell pepper flowers was higher at low levels of nitrate. For instance, when 4 milliequivalents per liter (meq L–1) of nitrates were supplied in comparison to the application of 9 and 14 meq L–1 nitrates. These results indicated a strong correlation between growth parameters and carbon or nitrogen content in plants, which is decreased when the supply of nitrates was lower [80].
A gradual rise in the concentration of total nitrogen (N), in progressive physiological stages, from 3 to 6 and 9 mM, augmented the numbre of flower and fruit set, and produced the greatest fruit yield (3444 g plant–1). This happen when NH4-N was 30% of the total (6 mM) during the vegetative phase, and NO3-N was the only source of N during the fruit filling period [81].
The abortion rate of flowers and fruits in bell peppers increased linearly as photosynthates availability decreased [73]. Practices that reduced photosynthate availability include pruning of unfolded leaves, high-plant densities, and reduction of solar radiation for a seven-day periods. Similarly, the existence of fruits of early formation in the first nodes (fruits with a high growth rate) triggered the flowers and fruits abortion. The period of greater susceptibility to abortion is from just before anthesis to about two weeks after anthesis.
The demand strength for vegetative structures tended to be lower in large-fruited cultivars compared to small-fruited ones. As fruit size increased, there was greater variability in fruiting and fruit yield. Large-fruited cultivars necessitated a higher ratio of resources to demand for fruiting, leading to an increased demand for photo-assimilates. Various methods were employed to reduce the heterogeneity of fruiting, such as enhancing the strength of the source, reducing the strength of vegetative demand, adjusting the source-demand relationship for fruit set, and controlling the rate of flower appearance. Additionally, individual fruits were harvested before reaching full maturity to achieve a reduction in fruiting heterogeneity [6].
3.2 Regulation of Abscission in Bell Pepper
Current published research on abscission in bell pepper addresses only the factors that induce or influence abscission, but the genetic, hormonal, and signaling regulatory mechanisms have not been elucidated. The advances made in the abscission process in model plants and species taxonomically related to the bell pepper are presented [82]. Further research is required since it could be different in Capsicum-plant species.
A model for the regulation of flower and fruit abscission in bell pepper has not fully been established. The model proposed for tomato could be used as a starting point due to the taxonomic and morphological proximity to bell pepper plants.
4 Conclusions and Future Outlooks
To our knowledge, there is no published research that fully describes the mechanisms, genes, signaling, PGR, and compounds involved in the flower and fruit abscission in bell peppers.
The genetic, biochemical, molecular, physiological, and phytohormonal factors that intervene in the abscission of reproductive organs were described in the model plant Arabidopsis thaliana. Mainly, in the identification of genes that take part in the abscission (IDA, IDL, KNATd, RLK, MAPK, AGL15), and it is regulated by the interaction of peptide hormone IDA, a pair of redundant receptor-like protein kinases, HAESA (HAE) and HAESA-LIKE2 (HSL2), and co-receptors of Somatic Embryogenesis Receptor-Like Kinase (SERK). In this sense, there have been important advances, such as the identification of orthologous genes for tomato (SlIDL6, SlBL4), mango (MiIDA1, MiIDA2) and litchi (LcKNAT1, LcHSL2, LcIDL1). Nonetheless, the functionality of the signaling module for any other species has not been demonstrated yet. It would be pertinent and appropriate to extend and carry out the mentioned studies also for non-model plants of global economic importance, such as the bell pepper. In the same way, it would be very important to identify each of the factors that cause the abortion of flowers and fruits within the plant and the cultivation system. If these goals are achieved, the bell pepper-fruit abscission process will be effortlessly controlled under growing conditions, increasing harvesting and yield.
Acknowledgement: We thank National Council of Humanities, Science and Technology (CONAHCYT Spanish Acronym) for its support program for the quality postgraduate courses and the Biosciences Doctorate of the Guanajuato University for the academic support that made this work possible.
Funding Statement: The authors received no specific funding for this study.
Author Contributions: The authors confirm contribution to the paper as follows: study conception and design: Héctor G. Núñez-Palenius, and Ramiro Reyes-Castro; draft manuscript preparation: Ramiro Reyes-Castro, Juan I. Valiente-Banuet, and Blanca E. Orosco-Alcalá; writing-review and editing: María E. Sosa-Morales, Graciela M. L. Ruiz-Aguilar, Rafael Guzmán-Mendoza, and Rogelio Costilla-Salazar. All authors reviewed the results and approved the final version of the manuscript.
Availability of Data and Materials: Not applicable.
Ethics Approval: Not applicable.
Conflicts of Interest: The authors declare that they have no conflicts of interest to report regarding the present study.
References
1. Tonutti P, Brizzolara S, Beckles DM. Reducing crop losses by gene-editing control of organ developmental physiology. Curr Opin Biotechnol. 2023;81:102925. doi:10.1016/j.copbio.2023.102925. [Google Scholar] [PubMed] [CrossRef]
2. Patharkar OR, Walker JC. Connections between abscission, dehiscence, pathogen defense, drought tolerance, and senescence. Plant Sci J. 2019;284:25–9. doi:10.1016/j.plantsci.2019.03.016. [Google Scholar] [PubMed] [CrossRef]
3. Sundaresan S, Philosoph-Hadas S, Riov J, Salim S, Meir S. Expression kinetics of regulatory genes involved in the vesicle trafficking processes operating in tomato flower abscission zone cells during pedicel abscission. Life. 2020;10(11):273. doi:10.3390/life10110273. [Google Scholar] [PubMed] [CrossRef]
4. Heuvelink E, Körner O. Parthenocarpic fruit growth reduces yield fluctuation and blossom-end-rot in sweet pepper. Ann Bot. 2001;88(1):69–74. doi:10.1006/anbo.2001.1427. [Google Scholar] [CrossRef]
5. Heuvelink E, Marcelis LFM, Körner O. How to reduce yield fluctuations in sweet pepper? Acta Hortic. 2004;633:349–55. doi:10.17660/ActaHortic.2004.633.42. [Google Scholar] [CrossRef]
6. Ma YT, Wubs AM, Mathieu A, Heuvelink E, Zhu JY, Hu BG et al. Simulation of fruit-set and trophic competition and optimization of yield advantages in six Capsicum cultivars using functional-structural plant modelling. Ann bot. 2011;107(5):793–803. doi:10.1093/aob/mcq223. [Google Scholar] [PubMed] [CrossRef]
7. Guilioni L, Wery J, Tardieu F. Heat stress-induced abortion of buds and flowers in pea: is sensitivity linked to organ age or to relations between reproductive organs? Ann Bot. 1997;80:159–68. doi:10.1006/anbo.1997.0425. [Google Scholar] [CrossRef]
8. Patharkar OR, Walker JC. Core mechanisms regulating developmentally timed and environmentally triggered abscission. Plant Physiol. 2016;172(1):510–20. doi:10.1104/pp.16.01004. [Google Scholar] [PubMed] [CrossRef]
9. Jin X, Zimmermann J, Polle A, Fischer U. Auxin is a long-range signal that acts independently of ethylene signaling on leaf abscission in Populus. Front Plant Sci. 2015;6(2625):1–10. doi:10.3389/fpls.2015.00634. [Google Scholar] [PubMed] [CrossRef]
10. Li R, Shi CL, Wang X, Meng Y, Cheng L, Jiang CZ, et al. Inflorescence abscission protein SlIDL6 promotes low light intensity-induced tomato flower abscission. Plant Physiol. 2021;186(2):1288–301. doi:10.1093/plphys/kiab121. [Google Scholar] [PubMed] [CrossRef]
11. Berjano R, De Vega C, Arista M, Ortiz PL, Talavera S. A multi-year study of factors affecting fruit production in Aristolochia paucinervis (Aristolochiaceae). Am J Bot. 2006;93(4):599–606. doi:10.3732/ajb.93.4.599. [Google Scholar] [PubMed] [CrossRef]
12. Dos Santos MSN, Wancura JHC, Oro CED, Dallago RM, Tres MV. Opportunities and challenges of plant bioactive compounds for food and agricultural-related areas. Phyton-Int J Exp Bot. 2022;91(6):1105–27. doi:10.32604/phyton.2022.020913. [Google Scholar] [CrossRef]
13. Zhao M, Shi CL, Li J. Abscission cues generated within the abscising organ and perceived by the abscission zone in woody fruit crops. Fruit Res. 2024;4(1):e014. doi:10.48130/frures-0024-0007. [Google Scholar] [CrossRef]
14. Olsson V, Butenko MA. Abscission in plants. Curr Biol. 2018;28(8):R338–9. doi:10.1016/j.cub.2018.02.069. [Google Scholar] [PubMed] [CrossRef]
15. Estornell LH, Agustí J, Merelo P, Talón M, Tadeo FR. Elucidating mechanisms underlying organ abscission. Plant Sci. 2013;199:48–60. doi:10.1016/j.plantsci.2012.10.008. [Google Scholar] [PubMed] [CrossRef]
16. Sawicki M, Aït Barka E, Clément C, Vaillant-Gaveau N, Jacquard C. Cross-talk between environmental stresses and plant metabolism during reproductive organ abscission. J Exp Bot. 2015;66(7):1707–19. doi:10.1093/jxb/eru533. [Google Scholar] [PubMed] [CrossRef]
17. Tranbarger TJ, Domonhédo H, Cazemajor M, Dubreuil C, Fischer U, Morcillo F. The PIP peptide of INFLORESCENCE DEFICIENT IN ABSCISSION enhances Populus leaf and Elaeis guineensis fruit abscission. Plants. 2019;8(6):143. doi:10.3390/plants8060143. [Google Scholar] [PubMed] [CrossRef]
18. Kim J. Four shades of detachment: regulation of floral organ abscission. Plant Signal Behav. 2014;9(11):e976154. [Google Scholar] [PubMed]
19. Kim J, Chun JP, Tucker ML. Transcriptional regulation of abscission zones. Plants. 2019;8(6):154. doi:10.3390/plants8060154. [Google Scholar] [PubMed] [CrossRef]
20. Botton A, Ruperti B. The yes and no of the ethylene involvement in abscission. Plants. 2019;8(6):187. doi:10.3390/plants8060187. [Google Scholar] [PubMed] [CrossRef]
21. Kaur S, Ghai N, Jindal SK. Improvement of growth characteristics and fruit set in bell pepper (Capsicum annuum L.) through IAA application. Indian J Plant Physiol. 2017;22(2):213–20. doi:10.1007/s40502-017-0293-0. [Google Scholar] [CrossRef]
22. Ying P, Li C, Liu X, Xia R, Zhao M, Li J. Identification and molecular characterization of an IDA-like gene from litchi, LcIDL1, whose ectopic expression promotes floral organ abscission in Arabidopsis. Sci Rep. 2016;6(1):37135. doi:10.1038/srep37135. [Google Scholar] [PubMed] [CrossRef]
23. Lee Y, Kwak JM. Cellular coordination controlling organ separation and surface integrity in plants. BMB Rep. 2018;51(7):317–8. doi:10.5483/bmbrep.2018.51.7.142. [Google Scholar] [PubMed] [CrossRef]
24. Patharkar OR, Walker JC. Advances in abscission signaling. J Exp Bot. 2018;69(4):733–40. doi:10.1093/jxb/erx256. [Google Scholar] [PubMed] [CrossRef]
25. Ventimilla D, Velázquez K, Ruiz-Ruiz S, Terol J, Pérez-Amador MA, Vives MC, et al. IDA (INFLORESCENCE DEFICIENT IN ABSCISSION)-like peptides and HAE (HAESA)-like receptors regulate corolla abscission in Nicotiana benthamiana flowers. BMC Plant Biol. 2021;21(1):226. doi:10.1186/s12870-021-02994-8. [Google Scholar] [PubMed] [CrossRef]
26. McKim SM, Stenvik GE, Butenko MA, Kristiansen W, Cho SK, Hepworth SR, et al. The BLADE-ON-PETIOLE genes are essential for abscission zone formation in Arabidopsis. Development. 2018;135(8):1537–46. doi:10.1242/dev.012807. [Google Scholar] [PubMed] [CrossRef]
27. Wang XQ, Xu WH, Ma LG, Fu ZM, Deng XW, Li LJ, et al. Requirement of KNAT1/BP for the development of abscission zones in Arabidopsis thaliana. J Integr Plant Biol. 2006;48(1):15–26. doi:10.1111/j.1744-7909.2005.00085.x-i1. [Google Scholar] [CrossRef]
28. Gubert CM, Christy ME, Ward DL, Groner WD, Liljegren SJ. ASYMMETRIC LEAVES1 regulates abscission zone placement in Arabidopsis flowers. BMC Plant Biol. 2014;14(1):195. doi:10.1186/s12870-014-0195-5. [Google Scholar] [PubMed] [CrossRef]
29. Gómez-Mena C, Sablowski R. ARABIDOPSIS THALIANA HOMEOBOX GENE1 establishes the basal boundaries of shoot organs and controls stem growth. Plant Cell. 2008;20(8):2059–72. doi:10.1105/tpc.108.059188. [Google Scholar] [PubMed] [CrossRef]
30. Mao L, Begum D, Chuang HW, Budiman MA, Szymkowiak EJ, Irish EE, et al. JOINTLESS is a MADS-box gene controlling tomato flower abscission zone development. Nat. 2000;406(6798):910–3. doi:10.1038/35022611. [Google Scholar] [PubMed] [CrossRef]
31. Ito Y, Nakano T. Development and regulation of pedicel abscission in tomato. Front Plant Sci. 2015;6:442. doi:10.3389/fpls.2015.00442. [Google Scholar] [PubMed] [CrossRef]
32. Chen MK, Hsu WH, Lee PF, Thiruvengadam M, Chen HI, Yang CH, et al. The MADS box gene, FOREVER YOUNG FLOWER, acts as a repressor controlling floral organ senescence and abscission in Arabidopsis. Plant J. 2011;68(1):168–85. doi:10.1111/j.1365-313X.2011.04677.x. [Google Scholar] [PubMed] [CrossRef]
33. Patharkar OR, Macken TA, Walker JC. Serine 231 and 257 of Agamous-like 15 are phosphorylated in floral receptacles. Plant Signal Behav. 2016;11(7):e1199314. doi:10.1080/15592324.2016.1199314. [Google Scholar] [PubMed] [CrossRef]
34. Stenvik GE, Butenko MA, Urbanowicz BR, Rose JK, Aalen RB. Overexpression of INFLORESCENCE DEFICIENT IN ABSCISSION activates cell separation in vestigial abscission zones in Arabidopsis. Plant Cell. 2006;8(6):1467–76. doi:10.1105/tpc.106.042036. [Google Scholar] [PubMed] [CrossRef]
35. Kućko A, Smoliński D, Wilmowicz E, Florkiewicz A, de Dios AJ. Spatio-temporal localization of LlBOP following early events of floral abscission in yellow lupine. Protoplasma. 2019;256(5):1173–83. doi:10.1007/s00709-019-01365-3. [Google Scholar] [PubMed] [CrossRef]
36. Zhao M, Li J. Molecular events involved in fruitlet abscission in litchi. Plants. 2020;9(2):151. doi:10.3390/plants9020151. [Google Scholar] [PubMed] [CrossRef]
37. Li Q, Chai L, Tong N, Yu H, Jiang W. Potential carbohydrate regulation mechanism underlying starvation-induced abscission of tomato flower. Int J Mol Sci. 2022;23(4):1952. doi:10.3390/ijms23041952. [Google Scholar] [PubMed] [CrossRef]
38. Kućko A, Florkiewicz AB, Wolska M, Miętki J, Kapusta M, Domagalski K, et al. Jasmonate-dependent response of the flower abscission zone cells to drought in yellow lupine. Plants. 2022;11(4):527. doi:10.3390/plants11040527. [Google Scholar] [PubMed] [CrossRef]
39. Ma X, Ying P, He Z, Wu H, Li J, Zhao M. The LcKNAT1-LcEIL2/3 regulatory module is involved in fruitlet abscission in litchi. Front Plant Sci. 2022;12:802016. doi:10.3389/fpls.2021.802016. [Google Scholar] [PubMed] [CrossRef]
40. Wilmowicz E, Kućko A, Alché JD, Czeszewska-Rosiak G, Florkiewicz AB, Kapusta M, et al. Remodeling of cell wall components in root nodules and flower abscission zone under drought in yellow lupine. Int J Mol Sci. 2022;23(3):1680. doi:10.3390/ijms23031680. [Google Scholar] [PubMed] [CrossRef]
41. Rai AC, Halon E, Zemach H, Zviran T, Sisai I, Philosoph-Hadas S, et al. Characterization of two ethephon-induced IDA-like genes from mango, and elucidation of their involvement in regulating organ abscission. Genes. 2021;12(3):439. doi:10.3390/genes12030439. [Google Scholar] [PubMed] [CrossRef]
42. Yan F, Gong Z, Hu G, Ma X, Bai R, Yu R, et al. Tomato SlBL4 plays an important role in fruit pedicel organogenesis and abscission. Hortic Res. 2021;8(1):78. doi:10.1038/s41438-021-00515-0. [Google Scholar] [PubMed] [CrossRef]
43. Ma X, Yuan Y, Li C, Wu Q, He Z, Li J, et al. Brassinosteroids suppress ethylene-induced fruitlet abscission through LcBZR1/2-mediated transcriptional repression of LcACS1/4 and LcACO2/3 in litchi. Hortic Res. 2021;8(1):105. doi:10.1038/s41438-021-00540-z. [Google Scholar] [PubMed] [CrossRef]
44. Liu D, Li J, Li Z, Pei Y. Hydrogen sulfide inhibits ethylene-induced petiole abscission in tomato (Solanum lycopersicum L.). Hortic Res. 2020;7(1):14. doi:10.1038/s41438-019-0237-0. [Google Scholar] [PubMed] [CrossRef]
45. Wang F, Zheng Z, Yuan Y, Li J, Zhao M. Identification and characterization of HAESA-like genes involved in the fruitlet abscission in litchi. Int J Mol Sci. 2019;20(23):5945. doi:10.3390/ijms20235945. [Google Scholar] [PubMed] [CrossRef]
46. Meng X, Zhou J, Tang J, Li B, De Oliveira MVV, Chai J, et al. Ligand-induced receptor-like kinase complex regulates floral organ abscission in Arabidopsis. Cell Rep. 2016;14(6):1330–8. doi:10.1016/j.celrep.2016.01.023. [Google Scholar] [PubMed] [CrossRef]
47. Hvoslef-Eide AK, Munster CM, Mathiesen CA, Ayeh KO, Melby TI, Rasolomanana P, et al. Primary and secondary abscission in Pisum sativum and Euphorbia pulcherrima–how do they compare and how do they differ? Front Plant Sci. 2016;6:1204. doi:10.3389/fpls.2015.01204. [Google Scholar] [PubMed] [CrossRef]
48. Domingos S, Fino J, Cardoso V, Sánchez C, Ramalho JC, Larcher R, et al. Shared and divergent pathways for flower abscission are triggered by gibberellic acid and carbon starvation in seedless Vitis vinifera L. BMC Plant Biol. 2016;16(1):38. doi:10.1186/s12870-016-0722-7. [Google Scholar] [PubMed] [CrossRef]
49. Lee Y, Do VG, Kim S, Kweon H, McGhie TK. Cold stress triggers premature fruit abscission through ABA-dependent signal transduction in early developing apple. PLoS One. 2021;16(4):e0249975. doi:10.1371/journal.pone.0249975. [Google Scholar] [PubMed] [CrossRef]
50. Giulia E, Alessandro B, Mariano D, Andrea B, Benedetto R, Angelo R. Early induction of apple fruitlet abscission is characterized by an increase of both isoprene emission and abscisic acid content. Plant Physiol. 2013;161(4):1952–69. doi:10.1104/pp.112.208470. [Google Scholar] [PubMed] [CrossRef]
51. Wang X, Meng J, Deng L, Wang Y, Liu H, Yao JL, et al. Diverse functions of IAA-leucine resistant PpILR1 provide a genic basis for auxin-ethylene crosstalk during peach fruit ripening. Front Plant Sci. 2021;12:655758. doi:10.3389/fpls.2021.655758. [Google Scholar] [PubMed] [CrossRef]
52. Estornell LH, Wildhagen M, Pérez-Amador MA, Talón M, Tadeo FR, Butenko MA, et al. The IDA peptide controls abscission in Arabidopsis and Citrus. Front Plant Sci. 2015;6(195):1003. doi:10.3389/fpls.2015.01003. [Google Scholar] [PubMed] [CrossRef]
53. Sundaresan S, Philosoph-Hadas S, Riov J, Belausov E, Kochanek B, Tucker ML, et al. Abscission of flowers and floral organs is closely associated with alkalization of the cytosol in abscission zone cells. J Exp Bot. 2015;66(5):1355–68. doi:10.1093/jxb/eru483. [Google Scholar] [PubMed] [CrossRef]
54. Patharkar OR, Walker JC. Floral organ abscission is regulated by a positive feedback loop. Proc Natl Acad Sci U S A. 2015;112(9):2906–11. doi:10.1073/pnas.1423595112. [Google Scholar] [PubMed] [CrossRef]
55. Oh Y, Baldwin IT, Galis I. A jasmonate ZIM-domain protein NaJAZd regulates floral jasmonic acid levels and counteracts flower abscission in Nicotiana attenuata plants. PLoS One. 2013;8(2):e57868. doi:10.1371/journal.pone.0057868. [Google Scholar] [PubMed] [CrossRef]
56. Butenko MA, Stenvik GE, Alm V, Saether B, Patterson SE, Aalen RB. Ethylene-dependent and -independent pathways controlling floral abscission are revealed to converge using promoter: :reporter gene constructs in the ida abscission mutant. J Exp Bot. 2006;57:3627–37. doi:10.1093/jxb/erl130. [Google Scholar] [PubMed] [CrossRef]
57. Meir S, Philosoph-Hadas S, Riov J, Tucker ML, Patterson SE, Roberts JA. Re-evaluation of the ethylene-dependent and -independent pathways in the regulation of floral and organ abscission. J Exp Bot. 2019;78(5):1461–7. doi:10.1093/jxb/erz038. [Google Scholar] [PubMed] [CrossRef]
58. Liu D, Wang D, Qin Z, Zhang D, Yin L, Wu L, et al. The SEPALLATA MADS-box protein SLMBP21 forms protein complexes with JOINTLESS and MACROCALYX as a transcription activator for development of the tomato flower abscission zone. Plant J. 2014;77:284–96. doi:10.1111/tpj.12387. [Google Scholar] [PubMed] [CrossRef]
59. Bar-Dror T, Dermastia M, Kladnik A, Znidaric MT, Novak MP, Meir S, et al. Programmed cell death occurs asymmetrically during abscission in tomato. Plant Cell. 2011;23(11):4146–63. doi:10.1105/tpc.111.092494. [Google Scholar] [PubMed] [CrossRef]
60. Meir S, Philosoph-Hadas S, Sundaresan S, Selvaraj KS, Burd S, Ophir R, et al. Microarray analysis of the abscission-related transcriptome in the tomato flower abscission zone in response to auxin depletion. Plant Physiol. 2010;154:1929–56. doi:10.1104/pp.110.160697. [Google Scholar] [PubMed] [CrossRef]
61. Nakano T, Ito Y. Molecular mechanisms controlling plant organ abscission. Plant Biotechnol. 2013;30(3):209–16. doi:10.5511/plantbiotechnology.13.0318a. [Google Scholar] [CrossRef]
62. Nakano T, Fujisawa M, Shima Y, Ito Y. The AP2/ERF transcription factor SlERF52 functions in flower pedicel abscission in tomato. J Exp Bot. 2014;65(12):3111–9. doi:10.1093/jxb/eru154. [Google Scholar] [PubMed] [CrossRef]
63. Ma C, Meir S, Xiao L, Tong J, Liu Q, Reid MS, et al. A KNOTTED1-LIKE HOMEOBOX protein regulates abscission in tomato by modulating the auxin pathway. Plant Physiol. 2015;167(3):844–53. doi:10.1104/pp.114.253815. [Google Scholar] [PubMed] [CrossRef]
64. Rhim JW, Hong SI. Effect of water activity and temperature on the color change of red pepper (Capsicum annuum L.) powder. Food Sci Biotechnol. 2011;20(1):215–22. doi:10.1007/s10068-011-0029-2. [Google Scholar] [CrossRef]
65. O’Neill J, Brock C, Olesen AE, Andresen T, Nilsson M, Dickenson AH, et al. Unravelling the mystery of capsaicin: a tool to understand and treat pain. Pharmacol Rev. 2012;64(4):939–71. doi:10.1124/pr.112.006163. [Google Scholar] [PubMed] [CrossRef]
66. Akram NA, Ashraf M. Regulation in plant stress tolerance by a potential plant growth regulator, 5-aminolevulinic acid (ALA). J Plant Growth Regul. 2013;32(3):663–79. doi:10.1007/s00344-013-9325-9. [Google Scholar] [CrossRef]
67. Kato T, Tanaka M. Studies on the fruit setting and development of sweet peppers. I. Fruiting behavior. J Jpn Soc Hortic Sci. 1971;40(4):359–66. doi:10.2503/jjshs.40.359. [Google Scholar] [CrossRef]
68. Rylski I. Pepper (Capsicum). In: Handbook of fruit set and development. Boca Raton: CRC Press; 1986. p. 341–54. [Google Scholar]
69. Wien HC, Tripp KE, Hernandez-Armenta R, Turner AD. Abscission of reproductive structures in pepper: causes, mechanisms and control. In: Tomato and pepper production in the tropics. Taipei, Taiwan: Asian Vegetable Research and Development Center; 1989. p. 150–65. [Google Scholar]
70. Wien HC. Screening pepper cultivars for resistance to flower abscission: a comparison of techniques. Hortic Sci. 1990;25(12):1634–6. doi:10.21273/HORTSCI.25.12.1634. [Google Scholar] [CrossRef]
71. Aquirre I, Gutiérrez MC, Cuartero J. Ethylene production during sweet pepper flowering. Acta Hortic. 1995;412:479–83. doi:10.17660/ActaHortic.1995.412.57. [Google Scholar] [CrossRef]
72. Aloni B, Karni L, Zaidman Z, Schaffer AA. Changes of carbohydrates in pepper (Capsicum annuum L.) flowers in relation to their abscission under different shading regimes. Ann Bot. 1996;78(2):163–8. doi:10.1006/anbo.1996.0109. [Google Scholar] [CrossRef]
73. Marcelis LFM, Heuvelink E, Baan Hofman-Eijer LR, Den Bakker J, Xue LB. Flower and fruit abortion in sweet pepper in relation to source and sink strength. J Exp Bot. 2004;55(406):2261–8. doi:10.1093/jxb/erh245. [Google Scholar] [PubMed] [CrossRef]
74. Huberman M, Riov J, Aloni B, Goren R. Role of ethylene biosynthesis and auxin content and transport in high temperature-induced abscission of pepper reproductive organs. J Plant Growth Regul. 1997;16(3):129–35. doi:10.1007/PL00006986. [Google Scholar] [CrossRef]
75. Das SK, Sarkar MD, Alam MJ, Robbani MG, Kabir MH. Influence of plant growth regulators on yield contributing characters and yield of bell pepper (Capsicum annum) varieties. J Plant Sci. 2015;10(2):63–9. doi:10.3923/jps.2015.63.69. [Google Scholar] [CrossRef]
76. Patiño AJ, Jaimez RE. Relación fuente-fuerza de la demanda en el aborto de estructuras reproductivas, tasa fotosintética y rendimiento en Capsicum annuum. Agrociencia. 2016;50(5):649–64. [Google Scholar]
77. Li J, Dai X, Zhao Y. A role for auxin response factor 19 in auxin and ethylene signaling in Arabidopsis. Plant Physiol. 2006;140(3):899–908 (In Spanish). doi:10.1104/pp.105.070987. [Google Scholar] [PubMed] [CrossRef]
78. Chen S, Marcelis LFM, Offringa R, Kohlen W, Heuvelink E. Far-red light-enhanced apical dominance stimulates flower and fruit abortion in sweet pepper. Plant Physiol. 2024;195:kiae088. doi:10.1093/plphys/kiae088. [Google Scholar] [PubMed] [CrossRef]
79. Motsenbocker CE, Arancibia RA. Tabasco pepper flower abscission at the pedicel-stem zone. Acta Hortic. 2000;514:213–8. doi:10.17660/ActaHortic.2000.514.24. [Google Scholar] [CrossRef]
80. Riga P. flower abscission in pepper plants grown under different regimes of nitrogen fertilization and photosynthetically active radiation. J Plant Nutr. 2014;37(6):907–27. doi:10.1080/01904167.2013.873464. [Google Scholar] [CrossRef]
81. Xu G, Wolf S, Kafkafi U. Effect of varying nitrogen form and concentration during growing season on sweet pepper flowering and fruit yield. J Plant Nutr. 2001;24(7):1099–116. doi:10.1081/PLN-100103806. [Google Scholar] [CrossRef]
82. Dong X, Ma C, Xu T, Reid MS, Jiang CZ, Li T. Auxin response and transport during induction of pedicel abscission in tomato. Hortic Res. 2021;8(1):192. doi:10.1038/s41438-021-00626-8. [Google Scholar] [PubMed] [CrossRef]
Cite This Article
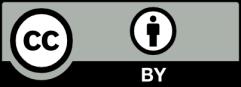
This work is licensed under a Creative Commons Attribution 4.0 International License , which permits unrestricted use, distribution, and reproduction in any medium, provided the original work is properly cited.