Open Access
ARTICLE
Summer Warming Limited Bud Output Drives a Decline in Daughter Shoot Biomass through Reduced Photosynthetis of Parent Shoots in Leymus chinensis Seedlings
1 Graduate School, Changchun University, Changchun, 130022, China
2 Key Laboratory of Vegetation Ecology, Ministry of Education, Institute of Grassland Science, School of Life Sciences, Jilin Songnen Grassland Ecosystem National Observation and Research Station, Northeast Normal University, Changchun, 130024, China
3 Jilin Agricultural Radio and Television School, Changchun, 130599, China
4 Department of Health and Environmental Sciences, Xi’an Jiaotong Liverpool University, Suzhou, 215000, China
* Corresponding Author: Junfeng Wang. Email:
(This article belongs to the Special Issue: Grassland Ecology in China under Global Change)
Phyton-International Journal of Experimental Botany 2024, 93(7), 1667-1675. https://doi.org/10.32604/phyton.2024.051548
Received 08 March 2024; Accepted 03 June 2024; Issue published 30 July 2024
Abstract
Understanding how summer warming influences the parent and daughter shoot production in a perennial clonal grass is vital for comprehending the response of grassland productivity to global warming. Here, we conducted a simulated experiment using potted Leymus chinensis, to study the relationship between the photosynthetic activity of parent shoots and the production of daughter shoots under a whole (90 days) summer warming scenario (+3°C). The results showed that the biomass of parents and buds decreased by 25.52% and 33.45%, respectively, under warming conditions. The reduction in parent shoot biomass due to warming directly resulted from decreased leaf area (18.03%), chlorophyll a (18.27%), chlorophyll b (29.21%) content, as well as a reduction in net photosynthetic rate (7.32%) and the maximum quantum efficiency of photosystem II (PSII) photochemistry (4.29%). The decline in daughter shoot biomass was linked to a decrease in daughter shoot number (33.33%) by warming. However, the number of belowground buds increased by 46.43%. The results indicated that long-term summer warming reduces biomass accumulation in parent shoot by increasing both limitation of stoma and non-stoma. Consequently, the parent shoot allocates relatively more biomass to the belowground organs to maintain the survival and growth of buds. Overall, buds, as a potential aboveground population, could remedy for the current loss of parent shoot density by increasing the number of future daughter shoots if summer warming subsides.Keywords
Clonal reproduction via belowground “bud bank” is the main reproductive mode of perennial grasses in natural grassland ecosystems [1–3]. Within a clonal plant, parent and daughter shoots can share energy through physical connections such as stolons, or rhizomes [4,5]. The resources supplied by the parent shoot directly impact the growth and quantity of the daughter shoots [6]. Photosynthesis, which is directly linked to plant growth and reproduction, and characteristics such as leaf area, leaf gas exchange, and chlorophyll fluorescence, are strongly influenced by environmental fluctuations [7–9]. Undoubtedly, these detectable changes in the parent shoots significantly affect the growth and development of the offspring.
Recently, the rise in greenhouse gases in the atmosphere has led to the expected increase in global mean temperature of 1.0°C~3.7°C [9]. Detecting and quantifying the answer of plant physiological and ecological processes to global warming is an important research goal in the field [10]. Research indicates that plant exposure to elevated temperature or brief extreme temperature events can negatively influence plant growth and reproduction [11,12]. It is now apparent that CO2 uptake and photosynthetic yield decline considerably or even cease in arid and semi-arid regions under warming [9,13–15]. Those changes are closely linked with physiological changes in photosynthesis like gas exchange and chlorophyll fluorescence. Previous studies have shown that climate warming can result in declines in leaf area for some species [16] and meanwhile increases in chlorophyll concentration [17,18], thereby light interception, resource allocation, and water uptake strategy [19]. Furthermore, previous research has shown that the maximum quantum efficiency of PSII photochemistry (Fv/Fm) and the base fluorescence (F0) are closely correlated with heat tolerance in plants [20]. Irreversible inhibition of PSII will result in a decline in maximum quantum yield if the capability of the PSII reaction center to repair damages is exceeded [21,22]. In perennial grass species, some species like Bromus inermis and Pascopyrum smithii show reduced bud development at 24°C compared with 18°C due to suppressed tiller recruitment at higher temperatures, while other species experience a significant increase in belowground bud number [23,24]. However, limited research has focused on explaining the underlying reasons for how changes in photosynthetic physiology drive the growth of belowground buds and daughter shoots. In the context of global change, examining the effects of warming on the growth of parent shoot, daughter shoot, and bud, and further exploring the underlying mechanism, could not only identify changes in the parent shoot population but also provide a better understanding of plant population renewal and succession strategies.
Leymus chinensis (Trin.) Tzvel is a rhizomatous, perennial grass widely distributed at the Russian Baikal, the northern and eastern regions of Mongolia, and the northern and northeast plains of China [25,26]. It exhibits high tolerance to low soil fertility, high pH, and drought conditions, often forming a mono-dominant population in the northeast plain of China. This species serves as an ideal object for exploring how biomass production in clonal perennial grass responds to global warming. In this study, we selected Leymus chinensis as the experimental material to examine the effects of prolonged (whole) summer warming on the parent shoot growth, daughter shoot and buds development in relation to parent photosynthetic physiology. We hypothesized that summer warming would result in: (i) a reduction in leaf area, chlorophyll content, and photosynthetic ability of parent shoots, leading to a reduction in aboveground biomass; (ii) a reduction in belowground biomass thus reducing the number of belowground buds and the production of daughter shoots.
The seeds used in the experiment were harvested from the Grassland Ecosystem Field Station of the Northeast Normal University (123°44′E, 44°40′N, 167 m asl). Under the natural condition, Leymus chinensis regrows beginning in early-mid April each year. Heading and flowering occur from mid May to late June, with seed maturation taking place in late July [27]. Parent shoots and daughter shoot leaves die when frost (usually late September or early October) arrives, but the belowground part continues to grow in the following season.
2.2 The Experimental Site, Design, and Treatments
The experimental design aligned to the previous experiment by Wang et al. [23] and was conduced in a large phytotron (LT/ACR-2002 Phytotron System, E-Sheng Tech., Beijing, China). To simulate field temperature variations, temperature settings for the control and warming phytotrons are detailed in Table 1. The temperature setting for warming phytotron during each period was 3°C ± 0.5°C higher than that of control phytotron. The photosynthetically active radiation (PAR) of plant canopy was maintained at 720 μmol−2 s−1 in both control and warming treatments. The relative humidity was maintained between 40%~60% during the experiment.
Seeds (15 grains per pot) were sown into pots (inside diameter 19 cm, capacity 8 L), filled with soil obtained from the natural Leymus chinensis grassland at the surfact of 0~25 cm. The soil pH is 8.63, conductivity is 794.3 ds m−1, total nitrogen (N) is 0.12%, and available nitrogen (N) is 91.42 mg kg−1. Kjeldahl method and alkaline hydrolysis diffusion method were used to measure total nitrogen and available nitrogen in the soil, respectively. After germination, 7 health plants of similar size were retained in each pot for the experiment. Each treatment was replicated 10 times (pots), totaling 20 pots. Thrity days after transplanting the seedling into pots, they began the warming treatment (last for 90 days) when the seedling looks a similar maturity as matue Leymus chinensis individuals. During this period, to avoid accelerated soil evaporation, pots wer watered at 5:00 p.m. daily to maintain a standard weight (50%~55% of field capacity) in both control and warming treatments. To ensure uniform lighting for each plant, the position of the each pot was tookturned every 2 days.
2.3 Leaf Area and Chlorophyll a, b Content
We used AM-350 leaf area meter (ADC BioScientific Ltd., Hoddesdon, UK) to measure leaf area. The criteria for leaf selection are as follows: 4 pots were randomly selected in each treatment, and 2 to 3 plants were randomly selected in each pot. Subsequently, the youngest and fully expanded leaf weighing 0.05 g from the parent shoot were extracted 48 h using a 10 ml 80% acetone solution in the dark condition. Chlorophyll a and b were measured at 663 and 646 nm, respectively, using a UV-5500 ultraviolet-visible spectrophotometer (Shanghai Meta-Analysis Instrument Co., Ltd., Shanghai, China).
2.4 Gas Exchange and Chlorophyll Fluorescence
In both the warming and control treatments, 20 parent shoot leaves from 4 pots were randomly selected to determine gas exchange parameters. Net photosynthetic rate (A), stomatal conductance (Gs), intercellular CO2 concentration (Ci), transpiration rate (E), etc., were measured using a LI-6400XT portable photosynthesis system equipped with a Li-Cor red/blue LED light source (LI-6400XT, Li-Cor, Inc., Lincoln, NE, USA). The photosynthetically active radiation (PAR) was set at 1000 μmol−2 s−1 and the reference CO2 concentration was maintained at 380 μmol mol−1 using CO2 injector. Water use efficiency (WUE) is calculated by A/E.
Chlorophyll fluorescence parameters were determined using a LI-6400XT portable photosynthesis system, paired with a LI-6400-40 Leaf Chamber Fluorometer (LI-6400XT, Li-Cor, Inc., Lincoln, NE, USA). Initially, the labeled parent shoot was placed in darkness for 12 h, and then measured the base fluorescence (F0), maximal fluorescence (Fm) and variable fluorescence (F) under darkness. Subsequently, all samples were placed in light conditions for 2 h, and measured the basic fluorescence (F0′), maximum fluorescence (Fm′) and steady-state fluorescence (Fs). During those measurements, CO2 concentration was maintained at 380 μmol mol−1 using the injection system. The maximum quantum efficiency of PSII photochemistry (Fv/Fm),: the actual quantum yield (Φp), the photochemical quenching coefficient (qP), and the non-photochemical quenching coefficient (qN) was calulated by: Fv/Fm = (Fm − F0)/Fm, Φp = (Fm′ − Fs)/Fm′, qP = (Fm′ − F)/(Fm′ − F0′), qN =1 − (Fm′ − F0′)/(Fm − F0), respectively [28].
2.5 Biomass, Bud Bank and Daughter Shoot Number
After finished all determines in gas exchange and chlorophyll fluorescence parameter, move each plant together with root out of the soil to determine the biomass and the bud bank. We counted the number of bud (with a length > 2 mm, exhibiting either white color or a brown growing point), the number of daughter shoots (emerging above the soil surface with green growing point) per parent shoot. Finally, the parent shoots, daughter shoots, roots were separated to determine dry biomass after oven-dried at 65°C for 48 h.
SPSS 22.0 was used for statistical analysis (SPSS for Windows, Chicago, IL, USA). Tested for normality was performed with the method of the Shapiro-Wilk statistic. For the data satisfied the assumption of normality, an independent sample t-test was employed to test the significances between two treatments. For variables of A, Gs, Ci, WUE, E, which did not meet the assumption and normality, significance testing was conducted using Wilcoxon-Mann-Whitney U method.
3.1 Biomass Production and Bud and Daughter Shoot Number
In contrast to the control, warming significantly reduced the parent shoot and daughter shoot biomass per plant by 25.52% (p < 0.05) and 33.45% (p < 0.05), respectively (Fig. 1). There was no significant impact of warming on root biomass (Fig. 1). Consistent with the change trend in biomass, warming also significantly reduced the number of daughter shoot per plant by 33.33% (p < 0.05) (Fig. 2). Conversely, there was a substantial increase of 46.43% in the buds number per plant (p < 0.05) (Fig. 2).
Figure 1: Dry matter mass (including mother shoot biomass, daughter shoot biomass and root biomass) of warming and without warming (Control) in Leymus chinensis. Data are presented as mean ± SE (n = 4). *p < 0.05
Figure 2: Bud number and daughter shoot number of warming and without warming (Control) in Leymus chinensis. Data are presented as mean ± SE (n = 12). *p < 0.05
3.2 Leaf Area, Chlorophyll Content, and Gas-Exchange Parameters
Summer warming significantly decreased the leaf area per plant by 18.03% (p < 0.05) (Fig. 3). In contrast to the control, chlorophyll a and b content was significantly decreased by 18.27% (p < 0.05) and by 29.21% (p < 0.05), respectively (Fig. 3).
Figure 3: Chlorophyll a and b content, leaf area of warming and without warming in Leymus chinensis (Control). Data are presented as mean ± SE. The sample size of the chlorophyll and the leaf area is 4 and 12, respectively. *p < 0.05
In contrast to control, warming separately, significantly reduced the net photosynthetic rate, transpiration rate and stomatal conductance by 7.32% (p < 0.05), 26.55% (p < 0.05) and 29.84% (p < 0.05). However, warming significantly increased intercellular carbon dioxide concentration by 3.51% (p < 0.05), increased water use efficiency by 28.34% (p < 0.05) (Table 2).
3.3 Chlorophyll Fluorescence Parameters
Compared to the control, summer warming resulted in a significant reductions in the maximum quantum efficiency of PSII photochemistry, the actual quantum yield, and photochemical quenching coefficient by 4.29% (p < 0.05), 23.84% (p < 0.05) and 9.63% (p < 0.05), respectively (Table 3). Additionally, warming significantly increased the non-photochemical quenching coefficient by 4.38% (p < 0.05), while only marginal increased the base fluorescence by 5.43% (p > 0.05) (Table 3).
In arid and semi-arid regions, climate changes exert a significant influence on plant carbon uptake and biomass production [7–9]. Our experimental results show that summer warming significantly decreased the leaf area, chlorophyll a and b content, net photosynthetic rate of parent shoot, and biomass production in Leymus chinensis. This aligns with the results of Leonsanchez et al. [29], who found that the net photosynthetic rate, leaf area and biomass production of Helianthemum squamatum shrubs were significantly reduced in the semi-arid Mediterranean ecosystem under warming (+2°C). Besides gas exchanges, summer warming also led to significant reductions in the maximum quantum efficiency of PSII photochemistry (Fv/Fm), the actual quantum yield (Φp) and photochemical quenching coefficient (qP). That is, leaf photosynthetic capability decreased in a summer warming environment. The reduction in Fv/Fm observed in our study was attributted to an increase in F0, indicating that whole summer warming stressed growth and damaged optical system, resulting irreversible declined of maximum quantum yield [30]. The degree of non-radiative energy dissipation in PSII is reflected by the non-photochemical quenching (qN) reaction, with an increase of it illustrated that the ability of light protection and non-radiative losses enhanced [9,31]. Previous study in Stylosanthes capitata Vogel, an important leguminous forage in tropical and subtropical regions, showed that warming (+2°C) significant increases in leaf area index and biomass, while there were no significant differences in the Fv/Fm and the qN. However, elevated temperature enhance the Vcmax and Jmax, thereby resulting in an increase in the maximum photosynthetic rate [21] In fact, high temperatures can alter the activities of various enzymes involved in wheat photosynthesis, resulting in a decrease in photosynthesis rate, accompanied by abnormal respiration, stomatal closure, and decreased leaf water use efficiency [32]. It is inconsistent with our research may be due to different plant functional types (PFTs), geographical locations, or the synergistic effects of atmospheric carbon dioxide concentration [33–35]. This study shows that 3°C summer warming did reduce the net photosynthetic rate and biomass accumulation through the stoma and non-stoma limitation.
In the study, prolonged summer warming obviouly increased the number of belowground bud, whereas reduced the number of daughter shoot (no death of daughter shoot were found) and total aboveground biomass. These results indicated that warming limits the conversion of belowground buds into daughter shoots when the carbon assimilaration of the parent shoot is constrained by warming. This finding contradicts our initial predictions (ii), and suggests that warming leads a relatively higher allocation of biomass to belowground structures to support the survival and growth of the buds. This result differs from the previous studies on nocturnal warming, which shows warming during growing season significantly increased leaf respiration rates at night, thereby promoting carbohydrate accumulation during the daytime [36], resulting in increased biomass production of the parent shoot. For perennial clonal plants, there exists a clear physiological integration behavior where the translocation of limiting resources can affect the growth of both the parent and daughter shoots. According to the physiological integration model, the translocation of essential nutrients such as carbohydrates from the parent shoot can improve the growth of daughter shoots under adverse environmental conditions. However, our strictly controlled temperature experiment yielded different results, as it demonstrated an increase in the bud bank size but not in daughter shoots. In summary, Leymuc chinensis, a typical perennial grass, experienced reduced productivity in both the current parent shoot and daughter shoots under prolonged summer warming conditon. However, the increased allocation of biomass to belowground organs, such as buds, can enhance the number of future tillers and help maintain population density. It is worth noting that despite the summer warming experiment start from seedlings, which have a similar appearance to the matue Leymus chinensis. Whereas, given that this species is long-lived, the responses to warming may vary with age. Therefore, further research should compare the detailed differences among different ages to better understand population dynamics under ongoing global warming.
We show here that the extension of warming time in summer significantly reduced the biomass of both parent and daughter shoots in Leymus chinensis. The decrease in parent shoot biomass can be attributed to a decrease in the photosynthetic yield, while the decrease in daughter biomass is linked to a decrease in the density of daughter shoot. Conversely, summer warming induces a notable augment in the density of belowground buds. It implied that despite the whole summer warming decreasing both current tillers density and productivity, the rise in the number of belowground buds may compensate for this loss in density and productivity in the future. This study not only provides references for a better understanding of how the current productivity responds to summer warming but also offers a cue to predict population development trends of Leymus chinensis and other rhizomatous perennial grasses under future global warming.
Acknowledgement: We would like to express our gratitude for the technical support provied by Xiuquan Yue, Yanan Li during data experiment. Additionally, we extend our appreciation to editors and anonymous reviewers for their valuable contructive comments.
Funding Statement: The research was funded by the NSFC (32371669), the Science and Technology Talent Project for Distinguished Young Scholars of Jilin Province (20240602009RC), the NSF of Jilin Province (20240101207JC), and the Scientific Research Project of the Department of Education, Jilin Province (JJKH20230687KJ).
Author Contributions: Song Gao collected, alalyzed data, and drafted the manuscript. Junfeng Wang (corresponding author) conceived, designed the research, and revised manuscript. Johannes M. H. Knops revised manuscript and the rest of the authors helped review of the manuscript. All authors reviewed the results and approved the final version of the manuscript.
Availability of Data and Materials: All data included in this study are available upon request by contact with the corresponding author.
Ethics Approval: Not applicable.
Conflicts of Interest: The authors declare that they have no conflicts of interest to report regarding the present study.
References
1. Harper JL. Population biology of plants. New York: Academic Press; 1977. [Google Scholar]
2. Teixeira AM, Curran TJ, Jameson PE, Meurk CD, Norton DA. Post-fire resprouting in New Zealand woody vegetation: implications for restoration. Forests. 2020;11(3):269. doi:10.3390/f11030269. [Google Scholar] [CrossRef]
3. Qian J, Wang Z, Liu Z, Busso CA. Belowground bud bank responses to grazing intensity in the Inner-Mongolia steppe, China. Land Degrad Dev. 2017;28(3):822–32. doi:10.1002/ldr.v28.3. [Google Scholar] [CrossRef]
4. Liu L, Zuo S, Ma M, Li J, Guo L, Huang D. Appropriate nitrogen addition regulates reproductive strategies of Leymus chinensis. Glob Ecol Conserv. 2021;27:e01599. [Google Scholar]
5. Wang P, Li H, Pang XY, Wang A, Dong BC, Lei JP, et al. Clonal integration increases tolerance of a phalanx clonal plant to defoliation. Sci Total Environ. 2017;593:236–41. [Google Scholar] [PubMed]
6. Cao XX, Xue W, Lei NF, Yu FH. Effects of clonal integration on foraging behavior of three clonal plants in heterogeneous soil environments. Forests. 2022;13(5):696. doi:10.3390/f13050696. [Google Scholar] [CrossRef]
7. Qi J, Nie J, Zhang Y, Xu S, Li Z, Zhang D, et al. Plastic film mulching does not increase the seedcotton yield due to the accelerated late-season leaf senescence of short-season cotton compared with non-mulching. Field Crop Res. 2022;287:108660. doi:10.1016/j.fcr.2022.108660. [Google Scholar] [CrossRef]
8. Blankenship RE. Early evolution of photosynthesis. Plant Physiol. 2010;154(2):434–8. doi:10.1104/pp.110.161687. [Google Scholar] [PubMed] [CrossRef]
9. Gray SB, Brady SM. Plant developmental responses to climate change. Dev Biol. 2016;419(1):64–77. doi:10.1016/j.ydbio.2016.07.023. [Google Scholar] [PubMed] [CrossRef]
10. Drebenstedt I, Schmid I, Poll C, Marhan S, Kahle R, Kandeler E, et al. Effects of soil warming and altered precipitation patterns on photosynthesis, biomass production and yield of barley. J Appl Bot Food Qual. 2020;93:44–53. [Google Scholar]
11. Ruizvera UM, Siebers M, Gray SB, Drag DW, Rosenthal DM, Kimball BA, et al. Global warming can negate the expected CO2 stimulation in photosynthesis and productivity for soybean grown in the Midwestern United States. Plant Physiol. 2013;162(1):410–23. doi:10.1104/pp.112.211938. [Google Scholar] [PubMed] [CrossRef]
12. Siebers MH, Yendrek CR, Drag D, Locke AM, Rios Acosta L, Leakey AD, et al. Heat waves imposed during early pod development in soybean (Glycine max) cause significant yield loss despite a rapid recovery from oxidative stress. Global Change Biol. 2015;21(8):3114–25. doi:10.1111/gcb.2015.21.issue-8. [Google Scholar] [CrossRef]
13. Haider S, Palm S, Bruelheide H, de Villemereuil P, Menzel A, Lachmuth S. Disturbance and indirect effects of climate warming support a plant invader in mountains. Oikos. 2022;(4):e08783. [Google Scholar]
14. Hebbar KB, Apshara E, Chandran KP, Prasad PV. Effect of elevated CO2, high temperature, and water deficit on growth, photosynthesis, and whole plant water use efficiency of cocoa (Theobroma cacao L.). Int J Biometeorol. 2020;64:47–57. doi:10.1007/s00484-019-01792-0. [Google Scholar] [PubMed] [CrossRef]
15. Li F, Peng Y, Zhang D, Yang G, Fang K, Wang G, et al. Leaf area rather than photosynthetic rate determines the response of ecosystem productivity to experimental warming in an alpine steppe. J Geophys Res-Biogeo. 2019;124(7):2277–87. doi:10.1029/2019JG005193. [Google Scholar] [CrossRef]
16. Valencia E, Mendez M, Saavedra N, Maestre FT. Plant size and leaf area influence phenological and reproductive responses to warming in semiarid Mediterranean species. Perspect Plant Ecol. 2016;21:31–40. doi:10.1016/j.ppees.2016.05.003. [Google Scholar] [PubMed] [CrossRef]
17. Bernacchi CJ, Ruiz-Vera UM, Siebers MH, DeLucia NJ, Ort DR. Short- and long-term warming events on photosynthetic physiology, growth, and yields of field grown crops. Biochem J. 2023;480(13):999–1014. doi:10.1042/BCJ20220433. [Google Scholar] [PubMed] [CrossRef]
18. Jin B, Wang L, Wang J, Jiang KZ, Wang Y, Jiang XX, et al. The effect of experimental warming on leaf functional traits, leaf structure and leaf biochemistry in Arabidopsis thaliana. BMC Plant Biol. 2011;11:1–0. [Google Scholar]
19. Li J, Liang Y, Li J, Li X, Yang J. Analysis of plant ecological strategies based on leaf functional traits on the Western slope of Helan mountain. Energy Ecol Environ. 2024;33(1):45. [Google Scholar]
20. Baker NR. Chlorophyll fluorescence: a probe of photosynthesis in vivo. Annu Rev Ecol Evol S. 2008;59:89–113. [Google Scholar]
21. Li YT, Xu WW, Ren BZ, Zhao B, Zhang J, Liu P, et al. High temperature reduces photosynthesis in maize leaves by damaging chloroplast ultrastructure and photosystem II. J Agron Crop Sci. 2020;206(5):548–64. doi:10.1111/jac.v206.5. [Google Scholar] [CrossRef]
22. Marchand FL, Mertens S, Kockelbergh F, Beyens L, Nijs I. Performance of high arctic tundra plants improved during but deteriorated after exposure to a simulated extreme temperature event. Global Change Biol. 2005;11(12):2078–89. doi:10.1111/gcb.2005.11.issue-12. [Google Scholar] [CrossRef]
23. Wang JF, Gao S, Lin JX, Mu YG, Mu CS. Summer warming effects on biomass production and clonal growth of Leymus chinensis. Crop Pasture Sci. 2010;61(8):670–6. doi:10.1071/CP10012. [Google Scholar] [CrossRef]
24. Ott JP, Butler JL, Rong Y, Xu L. Greater bud outgrowth of Bromus inermis than Pascopyrum smithii under multiple environmental conditions. J Plant Ecol. 2017;10(3):518–27. [Google Scholar]
25. Chen MJ, Jia SX. Fodder plants of China. Beijing: Agriculture Press of China; 2002. [Google Scholar]
26. Shi YJ, Gao S, Zhou DH, Liu MX, Wang JF, Knops JM, et al. Fall nitrogen application increases seed yield, forage yield and nitrogen use efficiency more than spring nitrogen application in Leymus chinensis, a perennial grass. Field Crop Res. 2017;214:66–72. doi:10.1016/j.fcr.2017.08.022. [Google Scholar] [CrossRef]
27. Shi YJ, Wang JF, Roux XL, Mu CS, Ao YN, Gao S, et al. Trade-offs and synergies between seed yield, forage yield, and N-related disservices for a semi-arid perennial grassland under different nitrogen fertilization strategies. Biol Fert Soils. 2019;55:497–509. doi:10.1007/s00374-019-01367-6. [Google Scholar] [CrossRef]
28. Peng JF, Feng YH, Wang XK, Li J, Xu GL, Phonenasay S, et al. Effects of nitrogen application rate on the photosynthetic pigment, leaf fluorescence characteristics, and yield of indica hybrid rice and their interrelations. Sci Rep. 2021;11(1):7485. doi:10.1038/s41598-021-86858-z. [Google Scholar] [PubMed] [CrossRef]
29. Leonsanchez L, Nicolas E, Nortes PA, Maestre FT, Querejeta JI. Photosynthesis and growth reduction with warming are driven by nonstomatal limitations in a Mediterranean semi-arid shrub. Ecol Evol. 2016;6(9):2725–38. doi:10.1002/ece3.2016.6.issue-9. [Google Scholar] [CrossRef]
30. Cuadra P, Fajardo V, Pimentel P, Moya-Leon MA, Herrera R. Changes in Chlorophyll a fluorescence and DNA as a plant response to UV-B radiation in Gnaphalium vira-vira. Pol Polar Res. 2022;43(4):325–39. [Google Scholar]
31. Martinez CA, Bianconi M, Silva L, Approbato A, Lemos M, Santos L, et al. Moderate warming increases PSII performance, antioxidant scavenging systems and biomass production in Stylosanthes capitata Vogel. Environ Exp Bot. 2014;102:58–67. doi:10.1016/j.envexpbot.2014.02.001. [Google Scholar] [CrossRef]
32. Qaseem MF, Qureshi R, Shaheen H. Effects of pre-anthesis drought, heat and their combination on the growth, yield and physiology of diverse wheat (Triticum aestivum L.) genotypes varying in sensitivity to heat and drought stress. Sci Rep. 2019;9(1):6955. doi:10.1038/s41598-019-43477-z. [Google Scholar] [PubMed] [CrossRef]
33. Lin D, Xia J, Wan SQ. Climate warming and biomass accumulation of terrestrial plants: a meta-analysis. New Phytol. 2010;188(1):187–98. doi:10.1111/nph.2010.188.issue-1. [Google Scholar] [CrossRef]
34. Wu Z, Dijkstra P, Koch GW, Penuelas J, Hungate BA. Responses of terrestrial ecosystems to temperature and precipitation change: a meta-analysis of experimental manipulation. Global Change Biol. 2011;17(2):927–42. doi:10.1111/gcb.2010.17.issue-2. [Google Scholar] [CrossRef]
35. Luo WT, Muraina TO, Griffin-Nolan RJ, Ma W, Song L, Fu W, et al. Responses of a semiarid grassland to recurrent drought are linked to community functional composition. Ecology. 2023;104(2):e3920. doi:10.1002/ecy.v104.2. [Google Scholar] [CrossRef]
36. Li ZL, Lin JX, Zhang T, Zhang N, Mu CS, Wang J. Effects of summer nocturnal warming on biomass production of Leymus chinensis in the Songnen Grassland of China: from bud bank and photosynthetic compensation. J Agron Crop Sci. 2014;200(1):66–76. doi:10.1111/jac.2013.200.issue-1. [Google Scholar] [CrossRef]
Cite This Article
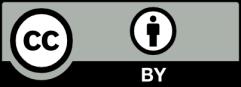
This work is licensed under a Creative Commons Attribution 4.0 International License , which permits unrestricted use, distribution, and reproduction in any medium, provided the original work is properly cited.