Open Access
ARTICLE
Combined Application of Biostimulants and EDTA Improved Wheat Productivity under Cadmium Stress
1 Department of Botany, The Women University, Multan, 54500, Pakistan
2 Institute of Agronomy, Bahauddin Zakariya University, Multan, 60800, Pakistan
3 Department of Forestry and Range Management, Bahauddin Zakariya University, Multan, 60800, Pakistan
4 Department of Botany, Division of Science & Technology, University of Education, Lahore, 54770, Pakistan
5 Department of Microbiology & Molecular Genetics, The Women University, Multan, 54500, Pakistan
6 Botany and Microbiology Department, College of Science, King Saud University, Riyadh, 201313, Saudi Arabia
7 Amity Institute of Biotechnology, Amity University, Noida, 201313, India
8 Plant Production Department, College of Food and Agricultural Sciences, King Saud University, Riyadh, 11451, Saudi Arabia
9 School of Agriculture, Yunnan University, Kunming, Yunan, 650504, China
10 Department of Environmental Sciences, The University of Lahore, Lahore, 54590, Pakistan
* Corresponding Author: Qamar uz Zaman. Email:
(This article belongs to the Special Issue: Abiotic Stress Impacts on Plant Physiology and Their Alleviation)
Phyton-International Journal of Experimental Botany 2024, 93(7), 1647-1665. https://doi.org/10.32604/phyton.2024.050974
Received 24 February 2024; Accepted 30 May 2024; Issue published 30 July 2024
Abstract
Wheat (Triticum aestivum L.) exhibits a greater capacity for cadmium (Cd) absorption compared to other cereal crops, leading to elevated daily Cd intake, and posing a significant threat to public health. For the mitigation of Cd stress in sustainable and environmentally friendly way, a pot study was designed by using exogenous application of various biostimulants, i.e., Nigella sativa and Ocimum sanctum extracts: 0%, 10%, and 20% in combination with the chelating agent ethylenediaminetetraacetic acid (EDTA) using 0 and 5 mg kg under various levels of Cd stress (i.e., 0, 5, 10, and 15 mg kg soil). Results revealed that Cd stress significantly reduced the seed emergence, growth, root, and allometric characters and yield-related parameters of wheat crops. The most observable reduction was documented in wheat plants exposed to a higher Cd concentration (15 mg kg), followed by the lower Cd level (control). The combined application of bio-stimulants and EDTA minimized the negative impacts of Cd stress. The highest increase in seedling emergence (5.44%), leaf area (50.60%), number of tillers (31.02%), grain yield per plant (24.28%), biological yield (13.97%), and decrease in Cd levels in grains (40%) was noticed where 20% foliar application of N. sativa and 10% of O. sanctum biostimulants were done using 5 mg kg of soil-applied EDTA. This intervention demonstrated a notable reduction in Cd-induced negative effects, highlighting the potential of these substances in promoting sustainable wheat cultivation in contaminated environments. Moreover, it is an eco-friendly and approachable method at the field level able to ensure food safety.Graphic Abstract
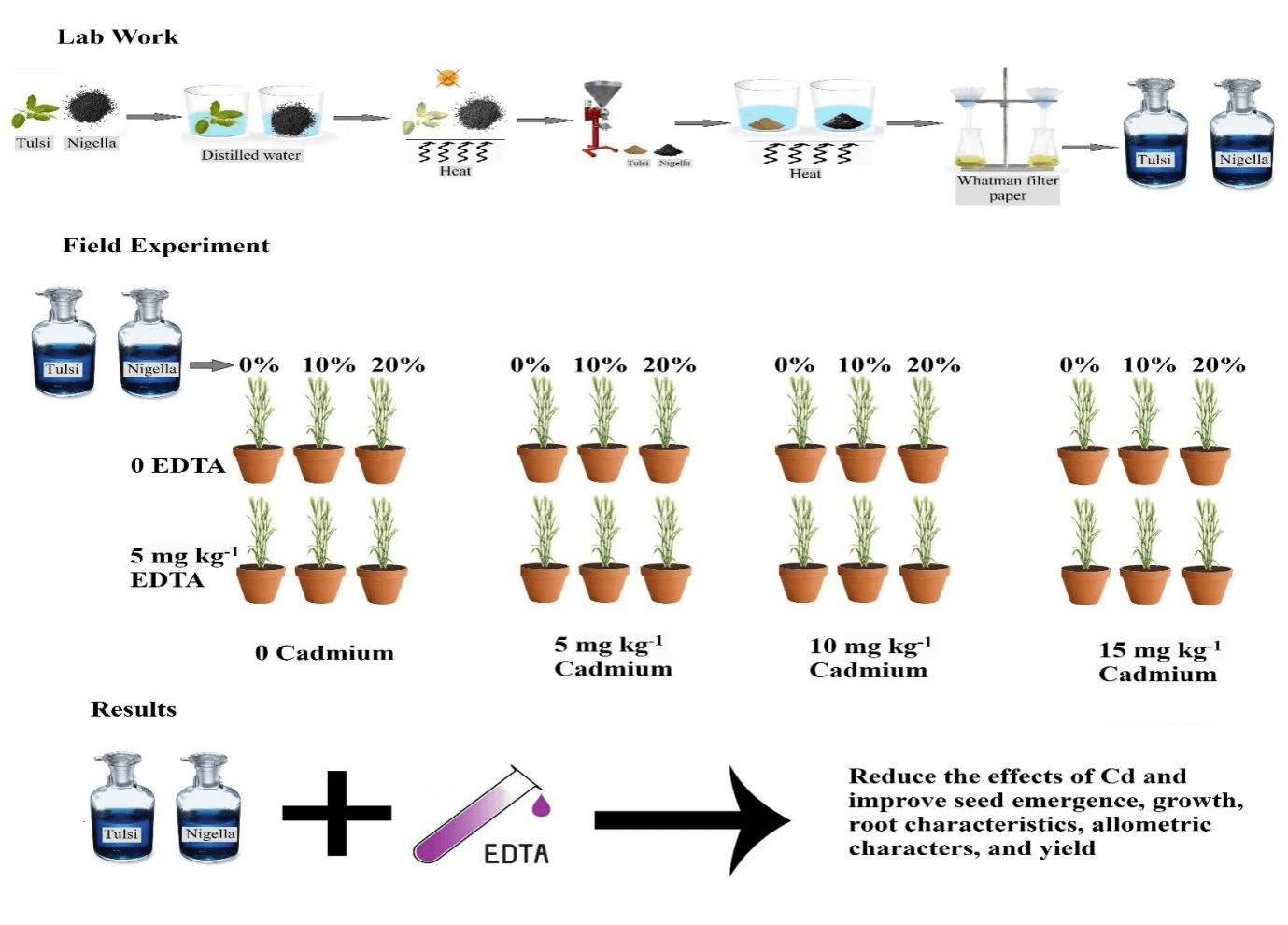
Keywords
The presence of heavy metals in the rhizosphere poses a significant health threat to the global population and raises concerns among environmentalists [1]. Cadmium (Cd), commonly released from industrial activities, fertilizer overuse, and natural processes like rock weathering, has become a pervasive environmental issue [2]. Uncontrolled industrialization, urbanization, and inadequate environmental planning contribute to severe yield reductions in cereal crops [3].
Wheat exhibits a higher Cd absorption ability than other cereal crops [4,5]. The substantial health risk associated with the possibility of excessive Cd consumption from grains is especially acute in developing nations, where wheat accounts for >80% of staple foods [6]. The Cd uptake in wheat occurs through roots, translocating to aerial parts, disrupting vital metabolic processes, and hindering normal growth and reproduction [7]. Elevated Cd levels in the rhizosphere induce severe phytotoxic effects, affecting plant morphology, physiology, and biochemistry, including the destruction of leaf chlorophyll structure, leading to altered photosynthetic and gas exchange functions [8]. The susceptibility of the photosynthetic system to environmental changes underscores the critical role it plays in plant growth and survival [9].
To mitigate the presence of toxic metals in the food chain, various biological, chemical, and physical methods are employed on contaminated soils [10]. Among these biological approaches, phytoremediation emerges as an environmentally friendly technique [11], utilizing plants to remediate soil contaminated with various metals [12]. Natural bio-stimulants are the most promising and suitable strategy to address yield losses caused by various stresses [13]. Bio-stimulants possess many diverse compounds, with positive effects on plants to increase growth, diminish stress-induced restrictions, and enhance yield [14]. The use of plant extracts, which are rich in bioactive compounds, demonstrated notable efficacy in ameliorating Cd-induced toxicity [15]. Plant extracts played a crucial role in mitigating the adverse effects of metal toxicity on wheat by enhancing the plant’s natural defense mechanisms and detoxification processes [16]. Through its bioactive constituents, the plant seed extract appeared to promote overall plant health, leading to improved growth and productivity under Cd stress conditions [17]. Similarly, the chelating agents are recognized for their ability to facilitate the accumulation of heavy metals in various plant tissues. EDTA is a chelating agent and proved beneficial in the remediation of contaminated soils [18,19]. The EDTA effectively eliminates or renders toxic microbes and metals in the soil unavailable to plants [20]. The impact of EDTA in reducing Cd accumulation was evident in Pelargonium spp. cultivated in Cd-contaminated soil [21].
Recent investigations have demonstrated that bio-stimulants and EDTA protect various crop plants from heavy metals, however, the combined application of various bio-stimulants and EDTA for the mitigation of Cd stress has limited literature. Cadmium is one of the most toxic soil metals which reduces the growth and yield of wheat; hence, extensive research is needed to reduce heavy metal stress-related yield losses. To our knowledge, no study has been conducted on the combined effect soil applied EDTA and foliar-applied bio-stimulants to alleviate the Cd toxicity in wheat. Given these findings, the current study was designed to mitigate Cd toxicity and improve the growth and yield of wheat crops. This study hypothesized that soil-applied EDTA and foliar application of bio-stimulants could improve plants’ growth and yield under various levels of Cd stress.
A pot experiment was conducted in the wire net house of Agronomic Research Centre, Bahauddin Zakariya University Multan, Pakistan during 2021–22 under natural environmental conditions. Uniformed-sized earthen pots were used with 10 kg of sandy loam soil. The physiochemical properties of soil were determined using standard protocols by taking a composite sample (0–20 cm depth) of soil before filling the pots. The initial values of soil-related attributes were pH = 8.1 pH, soil organic matter = 0.34%, soil EC = 2.04 m Scm−1, available potassium 210 mg kg−1, available phosphorus = 6.68 mg kg−1, and Cd contents were 0 mg kg−1.
2.2 Preparation of Plant Extracts
For the preparation of extracts, leaves, and seeds (Tulsi and Nigella) samples of both plants were obtained from the local market. To avoid contamination, samples were rinsed with distilled water, air dried, and subjected to low heat, avoiding direct sunlight, until they became crisp. Once dried, the leaves and seeds samples were ground using a Mortar and Pestle. After that, the powder was mixed with distilled water and heated till boiling. The mixture was allowed to steep for about 15–20 min. After steeping, filtration was done using Whatman filter paper. The stock solutions of both samples were stored in separate bottles to prepare the desired dilutions for further use.
A completely randomized design (CRD) under factorial arrangement was applied with 3 replicates. The total number of experimental units was [4 (Cd concentrations) × 2 (EDTA levels) × 3 (biostimulants) × 3 (replications) = 72]. Experimental treatments comprised of three factors, i.e., four Cd concentrations (0, 5, 10, and 15 mg kg−1 of soil), two EDTA levels (0 and 5 mg kg−1), and three concentrations of plant extracts (0%, 10%, and 20%).
A mixed culture of soil EDTA and Cd levels as per treatment was prepared separately and applied to pots. All the pots were kept under shade for one month so that the treatments were homogenized in soil using manual hoeing. All pots were kept moist until the wheat seeds were planted. The wheat seeds after decontamination with 0.1% (w/v) sodium dodecyl sulfate solution were thoroughly washed with deionized water and sown in the pots. Eight seeds were sown in each pot after that thinned to 5 seedlings per pot when shoots reached 5 cm height. The soil was fertilized with phosphorous (P) and nitrogen (N) at the rate of 200 (kg ha−1) and 150 (kg ha−1) by using di-ammonium phosphate (DAP) and urea. At the time of planting, the entire DAP was used, whereas urea was applied in two splits (1st at the sowing time, 2nd after 25 days after sowing (DAS). First irrigation was applied at 2 DAS and other irrigation was applied to keep up the best possible dampness level with 2 to 3 days’ interval relying upon the harvesting necessity. The foliar spray of PE (plant extract) was applied in two equal splits at 25 and 45 DAS. In 0% PE treatment, distilled water was sprayed. All the below-mentioned attributes were recorded with and without treatment. All the measurements were performed according to the set criterion to reduce the difference caused by determination time.
2.5 Determination of Seedling Emergence and Stand Establishment Attributes
From the second day after sowing, data were recorded to count the seedlings emergence. E50 (Time taken to 50% emergence) was calculated using Eq. (1) formulated by [22]
(1)
where N is the final number of emerged seedlings, and ni and nj are the cumulative number of seedlings that emerged on two adjacent days when n < MET (Mean emergence time) was recorded by Eq. (2) formulated by [23]
(2)
where n stands for the number of seedlings that emerged on D Day and D stands for the number of days counted from the onset of emergence. The final emergence count was measured in percentage and considered as the final germination percentage by counting the total number of seeding emerged at the final count.
2.6 Allometric and Root Traits
The allometric traits (plant growth and leaf area) and root traits (root elongation rate and root length) were measured at a 15-day interval starting from 55 DAS and terminated at 100 DAS. One plant was randomly selected and uprooted carefully from each pot on every data measurement day. After washing roots with tap water, the length was recorded with measuring tape, and the average for each experimental treatment was calculated. For leaf area, leaves were plucked from the intact plant and manually measured the average width and length of the leaf using a scale. The shoot and roots were dried at 75°C ± 5°C for 72 h in an oven. The root dry weight was calculated by Eq. (3) formulated by [24]
(3)
where W1 is the plant’s dry biomass at the first harvest W2 is the plant’s dry biomass at the second harvest and t2 and t1 are the DAS of the second and first harvests, respectively.
2.7 Agronomic and Yield-Related Traits
The yield data and related traits were noted at plant maturity. Plant height was measured with the help of measuring tape from the bottom to spikes awns after that average was calculated to obtain a single reading. The number of tillers was counted from the wheat plant of each pot. The spike length from every pot was measured with a measuring tape and the average was determined the length of the individual. Three plants from each pot were collected at maturity and sun-dried. After drying for three days, biological yield-related parameters were measured. The spikes were threshed physically to calculate the grain yield per plant. The spikes were threshed with hands and the number of grains per spike was noted.
Cd accumulation was determined in the wheat grains. The grains were ground to powder with a grinding mill. After a two-step acid digestion procedure, samples were analyzed on an Atomic Absorption Spectrometer (Ice9 3000 SERIES) to determine Cd accumulation and expressed as mg kg−1 of the plant biomass.
Analysis of variance was used to statistically evaluate the collected data using Statistix 8.1 at a probability level of 5%. Least significance difference (LSD) was used to compare the treatment means [25].
3.1 Seed Emergence and Early Stand Formation
Time to start emergence, mean emergence time, emergence 50% (E50%), and final emergence (%) EDTA at 0 mg kg−1 showed better results as compared to 5 mg kg−1. All Cd levels took some days to start emergence while means emergence time, E50%, and final emergence % were not changed. While two ways the interaction between EDTA and Cd had a significant effect on the start of emergence, time, E50%, and non-significant effect on the final emergence percentage (Table 1).
3.2 Root-Related and Allometric Traits
Root and Allometric parameters were decreased by rising Cd concentration; though, BCE (Bio-stimulants Extract) and EDTA decreased the unfavorable effect to some level. On the whole, plant extracts showed a maximum number of roots and root lengths under all Cd concentrations (Figs. 1–3). The highest and lowest number of roots and root length were observed under 0 and 15 mg kg−1 Cd concentration, correspondingly. Application of 10% Tulsi leave extract (TLE), showed the highest root length at 70 and 80 DAS, especially with the combination of 5 mg kg−1 EDTA at a lower 5 mg kg−1 Cd concentration. The appliance of 20% BCE has induced a maximum root number and length than the rest of the PE treatments (Figs. 1 and 2). Similarly, EDTA enhanced the number of roots and root length in all Cd concentrations as compared to those pots where EDTA was not applied. Applying EDTA along 20% Nigella seed extract (NSE) performs better results in all Cd concentrations. Increasing concentration lowered plant growth rate (PGR), where a 20% NSE application rate improved it as compared to TLE. But 10% TLE enhanced plant growth rate at 85–100 DAS without EDTA application (E1) performs better as compared to E2. The highest and the lowest PGR were observed for 0 and 15 mg kg−1 Cd concentration, respectively (Fig. 4). EDTA application counteracts the Cd effect in PGR with both plant extracts. Overall, Nigella seed extract improved better growth rate as compared to Tulsi leaf extract with EDTA application (Tables 2 and 3). Plant height was significantly changed by plant extracts, Cd, and EDTA concentrations (Fig. 5) however, plant extracts showed a non-significant effect on leaf area as indicated in Table 4. Increasing Cd level decreased plant height and leaf area while these qualities were enhanced with 10% and 20% plant extract along EDTA, rather than no plant extract (PE1 = 0%) and EDTA application. Wheat plants grown under 0 mg kg−1 Cd concentration with 20% NSE had the maximum plant height and leaf area. The minimum values of these parameters were observed for wheat plants grown in 15 mg kg−1 Cd concentration without EDTA and no plant extract (Table 3 and Fig. 5A).
Figure 1: Estimating the function of exogenous application of Nigella sativa seeds extract and Ocimum sanctum along with chelating agent EDTA under cadmium stress on the root length of Triticum aestivum ± SE
Note: Cd1 = 0 mg kg−1, Cd2 = 5 mg kg−1, Cd3 = 10 mg kg−1, Cd4 = 15 mg kg−1 soil, PE1 = 0 (%) control, PE2= 10 (%) Ocimum sanctum extract, PE3 = 20 (%) Nigella sativa seeds extract, E1 = 0 (mg kg−1) EDTA application, E2 = 5 (mg kg−1) EDTA application.
Figure 2: Estimating the function of exogenous application of Ocimum sanctum and Nigella sativa seeds extract along with chelating agent EDTA under cadmium stress on the number of roots of Triticum aestivum ± SE
Note: Cd1 = 0 mg kg−1, Cd2 = 5 mg kg−1, Cd3 = 10 mg kg−1, Cd4 = 15 mg kg−1 soil, PE1 = 0 (%) Control, PE2 = 10 (%) Ocimum sanctum extract, PE3 = 20 (%) Nigella sativa seeds, E1 = 0 (mg kg−1) EDTA application, E2 = 5 (mg kg−1) EDTA application.
Figure 3: Estimating the function of exogenous application of Ocimum sanctum and Nigella sativa seeds extract along with chelating agent EDTA under cadmium stress on the leaf area of Triticum aestivum ± SE
Note: Cd1 = 0 mg kg−1, Cd2 = 5 mg kg−1, Cd3 = 10 mg kg−1, Cd4 = 15 mg kg−1 soil. PE1 = 0 (%) control, PE2 = 10 (%) Ocimum sanctum extract, PE3 = 20 (%) Nigella sativa seeds extract, E1 = 0 (mg kg−1) EDTA application, E2 = 5 (mg kg−1) EDTA application.
Figure 4: Estimating the function of exogenous application of Ocimum sanctum and Nigella sativa seeds extract along with chelating agent EDTA under cadmium stress on the plant growth rate of Triticum aestivum ± SE
Note: Cd1 = 0 mg kg−1, Cd2 = 5 mg kg−1, Cd3 = 10 mg kg−1, Cd4 = 15 mg kg−1 soil. PE1 = 0 (%) control, PE2 = 10 (%) Ocimum sanctum extract, PE3 = 20 (%) Nigella sativa seeds extract, E1 = 0 (mg kg−1) EDTA application, E2 = 5 (mg kg−1) EDTA application.
Figure 5: Interactive effect of foliar application of plant extracts and EDTA application on (A) plant height and (B) number of tillers per plant ± SE of wheat sown in cadmium-contaminated soil. Here, PE1 = 0 (%) control, PE2 = 10 (%) Ocimum sanctum extract, PE3 = 20 (%) Nigella sativa seeds extract, E1 = 0 (mg kg−1) EDTA application, E2 = 5 (mg kg−1) EDTA application. Means sharing the same letters is statistically non-significant (p > 0.05)
3.3 Agronomic and Yield-Related Traits
The individual Cd and interactive effects with PE and EDTA treatments considerably (p < 0.05) affect the agronomic and yield-related parameters, i.e., tiller numbers per plant (Fig. 5B), spike length, number of grain/spikes (Fig. 6), grain yield/plant (Fig. 7), and biological yield (Table 2). All these parameters were greatly reduced with rising Cd concentrations. Agronomic trait grows best at control 0 mg kg−1 Cd while at high Cd concentration, 15 mg kg−1 growth decreased (Tables 2 and 3). However, in PE treatment, 20% NSE enhanced these traits as compared to 10% TLE. Spike length and number of grain per spike were significantly improved by 20% application of NSE as compared to 10% TLE and 0%. Other traits such as grain yield and biological yield are improved by both 10% and 20% PE as compared to control. While no. of tiller per plant was non-significantly altered by plant extract (Table 3). EDTA application at 5 mg kg−1 had a significant effect on biological yield, number of tillers per plant, spike length, and number of grains per spike rather than 0 mg kg−1 EDTA (Table 3). While grain yield per plant was non-significantly a by EDTA concentration. Cd uptake in grain is higher in 15 mg kg−1 as compared to control similar result found in soil while PE and EDTA show no result.
Figure 6: Interactive effect of foliar application of plant extracts and EDTA application on (A) Spike length and (B) number of grains per spike ± SE of wheat sown in cadmium-contaminated soil. Here, PE1 = 0 (%) control, PE2 = 10 (%) Ocimum sanctum extract, PE3 = 20 (%) Nigella sativa seeds extract, E1 = 0 (mg kg−1) without EDTA application, E2 = 5 (mg kg−1) EDTA application. Means sharing the same letters is statistically non-significant (p > 0.05)
Figure 7: Interactive effect of foliar application of plant extracts and EDTA application on number of (A) grain yield per plant (B) soil cadmium (mg kg−1) with ± SE wheat sown in cadmium contaminated soil. Here, PE1 = 0 (%) control, PE2 = 10 (%) Ocimum sanctum extract, PE3 = 20 (%) Nigella sativa seeds extract, E1 = 0 (mg kg−1) without EDTA application, E2 = 5 (mg kg−1) EDTA application. Means sharing the same letters is statistically non-significant (p > 0.05)
The individual and interactive effects of Cd, PE, and EDTA concentrations significantly (p < 0.05) affect the soil cadmium (Table 2). Grain Cd level was significantly affected (p < 0.05) by Cd and plant extract concentrations while EDTA had a non-significant effect; two-way interaction between Cd and EDTA, and three-way interaction had a non-significant effect on grain Cd concentration (Table 2). However, 20% NSE significantly decreased the grain Cd level in wheat as compared to 10% TLE. The top Cd concentration resulted in a higher grain Cd concentration than the control (Table 3).
Cadmium (Cd) is ranked 7th among 20 major toxic environmental contaminants and grouped in the 1st category of the carcinogenic elements (24). Cd contaminated soil and water, a recently occurring problem in agriculture due to industrialization is damaging plants ranging from germination to suppressed growth and yield. Plants growing in Cd-contaminated soil uptake the heavy metal through their roots, which accumulates in different organs, eventually reducing plant growth and productivity. Cd’s adverse impact and potential strategies for its remediation were reported in various studies [26,27]. Phytoremediation or plant extract-based strategies are eco-friendly and are decisive for eliminating or reducing Cd toxicity. However, limited studies on Cd remediation strategies for wheat are discussed; therefore, in the present study, the effect of foliar application of various levels of novel bio-stimulants and soil-applied EDTA on different morphological and yield attributes of wheat under Cd stress was investigated.
Our results showed that different Cd levels did not affect final seed germination and early stand establishment which was supported by [28,29]. The lack of significance could be attributed that seeds could not ingest Cd in the germination process. Heavy metals in the soil have little effect on germination, according to [30], because seeds use their reserves throughout this process. As a result, germination was unaffected by any of the Cd doses utilized in the current investigation. Germination results under strong metal stress were comparable to a previous study [31]. The extent to which Cd poisoning slows seedling growth depends on the amount of metal in the soil [32]. Different environmental factors have an impact on how different plant species’ seeds germinate [24,33].
The result indicated that 5 mg kg−1 EDTA concentration did not affect seed germination. On the other hand, some researchers also observed non-significant effects of EDTA on metal uptake by plants. Sometimes, metal-EDTA complexes solutions are present in non-plant available form [27]. This could be caused by several variables, such as metal content, pH, capacity of cation, and chelated metal species [34]. Growth parameters with both EDTA concentrations were negatively affected by increasing Cd. The maximum negative effects were observed on several roots and root lengths in 0 mg kg−1 EDTA concentrations under higher Cd concentration as compared to 5 mg kg−1 EDTA. The conclusion of the present study is supported by various other researchers who mentioned that Cd has a deleterious impact on root structure and root growth was suppressed with increasing Cd concentrations [35,36]. Our results with respective to root parameters are in line with earlier reports [27,37].
These features may have declined as a result of Cd interfering with plant mineral intake, which would have disrupted its uptake and translocation from the root zone [38,39]. Cadmium stress in plants causes drastic changes in enzyme activity causing oxidative stress in plant cells [26,40]. Plant development and yield are decreased as a result of the phytotoxic effects of heavy metals, which cannot be fully mitigated by antioxidant enzymes [41]. Growth rate and height were significantly affected by higher Cd concentrations (Figs. 4 and 5A) whereas leaf area was non significantly affected as depicted in Fig. 3. These results are similar to numerous earlier studies in which plant growth rates reduced [42–44].
Reduced nutrient and water uptake under heavy metal stress results in a decline in plant growth and biomass [45,46]. This could also be a result of cadmium stress, which increases the synthesis of malondialdehyde and hydrogen peroxide in plants, leading to oxidative stress, which affects plant growth and development [29,47]. Leaf area and leaf number of wheat plants decreased by excess Cd [48], which might be due to the destruction of the ultra-structure portion of the chloroplast [49,50]. The negative effects of Cd on photosynthetic pigments such as Chlorophyll a, b, and carotenoids in plants are due to a range of interactions between Cd and plant metabolism. The increase in chlorophyllase production in leaves is reported to alter the biosynthesis of carotenoids and chlorophyll, leading to chlorophyll breakdown [51,52]. Additionally, decreasing the leaf area hurts the plant’s canopy, which unintentionally alters the plant’s height by obstructing the flow of oxygen because of photo radiation [53].
The grain yield of wheat is mainly determined by yield components, the number of grains per spike, and other yield-related traits, and these traits significantly decreased (Table 3). Experiments have shown that wheat under Cd stress had significantly lower height, tiller count, fresh and dry weight, flag leaf area, and yield analysis (weight, spike count, and weight of 100 grains) [51]. When plants are under Cd stress, it affects their physiology and yield, which results in decreased grain production [54,55]. After cd stress, the number of spikes, spike length, and spike weight all reduced, which resulted in a decrease in wheat yield [16]. Cd can have an impact on the yield, morphology of the plants, and the production of grain [54].
In our findings, EDTA concentration at 5 mg kg−1 shows better performance at high Cd level in root length and number of roots as compared to control (0 mg kg−1 EDTA). There is little research on how using EDTA, which is typically applied directly in polluted soil, can lower Cd levels in wheat roots [56,57]. The application of EDTA improved the wheat plant’s performance in our experiment by accelerating the plant growth rate. This was supported by [56]. When EDTA is combined with heavy metals, forms a metal-ligand-complex [20,21]. Adding EDTA to the soil enhances the solubility of Cd, but separating Cd-EDTA reduces the solubility of free Cd2+ on the root surface, thus reducing the amount of metal that plants can absorb [58–60]. It was noted in the current study that the application of EDTA improved the plant height and dry matter in wheat which is reliable with the findings of [61]. In the present study our findings similar to the [62] which demonstrated that, in the leaf, the promoting effect of EDTA on plant growth only occurred by EDTA application.
These findings demonstrate the value of EDTA in improving wheat plant tolerance to cadmium stress, as previously indicated by [63]. In our experiment, 5 mg kg−1 EDTA significantly increased the yield character except grain yield rather than control (Table 3). Both the buildup of Cd in grains and the movement of Cd from the lower tissue to the upper tissue in the stem were greatly reduced by the addition of EDTA [64]. Agronomic character improved by EDTA application under Cd stress (Tables 2 and 3) which is supported by [63].
Various plants’ Cd toxicity was lowered by exogenous foliar spray [50,65,66]. Mineral nutrients are absorbed by plants, who then provide them to tissues and organs [67]. The foliar application of NSE increased gas exchange metrics and growth-related features while reducing the harmful effects of Cd-toxicity. Previous research has shown that applying foliar applications of several compounds under metal stress increased growth and gas exchange parameters in maize [68–70]. In a recent study, NSE enhanced maize growth and associated characteristics when exposed to Cr and Cd stress [29,69]. In Cd toxicity, NSE foliar application dramatically improved wheat growth and yield characteristics. Due to its bioactive components, N. sativa seeds have excellent antioxidant properties that can scavenge free radicals. Thymoquinone [71–73]. Exogenous Foliar application of 20% NSE mitigated Cd toxicity which is consistent with the findings of [69] and [29] might be due to TQ. Conversely, the efficient mechanism of NSE concerned with improving Cd tolerance needs to be additionally considered.
In our research, aqueous extracts of O. sanctum were applied to Cd-containing plants. In comparison to the Cd level, all growth parameters in Cd-treated plants showed a significant restoration in seedling growth, a large reduction in Cd uptake, and comparatively less ROS formation which is consistent with the finding of [74]. The leaves of O. sanctum contain a variety of naturally occurring compounds, including euginal, eugenol, carvacrol, rosameric acid, apigenin, saponins, triterpenoids, tannins, flavonoids, propanoic acid, and some water-soluble flavonoids like orientin and vicenin that have pharmacological effects like antioxidants [75,76]. O. sanctum aqueous extract has strong chelating and free radical scavenging capabilities as a result of the presence of these chemicals [77]. When compared to O. sanctum extract, Cd absorption was more significantly decreased with N. sativa seed extract, indicating that N. sativa reduces Cd bioavailability more effectively than O. sanctum does in lowering Cd toxicity in wheat plants. This might be one of the causes of the decreased absorption of Cd by the roots and subsequently decreased bioavailability of Cd in the tissues as a result of the use of EDTA and plant extracts.
It is suggested that exogenous application of seed extract N. sativa and leaf extract of O. sanctum enhanced the growth, biomass, and yield traits of wheat crops under Cd stress in contrast to non-spray and non-soil applied EDTA plants under Cd toxicity. In a nutshell, an exogenous application of easily available and inexpensive natural bio-stimulants especially Nigella sativa and Ocimum sanctum could be a useful strategy to enhance Cd tolerance in wheat plants by improving growth, biomass and reducing Cd uptake in various tissues on the wheat plants. Further research should explore optimal application rates and timing for these interventions. Long-term field studies are warranted to assess their efficacy under diverse environmental conditions. Additionally, investigating the molecular mechanisms underlying the observed improvements could provide valuable insights for future applications in sustainable agriculture.
Acknowledgement: The authors would like to extend their sincere appreciation to the Researchers Supporting Project Number (RSP2024R356), King Saud University, Riyadh, Saudi Arabia.
Funding Statement: The authors would like to extend their sincere appreciation to the Researchers Supporting Project Number (RSP2024R356), King Saud University, Riyadh, Saudi Arabia.
Author Contributions: Abida Aziz, Shafiqa Bano, Mubshar Hussain, Qamar uz Zaman, Ghulam Yasin: conceptualization, methodology, formal analysis; Mubshar Hussain, Muhammad Farooq Azhar, Naila Hadayat, Iqra Arooj: conceptualization, methodology; Abeer Hashem, Ajay Kumar, Elsayed Fathi Abd_Allah; writing, review, and editing; Shafiqa Bano, Ghulam Yasin, Mubshar Hussain: validation, data curation; Iqra Arooj, Abida Aziz, Shafiqa Bano, Ghulam Yasin: writing original draft; Muhammad Farooq Azhar, Iqra Arooj, Mubshar Hussain, Ghulam Yasin: formal analysis, investigation; Iqra Arooj, Abida Aziz, Qamar uz Zaman, Naila Hadayat: investigation, validation, data curation. All authors reviewed the results and approved the final version of the manuscript.
Availability of Data and Materials: The data used during the current study are available from the corresponding author upon reasonable request.
Conflicts of Interest: The authors declare that they have no conflicts of interest to report regarding the present study.
References
1. Rizwan MS, Imtiaz M, Zhu J, Yousaf B, Hussain M, Ali L, et al. Immobilization of Pb and Cu by organic and inorganic amendments in contaminated soil. Geoderma. 2021;385:114803. doi:10.1016/j.geoderma.2020.114803. [Google Scholar] [CrossRef]
2. Chen D, Chen D, Xue R, Long J, Lin X, Lin Y, et al. Effects of boron, silicon and their interactions on cadmium accumulation and toxicity in rice plants. J Hazard Mat. 2019;367:447–55. doi:10.1016/j.jhazmat.2018.12.111. [Google Scholar] [PubMed] [CrossRef]
3. Haider FU, Cai L, Coulter JA, Cheema SA, Wu J, Zhang R, et al. Cadmium toxicity in plants: impacts and remediation strategies. Ecotoxicol Environ Saf. 2021;211:111887. doi:10.1016/j.ecoenv.2020.111887. [Google Scholar] [PubMed] [CrossRef]
4. Du B, Zhou J, Lu B, Zhang C, Li D, Zhou J, et al. Environmental and human health risks from cadmium exposure near an active lead-zinc mine and a copper smelter, China. Sci Total Environ. 2020;720:137585. doi:10.1016/j.scitotenv.2020.137585. [Google Scholar] [PubMed] [CrossRef]
5. Ahmad I, Akhtar MJ, Zahir ZA, Jamil A. Effect of cadmium on seed germination and seedling growth of four wheat (Triticum aestivum L.) cultivars. Pak J Bot. 2012;44(5):1569–74. [Google Scholar]
6. Fittipaldi S, Bimonte V, Soricelli A, Aversa A, Lenzi A, Greco E, et al. Cadmium exposure alters steroid receptors and proinflammatory cytokine levels in endothelial cells in vitro: a potential mechanism of endocrine disruptor atherogenic effect. J Endocrinol Investig. 2019;42:727–39. doi:10.1007/s40618-018-0982-1. [Google Scholar] [PubMed] [CrossRef]
7. Gratão PL, Monteiro CC, Tezotto T, Carvalho RF, Alves LR, Peters LP, et al. Cadmium stress antioxidant responses and root-to-shoot communication in grafted tomato plants. Biometals. 2015;28(5):803–16. doi:10.1007/s10534-015-9867-3. [Google Scholar] [PubMed] [CrossRef]
8. Hamid Y, Tang L, Sohail MI, Cao X, Hussain B, Aziz MZ, et al. An explanation of soil amendments to reduce cadmium phytoavailability and transfer to food chain. Sci Total Environ. 2019;660:80–96. doi:10.1016/j.scitotenv.2018.12.419. [Google Scholar] [PubMed] [CrossRef]
9. Khanna K, Kohli SK, Ohri P, Bhardwaj R, Ahmad P. Agroecotoxicological aspect of Cd in soil-plant system: uptake, translocation and amelioration strategies. Environ Sci Pollut Res. 2022;29(21):30908–34. doi:10.1007/s11356-021-18232-5. [Google Scholar] [PubMed] [CrossRef]
10. Kumar S, Prasad S, Yadav KK, Shrivastava M, Gupta N, Nagar S, et al. Hazardous heavy metals contamination of vegetables and food chain: role of sustainable remediation approaches—a review. Environ Res. 2019;179:108792. doi:10.1016/j.envres.2019.108792. [Google Scholar] [PubMed] [CrossRef]
11. Saxena G, Purchase D, Mulla SI, Saratale GD, Bharagava RN. Phytoremediation of heavy metal-contaminated sites: eco-environmental concerns, field studies, sustainability issues, and future prospects. Rev Environ Contam Toxicol. 2020;249:71–131. [Google Scholar] [PubMed]
12. Singh A, Singh S, Kurella A, Verma A, Mahatama M, Venkatesh I. Plant bio-stimulants, their functions and use in enhancing stress tolerance in oilseeds. In: New and future developments in microbial biotechnology and bioengineering. Amsterdam, Netherlands: Elsevier; 2022. p. 239–59. [Google Scholar]
13. Shubha K, Mukherjee A, Kumari M, Tiwari K, Meena VS. Bio-stimulants: an approach towards the sustainable vegetable production. In: Agriculturally important microbes for sustainable agriculture: Volume I: Plant-soil-microbe nexus. Singapore: Springer; 2017. p. 259–77. [Google Scholar]
14. Bulgari R, Cocetta G, Trivellini A, Vernieri P, Ferrante A. Biostimulants and crop responses: a review. Biol Agric Hortic. 2015;31(1):1–17. doi:10.1080/01448765.2014.964649. [Google Scholar] [CrossRef]
15. Požgajová M, Navrátilová A, Kovár M. Curative potential of substances with bioactive properties to alleviate Cd toxicity: a review. Int J Environ Res Public Health. 2022;19(19):12380. doi:10.3390/ijerph191912380. [Google Scholar] [PubMed] [CrossRef]
16. Khan R, Ali S, Mumtaz S, Kanwal L, Mumtaz S, Nauroze T. Ameliorating and pharmacological intervention potential of grape seed extract against lead-and cadmium-induced toxicity. Int J Environ Sci Technol. 2021;19:1–16. [Google Scholar]
17. Kerdsomboon K, Chumsawat W, Auesukaree C. Effects of Moringa oleifera leaf extracts and its bioactive compound gallic acid on reducing toxicities of heavy metals and metalloid in Saccharomyces cerevisiae. Chemosphere. 2021;270:128659. doi:10.1016/j.chemosphere.2020.128659. [Google Scholar] [PubMed] [CrossRef]
18. Shahid M, Austruy A, Echevarria G, Arshad M, Sanaullah M, Aslam M, et al. EDTA-enhanced phytoremediation of heavy metals: a review. Soil Sediment Contam: an Int J. 2014;23(4):389–416. doi:10.1080/15320383.2014.831029. [Google Scholar] [CrossRef]
19. Habiba U, Ali S, Farid M, Shakoor MB, Rizwan M, Ibrahim M, et al. EDTA enhanced plant growth, antioxidant defense system, and phytoextraction of copper by Brassica napus L. Environ Sci Pollut Res. 2015;22:1534–44. doi:10.1007/s11356-014-3431-5. [Google Scholar] [PubMed] [CrossRef]
20. Seuntjens P, Nowack B, Schulin R. Root-zone modeling of heavy metal uptake and leaching in the presence of organic ligands. Plant Soil. 2004;265:61–73. doi:10.1007/s11104-005-8470-8. [Google Scholar] [CrossRef]
21. Gul I, Manzoor M, Silvestre J, Rizwan M, Hina K, Kallerhoff J, et al. EDTA-assisted phytoextraction of lead and cadmium by Pelargonium cultivars grown on spiked soil. Int J Phytoremediation. 2019;21(2):101–10. doi:10.1080/15226514.2018.1474441. [Google Scholar] [PubMed] [CrossRef]
22. Coolbear P, Francis A, Grierson D. The effect of low temperature pre-sowing treatment on the germination performance and membrane integrity of artificially aged tomato seeds. J Exp Bot. 1984;35(11):1609–17. doi:10.1093/jxb/35.11.1609. [Google Scholar] [CrossRef]
23. Ellis R, Roberts E. The quantification of ageing and survival in orthodox seeds. Seed Sci Technol. 1981;9(2):373–409. [Google Scholar]
24. Farooq S, Onen H, Ozaslan C, Baskin C, Gunal H. Seed germination niche for common ragweed (Ambrosia artemisiifolia L.) populations naturalized in Turkey. South Afr J Bot. 2019;123:361–71. doi:10.1016/j.sajb.2019.03.031. [Google Scholar] [CrossRef]
25. d Steel RG, Torrie JH. Principles and procedures of statistics: a biometrical approach. New York, NY, USA: McGraw-Hill Company; 1986. p. 95–106. [Google Scholar]
26. Gupta N, Yadav KK, Kumar V, Kumar S, Chadd RP, Kumar A. Trace elements in soil-vegetables interface: translocation, bioaccumulation, toxicity and amelioration—a review. Sci Total Environ. 2019;651:2927–42. doi:10.1016/j.scitotenv.2018.10.047. [Google Scholar] [PubMed] [CrossRef]
27. Saeed Z, Naveed M, Imran M, Bashir MA, Sattar A, Mustafa A, et al. Combined use of Enterobacter sp. MN17 and zeolite reverts the adverse effects of cadmium on growth, physiology and antioxidant activity of Brassica napus. PLoS One. 2019;14(3):e0213016. doi:10.1371/journal.pone.0213016. [Google Scholar] [PubMed] [CrossRef]
28. Du YL, He MM, Xu M, Yan ZG, Zhou YY, Guo GL, et al. Interactive effects between earthworms and maize plants on the accumulation and toxicity of soil cadmium. Soil Biol Biochem. 2014;72:193–202. doi:10.1016/j.soilbio.2014.02.004. [Google Scholar] [CrossRef]
29. Hussain I, Iqbal M, Qurat-Ul-Ain S, Rasheed R, Mahmood S, Perveen A, et al. Cadmium dose and exposure-time dependent alterations in growth and physiology of maize (Zea mays). Int J Agric Biol. 2012;14(6):959–64. [Google Scholar]
30. Stefani A, Arduini I, Onnis A. Juncus acutus: germination and initial growth in presence of heavy metals. Annales Botanici Fennici. 1991;28:37–43. [Google Scholar]
31. Mahmood S, Hussain A, Saeed Z, Athar M. Germination and seedling growth of corn (Zea mays L.) under varying levels of copper and zinc. Int J Environ Sci Technol. 2005;2:269–74. doi:10.1007/BF03325886. [Google Scholar] [CrossRef]
32. Hema D, Altaf A, Muhammad I. Characterization of chromium toxicity in food crops and their role in phytoremediation. J Bioremed Biodegradation. 2012;3(8):54–61. [Google Scholar]
33. Rahman SU, Qi XB, Kamran M, Yasin G, Cheng H, Rehim A, et al. Silicon elevated cadmium tolerance in wheat (Triticum aestivum L.) by endorsing nutrients uptake and antioxidative defense mechanisms in the leaves. Plant Physiol Biochem. 2021;166:148–59. doi:10.1016/j.plaphy.2021.05.038. [Google Scholar] [PubMed] [CrossRef]
34. Shahid M, Pinelli E, Dumat C. Review of Pb availability and toxicity to plants in relation with metal speciation; role of synthetic and natural organic ligands. J Hazard Mat. 2012;219:1–12. [Google Scholar]
35. Wang M, Chen L, Chen S, Ma Y. Alleviation of cadmium-induced root growth inhibition in crop seedlings by nanoparticles. Ecotoxicol Environ Saf. 2012;79:48–54. doi:10.1016/j.ecoenv.2011.11.044. [Google Scholar] [PubMed] [CrossRef]
36. Yasin G, Ur Rahman S, Yousaf MTB, Azhar MF, Zahid DM, Imtiaz M, et al. Phytoremediation potential of E. camaldulensis and M. alba for copper, cadmium, and lead absorption in urban areas of Faisalabad City, Pakistan. Int J Environ Res. 2021;15:597–612. doi:10.1007/s41742-021-00330-4. [Google Scholar] [CrossRef]
37. Hasanuzzaman M, Nahar K, Gill SS, Alharby HF, Razafindrabe BH, Fujita M. Hydrogen peroxide pretreatment mitigates cadmium-induced oxidative stress in Brassica napus L.: an intrinsic study on antioxidant defense and glyoxalase systems. Front Plant Sci. 2017;8:115. [Google Scholar] [PubMed]
38. Ismael MA, Elyamine AM, Moussa MG, Cai M, Zhao X, Hu C. Cadmium in plants: uptake, toxicity, and its interactions with selenium fertilizers. Metallomics. 2019;11(2):255–77. doi:10.1039/C8MT00247A. [Google Scholar] [PubMed] [CrossRef]
39. Mourato M, Pinto F, Moreira I, Sales J, Leitão I, Martins LL. The effect of Cd stress in mineral nutrient uptake in plants. In: Cadmium toxicity and tolerance in plants. Amsterdam, Netherlands: Elsevier; 2019. p. 327–48. [Google Scholar]
40. Hasanuzzaman M, Nahar K, Fujita M. Plants under metal and metalloid stress: responses, tolerance and remediation. Singapore: Springer; 2018. p. 213–29. [Google Scholar]
41. Chauhan SK, Sharma S, Beri V, Ritu B, Yadav S, Gupta N. Yield and carbon sequestration potential of wheat (Triticum aestivum)-poplar (Populus deltoides) based agri-silvicultural system. Indian J Agric Sci. 2010;80(2):89–97. [Google Scholar]
42. Arshad M, Ali S, Noman A, Ali Q, Rizwan M, Farid M, et al. Phosphorus amendment decreased cadmium (Cd) uptake and ameliorates chlorophyll contents, gas exchange attributes, antioxidants, and mineral nutrients in wheat (Triticum aestivum L.) under Cd stress. Arch Agron Soil Sci. 2016;62(4):533–46. doi:10.1080/03650340.2015.1064903. [Google Scholar] [CrossRef]
43. Kamran M, Malik Z, Parveen A, Zong Y, Abbasi GH, Rafiq MT, et al. Biochar alleviates Cd phytotoxicity by minimizing bioavailability and oxidative stress in pak choi (Brassica chinensis L.) cultivated in Cd-polluted soil. J Environ Manage. 2019;250:109500. doi:10.1016/j.jenvman.2019.109500. [Google Scholar] [PubMed] [CrossRef]
44. Rizwan M, Ali S, Qayyum MF, Ok YS, Zia-Ur-Rehman M, Abbas Z, et al. Use of maize (Zea mays L.) for phytomanagement of Cd-contaminated soils: a critical review. Environ Geochem Health. 2017;39:259–77. doi:10.1007/s10653-016-9826-0. [Google Scholar] [PubMed] [CrossRef]
45. Ahmad A, Hadi F, Ali N, Jan AU. Enhanced phytoremediation of cadmium polluted water through two aquatic plants Veronica anagallis-aquatica and Epilobium laxum. Environ Sci Pollut Res. 2016;23:17715–29. doi:10.1007/s11356-016-6960-2. [Google Scholar] [PubMed] [CrossRef]
46. Rahman SU, Nawaz MF, Gul S, Yasin G, Hussain B, Li Y, et al. State-of-the-art OMICS strategies against toxic effects of heavy metals in plants: a review. Ecotoxicol Environ Saf. 2022;242:113952. doi:10.1016/j.ecoenv.2022.113952. [Google Scholar] [PubMed] [CrossRef]
47. Rahman SU, Yasin G, Nawaz MF, Cheng H, Azhar MF, Riaz L, et al. Evaluation of heavy metal phytoremediation potential of six tree species of Faisalabad city of Pakistan during summer and winter seasons. J Environ Manage. 2022;320:115801. doi:10.1016/j.jenvman.2022.115801. [Google Scholar] [PubMed] [CrossRef]
48. Khan N, Samiullah, Singh S, Nazar R. Activities of antioxidative enzymes, sulphur assimilation, photosynthetic activity and growth of wheat (Triticum aestivum) cultivars differing in yield potential under cadmium stress. J Agron Crop Sci. 2007;193(6):435–44. doi:10.1111/jac.2007.193.issue-6. [Google Scholar] [CrossRef]
49. Choppala G, Saifullah, Bolan N, Bibi S, Iqbal M, Rengel Z, et al. Cellular mechanisms in higher plants governing tolerance to cadmium toxicity. Crit Rev Plant Sci. 2014;33(5):374–91. doi:10.1080/07352689.2014.903747. [Google Scholar] [CrossRef]
50. Duan MM, Wang S, Huang DY, Zhu QH, Liu SL, Zhang Q, et al. Effectiveness of simultaneous applications of lime and zinc/iron foliar sprays to minimize cadmium accumulation in rice. Ecotoxicol Environ Saf. 2018;165:510–5. doi:10.1016/j.ecoenv.2018.09.037. [Google Scholar] [PubMed] [CrossRef]
51. Saleh SR, Kandeel MM, Ghareeb D, Ghoneim TM, Talha NI, Alaoui-Sossé B, et al. Wheat biological responses to stress caused by cadmium, nickel and lead. Sci Total Environ. 2020;706:136013. doi:10.1016/j.scitotenv.2019.136013. [Google Scholar] [PubMed] [CrossRef]
52. Rahman SU, Han JC, Ahmad M, Ashraf MN, Khaliq MA, Yousaf M, et al. Aluminum phytotoxicity in acidic environments: a comprehensive review of plant tolerance and adaptation strategies. Ecotoxicol Environ Saf. 2024;269:115791. doi:10.1016/j.ecoenv.2023.115791. [Google Scholar] [PubMed] [CrossRef]
53. Ulameer OQA, Ahmed SAA-H. Anti-transpirant role in improving the morphological growth traits of maize plants subjected to water stress. Res Crops. 2018;19(4):593–603. [Google Scholar]
54. Hirzel J, Retamal-Salgado J, Walter I, Matus I. Cadmium accumulation and distribution in plants of three durum wheat cultivars under different agricultural environments in Chile. J Soil Water Conserv. 2017;72(1):77–88. doi:10.2489/jswc.72.1.77. [Google Scholar] [CrossRef]
55. Rahman SU, Han JC, Zhou Y, Ahmad M, Li B, Wang Y, et al. Adaptation and remediation strategies of mangroves against heavy metal contamination in global coastal ecosystems: a review. J Clean Prod. 2024;441:140868. doi:10.1016/j.jclepro.2024.140868. [Google Scholar] [CrossRef]
56. Guo J, Feng R, Ding Y, Wang R. Applying carbon dioxide, plant growth-promoting rhizobacterium and EDTA can enhance the phytoremediation efficiency of ryegrass in a soil polluted with zinc, arsenic, cadmium and lead. J Environ Manage. 2014;141:1–8. doi:10.1016/j.jenvman.2013.12.039. [Google Scholar] [PubMed] [CrossRef]
57. Al Mahmud J, Hasanuzzaman M, Nahar K, Rahman A, Fujita M. EDTA reduces cadmium toxicity in mustard (Brassica juncea L.) by enhancing metal chelation, antioxidant defense and glyoxalase systems. Acta Agrobot. 2019;72(2):1–17. [Google Scholar]
58. Najeeb U, Jilani G, Ali S, Sarwar M, Xu L, Zhou W. Insights into cadmium induced physiological and ultra-structural disorders in Juncus effusus L. and its remediation through exogenous citric acid. J Hazard Mat. 2011;186(1):565–74. doi:10.1016/j.jhazmat.2010.11.037. [Google Scholar] [PubMed] [CrossRef]
59. Wei JL, Lai HY, Chen ZS. Chelator effects on bioconcentration and translocation of cadmium by hyperaccumulators, Tagetes patula and Impatiens walleriana. Ecotoxicol Environ Saf. 2012;84:173–8. doi:10.1016/j.ecoenv.2012.07.004. [Google Scholar] [PubMed] [CrossRef]
60. Custos JM, Moyne C, Treillon T, Sterckeman T. Contribution of Cd-EDTA complexes to cadmium uptake by maize: a modelling approach. Plant Soil. 2014;374:497–512. doi:10.1007/s11104-013-1906-7. [Google Scholar] [CrossRef]
61. Ochoa M, Tierra W, Tupuna-Yerovi DS, Guanoluisa D, Otero XL, Ruales J. Assessment of cadmium and lead contamination in rice farming soils and rice (Oryza sativa L.) from Guayas province in Ecuador. Environ Pollut. 2020;260:114050. doi:10.1016/j.envpol.2020.114050. [Google Scholar] [PubMed] [CrossRef]
62. Liu J, Shen Y, Luo L. Evaluating the effect of EDTA on the internal mechanisms of uptake and translocation of Pb in Bidens pilosa L. Plant Soil. 2022;479(1–2):649–62. [Google Scholar]
63. Önen H, Farooq S, Tad S, Özaslan C, Gunal H, Chauhan BS. The influence of environmental factors on germination of Burcucumber (Sicyos angulatus) seeds: implications for range expansion and management. Weed Sci. 2018;66(4):494–501. doi:10.1017/wsc.2018.20. [Google Scholar] [CrossRef]
64. Yan L, Li C, Zhang J, Moodley O, Liu S, Lan C, et al. Enhanced phytoextraction of lead from artificially contaminated soil by Mirabilis jalapa with chelating agents. Bull Environ Contam Toxicol. 2017;99:208–12. doi:10.1007/s00128-017-2127-1. [Google Scholar] [PubMed] [CrossRef]
65. Chen R, Zhang C, Zhao Y, Huang Y, Liu Z. Foliar application with nano-silicon reduced cadmium accumulation in grains by inhibiting cadmium translocation in rice plants. Environ Sci Pollut Res. 2018;25:2361–8. doi:10.1007/s11356-017-0681-z. [Google Scholar] [PubMed] [CrossRef]
66. Gao M, Zhou J, Liu H, Zhang W, Hu Y, Liang J, et al. Foliar spraying with silicon and selenium reduces cadmium uptake and mitigates cadmium toxicity in rice. Sci Total Environ. 2018;631:1100–8. [Google Scholar] [PubMed]
67. Li N, Feng A, Liu N, Jiang Z, Wei S. Silicon application improved the yield and nutritional quality while reduced cadmium concentration in rice. Environ Sci Pollut Res. 2020;27:20370–9. doi:10.1007/s11356-020-08357-4. [Google Scholar] [PubMed] [CrossRef]
68. Danish S, Tahir FA, Rasheed MK, Ahmad N, Ali MA, Kiran S, et al. Effect of foliar application of Fe and banana peel waste biochar on growth, chlorophyll content and accessory pigments synthesis in spinach under chromium (IV) toxicity. Open Agric. 2019;4(1):381–90. doi:10.1515/opag-2019-0034. [Google Scholar] [CrossRef]
69. Allah Ditta HM, Aziz A, Hussain MK, Mehboob N, Hussain M, Farooq S, et al. Exogenous application of black cumin (Nigella sativa) seed extract improves maize growth under chromium (Cr) stress. Int J Phytoremediation. 2021;23(12):1231–43. doi:10.1080/15226514.2021.1889965. [Google Scholar] [PubMed] [CrossRef]
70. Habiba U, Ali S, Rizwan M, Ibrahim M, Hussain A, Shahid MR, et al. Alleviative role of exogenously applied mannitol in maize cultivars differing in chromium stress tolerance. Environ Sci Pollut Res. 2019;26:5111–21. doi:10.1007/s11356-018-3970-2. [Google Scholar] [PubMed] [CrossRef]
71. Aljabre SHM, Randhawa MA, Akhtar N, Alakloby OM, Alqurashi AM, Aldossary A. Antidermatophyte activity of ether extract of Nigella sativa and its active principle, thymoquinone. J Ethnopharmacol. 2005;101(1–3):116–9. [Google Scholar] [PubMed]
72. Solati Z, Baharin BS, Bagheri H. Antioxidant property, thymoquinone content and chemical characteristics of different extracts from Nigella sativa L. seeds. J Am Oil Chem Soc. 2014;91:295–300. doi:10.1007/s11746-013-2362-5. [Google Scholar] [CrossRef]
73. Benkaci-Ali F, Baaliouamer A, Wathelet JP, Marlier M. Chemical composition of volatile oils from Algerian Nigella sativa L. seeds. J Essent Oil Res. 2010;22(4):318–22. doi:10.1080/10412905.2010.9700335. [Google Scholar] [CrossRef]
74. Gopal R, Rizvi AH. Excess lead alters growth, metabolism and translocation of certain nutrients in radish. Chemosphere. 2008;70(9):1539–44. doi:10.1016/j.chemosphere.2007.08.043. [Google Scholar] [PubMed] [CrossRef]
75. Pattanayak P, Behera P, Das D, Panda SK. Ocimum sanctum Linn. A reservoir plant for therapeutic applications: an overview. Pharmacogn Rev. 2010;4(7):95–105. doi:10.4103/0973-7847.65323. [Google Scholar] [PubMed] [CrossRef]
76. Kumar A, Agarwal K, Maurya AK, Shanker K, Bushra U, Tandon S, et al. Pharmacological and phytochemical evaluation of Ocimum sanctum root extracts for its antiinflammatory, analgesic and antipyretic activities. Pharmacogn Mag. 2015;11(Suppl 1):S217. [Google Scholar] [PubMed]
77. Hakkim FL, Shankar CG, Girija S. Chemical composition and antioxidant property of holy basil (Ocimum sanctum L.) leaves, stems, and inflorescence and their in vitro callus cultures. J Agric Food Chem. 2007;55(22):9109–17. doi:10.1021/jf071509h. [Google Scholar] [PubMed] [CrossRef]
Cite This Article
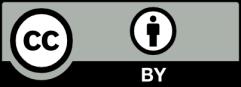
This work is licensed under a Creative Commons Attribution 4.0 International License , which permits unrestricted use, distribution, and reproduction in any medium, provided the original work is properly cited.