Open Access
ARTICLE
In Vitro Propagation and Artificial Seed Production of Fritillaria cirrhosa D. Don, an Endangered Medicinal Plant
Chengdu University of Traditional Chinese Medicine, State Key Laboratory of Characteristic Chinese Medicine Resources in Southwest China, Chengdu, 610000, China
* Corresponding Author: Zhuyun Yan. Email:
Phyton-International Journal of Experimental Botany 2024, 93(6), 1297-1310. https://doi.org/10.32604/phyton.2024.051923
Received 18 March 2024; Accepted 20 May 2024; Issue published 27 June 2024
Abstract
Fritillaria cirrhosa D. Don (Liliaceae) is an endangered perennial bulbous plant and its dry bulb is a valuable medicinal material with antitussive and expectorant effects. Nevertheless, lack of resources and expensive prices make it difficult to meet clinical needs. This study presents a regeneration system aimed at overcoming the challenge of inadequate supply in F. cirrhosa, focusing on: (1) callus induction, (2) bulblets and adventitious bud induction, and (3) artificial seed production. Callus development was achieved in 84.93% on Murashige and Skoog (MS) medium fortified with 1.0 mg·L picloram. The optimal medium for callus differentiation into regenerated bulblets was MS medium supplemented with 1.0 mg·L 6-benzyladenine (6-BA) and 0.2 mg·L α-naphthaleneacetic acid (NAA). Subsequently, bulblets and adventitious buds were induced from regenerated bulblet sections cultured on MS medium fortified with 0.3 mg·L 6-BA + 1.0 mg·L 2,4-dichlorophenoxyacetic acid (2,4-D), 2.0 mg·L 6-BA + 0.5 mg·L, and the induction rates were 88.17% and 84.24%, respectively. The regenerated bulblets were transplanted into a substrate of humus soil, river sand, and pearlite (1:1:1) after low-temperature treatment. The germination rate was 42.80% after culture for 30 days. Regenerated bulblets were used for encapsulations in liquid MS medium containing 3% sucrose (w/v) + 0.5 mg·L NAA + 2.0 mg·L 6-BA + 3% sodium alginate (w/v) with a 10 min exposure to 2% CaCl. Under non-aseptic conditions, the germination rate reached 81.67%, while the rooting rate was 20.56% after 45 days. The capsule added 1.0 g·L carbendazim and 1.0 g·L activated carbon was the best component of artificial seeds. This study successfully established an efficient regeneration system for the rapid propagation of F. cirrhosa, involving in vitro bulblet regeneration and artificial seed production. This method introduces a novel approach for efficient breeding and germplasm preservation, making it suitable for large-scale industrial resource production.Keywords
Supplementary Material
Supplementary Material FileThe genus Fritillaria L., in the family Liliaceae, comprises approximately 150 species, which are distributed in temperate zones of the northern hemisphere [1]. There are 24 species in China (http://www.iplant.cn/foc), and Fritillaria plants are known to possess medicinal attributes such as steroidal alkaloids exhibit antitussive and expectorant effects. Among them, Fritillaria cirrhosa D. Don is precious Chinese herbal medicine generally used for treating lung heat and dry cough, dry cough with less sputum, tuberculosis caused by yin deficiency, sputum with blood, scrofulous dysentery, mammary carbuncle, and lung carbuncle [2,3]. F. cirrhosa is an authentic medicinal herb produced in China, as the “holy medicine of cough”, with a long history of medicinal use and significant clinical efficacy [4]. Due to its unique growth environment, long seed dormancy period, low natural germination rate, complex life cycle of growth, and excessive exploitation of wild resources [5,6]. As a result, F. cirrhosa resources are scarce and expensive, making it difficult to meet clinical needs. Therefore, it is urgent to formulate an effective propagation strategy to address the problem of resource shortage and conservation of wild resources in the cultivation and production of F. cirrhosa.
Plant tissue culture has been recognized as an efficient method for obtaining in vitro grown seedlings or regenerated seedlings, facilitating rapid plant propagation, and conserving endangered plant germplasm resources [7–9]. This technique provides a propagation method for Fritillaria species with a long growth cycle. Compact embryogenic callus of F. meleagris was successfully induced by Petrić, et al. on a basal medium supplemented with thidiazuron (TDZ) [10]. Cakmak et al. utilized various combinations and concentrations of 6-benzyl aminopurine (6-BA), inaphthalene acetic acid (NAA), kinetin, and thidiazuron to induce bulblet formation in F. imperialis and F. persica. The regenerated bulblets are successfully rooted and domesticated in greenhouse [11]. F. cirrhosa seed germination was recorded on MSBM supplemented with 1.0 mg·L−1 BA and 0.4 mg·L−1 NAA, and the induction of callus and bulblet formation was observed at seedlings basal ends [12]. Callus was induced of F. thunbergii by Hao et al. using MS medium fortified with 2.0 mg·L−1 NAA and 0.5 mg·L−1 BAP [13]. Similarly, high efficiency callus of F. roylei was induced by adding and 2.0 mg·L−1 picloram and 0.5 mg·L−1 TDZ on MS medium, and accomplished in vitro plantlets regeneration [5]. Zhao et al. achieved in vitro regeneration of F. cirrhosa by using bulb scales on MS medium containing 2.0 mg·L−1 BAP and 0.5 mg·L−1 NAA [14]. Those aforementioned studies indicate that plant tissue culture technique is anticipated to be applied to the callus and bulblets induction of F. cirrhosa, so as to shorten the reproductive cycle of F. cirrhosa.
Compared to regenerated bulblets, artificial seed has the strength of being inexpensive, convenient for transport and storage, and suitable for direct or mechanized sowing [15]. Various plant materials, such as somatic embryos [16], shoot tips [17], protocorm-like bodies [18], etc., can be used as artificial seed propagules. Nutrients, plant growth regulators (PGRs), adsorbents, and other substances can be added to the artificial endosperm to maintain the normal growth and development of the propagule [19]. Andrographis paniculate [20], Dendrobium nobile [21] and other artificial seeds of medicinal plants have been reported. Therefore, using plant tissue culture technology to produce artificial seed is an effective way to realize large-scale cultivation and production of medicinal resources, as well as for preserving wild resources.
However, no research has been conducted on F. cirrhosa artificial seed production. Therefore, in this study, the bulbs of F. cirrhosa were used as explants to establish the regeneration of bulblets and artificial seeds production technology by callus induction, differentiation, and bulblet induction. This aims to realize the rapid propagation of regenerated bulblets and artificial seeds of F. cirrhosa and meet the requirements of germplasm preservation and reproduction.
2.1 Plant Materials, Sterilization, and Culture Conditions
Bulbs were collected from Bamei Town, Daofu County, Ganzi Tibetan Autonomous Prefecture, Sichuan Province, China (100°27′14.03′′ E, 29°35′6.0864′′ N, 3566 m). A voucher specimen was deposited at the State Key Laboratory of Characteristic Chinese Medicine Resources in Southwest China at Chengdu University of Traditional Chinese Medicine. Remove surface soil and fibrous roots from the bulbs, then separated the scales and washed under running water for 30 min. Then, the scales were immersed in 75% (v/v) ethanol for 30 s, and rinsed 3–4 times with sterile water. Subsequently immersed in a 0.1% (w/v) HgCl2 solution with 2–3 drops of Tween 80 for 8–15 min. Finally, the scales were rinsed five times with sterile water.
All mediums were Murashige and Skoog (MS; Phyto Tech Labs) [22] medium fortified with 3% (w/v) sucrose and 0.8% (w/v) agar, and MS medium were place in glass culture flasks, each culture flask added 50 mL medium. The pH of the media was set to 5.8 prior to being autoclaved at 121°C for 20 min. The culture temperature was set at 20°C, and light intensity was set at 312 μmol·m−2s−1, and light-dark cycle was 12 h in the intelligent illumination incubator.
The aseptic scales were cut into 5 mm × 5 mm segments, inoculated into MS medium, and supplemented with various concentrations of TDZ (Solarbio), picloram (Shyuany bio), 2,4-dichlorophenoxyacetic acid (2,4-D; Phyto Tech Labs), and NAA (Phyto Tech Labs) either individually or in combination to induce callus. Each treatment had three replicates, 30 explants per replicate. After 45 days of culture, the callus induction rate was measured and the callus growth status was observed. Callus induction rate = (number of explants with callus formation/number of explants) × 100%.
Callus pieces were inoculated on MS differentiation medium supplemented with various concentrations of 6-BA (Phyto Tech Labs), NAA, and 2,4-D in combinations for induction of bulblets. Each treatment was replicated three times, with 25 calli in each replicate. Callus differentiation status was observed during the culture process, and the callus differentiation rate and regeneration coefficient were counted after 45 days of culture. Callus differentiation rate = (number of callus differentiations/number of callus) × 100%, and callus regeneration coefficient = (number of callus regenerating bulblets/number of the callus).
2.4 Bulblet and Adventitious Bud Induction
Bulbs formed on MS medium fortified with 0.3 mg·L−1 and 1.0 mg·L−1 6-BA were cut into 5 mm × 5 mm sections and inoculated on MS medium supplemented with 6-BA, NAA, 2,4-D, and TDZ in combinations to induce bulblet and adventitious bud. Each treatment consisted of 30 explants, and three replicates were performed for each treatment. After 45 days of culture in the induction medium, the induction rate and regeneration coefficient of bulblets and adventitious buds were counted. Bulblets or adventitious bud induction rate = (number of explants with bulblets or adventitious buds/number of inoculated explants) × 100%, and regeneration coefficient = (number of bulblets or adventitious buds induction/number of inoculated explants) × 100%.
2.5 Regenerated Bulblets Germination
The regenerated bulblets were inoculated on MS medium without PGRs and cultured in a refrigerator at 4°C for 45 days to break dormancy. The control group regenerated bulblets without the 4°C treatment. The regenerated bulblets were transferred to a soil matrix composed of humus soil, river sand, and perlite in a 1:1:1 ratio. Those bulblets were cultivated in a light incubator and light intensity was set at 312 μmol·m−2s−1 with 12:12 h light: dark cycle, and the temperature was 16°C. The germination rate was counted after 30 days of culture. The percentage of water content was maintained at 30% by adding sterile water at regular intervals. Each treatment was repeated three times with 25 regenerated bulblets in each replicate. Bulblet germination was determined based on the standard that the bulblet bud grew to 1 cm. Germination rate = (number of germination bulblets/number of transplanted bulblets) × 100%.
2.6 Artificial Seeds Production
Aseptic regenerated bulblets (with a diameter of 0.5–1.0 cm) were selected as explants. MS liquid medium was used to prepare 3.0% sodium alginate solution, and various concentrations of 6-BA, NAA, and 2,4-D were added to form the artificial endosperm. Initially, each explant was immersed in a 3.0% sodium alginate solution for 3–4 min to ensure that it covered the bulblets. Subsequently, the explants were exposed to a 2% CaCl2 (Chronchem) solution for 10 min. After washing with sterile water, the resulting alginate spheroids were used as artificial seeds. Each treatment was repeated three times, with each replicate containing 20 artificial seeds. The culture condition of artificial seeds germination was MS medium without PGRs and cultured in a light incubator. After 45 days, the germination rate was counted. The standard for germination was considered the explants broke through the seed coat by 3 mm. Germination rate = (number of germinated artificial seeds/number of artificial seeds sown) × 100%.
2.7 Addition of Other Additives and Production of Double-Layer Artificial Seeds
A 3.0% sodium alginate solution with 2.0 mg·L−1 6-BA and 0.5 mg·L−1 NAA was prepared using MS liquid medium. Additionally, various concentrations of chitosan (Macklin), carbendazim (Sichuan Runer Technology, China), and activated carbon (Chronchem) were added as other additives and an orthogonal experiment was designed with three factors and three levels. The production method for single-layer artificial seed was the same as described above. After the first encapsulation, the double-layer artificial seeds were washed three times in sterile water. Subsequently, they were mixed with a 3% sodium alginate solution for 3–4 min again, and then placed in a 2% CaCl2 solution for 10 min. Finally, washed with sterile water three times to obtain the double-layer artificial seeds. Each replicate contained 25 artificial seeds, and each treatment was repeated three times. Artificial seeds were incubated in a MS medium without PGRs and cultured in a light incubator. The germination rate was counted after 45 days.
2.8 Data Analysis and Statistics
IBM SPSS Statistics 26.0 software (International Business Machines Corporation, New York, USA) was used for analysis of variance and multiple comparisons (Duncan), and the results were presented as the mean ± standard deviation.
3.1 Effects of PGRs on Callus Induction
As shown in Table 1, explants are initiated callus formation on MS medium supplemented with various concentrations of cytokinin and auxin. The results indicated that three different types of calli were induced under the treatment of PGRs. Fig. 1A shows that the callus induced on the MS medium supplemented with 3.0 mg·L−1 NAA and 0.2 mg·L−1 TDZ was more compact, with an induction rate of 61.22%. In contrast, loose callus was induced on MS medium supplemented with 3.0 mg·L−1 TDZ and 0.2 mg·L−1 NAA (Fig. 1B) with an induction rate of 72.84%, while the MS medium containing picloram induced light yellow, translucent embryogenic callus (Fig. 1C). The highest callus induction rate (84.93%) was observed at picloram concentration of 1.0 mg·L−1. However, the rate of callus induction reduced as with concentration increase, indicating that callus induction was dependent on a certain range concentration. Compared to the combination of TDZ with NAA or 2,4-D, the callus induction rate was the highest when picloram alone use. Therefore, supplemented with 1.0 mg·L−1 picloram on MS medium was more conducive to induced callus.
Figure 1: Different stages of in vitro regeneration and artificial seeds of Fritillaria cirrhosa. (A) Compact callus was induced on Murashige and Skoog (MS) medium supplemented with 3.0 mg·L−1 α-naphthaleneacetic acid (NAA) and 0.2 mg·L−1 TDZ (thidiazuron). (B) Loose callus was induced on MS medium containing 3.0 mg·L−1 TDZ and 0.2 m mg·L−1 NAA. (C) Embryogenic callus was induced on MS medium supplemented with 1.0 mg·L−1 picloram. (D) Regenerated bulblets were induced from callus on MS medium containing 1.0 mg·L−1 6-benzyladenine (6-BA) and 0.2 mg·L−1 NAA. (E) Adventitious buds were induced from regenerated bulblet sections on MS medium supplemented with 2.0 mg·L−1 6-BA and 0.5 mg·L−1 NAA. (F) Bulblets were induced from regenerated bulblet sections on MS medium appended to 2.0 mg·L−1 6-BA and 0.5 mg·L−1 NAA. (G) Regenerated bulblets germinated in soil matrix. (H) Artificial seeds encapsulated in 3% sodium alginate. (I) Artificial seed germination. The scale in the figure is 0.5 cm
3.2 Effects of PGRs on Callus Differentiation
After the callus was inoculated into the differentiation MS medium for 10 days, the callus volume increased, and the protruding part changed color to yellowish green. About 20 days later, a small number of bulblets began to grow, and a dense cluster of bulblets grew after 45 days (Fig. 1D). Table 2 shows that the callus differentiation rate and regeneration coefficient vary among the six PGRs combinations. Adding 1.0 mg·L−1 NAA and 1.0 mg·L−1 2,4-D on MS medium had the highest callus regeneration rate of 84.62%. However, the regeneration coefficient was modest, with an average of 3.20 bulblets per callus. The highest regeneration coefficient was exhibited on MS medium containing 1.0 mg·L−1 6-BA and 0.2 mg·L−1 NAA, the number of bulblets that were differentiated from each callus amounted to 6.70, and the differentiation rate was 77.92%. Therefore, MS medium supplemented with 1.0 mg·L−1 6-BA and 0.2 mg·L−1 NAA was more suitable for differentiation culture of callus.
3.3 Effects of PGRs on Bulblet and Adventitious Bud Induction
After inoculating bulblet sections into the MS induction medium, bulblets and adventitious buds formed within 45 days. Different PGRs had different effect on bulblet and adventitious bud induction rates (Table 3). MS medium supplemented with 1.0 mg·L−1 2,4-D and 0.3 mg·L−1 6-BA had the highest bulblet induction rate (88.17%) with a regeneration coefficient of 4.27 (Fig. 1F). However, when the 6-BA concentration increased to 1.0 mg·L−1, the bulblets induction rate decreased significantly. This indicated that a high cytokinin concentration was not conducive to the bulblets inducted at a certain auxin concentration. The combination of TDZ and NAA only induced adventitious buds (Fig. 1E). 1.0 mg·L−1 TDZ and 0.3 mg·L−1 NAA had the highest adventitious bud induction rate (76.39%) and regeneration coefficient (4.15). However, the highest adventitious bud induction rate was on the MS medium + 2.0 mg·L−1 6-BA + 0.5 mg·L−1 NAA (84.24%), and the regeneration coefficient was 4.56. Therefore, the combination of 6-BA and 2,4-D was relatively effective in inducing bulblets, and the combination of 6-BA and NAA was more suitable for adventitious bud induction.
3.4 Germination Conditions of Regenerated Bulblets
The regenerated bulblets germinated after transplanting to mixed soil for 30 days (Fig. 1G). The results showed that low-temperature treatment was helpful in breaking dormancy and germination of bulblets (Table 4). After 45 days of low-temperature treatment at 4°C, the germination rate increased to 42.8%. In contrast, the germination rate of regenerated bulblets without low-temperature treatment was only 8.9%.
3.5 Effects of PGRs on Germination and Rooting of Artificial Seeds
The types and combinations of PGRs added to artificial endosper (Fig. 1H) directly affected the germination and rooting of artificial seeds, and the effects were inconsistent (Table 5). The constituent of 6-BA and NAA had the highest germination and rooting rate. When the 6-BA concentration was 1.0 mg·L−1, the germination and rooting rate increased with the concentration NAA. When the concentration of 6-BA increased to 2.0 mg·L−1, with increasing NAA concentration germination rate first increased and then decreased, the rooting rate showed an increasing trend. Adding 0.3 mg·L−1 NAA and 2.0 mg·L−1 6-BA to the artificial endosperm had the highest germination rate (84.62%), but the rooting rate was only 11.68%. When the NAA concentration was 0.5 mg·L−1, the germination rate was 81.67% and the rooting rate was increased to 20.56%. Therefore, in a comprehensive comparison, adding 2.0 mg·L−1 6-BA and 0.5 mg·L−1 NAA to artificial endosperm has a positive effect on artificial seeds germination and rooting.
3.6 Effects of Other Additives and Artificial Seed Coat on Artificial Seed Germination
Under sterile conditions, single-layer artificial seeds began to germinate about 16 days, and the initial germination time of double-layer artificial seeds was extended to about 20 days. After 30 days, both single-layer and double-layer artificial seeds grew into regenerated plantlets (Fig. 1I). Table 6 shows that the germination rate of single-layer artificial seeds under nine treatments is higher than that of double-layer artificial seeds. The range analyses revealed that factor A (chitosan) exhibited the largest effect on germination rate of single-layer artificial seeds among three factors, followed by carbendazim, and activated carbon was the smallest; that is, the order of affecting the germination rate of artificial seeds was chitosan > carbendazim > activated carbon. The analysis of variance indicated that the germination rate was significantly (p < 0.05) affected by chitosan (Table S1). With the increase in chitosan and carbendazim concentrations, the K value decreased, and the K value was the largest when the chitosan concentration was 0 g·L−1 and carbendazim concentration was 1 g·L−1. The K value of activated carbon first increased and then decreased as the concentration increase. Therefore, adding 1.0 g·L−1 carbendazim and 1.0 g·L−1 activated carbon was beneficial to the germination of single-layer artificial seeds.
The range analyses of double-layer artificial seed germination rate showed that the R value of chitosan was the largest, followed by activated carbon and carbendazim. Consistent with the results of single-layer artificial seed variance analysis, chitosan has an indispensable effect on germination rate (p < 0.05) (Table S2). The results of adding chitosan and carbendazim were consistent with single-layer artificial seeds, but the K values of activated carbon decreased with the concentration increased, indicating that 1.0 g·L−1 carbendazim was helpful to the germination of double-layer artificial seeds, and the germination rate was 60.03%. Nevertheless, the germination rate was lower than that of single-layer artificial seeds. Therefore, the best component of artificial seeds was a single-layer artificial seed coat + 3% sodium alginate (w/v) + 3% sucrose (w/v) + MS + 2.0 mg·L−1 6-BA + 0.5 mg·L−1 NAA + 1.0 g·L−1 carbendazim + 1.0 g·L−1 activated carbon.
Selecting a suitable propagation method is an effective measure for protecting endangered plants and conserving wild resources. In vitro culture technology has been successfully applied to protect threatened plants [23,24]. Bulb explants are the most commonly used primary explants for in vitro culture of Fritillaria [10,25]. In this study, Fritillaria cirrhosa D. Don bulbs were used as explants and successful induced callus and bulblet regeneration by using differeent cytokinins (TDZ, 6-BA) and auxins (NAA, picro-larrn,2,4-D) in vitro. The type and concentration of PGRs are dominant factors in modulating callus induction and morphogenesis formation [26]. Previous studies have demonstrated that 2,4-D is widely used for callus induction and proliferation, successfully inducing callus in various plant species [27,28]. However, this study identified the picloram concentration had a distinctive effect in embryogenic callus induction in F. cirrhosa, which was concentration-dependent. 1.0 mg·L−1 picloram could induce embryogenic callus up to 84.93%, but too high concentration of picloram inhibited callus formation, which was also reported in Urochloa brizantha cvs. Xaraés and Piatã [29]. High efficiency callus formation was also achieved of F. roylei by the addition of 0.5 mg·L−1 TDZ and 2.0 mg·L−1 picloram on MS medium [5]. Simultaneously, embryogenic callus showed higher differentiation ability and higher regeneration coefficient.
For F. cirrhosa, as a geophyte plant, bulblets formation is an essential step in the micropropagation process because only bulblets show strong high rooting ability [30]. A combination of cytokinins and auxins can effectively induce bulblet formation [31]. TDZ and 6-BA were commonly used cytokinins to induce bulblets in vitro [32,33], highest regeneration frequency of F. imperialis and F. persica were observed on MS medium supplemented with 0.5 mg·L−1 TDZ and 0.5 mg·L−1 NAA [11]. However, in this study, only the combination of TDZ and NAA induced adventitious buds. In contrast, 6-BA had a significant effect on bulblet induction, and 6-BA combined with low concentration of 2,4-D decidedly increased the induction rate of bulblets. Zhao et al. utilized 2.0 mg·L−1 6-BA and 0.5 mg·L−1 NAA in MS medium for bulb regeneration [12]. This suggests that various species necessitate distinct PGRs for stimulating bulblet formation, even within the same genus.
Transplantation of regenerated bulblets requires an indispensable step of domestication. However, bulbous species have the characteristic of bulb dormancy. Transferring bulblets to in vitro culture delays germination and hampers further development [34]. Several authors have obtained results showing that low-temperature have a stimulating effect on root formation and overcome dormancy during bulblet culture in vitro [35,36]. The regenerated bulblets germination rate in this study was significantly improved after low-temperature treatment. Then, some studies suggested that the use of artificial seeds can avoid the domestication steps that must be performed during transplanting, providing greater flexibility to transplanting [15]. The artificial seeds formed by encapsulating somatic embryos of the endangered plant Spathoglottis plicata can germinate and root on MS medium, and successfully survive in the greenhouse, which is helpful to relieve the pressure on its wild population [37]. The addition of nutrients and PGRs to the artificial endosperm is a critical component to the success of the artificial seed. Basal medium and sucrose can provide nutrients for the germination of artificial seed. Ananthan et al. found that MS medium encapsulating nodes showed a higher conversion rate compare to encapsulating with distilled water in artificial endosperm matrix, indicating that appropriate nutrient supply may be a determinant of artificial seed germination [24]. PGRs can control the germination and rooting of artificial seeds and reduce the difficulty of germination [38]. NAA is commonly used to stimulate the rhizogenesis of monocotyledonous geophytes [39]. The seeds of F. cirrhosa were successfully germinated by Cheng et al. adding 0.4 mg·L−1 NAA and 1.0 mg·L−1 6-BA in MS medium [12]. At the same time, this study found that the addition of NAA in artificial endosperm significantly increased the rooting rate of artificial seeds. Hence, adding suitable PGRs to artificial endosperrn is essential for enhancing the growth of artificial seeds.
Furthermore, activated carbon and carbendazim also had positive effects on the germination of artificial seed. Wang et al. added 1% activated carbon to sodium alginate to encapsulate Pogonatherum paniceum cluster buds, which had a high conversion rate and strong seedling ability [40]. The results obtained in the aforementioned studies are consistent with the results of this study. The use of activated carbon in artificial seeds may be attributed to its ability to create a dark environment and adsorb organic compounds, as well as inhibit the diffusion and respiration of nutrients [41]. The addition of carbendazim has been shown to decrease the microbial contamination of artificial seeds [42]. After the addition of carbendazim, there was no fungal contamination in the artificial seeds from storage to plant transformation [43].
Certain medicinal plants face challenges in traditional reproductive processes, such as a small endo-sperm, a low germination rate, and seeds that are perishable over time, and F. cirrhosa is among these medicinal plants [44,45]. Artificial seed technology offers clear advantages in mitigating these issues and holds promise for large-scale production [15], thereby aiding the industrialization of F. cirrhosa resources.
This study reported a method for rapid acquisition of regenerated bulblets and artificial seeds of the endangered medicinal plant F. cirrhosa. Callus was induced from bulb explants on MS medium supplemented with 1.0 mg·L−1 picloram, and callus formed bulblets on MS medium enriched with 1.0 mg·L−1 6-BA + 0.2 mg·L−1 NAA. Bulblets and adventitious buds were induced from aseptic bulblet sections in MS medium fortified with 1.0 mg·L−1 2,4-D + 0.3 mg·L−1 6-BA and 0.5 mg·L−1 NAA + 2.0 mg·L−1 6-BA, respectively. The survival rate of regenerated bulblets in the soil matrix after low-temperature treatment was 42.8%. The germination rate of artificial seeds supplemented with 2.0 mg·L−1 6-BA + 0.5 mg·L−1 NAA in the artificial endosperm was 81.67%. The addition of 1.0 g·L−1 carbendazim and 1.0 g·L−1 activated carbon promoted artificial seeds germination, and the germination rate was higher than that of regenerated bulblets. This study provides an artificial regeneration technology scheme for the large-scale production of F. cirrhosa, provides technical conditions related to the preservation of F. cirrhosa germplasm resources and the protection of wild resources, and provides a solution for the in vitro propagation of other endangered plants.
Acknowledgement: The authors thank the reviewers for their thoughtful comments to improve our manuscript.
Funding Statement: This research was funded by the National Key Research and Development Program of China (2018YFC1706101) and the Science and Technology Program of Sichuan Province, China (2021YFS0045).
Author Contributions: The authors confirm contribution to the paper as follows: study conception and design: Q. Tao, B. Ma and Z. Yan; data collection: Q. Tao, C. Zhao and B. Ma; H. Jia, G. Han and W. Li prepared samples for all analyses, Q. Tao, B. Ma and G. Han conducted the experiments and wrote the manuscript. All authors reviewed the results and approved the final version of the manuscript.
Availability of Data and Materials: Data are available from the corresponding author on request.
Ethics Approval: Not applicable.
Conflicts of Interest: The authors declare that they have no conflicts of interest to report regarding the present study.
Supplementary Materials: The supplementary material is available online at https://doi.org/10.32604/phyton.2024.051923.
References
1. El Merzougui S, Benelli C, El Boullani R, Serghini MA. The cryopreservation of medicinal and ornamental geophytes: application and challenges. Plants. 2023;12(11):2143. doi:10.3390/plants12112143. [Google Scholar] [PubMed] [CrossRef]
2. Chen T, Zhong FR, Yao C, Chen J, Ma YT. A systematic review on traditional uses, sources, phytochemistry, pharmacology, pharmacokinetics, and toxicity of Fritillariae Cirrhosae Bulbus. Evid-Based Compl Alt. 2020;2020(5):1–26. [Google Scholar]
3. Rashid I, Yaqoob U. Traditional uses, phytochemistry and pharmacology of genus Fritillaria—a review. Bull Natl Res Cent. 2021;45(1):124. doi:10.1186/s42269-021-00577-z. [Google Scholar] [CrossRef]
4. Wu F, Tian M, Sun Y, Wu C, Liu X. Efficacy, chemical composition, and pharmacological effects of herbal drugs derived from Fritillaria cirrhosa D. Don and Fritillaria thunbergii Miq. Front Pharmacol. 2022;13:985935. doi:10.3389/fphar.2022.985935. [Google Scholar] [PubMed] [CrossRef]
5. Kumar P, Partap M, Rana D, Warghat AR. Metabolite and expression profiling of steroidal alkaloids in wild tissues compared to bulb derived in vitro cultures of Fritillaria roylei-high value critically endangered Himalayan medicinal herb. Ind Crop Prod. 2020;145:111945. doi:10.1016/j.indcrop.2019.111945. [Google Scholar] [CrossRef]
6. Yu J, Wei JH, Chen SL, Dai Y, Yang CM. Dormancy and germination characteristics of Fritillaria cirrhosa seed. Chin Tradi Herb. 2008;39:1081–4 (In Chinese). [Google Scholar]
7. Loyola-Vargas VM, Ochoa-Alejo N. An introduction to plant tissue culture: advances and perspectives. Methods Mol Biol. 2018;1815:3–13. doi:10.1007/978-1-4939-8594-4. [Google Scholar] [CrossRef]
8. Yang L, Wu KL, Qiu SX, Cao HL, Deng SL. A plant regeneration protocol from callus cultures of medicinal plant Rhodomyrtus tomentosa. Plant Cell Tiss Org. 2023;153(2):307–17. doi:10.1007/s11240-023-02464-z. [Google Scholar] [CrossRef]
9. Ebrahimzadegan R, Maroufi A. In vitro regeneration and Agrobacterium-mediated genetic transformation of Dragon’s Head plant (Lallemantia iberica). Sci Rep. 2022;12(1):1784. doi:10.1038/s41598-022-05776-w. [Google Scholar] [PubMed] [CrossRef]
10. Petrić M, Subotić A, Jevremović S, Trifunović M. Somatic embryogenesis and bulblet regeneration in snakehead fritillary (Fritillaria meleagris L.). Afr J Biotechnol. 2011;10:16181–8. [Google Scholar]
11. Cakmak D, Sancak C, Karaoglu C, Aasim M, Parmaksiz I, Ozcan S. Efficient in vitro bulblet regeneration from immature zygotic embryos of Fritillaria imperialis and F. persica. Fresen Environ Bull. 2016;25(8):2795–802. [Google Scholar]
12. Chang HC, Xie HM, Lee MR, Lin CY, Yip MK, Agrawal DC, et al. In vitro propagation of bulblets and LC-MS/MS analysis of isosteroidal alkaloids in tissue culture derived materials of Chinese medicinal herb Fritillaria cirrhosa D. Don. Bot Stud. 2020;61:1–9. [Google Scholar]
13. Hao L, Teixeira da Silva JA, Yu X. Bulblet regeneration in vitro from bulb-scales of Fritillaria thunbergii. Acta Hortic. 2017;1171:25–30. [Google Scholar]
14. Zhao Q, Li R, Zhang Y, Huang K, Wang W, Li J. Transcriptome analysis reveals in vitro-cultured regeneration bulbs as a promising source for targeted Fritillaria cirrhosa steroidal alkaloid biosynthesis. 3 Biotech. 2018;8:1–10. [Google Scholar]
15. Rihan HZ, Kareem F, El-Mahrouk ME, Fuller MP. Artificial seeds (principle, aspects and applications). Agronomy. 2017;7(4):71. doi:10.3390/agronomy7040071. [Google Scholar] [CrossRef]
16. Ouzhand B, Mohayeji M, Pourseyedi S, Abdolshahi R. Dormancy breaking, indirect somatic embryogenesis, and encapsulation of somatic embryos in Black Zira. Plant Cell Tiss Org. 2023;155(1):197–208. doi:10.1007/s11240-023-02571-x. [Google Scholar] [CrossRef]
17. Hatzilazarou S, Kostas S, Nendou T, Economou A. Conservation, regeneration and genetic stability of regenerants from Alginate-Encapsulated shoot explants of Gardenia jasminoides Ellis. Polymers. 2021;13(10):1666. doi:10.3390/polym13101666. [Google Scholar] [PubMed] [CrossRef]
18. Bustam S, Sinniah UR, Kadir MA, Zaman FQ, Subramaniam S. Selection of optimal stage for protocorm-like bodies and production of artificial seeds for direct regeneration on different media and short term storage of Dendrobium Shavin White. Plant Growth Regul. 2013;69:215–24. doi:10.1007/s10725-012-9763-6. [Google Scholar] [CrossRef]
19. Kamińska M, Kęsy J, Trejgell A. Abscisic acid in preservation of Taraxacum pieninicum in the form of synthetic seeds in slow growth conditions. Plant Cell Tiss Org. 2021;144:295–312. doi:10.1007/s11240-020-01924-0. [Google Scholar] [CrossRef]
20. Vikram P, Ripain IHA, Chiruvella KK, Arifullah M. Rapid induction of somatic embryos and production of synthetic seeds from hempedu bumi (Andrographis paniculata)–a malay ethnomedicinal plant. J Tropic Resou Sustain. 2015;3(1):34–8. [Google Scholar]
21. Nandini B, Giridhar P. Insight view of topical trends on synthetic seeds of rare and endangered plant species and its future prospects. In: Faisal M, Alatar AA, editors. Synthetic seeds. Cham: Springer International Publishing; 2019. p. 113–54. [Google Scholar]
22. Murashige T, Skoog F. A revised medium for rapid growth and bio assays with tobacco tissue cultures. Physiol Plantarum. 1962;15(3):473–97. doi:10.1111/ppl.1962.15.issue-3. [Google Scholar] [CrossRef]
23. Beena MR, Martin KP, Kirti PB, Hariharan M. Rapid in vitro propagation of medicinally important Ceropegia candelabrum. Plant Cell Tiss Org. 2003;72(3):285–9. doi:10.1023/A:1022395809204. [Google Scholar] [CrossRef]
24. Ananthan R, Mohanraj R, Bai VN. In vitro regeneration, production, and storage of artificial seeds in Ceropegia barnesii, an endangered plant. In Vitro Cell Dev-Pl. 2018;54(5):553–63. doi:10.1007/s11627-018-9934-x. [Google Scholar] [CrossRef]
25. Witomska M. Effect of temperature on the in vitro propagation Fritillaria imperialis L. Haematologica. 2008;94(1):146–8. [Google Scholar]
26. Fan Y, Tang Z, Wei J, Yu X, Guo H, Li T, et al. Dynamic transcriptome analysis reveals complex regulatory pathway underlying induction and dose effect by different exogenous auxin IAA and 2,4-D during in vitro embryogenic redifferentiation in cotton. Front Plant Sci. 2022;13:931105. doi:10.3389/fpls.2022.931105. [Google Scholar] [PubMed] [CrossRef]
27. Al-Ajlouni ZI, Abbas S, Shatnawi M, Al-Makhadmeh I. In vitro propagation, callus induction, and evaluation of active compounds on Ruta graveolens. J Food Agric Environ. 2015;13(2):101–6. [Google Scholar]
28. Farhadi N, Panahandeh J, Azar AM, Salte SA. Effects of explant type, growth regulators and light intensity on callus induction and plant regeneration in four ecotypes of Persian shallot (Allium hirtifolium). Sci Hortic. 2017;218:80–6. doi:10.1016/j.scienta.2016.11.056. [Google Scholar] [CrossRef]
29. Takamori LM, Neto NBM, Vieira LGE, Ribas AF. Optimization of somatic embryogenesis and in vitro plant regeneration of Urochloa species using picloram. In Vitro Cell Dev-Pl. 2015;51:554–63. doi:10.1007/s11627-015-9701-1. [Google Scholar] [CrossRef]
30. Podwyszyńska M. The mechanisms of in vitro storage organ formation in ornamental geophytes. Floric Orna Biotec. 2015;6:9–23. [Google Scholar]
31. Kim M, Jeon J, Youm J, Kim J, Lee B, Kang W, et al. Efficient plantlet regeneration via callus formation from leaf segment of Lilium oriental hybrid ‘Casa Blanca’. J Plant Biotechnol. 2005;7:129–34. [Google Scholar]
32. Uranbey S, Ipek A, Caliskan M, Dundar E, Cocu S, Basalma D, et al. In vitro bulblet induction from bulb scales of endangered ornamental plant Muscari Azureum. Biotechnol Biotec Equip. 2010;24(2):1843–8. doi:10.2478/V10133-010-0024-4. [Google Scholar] [CrossRef]
33. Thomas TD. High-frequency, direct bulblet induction from rhizome explants of Curculigo orchioides Gaertn., an endangered medicinal herb. In Vitro Cell Dev-Pl. 2007;43(5):442–8. doi:10.1007/s11627-007-9091-0. [Google Scholar] [CrossRef]
34. Nikolić M, Mišić D, Maksimović V, Jevremović S, Subotić A. Effect of low temperature on rooting rate and carbohydrate content of Fritillaria meleagris bulbs formed in culture in vitro. Arch Biol Sci. 2008;60(1):5–6. doi:10.2298/ABS0801167N. [Google Scholar] [CrossRef]
35. Yae BW, Han BH, Goo DH. Dormancy breaking and in vivo growth of in vitro bulblets in Lilium 0riental hybrid ‘Casa Blanca’. Hortic Environ Biote. 2001;42(1):99–102. [Google Scholar]
36. Langens-Gerrits MM, Miller WBM, Croes AF, Klerk GJ. Effect of low temperature on dormancy breaking and growth after planting in lily bulblets regenerated in vitro. Plant Growth Regul. 2003;40(3):267–75. doi:10.1023/A:1025018728178. [Google Scholar] [CrossRef]
37. Manokari M, Priyadharshini S, Shekhawat MS. Direct somatic embryogenesis using leaf explants and short term storage of synseeds in Spathoglottis plicata Blume. Plant Cell Tiss Org. 2021;145(2):321–31. doi:10.1007/s11240-021-02010-9. [Google Scholar] [CrossRef]
38. Malabadi RB, Staden J. Storability and germination of sodium alginate encapsulated somatic embryos derived from the vegetative shoot apices of mature Pinus patula trees. Plant Cell Tiss Org. 2005;82(3):259–65. doi:10.1007/s11240-005-1313-8. [Google Scholar] [CrossRef]
39. Muraseva DS, Novikova TI. Efficient protocol for in vitro propagation from bulb scale explants of Fritillaria ruthenica Wikstr. (Liliaceaea rare ornamental species. Rend Lincei-Sci Fis. 2018;29:491–7. doi:10.1007/s12210-018-0693-8. [Google Scholar] [CrossRef]
40. Wang WG, Wang SH, Wu XA, Jin XY, Chen F. High frequency plantlet regeneration from callus and artificial seed production of rock plant Pogonatherum paniceum (Lam.) Hack. (Poaceae). Sci Hortic. 2007;113(2):196–201. doi:10.1016/j.scienta.2007.03.006. [Google Scholar] [CrossRef]
41. Cheruvathur MK, Abraham J, Mani B, Dennis Thomas T. Adventitious shoot induction from cultured internodal explants of Malaxis acuminata D. Don, a valuable terrestrial medicinal orchid. Plant Cell Tiss Org. 2010;101(2):163–70. doi:10.1007/s11240-010-9673-0. [Google Scholar] [CrossRef]
42. Pattnaik SK, Sahoo Y, Chand PK. Efficient plant retrieval from alginate-encapsulated vegetative buds of mature mulberry trees. Sci Hortic. 1995;61(3–4):227–39. [Google Scholar]
43. Ramesh M, Marx R, Mathan G, Pandian SK. Effect of bavistin on in vitro plant conversion from encapsulated uninodal microcuttings of micropropagated Bacopa monnieri (L.)—an ayurvedic herb. J Environ Biol. 2009;30(3):441–4. [Google Scholar]
44. Ahmad Z, Shahzad A. Synseed production, propagation, and conservation. In: Faisal M, Alatar AA, editors. Synthetic seeds: germplasm regeneration, preservation and prospects. Cham: Springer International Publishing; 2019. p. 217–31. [Google Scholar]
45. Gantait S, Kundu S, Ali N, Sahu NC. Synthetic seed production of medicinal plants: a review on influence of explants, encapsulation agent and matrix. Acta Physiol Plant. 2015;37(5):98. doi:10.1007/s11738-015-1847-2. [Google Scholar] [CrossRef]
Cite This Article
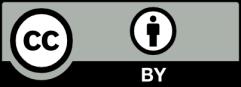