Open Access
ARTICLE
Belowground Bud Bank Is Insensitive to Short-Term Nutrient Addition in the Meadow Steppe of Inner Mongolia
1 College of Forestry, Henan Agricultural University, Zhengzhou, 450002, China
2 Institute of Ecological Conservation and Restoration, Chinese Academy of Forestry, Beijing, 100093, China
3 Institute of Applied Ecology, Chinese Academy of Sciences, Shenyang, 110164, China
4 Institute of Botany, Chinese Academy of Sciences, Beijing, 100093, China
5 Liaoning Metallurgical Geological Exploration Research Institute Co., Anshan, 114038, China
* Corresponding Author: Jianqiang Qian. Email:
(This article belongs to the Special Issue: Grassland Ecology in China under Global Change)
Phyton-International Journal of Experimental Botany 2024, 93(6), 1129-1141. https://doi.org/10.32604/phyton.2024.051405
Received 05 March 2024; Accepted 18 April 2024; Issue published 27 June 2024
Abstract
Human activities and industrialization have significantly increased soil nutrients, such as nitrogen (N) and phosphorus (P), profoundly impacting the composition and structure of plant community, as well as the ecosystem functions, especially in nutrient-limited ecosystems. However, as the key propagule pool of perennial grasslands, how belowground bud bank and its relationship with aboveground vegetation respond to short-term changes in soil nutrients was still unclear. In this study, we conducted a short-term (2021–2022) soil fertilization experiment with N addition (10 g N m yr) and P addition (5 g N m yr) in the meadow steppe of Inner Mongolia, China, to explore the responses of belowground bud bank, aboveground shoot population and their relationships (represented by the ratio of bud to shoot density-meristem limitation index (MLI)) for the whole community and three plant functional groups (perennial rhizomatous grasses-PR, perennial bunchgrasses-PB, and perennial forbs-PF) to nutrient addition. The short-term nutrient addition had no significant influences on belowground bud density, aboveground shoot density, and MLI of the whole plant community. Plant functional groups showed different responses to soil fertilization. Specifically, N addition significantly increased the bud density and shoot density of PR, especially in combination with P addition. N addition reduced the shoot density of PF but had no influence on its bud density and MLI. Nutrient addition had significant effects on the three indicators of PB. Our study indicates that the belowground bud bank and its relationship with aboveground vegetation in temperate meadow steppe are insensitive to short-term soil fertilization, but plant functional groups exhibit specific responses in terms of population regeneration, which implies that plant community composition and ecosystem functions will be changed under the ongoing global change.Keywords
Under global change and human activities, the increasing atmospheric deposition has profoundly influenced terrestrial ecosystems by continually enhancing soil nutrient availability [1]. Fossil fuels combustion and agricultural activities have markedly increased the influx of nitrogen (N) and phosphorus (P) into terrestrial ecosystems [2], and the N and P inputs are anticipated to rise to 3–4 times the preindustrial levels by the year 2050 [3,4]. The increased soil nutrient leads to the shift in plant community composition and structure [5], and thus poses great influences on ecosystem functions (e.g., aboveground productivity) [6–8]. However, the mechanisms underlying the changes in the composition of the community and functions of the ecosystem under changing soil nutrients have not been fully explored.
Grasslands are important terrestrial ecosystems providing numerous functions and services such as animal husbandry production, biodiversity conservation, carbon sequestration, wind-breaking, and sand fixation [9]. Grassland ecosystems have been confirmed to be limited by soil nutrient availability [10]. The increasing N input caused by atmospheric deposition or anthropogenic addition may to some extent alleviate or eliminate N limitation in grasslands [11], thereby modifying diverse ecological processes and exerting profound impacts on community composition, structure, and ecosystem functions in grasslands [12,13]. For instance, N deposition enhances aboveground net primary productivity (ANPP) while reducing species diversity [14–18]. Moreover, N enrichment often results in a pronounced stoichiometric imbalance between N and P, causing grasslands being N limited to become P limited or the co-limitation by both N and P [19,20]. In this case, P is also considered as a limited nutrient in grasslands. However, the influence of P addition on grassland ecosystems remains controversial. For instance, short-term P addition increases the aboveground biomass [21,22], whereas long-term P addition does not influence the primary productivity of different types of grasslands [8,23,24]. The contrasting effects of P addition on grassland productivity might be attributed to the duration of experiments. Up to date, how population regeneration and/or recruitment in grasslands respond to changing soil nutrient availability still needs to be clarified.
In perennial grassland ecosystems, belowground bud banks are crucial for plant populations and communities to disturbances and environmental changes. Belowground bud banks could offer an effect of demographic storage, thus mitigating population fluctuations and stabilizing plant communities [25]. Besides, the resistance of bud banks to environmental changes or disturbances (e.g., drought and fire) can enhance ecosystem resilience once those adverse events cease [26–29]. Meanwhile, plants could adjust their belowground bud banks in response to changing soil nutrient availability. For instance, N addition significantly modified the demography of the bud bank by increasing the emergence of bud banks in the tallgrass prairie of North America [30]. Similarly, N addition negatively impacted the belowground bud bank of temperate steppe in Inner Mongolia [31]. However, the majority of research have concentrated on the impact of N addition on belowground bud banks, and few studies have explored the influence of P addition and the combined treatment of N and P on belowground bud banks and their relationship with aboveground vegetation.
Given that the distinct responses of various bud types to environmental changes and disturbances, as well as their different contributions to population regeneration and vegetation development [32], the influence of changing soil resource availability on belowground bud banks may vary in plant functional groups. For example, species with bulb buds in their swollen bases predominate in more arid regions while rhizomatous grasses with rhizome buds are found in the relatively moist grassland along the natural climatic gradient in Northern China [33]. And the belowground bud bank of bunchgrasses and rhizomatous grasses exhibit distinct responses to extreme drought in the typical steppe of Inner Mongolia [29]. Besides, the belowground bud bank, aboveground shoot population, and their relationships of grasses and forbs respond differently to extreme drought across the aridity gradient in the grasslands of Inner Mongolia, China [34]. Perennial forbs produce more buds and their sprouting shoots in nutrient-rich environments [35]. The bud bank of bunchgrasses exhibited an increase in short-term N addition but a decrease in long-term N addition, whereas the bud bank size of rhizomatous grasses increased under long-term N addition [36,37]. Therefore, the changes in soil nutrients might not only affect the belowground bud bank demographic of the whole plant community but also lead to the shift in belowground bud bank composition (i.e., different plant functional groups). Exploring the reactions of both bud bank density and composition is crucial for forecasting the dynamics of community structure and functions of ecosystem in grasslands amid global changes, especially for the increased N deposition and changing soil nutrient availability.
Here, we conducted a short-term (2 years) soil fertilization (N addition, P addition, and the combined addition of N and P) experiment in a meadow steppe in Inner Mongolia, China. The belowground bud density, aboveground shoot density, and their ratios (represented by the meristem limitation index-MLI) of the whole plant community and three key functional groups (perennial rhizomatous grasses, perennial bunchgrasses, and perennial forbs) were investigated with the purpose to address two questions: (1) how the belowground bud bank and its relationship with aboveground vegetation respond to short-term soil nutrient addition; (2) whether soil fertilization has varying influences on belowground bud bank among various functional groups?
This study was conducted at Eco-animal Husbandry Experimental Zone, Chinese Academy of Sciences, located in the Xeltala cattle breeding farm in Hulunbuir, Inner Mongolia (120°0′E, 49°17′N, 680 m a.s.l.). The primary soil classification prevalent in this area is kastanozems, categorized in accordance with the FAO/Unesco System of Soil Classification. This area is situated within a semi-arid continental climate, characterized by an average annual temperature of −3°C and an average annual precipitation of 375 mm. The vegetation is characterized as meadow steppe, including Leymus chinensis, Carex duriuscula, Carex pediformis, Poa pratensis, Thalictrum squarrosum, Pulsatilla turczaninovii, Cleistogenes squarosa, Artemisia tanacetifolia, and Potentilla bifurca.
Within the enclosed natural steppe, the Natural Grassland Restoration Multi-factor Experiment Platform (207 m × 111 m) took place in 2021. The experiment was a split-plot design involving seed addition and fertilizer application. We selected the N and P addition treatments on this platform, resulting in a full-factorial block-designed experiment. It consisted of 40 plots divided into ten blocks, each accommodating four plots (10 m2), including Control (ambient, no nutrient addition), P addition (5 g P m−2 yr−1, calcium superphosphate), N addition (10 g N m−2 yr−1, urea), and the combined treatment of N and P addition (10 g N m−2 yr−1 and 5 g P m−2 yr−1). During mid-May of each year, the solid particles of nutrients were manually scattered to experimental plots by hand in an even distribution manner.
2.3 Field Investigation and Sampling
In early September of 2022 (at the end of growing season), we conducted an assessment of the belowground bud density and aboveground shoot density within each experimental plot’s 0.3 m2 square quadrat. Recognizing that the majority of belowground buds are concentrated within the 0–30 cm soil profile of this steppe, we excavated all belowground components to this depth [31,34]. Throughout the sampling process, the integrity of the connection between aboveground shoots and belowground organs was maintained to facilitate subsequent species identification for belowground buds. Soil was meticulously cleaned, and the samples (comprising rhizomes, roots, and aboveground plant parts) were placed in plastic bags and transported to the laboratory. The samples stored in an incubator (4°C) before bud identification and counting. According to the procedure of previous studies [31], belowground buds were categorized into four types: tiller buds (axillary buds growing at the base of shoot in caespitose species and rhizomatous grasses), rhizome buds (axillary buds and apical buds on hypogeous rhizomes), bulb buds (meristems wrapped in the swollen leaf base or scale leaf of bulbous species) and dicot buds (buds growing on belowground parts of dicotyledonous herbs) (Table A1). Direct counting was feasible for buds on rhizomes and roots, whereas shoot bases needed to be dissected for tiller bud and bulb bud counting. During this process, only turgid buds were considered for counting, excluding any necrotic or obviously dead tissues. All tasks should be completed within one week to prevent any deterioration of the samples. Meanwhile, aboveground shoots were counted for each species, and then sorted into perennial rhizomatous grasses (featuring tiller and rhizome buds), perennial bunchgrasses (featuring tiller buds), and perennial forbs (featuring bulb and dicot buds).
The ANPP in each experimental plot was also estimated by harvesting all aboveground biomass at its growth peak in the middle of August 2022 by mowing all plant species to soil surface within a 1 m2 square quadrat each plot. The samples were put in a drying oven at 60°C for 48 h and weighted (0.1 g in accuracy).
Prior to conducting statistical analysis, the quantity of belowground buds and aboveground shoots within per quadrat were used to calculate the densities of buds and shoots, respectively, expressed on a per square meter basis. In this study, annual species (their relative abundance in most quadrats <10%, and they constituted less than 5% of total aboveground biomass) were excluded from the analyses. We mainly focused on perennial species, which were classified into three functional groups: perennial rhizomatous grasses, perennial bunchgrasses, and perennial forb. We also calculated the ratio of bud to shoot density (i.e., meristem limitation index-MLI) to estimate the degree to which aboveground population regeneration was constrained by belowground bud bank [38].
Levene’s tests for heteroscedasticity and Shapiro–Wilk tests for normality were performed on all data before statistical analyses. The confirmation of variance homogeneity and normal distribution allowed for the use of untransformed data in our statistical analyses. To examine the interactive effects of nutrient addition treatments on belowground bud density, aboveground shoot density, and MLI for the whole community and three plant functional groups, we used Generalized Linear Mixed Effects Models with N addition and P addition as fixed factors and block as a random factor. We compared the bud density, shoot density, and MLI across four treatment using analysis of variance (ANOVA), followed by Tukey Test for subsequent multiple comparisons within different functional groups and the whole plant community. We further performed regression analysis to explore the relationship between the belowground bud bank and aboveground vegetation. All analyses and figures were carried out using R 4.2.1 software (R Core Team, 2022).
3.1 Response of Whole Plant Community to Short-Term Nutrient Addition
For the whole plant community, there were no N × P interaction on total bud density, shoot density and MLI (Table 1). Both N addition and P addition and their combination had no significant influences on the bud density, shoot density and MLI of the whole plant community (Fig. 1).
Figure 1: The effects of N addition, P addition, and their combined treatments on belowground bud density, aboveground shoot density, and the ratio of bud to shoot density (i.e., meristem limitation index-MLI) for the whole plant community. Values are shown as means (n = 10) and standard error (SE). Different capital and lowercase letters indicate significant differences (p < 0.05) in total bud density and total shoot density among treatments, respectively, and different lowercase alpha letters indicate significant differences (p < 0.05) in MLI among treatments
3.2 Response of Different Plant Groups to Short-Term Nutrient Addition
As regard to belowground bud bank composition, we found specific responses in bud density, shoot density and MLI of three plant functional groups to soil nutrient addition. N addition significantly increased the bud density and shoot density of PR (bud density: p < 0.001, shoot density: p < 0.01, Table 1, Figs. 2A and 2B), especially with combination of P addition, even though P addition had no significant influences (p > 0.05, Table 1). N addition, P addition and their combination had no significant effects on the MLI of PR (p > 0.05, Table 1, Fig. 2C). Conversely, N addition significantly reduced shoot density of PF (p < 0.01, Table 1, Fig. 2H), particularly combined with P addition, although P addition alone did not have any significant effects (p > 0.05, Table 1), while the soil fertilization treatment had no significant influences on their bud bank and MLI (p > 0.05, Table 1, Figs. 2G and 2I). In addition, N addition, P addition, and their interactions had no significant influences on the bud density, shoot density, and MLI of PB (p > 0.05, Table 1, Figs. 2D–2F).
Figure 2: The effects of N addition, P addition, and their combined treatments on belowground bud density, aboveground shoot density, and the ratio of bud to shoot density (i.e., meristem limitation index-MLI) for different plant functional groups (perennial rhizomatous grasses-PR, perennial bunchgrasses-PB, and perennial forb-PF). Values are shown as means (n = 10) and standard error (SE). Different lowercase letters indicate significant differences (p < 0.05) in bud density, shoot density, and the MLI among treatments
3.3 Correlations between Belowground Bud Bank and Aboveground Vegetation
Considering all nurtient treatments together, we found the significantly linear relationship between belowground bud density and aboveground shoot density (R2 = 0.39, p < 0.01, Fig. 3A). However, no clear relationships were observed between aboveground biomass and either bud density or shoot density. (Figs. 3B and 3C).
Figure 3: The relationship between belowground bud bank and aboveground vegetation (shoot density (A) and aboveground biomass (B)) and the relationship between aboveground shoot density and aboveground biomass (C) in meadow steppe
4.1 Response of Belowground Bud Bank and Its Relationship with Aboveground Vegetation to Nutrient Addition
Under soil nutrient addition, the enhanced grassland productivity could arise from the increased tiller production, as observed in two bunchgrasses [30], or from larger tiller size, as documented in the rhizomatous grass Panicum virgatum [39]. In this study, although a positive correlation was observed between belowground bud density and aboveground shoot density (Fig. 3A), the increased aboveground biomass following nutrient addition might be attributed to the enlargement of individual aboveground shoot size (Table A2, Fig. A1). This could be inferred by the lack of significant relationship between aboveground biomass and shoot density (Fig. 3B), along with no significant influences of nutrient addition on aboveground shoot density (Table 1). Previous studies showed that nutrient addition may modify biomass allocation pattern of plant species [40], resulting in a higher proportion of biomass being allocated to aboveground organs relative to belowground parts [41]. These results suggest that the enhancement of aboveground biomass under short-term nutrient addition may be driven more by enlarging individual size than by increasing shoot abundance.
In the temperate semiarid steppe with both N and P limitation [42], soil nutrient addition to some extent could alleviate soil nutrient limitation. Under these conditions, plant species tend to reduce the biomass allocation to belowground for nutrient competition, while increasing resource allocation to aboveground components to compete for light and space [43]. The reduced biomass allocation to belowground constrains the resource supply for bud production, thus nutrient addition has negative influences on belowground bud density [44]. However, our result showed that belowground bud bank and its relationship with aboveground vegetation have no significant responses to short-term soil nutrient addition. This contrasts with our previous findings in temperate typical steppe, where a decrease in belowground bud density was observed under long-term (7 years) N addition [31]. This might be attributed to the fact that the resources stored in the belowground bud-bearing organs [24,25] could alleviate the instantaneous pressure of reducing belowground input under short-term soil nutrient addition, and thus the aboveground population regeneration/recruitment originated from belowground bud bank remains unaffected. Thus, we infer that a response threshold may exist for the belowground bud bank to nutrient addition or other environmental changes (e.g., drought) [34,45,46]. Short-term nutrient addition does not exceed the buffering capacity of bud banks, resulting in no response in total bud density. Besides, P addition was also found to have no significant impact on aboveground vegetation (i.e., ANPP [24]) and consequently may not change the distribution of biomass between aboveground and belowground, resulting in the constraint imposed by the belowground bud bank on aboveground population regeneration remains unaffected. Meanwhile, the response of belowground bud density may be attributed to the characteristic of P element, with low diffusion and high fixation in soil [47]. The added P might initially be adsorbed onto the surface of clay minerals and Fe/Al oxides through the formation of various complexes, and thereby become unavailable to plants [48]. Therefore, belowground bud bank had no significant response to short-term P addition. In addition, the lack of interaction between N addition and P addition on bud density indicates that N and P might independently influence the belowground bud bank and its potential regenerative contribution in semi-arid meadow steppe.
Nutrient addition did not change MLI for the whole plant community (Table 1), and the MLI kept constant under both N addition and P addition (Fig. 1). This implies that short-term soil nutrient addition also does not modify the relationship between belowground bud bank and aboveground shoot population. In simpler terms, the constraint imposed by the belowground bud bank on aboveground population regeneration/recruitment does not seem to be influenced by short-term nutrient addition.
4.2 Divergent Responses of Different Plant Functional Groups to Nutrient Addition
The responses of various bud types to environmental changes would significantly influence the relative dominance of plant functional groups, thereby reshaping the composition and structure of plant communities under global changes [29,31,34]. Our results demonstrate that PF and PR showed contrasting responses to the short-term soil nutrient addition, i.e., N addition (itself as well as combined with P addition) increased the bud density and shoot density of PR but decreased the shoot density of PF. The varying responses between PR and PF might be ascribed to their differing physiological characteristics. Under nutrient addition, the increasing NH4+ could to a greater extent suppress the enzymes activity and reduce the photosynthetic rate of PF in compared with PR [37], resulting in the reduced resource allocation to belowground for bud production and thus affecting the population regeneration of PF. Compared with PF, PR usually elongated rhizomes and increased rhizome bud density to occupy more growth space [40]. Previous studies have confirmed that under N addition, rhizomatous grasses could allocate more photoassimilates to belowground organs (i.e., rhizomes) to produce more rhizome buds for space occupation and population colonization [36,37]. These changes may significantly increase the belowground bud bank and facilitate the population regeneration/recruitment of PR. Therefore, our study suggests that nutrient addition has positive effects on rhizomatous grasses both for belowground bud bank and aboveground vegetation, while its negative impacts on forbs mainly act on their aboveground shoot population. The addition of N, P, and their interaction all have no significantly influences on the bud density, shoot density, and MLI of PB, suggesting that both the belowground bud bank and its regenerative capacity of this functional group were insensitive to short-term nutrient addition. This insensitivity of both whole plant community and PB might be attributed to grassland soil nutrient context and fertilization experimental duration [36], thus different grassland types and experimental durations should be incorporated in future studies to explore the potential responses of belowground bud density and composition as well as its regenerative contribution to nutrient addition.
To our knowledge, this study is the first to explore the responses of the belowground bud bank and its relationship with aboveground vegetation to multiple nutrient additions in the meadow steppe. Our results showed short-term soil nutrient addition has no influence on the belowground bud bank, aboveground shoot population, and their relationships with the whole plant community, which implies the belowground bud bank and its relationship with aboveground vegetation seem to be insensitive to short-term soil nutrient addition in temperate meadow steppe. Meanwhile, different plant functional groups’ bud banks exhibited different responses to short-term soil nutrient addition, which highlights the specific sensitivity of population regeneration in different functional groups to nutrient addition. Therefore, the belowground bud bank demographics, including bud bank size and composition, might be used to clarify and forecast the changes in composition and structure of plant community under global environment changes, which inevitably influence the functions and stability of grassland ecosystems.
Acknowledgement: We thank Jungang Chen and Xiaoyan Wang for their assistance during the field investigation and sampling. We are also very grateful for the field assistance of Eco-Animal Husbandry Experimental Zone and the State Key Laboratory of Vegetation and Environmental Change, Institute of Botany, Chinese Academy of Sciences.
Funding Statement: This research received financial support from the National Natural Science Foundation of China (41877542).
Author Contributions: The contribution of the authors to the paper is as follows: Jianqiang Qian and Jungang Chen were responsible for the study’s conception and design; data collection was conducted by Jin Tao, Jiatai Tian, Dongmei Li and Zhiming Zhang; Jin Tao and Jinlei Zhu analyzed and interpreted the results; the draft manuscript was prepared by Jin Tao, Qun Ma and Jianqiang Qian. All authors reviewed the results and approved the final version of the manuscript.
Availability of Data and Materials: Readers can access the data used in this study by contacting corresponding author.
Ethics Approval: Not applicable.
Conflicts of Interest: The authors declare that they have no conflicts of interest to report regarding the present study.
References
1. Smith MD, Knapp AK, Collins SL. A framework for assessing ecosystem dynamics in response to chronic resource alterations induced by global change. Ecology. 2009;90(12):3279–89. doi:10.1890/08-1815.1. [Google Scholar] [PubMed] [CrossRef]
2. Peñuelas J, Poulter B, Sardans J, Ciais P, van der Velde M, Bopp L, et al. Human-induced nitrogen-phosphorus imbalances alter natural and managed ecosystems across the globe. Nat Commun. 2013;4(1):2934. doi:10.1038/ncomms3934. [Google Scholar] [PubMed] [CrossRef]
3. Tilman D, Fargione J, Wolff B, D’Antonio C, Dobson A, Howarth R, et al. Forecasting agriculturally driven global environmental change. Science. 2001;292(5515):281–4. doi:10.1126/science.1057544. [Google Scholar] [PubMed] [CrossRef]
4. Hautier Y, Zhang P, Loreau M, Wilcox KR, Seabloom EW, Borer ET, et al. General destabilizing effects of eutrophication on grassland productivity at multiple spatial scales. Nat Commun. 2020;11(1):5375. doi:10.1038/s41467-020-19252-4. [Google Scholar] [PubMed] [CrossRef]
5. Clark CM, Cleland EE, Collins SL, Fargione JE, Gough L, Gross KL, et al. Environmental and plant community determinants of species loss following nitrogen enrichment. Ecol Lett. 2007;10(7):596–607. doi:10.1111/j.1461-0248.2007.01053.x. [Google Scholar] [PubMed] [CrossRef]
6. Harpole WS, Ngai JT, Cleland EE, Seabloom EW, Borer ET, Bracken MES, et al. Nutrient co-limitation of primary producer communities. Ecol Lett. 2011;14(9):852–62. [Google Scholar] [PubMed]
7. Isbell F, Reich PB, Tilman D, Hobbie SE, Polasky S, Binder S. Nutrient enrichment, biodiversity loss, and consequent declines in ecosystem productivity. Proc Natl Acad Sci U S A. 2013;110(29):11911–6. [Google Scholar] [PubMed]
8. Avolio ML, Koerner SE, La Pierre KJ, Wilcox KR, Wilson GWT, Smith MD, et al. Changes in plant community composition, not diversity, during a decade of nitrogen and phosphorus additions drive above-ground productivity in a tallgrass prairie. J Ecol. 2014;102(6):1649–60. [Google Scholar]
9. Fang J, Geng X, Zhao X, Shen H, Hu H. How many areas of grasslands are there in China? Chin Sci Bull. 2018;63(17):1731–9 (In Chinese). [Google Scholar]
10. Galloway JN, Dentener FJ, Capone DG, Boyer EW, Howarth RW, Seitzinger SP, et al. Nitrogen cycles: past, present, and future. Biogeochemistry. 2004;70(2):153–226. [Google Scholar]
11. Parmesan C, Morecroft MD, Trisurat Y, Adrian R, Anshari GZ, Arneth A, et al. Terrestrial and freshwater ecosystems and their services. In: Pörtner HO, Roberts DC, Tignor M, Poloczanska ES, Mintenbeck K, Alegría A, et al., editors. Climate change 2022: impacts, adaptation and vulnerability. Cambridge: Cambridge University Press; 2022. p. 197–377. [Google Scholar]
12. Isbell F, Calcagno V, Hector A, Connolly J, Harpole WS, Reich PB, et al. High plant diversity is needed to maintain ecosystem services. Nature. 2011;477(7363):199–202. doi:10.1038/nature10282. [Google Scholar] [PubMed] [CrossRef]
13. Zhang Y, Feng J, Loreau M, He N, Han X, Jiang L. Nitrogen addition does not reduce the role of spatial asynchrony in stabilising grassland communities. Ecol Lett. 2019;22(4):563–71. doi:10.1111/ele.13212. [Google Scholar] [PubMed] [CrossRef]
14. Baer SG, Blair JM, Collins SL, Knapp AK. Soil resources regulate productivity and diversity in newly established tallgrass prairie. Ecology. 2003;84(3):724–35. doi:10.1890/0012-9658(2003)084[0724:SRRPAD]2.0.CO;2. [Google Scholar] [CrossRef]
15. Collins SL, Sinsabaugh RL, Crenshaw C, Green L, Porras-Alfaro A, Stursova M, et al. Pulse dynamics and microbial processes in aridland ecosystems. J Ecol. 2008;96(3):413–20. doi:10.1111/j.1365-2745.2008.01362.x. [Google Scholar] [CrossRef]
16. Bai Y, Wu J, Clark CM, Naeem S, Pan Q, Huang J, et al. Tradeoffs and thresholds in the effects of nitrogen addition on biodiversity and ecosystem functioning: evidence from Inner Mongolia Grasslands. Global Change Biol. 2010;16(1):358–72. doi:10.1111/j.1365-2486.2009.01950.x. [Google Scholar] [CrossRef]
17. Stevens CJ, Duprè C, Dorland E, Gaudnik C, Gowing DJG, Bleeker A, et al. Nitrogen deposition threatens species richness of grasslands across Europe. Environ Pollut. 2010;158(9):2940–5. doi:10.1016/j.envpol.2010.06.006. [Google Scholar] [PubMed] [CrossRef]
18. Seabloom EW, Adler PB, Alberti J, Biederman L, Buckley YM, Cadotte MW, et al. Increasing effects of chronic nutrient enrichment on plant diversity loss and ecosystem productivity over time. Ecology. 2021;102(2):e03218. doi:10.1002/ecy.3218. [Google Scholar] [PubMed] [CrossRef]
19. Li Y, Niu S, Yu G. Aggravated phosphorus limitation on biomass production under increasing nitrogen loading: a meta-analysis. Global Change Biol. 2016;22(2):934–43. doi:10.1111/gcb.13125. [Google Scholar] [PubMed] [CrossRef]
20. Zhao Y, Yang B, Li M, Xiao R, Rao K, Wang J, et al. Community composition, structure and productivity in response to nitrogen and phosphorus additions in a temperate meadow. Sci Total Environ. 2019;654(7179):863–71. doi:10.1016/j.scitotenv.2018.11.155. [Google Scholar] [PubMed] [CrossRef]
21. Yu L, Song X, Zhao J, Wang H, Bai L, Yang D. Responses of plant diversity and primary productivity to nutrient addition in a Stipa baicalensis grassland, China. J Integr Agr. 2015;14(10):2099–108. doi:10.1016/S2095-3119(14)61001-7. [Google Scholar] [CrossRef]
22. Liu C, Liu Y, Guo K, Qiao X, Zhao H, Wang S, et al. Effects of nitrogen, phosphorus and potassium addition on the productivity of a karst grassland: plant functional group and community perspectives. Ecol Eng. 2018;117:84–95. [Google Scholar]
23. Ford H, Roberts A, Jones L. Nitrogen and phosphorus co-limitation and grazing moderate nitrogen impacts on plant growth and nutrient cycling in sand dune grassland. Sci Total Environ. 2016;542:203–9. [Google Scholar] [PubMed]
24. Guo Y, Zhou M, Sheng J, Yuan Y, Yuan G, Zhang WH, et al. Aboveground net primary productivity was not limited by phosphorus in a temperate typical steppe in Inner Mongolia. J Plant Ecol. 2023;16(4):rtac085. [Google Scholar]
25. Ott JP, Klimešová J, Hartnett DC. The ecology and significance of below-ground bud banks in plants. Ann Bot. 2019;123(7):1099–118. [Google Scholar] [PubMed]
26. Dalgleish HJ, Hartnett DC. Below-ground bud banks increase along a precipitation gradient of the North American Great Plains: a test of the meristem limitation hypothesis. New Phytol. 2006;171(1):81–9. [Google Scholar] [PubMed]
27. Dalgleish HJ, Hartnett DC. The effects of fire frequency and grazing on tallgrass prairie productivity and plant composition are mediated through bud bank demography. Plant Ecol. 2009;201(2):411–20. [Google Scholar]
28. Clarke PJ, Lawes MJ, Midgley JJ, Lamont BB, Ojeda F, Burrows GE, et al. Resprouting as a key functional trait: how buds, protection and resources drive persistence after fire. New Phytol. 2013;197(1):19–35. doi:10.1111/nph.12001. [Google Scholar] [PubMed] [CrossRef]
29. Qian J, Guo Z, Muraina TO, Te N, Griffin-Nolan RJ, Song L, et al. Legacy effects of a multi-year extreme drought on belowground bud banks in rhizomatous vs bunchgrass-, dominated grasslands. Oecologia. 2022;198(3):763–71. doi:10.1007/s00442-022-05133-8. [Google Scholar] [PubMed] [CrossRef]
30. Dalgleish HJ, Kula AR, Hartnett DC, Sandercock BK. Responses of two bunchgrasses to nitrogen addition in tallgrass prairie: the role of bud bank demography. Am J Bot. 2008;95(6):672–80. doi:10.3732/ajb.2007277. [Google Scholar] [PubMed] [CrossRef]
31. Qian J, Wang Z, Klimešová J, Lü X, Zhang C. Belowground bud bank and its relationship with aboveground vegetation under watering and nitrogen addition in temperate semiarid steppe. Ecol Indic. 2021;125(7005):107520. doi:10.1016/j.ecolind.2021.107520. [Google Scholar] [CrossRef]
32. Hendrickson JR, Briske DD. Axillary bud banks of two semiarid perennial grasses: occurrence, longevity, and contribution to population persistence. Oecologia. 1997;110(4):584–91. doi:10.1007/s004420050199. [Google Scholar] [PubMed] [CrossRef]
33. Qian J, Wang Z, Klimešová J, Lü X, Kuang W, Liu Z, et al. Differences in below-ground bud bank density and composition along a climatic gradient in the temperate steppe of Northern China. Am J Bot. 2017;120(5):755–64. doi:10.1093/aob/mcx072. [Google Scholar] [PubMed] [CrossRef]
34. Qian J, Zhang Z, Dong Y, Ma Q, Yu Q, Zhu J, et al. Responses of bud banks and shoot density to experimental drought along an aridity gradient in temperate grasslands. Funct Ecol. 2023;37(5):1211–20. doi:10.1111/1365-2435.14301. [Google Scholar] [CrossRef]
35. Klimeš L, Klimešová J. Root sprouting in Rumex acetosella under different nutrient levels. Plant Ecol. 1999;141(1/2):33–9. doi:10.1023/A:1009877923773. [Google Scholar] [CrossRef]
36. Zheng Z, Bai W, Zhang WH. Clonality-dependent dynamic change of plant community in temperate grasslands under nitrogen enrichment. Oecologia. 2019;189(1):255–66. doi:10.1007/s00442-018-4317-x. [Google Scholar] [PubMed] [CrossRef]
37. Tian Q, Yang L, Ma P, Zhou H, Liu N, Bai W, et al. Below-ground-mediated and phase-dependent processes drive nitrogen-evoked community changes in grasslands. J Ecol. 2020;108(5):1874–87. doi:10.1111/1365-2745.13415. [Google Scholar] [CrossRef]
38. Benson EJ, Hartnett DC, Mann KH. Belowground bud banks and meristem limitation in tallgrass prairieplant populations. Am J Bot. 2004;91(3):416–21. [Google Scholar] [PubMed]
39. Hartnett DC. Regulation of clonal growth and dynamics of Panicum virgatum (Poaceae) in tallgrass prairie: effects of neighbor removal and nutrient addition. Am J Bot. 1993;80(10):1114–20. [Google Scholar]
40. Bai W, Sun X, Wang Z, Li L. Nitrogen addition and rhizome severing modify clonal growth and reproductive modes of Leymus chinensis population. Plant Ecol. 2009;205(1):13–21. [Google Scholar]
41. Feng H, Guo J, Peng C, Kneeshaw D, Roberge G, Pan C, et al. Nitrogen addition promotes terrestrial plants to allocate more biomass to aboveground organs: a global meta-analysis. Global Change Biol. 2023;29(14):3970–89. [Google Scholar]
42. Bai X, Cheng H, Zheng S, Zhan S, Bai Y. Ecophysiological responses of Leymus chinensis to nitrogen and phosphorus additions in a typical steppe. Chin J Plant Ecol. 2014;38(2):103–15. [Google Scholar]
43. Hautier Y, Niklaus PA, Hector A. Competition for light causes plant biodiversity loss after eutrophication. Science. 2009;324(5927):636–8. [Google Scholar] [PubMed]
44. Wang J, Gao Y, Zhang Y, Yang J, Smith MD, Knapp AK, et al. Asymmetry in above- and belowground productivity responses to N addition in a semi-arid temperate steppe. Glob Change Biol. 2019;25(9):2958–69. [Google Scholar]
45. VanderWeide BL, Hartnett DC. Belowground bud bank response to grazing under severe, short-term drought. Oecologia. 2015;178(3):795–806. [Google Scholar] [PubMed]
46. VanderWeide BL, Hartnett DC, Carter DL. Belowground bud banks of tallgrass prairie are insensitive to multi-year, growing-season drought. Ecosphere. 2014;5(8):1–17. [Google Scholar]
47. Shen J, Yuan L, Zhang J, Li H, Bai Z, Chen X, et al. Phosphorus dynamics: from soil to plant. Plant Physiol. 2011;156(3):997–1005. [Google Scholar] [PubMed]
48. Arai Y, Sparks DL. Phosphate reaction dynamics in soils and soil minerals: a multiscale approach. Adv Agron. 2007;94:135–79. doi:10.1016/S0065-2113(06)94003-6. [Google Scholar] [CrossRef]
Appendix
Additional supporting information can be found online in the Supporting Information section at the end of this article.
Figure A1: The effects of N addition, P addition, and their combined treatments on aboveground biomass. Values are shown as means (n = 10) and standard error (SE). Different capital and lowercase letters indicate significant differences (p < 0.05) among treatments
Cite This Article
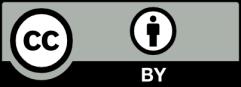
This work is licensed under a Creative Commons Attribution 4.0 International License , which permits unrestricted use, distribution, and reproduction in any medium, provided the original work is properly cited.