Open Access
ARTICLE
Physiological Response Mechanism and Drought Resistance Evaluation of Passiflora edulis Sims under Drought Stress
1 College of Forestry, Guizhou University, Guiyang, 550025, China
2 Guizhou Institute of Biology, Guizhou Academy of Sciences, Guiyang, 550009, China
3 College of Resources and Environment, Hunan Agricultural University, Changsha, 410128, China
* Corresponding Author: Anding Li. Email:
(This article belongs to the Special Issue: Abiotic Stress Impacts on Plant Physiology and Their Alleviation)
Phyton-International Journal of Experimental Botany 2024, 93(6), 1345-1363. https://doi.org/10.32604/phyton.2024.050950
Received 26 February 2024; Accepted 21 May 2024; Issue published 27 June 2024
Abstract
In order to explore the response mechanism of Passiflora edulis Sims to drought stress, the changes in morphological and physiological traits of Passiflora edulis Sims under different drought conditions were studied. A total of 7 germplasm resources of Passiflora edulis Sims were selected and tested under drought stress by the pot culture method under 4 treatment levels: 75%–80% (Control, CK) of maximum field water capacity, 55%–60% (Light Drought, LD) of maximum field water capacity, i.e., mild drought, 40%–45% (Moderate Drought, MD) of maximum field water capacity, i.e., moderate drought and 30%–35% (Severe Drought, SD) of maximum field water capacity, i.e., severe drought. On the 40th day of drought treatment, 13 indices, including seedling growth morphology, physiology, and biochemistry, were measured. The results showed that under drought stress, the height and ground diameter of P. edulis Sims gradually decreased with increasing drought stress, and there were significant differences in seedling height and ground diameter among the treatments. Drought stress significantly inhibited the growth of seven P. edulis Sims varieties. The contents of soluble sugar (SS), soluble protein (SP), proline (Pro), and other substances in P. edulis Sims basically increased with increasing drought stress. With the aggravation of drought stress, the malondialdehyde (MDA) content of P. edulis Sims tended to increase to different degrees, the superoxide dismutase (SOD) activity and peroxidase (POD) activity both tended to increase at first and then decrease, and the change in catalase (CAT) activity mostly showed a gradual increasing trend. The contents of endogenous hormones in P. edulis Sims significantly differed under different degrees of drought stress. With the aggravation of drought stress, the abscisic acid (ABA) content of P. edulis Sims tended to increase, whereas the contents of gibberellin (GA), indoleacetic acid (IAA), and zeatin nucleoside (ZR) exhibited a downward trend. A comprehensive evaluation of the drought resistance of seven P. edulis Sims varieties was conducted based on the principal component analysis method, and the results showed that the drought resistance decreased in the order XH-BL > XH-TWZ > TN1 > GH1 > ZJ-MT > LP-LZ > DH-JW.Keywords
Passiflora edulis Sims, also known as Passiflora edulis, is a perennial, often grassy or semi-woody vine climbing plant of Passiflora of Passifloraceae [1,2]. It is distributed mainly in warm temperate and tropical regions of North America, southeastern Asia, India, Myanmar, and Australia. Many species of the Passiflora genus, due to their edible value, efficacy, and ornamental characteristics, are widely cultivated [3–5]. At the beginning of the 20th century, P. edulis Sims was introduced into China as an edible fruit and is distributed mainly in Taiwan, Guangdong, Guangxi, and, Fujian Province, and the planting area has exceeded 600 thousand mu in recent years [6,7]. Drought is one of the most important agrometeorological disasters in China and is also a global problem that restricts the development of agroforestry [8,9]. Due to the decrease in regional precipitation and increase in evapotranspiration caused by global warming, the frequency and severity of drought events are expected to continue to increase in the near future [10–13]. Drought induces water stress in plants, adversely affecting their growth, photosynthesis, stomatal activity, and respiration [14–17]. Additionally, water stress impacts nutrient metabolism, hormonal regulation, and infiltration in plants, which can further hinder their growth and development, ultimately leading to plant death [18,19]. Due to the shallow soil layer, low water-holding capacity, and strong permeability of rocks in typical karst habitats, there is widespread stress from frequent alternating dry and wet conditions, which has had strong negative impacts on the development of the P. edulis Sims industry [20]. Especially in karst habitats, due to the high rock exposure rate, the soil is discontinuous, and the topsoil is shallow, resulting in strong soil permeability; additionally, water shortages are one of the main characteristics of karst habitats and constitute a kind of temporary soil drought phenomenon in humid climates [21,22]. More than 70% of the cultivated land in Guizhou is threatened by drought. The rainfall in the summer half of the year accounts for more than 80% of the total annual rainfall and is mainly concentrated from May to August. Therefore, drought occurs mainly in spring and summer [23,24]. Studies have shown that P. edulis Sims is a good resource for rocky desertification control and industrial development [25]. Current research on the drought response mechanisms of P. edulis Sims under simulated karst climate conditions remains exceedingly limited. Systematic comparative studies on the growth changes, protective enzyme activities, osmoregulatory substance contents, and endogenous hormone levels among different varieties of P. edulis Sims under varying levels of drought stress in karst regions have yet to be reported. Understanding these variations is crucial for revealing the physiological mechanisms through which P. edulis Sims counteracts drought stress in karst areas. Moreover, it holds significant importance in providing scientific guidance for the cultivation of P. edulis Sims in these regions.
The expansion of arid lands globally, driven by climate change, has garnered widespread attention. Rising temperatures and shifting precipitation patterns are enlarging arid and semi-arid regions, posing significant challenges to agricultural production, water resource management, and ecosystem stability [26,27]. Changes in plant growth morphology under drought stress visually represent the ecological response of plants to water changes. Changes in cell membrane permeability and osmoregulatory substances and trends in the changes of many plants under water stress have been studied, and many studies have shown that the malondialdehyde, proline, soluble sugar, and soluble protein contents in plants are strongly correlated with the degree of water stress [28,29]. Under drought conditions, plants produce many active oxygen free radicals in a variety of ways, including O2−, H2O2, O2, and OH−, and many studies have investigated the response mechanism of protective enzyme systems when plants are under water stress. For example, superoxide dismutase (SOD) is the first line of defense for removing oxygen free radicals and plays a key role in enzyme systems. SOD can catalyze the disproportionation of oxygen free radicals, ultimately forming harmless molecular oxygen and water after a series of reactions [30,31]. Plant endogenous hormones are also important signaling substances for drought stress. Endogenous hormone levels exhibit different trends under drought stress. Plant endogenous hormones participate in the whole process of adaptation to stress, including hormone synthesis, transport, and signal control. Moreover, different endogenous hormones have different effects under stress [32–34]. Drought-tolerant genotypes play a crucial role in enabling plants to withstand drought stress. Such plants exhibit resilience through the overexpression of specific dehydration-responsive genes like cdDHN4 and antioxidant genes like Cu/ZnSOD. They adeptly regulate stomatal conductance, ensuring the maintenance of high osmotic water potential and optimizing water use efficiency via osmotic regulators. Furthermore, these genotypes foster elongation in the mesophyll, palisade, and spongy cells, contributing to the preservation of vital morphological and physiological functions even under severe drought conditions [35].
This study aims to investigate the physiological response mechanisms to drought conditions of different P. edulis Sims varieties in the karst habitats of Guizhou. In this study, seven P. edulis Sims varieties were subjected to a pot-simulated drought test to determine the changes in growth, protective enzyme activity, and osmoregulatory substance content as well as in the endogenous hormone content of the passionfruit under drought stress. The drought resistance physiological mechanism of different varieties of passionfruit was explored, the drought resistance of each variety was comprehensively evaluated via mathematical methods, and varieties with strong drought resistance were screened out, which will provide basic theoretical support for variety breeding and reasonable cultivation in the development of the P. edulis Sims industry.
2.1 Experimental Site Overview
The study commenced in April 2020 at the Guizhou Passion Fruit Quality Seedling Breeding Center, located in Kedu Town, Pingtang County, Qiannan Prefecture, Guizhou Province (E: 106°50′32″∼107°11′47′′, N: 25°38′19′′∼25°59′9′′). Situated in Southern Guizhou, this region is part of a middle subtropical karst landscape, with an average elevation of 708 m. It features a middle subtropical monsoon climate, marked by mild winters and cool summers, optimal sunlight, abundant heat, a long frost-free period, an average annual temperature of 16.7°C, and an annual average precipitation of 1217 mm.
Seven P. edulis Sims varieties were selected for seedling culture in the experiment, and the specific varieties tested are shown in Table 1. Seeds with full grains that were free of disease and insect pests were selected, soaked in clean water for 24 h, disinfected with 0.2% potassium permanganate, and subsequently sown in a seedling-raising tray (The seedling substrate was composed of a mixture of coco peat, carbonized rice husk, peat moss, perlite, and vermiculite in a volumetric ratio of 1:1.5:1:0.5:1, respectively. The physicochemical properties of the substrate were as follows: total porosity of 86.56%, bulk density of 0.35 g/cm³, organic matter content of 26.78%, and a pH value of 6.1) [36]. Approximately 30 days after sowing, when one to two true leaves appeared in the tray, the plants were moved to a nutrition cup with a diameter of 9 cm and unified management was carried out. The seedlings were grown to approximately 60 cm in length and subsequently subjected to drought stress treatment.
Our experiment utilized a Completely Randomized Design (CRD) to assess the changes in growth and physiological parameters of P. edulis Sims under varying levels of drought stress. In June 2020, the seedlings were grown to approximately 60 cm, Passiflora edulis typically transitions from the juvenile to a more mature phase, where physiological processes such as photosynthesis and nutrient uptake are highly active and thus sensitive to environmental stresses. Therefore, applying drought stress during this period results in particularly significant effects. Rhizosphere water stress was used mainly for drought treatment, and a pot experiment was conducted using the water control method. The plant water gradient division method was used to establish four treatment levels by Koide et al: the control was 75%–80% (CK) of the maximum field water capacity, the mild drought was 55%–60% (LD), the moderate drought was 40%–45% (MD), and the severe drought was 30%–35% (SD) [37]. Seven varieties of P. edulis Sims seedlings under the same management conditions were selected as the experimental materials. There were 36 pots per gradient, and one plant was planted per pot. The method and operation for maintaining the corresponding field capacity during irrigation are based on the experimental design by Chen [38]. The pot has a top diameter of 22 cm, a bottom diameter of 18 cm, and a height of 15 cm. The soil used in the pot is topsoil from the experimental site, with each pot containing 15 kg of soil. The pots are placed outdoors and are sheltered by a rain cover.
On the 40th day of drought treatment, P. edulis Sims begins to branch, with the main focus being on the growth of the plant’s main stem. Measuring the plant’s physiological responses at this time can provide insights into the overall growth condition of the plant. Therefore, the drought stress is terminated on day 40 to begin sampling and testing. Sampling is conducted between 9 and 11 a.m., a timeframe that minimizes the impact of diurnal variations on plant physiological activity, thus ensuring more accurate and consistent results. Thirty seedlings were randomly selected from each treatment for the growth morphology indices to measure seedling height and ground diameter. When the physiological and biochemical indices were measured, three seedlings were mixed as a sample for one treatment, and each index sample was measured three times. After the samples were quickly washed with distilled water, they were put into a self-sealing bag, sealed, and immediately put into liquid nitrogen for storage.
The activities and levels of superoxide dismutase (SOD), peroxidase (POD), catalase (CAT), malondialdehyde (MDA), proline (Pro), soluble sugar (SS), soluble protein (SP), gibberellin (GA), zeatin riboside (ZR), indoleacetic acid (IAA), and abscisic acid (ABA) were all determined and calculated according to the instructions provided with the Solarbio reagent kits. The specific product codes are as follows: SOD (BC5160), POD (BC0095), CAT (BC0205), MDA (BC0025), Pro (BC0290), SS (BC0035), SP (BC3720), GA (G8040), ZR (Z8040), IAA (I8020), and ABA (A8060). These reagents are supplied by Solarbio Science & Technology Co., Ltd., located in Beijing, China.
One-way analysis of variance (ANOVA) (Duncan’s method, p < 0.05) was performed using WPS Office 2019 and SPSS 23.0 software. The data in the graphs are presented as the mean and standard deviation. The data analysis section using Origin 2021 was conducted as follows: we generated a hierarchical clustering heatmap using the average linkage method and Euclidean distance, and we created a heatmap of correlations using Pearson’s correlation coefficient. Additionally, we used Origin 2021 to produce the biplot of the PCA. This approach helps visualize the relationships and principal components effectively, providing insights into the dataset’s underlying structure.
3.1 Changes in P. edulis Sims Plant Growth under Drought Stress
As shown in Fig. 1, the seedling height and ground diameter of each variety of P. edulis Sims gradually decreased under increasing drought stress, and the seedling height and ground diameter among different treatments were significantly different (p < 0.05). XH-TWZ exhibited the best growth, with the smallest reductions in both plant height and ground diameter under drought stress. Under severe and moderate stress conditions, the height of P. edulis Sims was significantly lower than that of the control group (p < 0.05). Except for ZJ-MT and LP-LZ, the height of other P. edulis Sims varieties showed no significant difference from the control group under mild drought stress. Under severe stress, the seedling height of DH-JW was only 23.38% of the control, marking the greatest reduction. Across all levels of drought stress—mild, moderate, and severe—all P. edulis Sims varieties exhibited significantly lower ground diameters compared to the control group (p < 0.05). Among them, ZJ-MT performed the worst, with its ground diameter under severe drought stress being only 63.17% of that of the control group.
Figure 1: Growth parameters including Height (A) and Diameter (B) of Passiflora edulis Sims subjected to various irrigation regimes: 75%–80% (Control, CK), 55%–60% (Light Drought, LD), 40%–45% (Moderate Drought, MD), and 30%–35% (Severe Drought, SD) of maximum field water capacity. Data are presented as means ± SE (n = 3); different letters within each breed signify statistically significant differences as determined by Duncan’s test (p < 0.05)
3.2 Changes in Osmoregulatory Substances in P. edulis Sims under Drought Stress
Under drought stress, the contents of soluble sugars, soluble proteins, proline, and other substances in P. edulis Sims basically increased with increasing drought stress. As shown in Fig. 2, the soluble sugar content of P. edulis Sims under severe drought stress significantly increased and was greater than that of the control treatment (p < 0.05), and the increase was most significant in TN1, which exhibited a value 2.26 times greater than that in the control treatment. With the aggravation of drought stress, the soluble protein contents of all P. edulis Sims varieties exhibited an increasing trend, of which TN1 exhibited the greatest increase and DH-JW exhibited the smallest increase; moreover, compared with that in the control treatment, the soluble protein content of these varieties increased by 1.78 and 0.83 times, respectively, in the severe drought stress treatment. Except for ZJ-MT and GH1, which showed no significant differences in proline content compared to the control group under mild drought stress, all other treated P. edulis Sims varieties exhibited significantly higher proline levels than those in the control treatment. (p < 0.05). Compared with soluble sugar and soluble protein, the proline content of P. edulis Sims increased more significantly with increasing drought stress. Under severe drought stress, TN1 showed the greatest increase, and the proline content under severe drought stress increased by 11.41 times compared with that under the control treatment (Table A1). In summary, the synthesis of large amounts of proline under drought stress might be one of the main ways for P. edulis Sims to adapt to drought stress.
Figure 2: Variations in osmoregulatory substances (Soluble sugar (A), Soluble protein (B), Free proline (C)) in Passiflora edulis Sims under differing water availabilities: 75%–80% (CK), 55%–60% (LD), 40%–45% (MD), and 30%–35% (SD). Mean values are shown with standard errors (SE, n = 3); statistically significant differences are indicated by different letters within each breed following Duncan’s test (p < 0.05)
3.3 Changes in the Antioxidant Enzyme Activity and Malondialdehyde Content of P. edulis Sims under Drought Stress
When plants suffer from stress, MDA is produced in cells. As shown in Fig. 3, under drought stress, the MDA content of all P. edulis Sims varieties presented an increasing trend with increasing stress severity. The elevation in MDA content was most notable under moderate and severe drought stress conditions, where it significantly exceeded that of the control group (p < 0.05). XH-TWZ consistently had the highest MDA levels across all treatments and exhibited the greatest increase in MDA under drought stress, indicating its superior drought resistance. Antioxidative enzymes are important physiological adaptation mechanisms for plants to resist stress damage. With the increase in the degree of drought stress, the SOD of P. edulis Sims seedlings tended to increase first and then decrease, and the difference among the different drought treatments was significant. However, compared with the control treatment, SOD still showed an upward trend. Among the varieties, TN1 maintained the highest SOD activity at all levels of drought, showcasing good drought tolerance. Consistent with the change in SOD, the change in POD also increased first and then decreased with increasing stress severity, and the POD significantly differed among the different drought treatments. Except for DH-JW, which reached its peak under mild drought stress, the other varieties all reached their peak under moderate drought stress. The CAT of DH-JW and ZJ-MT under drought stress were not significantly different from those under the control treatment, while the CAT of several other P. edulis Sims varieties under different treatments were significantly different (p < 0.05). The CAT of P. edulis Sims basically increased with increasing drought stress. Under severe drought conditions, TN1 exhibited the greatest increase in CAT activity, reaching 2.07 times that of the control group.
Figure 3: Antioxidant enzyme activities (SOD (B), POD (C), CAT (D)) and malondialdehyde content (MDA (A)) in Passiflora edulis Sims across varying water conditions: 75%–80% (CK), 55%–60% (LD), 40%–45% (MD), and 30%–35% (SD). Presented data are means ± SE (n = 3), with significant differences indicated by differing letters within each breed according to Duncan’s test (p < 0.05)
3.4 Changes in the Endogenous Hormone Content of P. edulis Sims under Drought Stress
As shown in Fig. 4, the ABA content of P. edulis Sims varied significantly under the different drought stress treatments, it increased with the increase of drought stress. Except for DH-JW, the ABA levels in other P. edulis Sims exposed to moderate and severe stress treatments were significantly higher than those in the control treatment (p < 0.05). The ABA content in DH-JW plants first increased slowly but then decreased sharply under severe drought stress, while the ABA content in the remaining varieties basically increased. In both severe and moderate stress treatments, XH-TWZ and TN1 had the highest levels of ABA, and also exhibited the greatest increase relative to the control group, indicating that they possess strong drought resistance. With the increase in the degree of drought stress, the GA content of ZJ-MT exhibited an upward trend, whereas that of DH-JW and XH-BL exhibited a gradual decrease; however, there were no significant differences among the different drought stress treatments (p < 0.05). The GA content of the other varieties exhibited a downward trend. Among them, LP-LZ exhibited the largest decrease in GA levels. With the aggravation of drought stress, the IAA content and the ZR content of P. edulis Sims showed a downward trend. Under severe drought conditions, the reduction in IAA for P. edulis Sims was most pronounced, significantly lower than that of the control group (p < 0.05). There was no significant change in the ZR content under mild drought stress, but the decrease in the ZR under moderate and severe drought stress was particularly significant (p < 0.05).
Figure 4: Endogenous hormone levels (ABA (A), GA (B), IAA (C), ZR (D)) in Passiflora edulis Sims under four moisture regimes: 75%–80% (CK), 55%–60% (LD), 40%–45% (MD), and 30%–35% (SD). Data are means ± SE (n = 3), with differences marked by varying letters within each breed based on Duncan’s test (p < 0.05)
3.5 Correlation Analysis of Morphological and Physiological Traits and Comprehensive Evaluation of P. edulis Sims Drought Resistance
Under drought stress, there is a significant correlation between morphological and physiological traits in P. edulis Sims as shown in Fig. 5. Seedling height is significantly positively correlated with ZR (zeatin riboside) (r = 0.79**), IAA (indole-3-acetic acid) (r = 0.76**), and GA (gibberellic acid) (r = 0.49**). Ground diameter shows a similar trend, exhibiting significant positive correlations with ZR (r = 0.77**), IAA (r = 0.77**), and GA (r = 0.51**). Additionally, seedling height and ground diameter also display a significant positive correlation with each other (r = 0.87**). Both height and diameter are significantly negatively correlated with soluble sugars, soluble proteins, malondialdehyde, proline, catalase, and abscisic acid.
Figure 5: Correlations between morphological and physiological traits in Passiflora edulis Sims under drought conditions. Parameters include Height (H), Ground Diameter (D), and biochemical markers such as superoxide dismutase (SOD), peroxidase (POD), catalase (CAT), malondialdehyde (MDA), Proline (Pro), Soluble Sugar (SS), Soluble Protein (SP), Gibberellin (GA), Zeatin (ZR), Auxin (IAA), and Abscisic Acid (ABA). In this correlation analysis chart, the different shades of red and blue illustrate the strength and direction of correlations. Red indicates a positive correlation, while blue signifies a negative correlation. The intensity and size of the circles correspond to the magnitude of the correlation: larger and darker circles denote stronger correlations, whereas smaller and lighter circles indicate weaker correlations.Asterisks denote significance levels: *p < 0.05; **p < 0.01
Principal Component Analysis (PCA) can identify the characteristics of each group of varieties and ascertain the uniqueness of each experiment in terms of target component expression [39]. Principal component analysis was used to evaluate the comprehensive drought resistance of seven P. edulis Sims varieties and to screen out the best indicators. Principal component analysis was performed on the 13 individual indicators (Fig. 6). Two principal components with eigenvalues greater than 1 were extracted using PCA. The eigenvalues of the first and second principal components were 8.168 and 1.440, respectively. The first two PCs accounted for 73.9% of the total variance: PC1 accounted for 62.8%, and PC2 for 11.1%. The free proline, soluble protein, soluble sugar, ABA, MDA, and CAT were more prominent on the first principal component, while SOD and POD were more significant on the second principal component. The different drought stress treatment levels of P. edulis Sims were mainly distinguished on PC1; the control and mild drought stress were primarily positioned on the negative side, in proximity to the characteristic vectors of height, diameter, IAA, ZR, and GA, with the control group being closer. In contrast, moderate and severe drought stress was mainly on the positive side, nearer to the characteristic vectors of free proline, soluble protein, soluble sugar, ABA, MDA, and CAT, with the severe drought stress being closer.
Figure 6: PCA projections on axes 1 and 2 accounting for 73.9% of total variance. Eigenvalues of the correlation matrix are symbolized as vectors representing traits that most influence each axis. The 28 points representing trait means for each drought stress treatment (Control (CK), mild drought (LD), moderate drought (MD), and severe drought (SD)) are plotted on the plane determined by axes 1 and 2. Height (H), ground diameter (D), superoxide dismutase (SOD), peroxidase (POD), catalase (CAT), malondialdehyde (MDA), proline (Pro), soluble sugar (SS), soluble protein (SP), gibberellin (GA), zeatin nucleoside (ZR), auxin (IAA), and abscisic acid (ABA)
Using the proportion of the total contribution rate corresponding to the two principal components as weights, the comprehensive drought resistance evaluation function for P. edulis Sims was established as F = 0.628Y1 + 0.111Y2. The comprehensive drought resistance scores were calculated (Table 2). The higher the composite score, the stronger the drought resistance under stress, and vice versa. The drought resistance of the seven varieties ranked from strongest to weakest is as follows: XH-BL > XH-TWZ > TN1 > GH1 > ZJ-MT > LP-LZ > DH-JW.
3.6 Evaluate the Effects of Drought Stress on P. edulis Sims Using Hierarchical Clustering Heat Maps
The clustering heatmap (Fig. 7) shows that, compared with that of the control, the changing trend in all varieties of P. edulis Sims under the three drought stresses was as follows. The soluble sugar, soluble protein, free proline, MDA, CAT, and ABA contents all showed a gradually increasing trend. The peaks in SOD and POD activity occurred under moderate drought conditions, during which the SOD and POD activities first increased and then decreased. However, the height, diameter, GA, IAA, and ZR all showed a gradually decreasing trend. In particular, the CAT activity in DH-JW and ZJ-MT did not change significantly, the GA activity in DH-JW did not change significantly, and the GA activity in ZJ-MT exhibited an abnormal upward trend.
Figure 7: DH-JW(A), ZJ-MT (B), XH-BL (C), XH-TWZ (D), LP-LZ (E), TN1 (F), GH1 (G) hierarchical clustering heat map of drought stress in Passiflora edulis Sims under 75%–80% of the maximum field water capacity (CK), 55%–60% of the maximum field water capacity (LD), 40%–45% of the maximum field water capacity (MD), and 30%–35% of the maximum field water capacity (SD)
4.1 Changes in P. edulis Sims Growth under Drought Stress
Water stress can affect plant growth because cell growth is one of the most sensitive physiological processes to drought due to a reduction in turgor pressure [40]. In Masson, pine [41] and Sedum aizoon [42], under different drought stresses, seedling height, ground diameter, biomass, and other indicators decrease with increasing drought severity. In this study, the seedling height and ground diameter of P. edulis Sims gradually decreased with increasing drought stress, which might be because in the drought-stress environment, plants allocated more growth to the belowground part of the root system to absorb more water to maintain normal life activities, and at the same time slowed the growth of the aboveground part to reduce water and energy consumption; a reduction in biomass occurred in exchange for the extension and continuation of life.
4.2 Changes in the Osmotic Adjustment Substance Content of P. edulis Sims under Drought Stress
Under drought stress, plants accumulate large amounts of organic and inorganic ions to increase the concentration of cell sap, reduce the osmotic potential, improve the water retention of cells, maintain the cellular structure and spatial structure of biological molecules, and delay the senescence and death of plants. Organic substances such as proline, soluble sugars, and soluble proteins are important osmoregulatory substances [43]. Lozano-Montaña et al. [29] studied progressive drought stress in Passiflora edulis and showed that under drought stress, plants increased their soluble sugar and proline levels to regulate osmotic pressure and prevent cell dehydration. This phenomenon also occurs in other plants that are subjected to drought stress, and plants tend to compensate for drought stress by producing osmoregulatory substances such as soluble sugars and proline [44,45]. In this study, the contents of soluble sugar, proline, soluble protein, and other substances increased with increasing drought stress. Specifically, proline increased the most, and the increase in soluble sugar was mainly associated with mild and moderate drought stress. The increase in proline was mainly associated with severe drought stress, indicating that proline was an important osmoregulatory substance when P. edulis Sims was subjected to severe drought stress, which is consistent with the response of plants such as Eucommia ulmoides, Astragalus mongholicus, Elaeagnus angustifolia, and Schizonellia tenuifolia when they were subjected to drought stress [46–49]. Proline, an ideal osmotic adjustment substance and anti-dehydration agent in plant tissues, plays an important role in stabilizing the structure of protective films and biological macromolecules, scavenging free radicals, storing energy, and eliminating ammonia toxicity [50]. In this study, the TN1 variety of P. edulis Sims demonstrated a significant capacity to accumulate osmoregulatory substances such as proline, soluble sugars, and soluble proteins, especially under conditions of severe drought where the increase in these substances was the most pronounced. This suggests that TN1 may possess a more effective osmoregulatory mechanism, significantly enhancing the solute concentration within its cells, thereby better maintaining cell hydration and protecting against the damage induced by drought stress. Conversely, the DH-JW and ZJ-MT varieties showed the smallest increase in proline, soluble sugars, and soluble proteins under drought conditions, indicating that their abilities to retain cellular water and regulate osmotic pressure may not be as effective as in TN1. As a result, DH-JW and ZJ-MT are more susceptible to damage under long-term or intense drought conditions, which could lead to inhibited growth or premature senescence. Therefore, it can be preliminarily concluded that TN1 exhibits higher drought resistance and is more suitable for planting in karst-arid habitats, while DH-JW and ZJ-MT may be comparatively less resilient.
4.3 Changes in the Antioxidant Enzyme Activity and Malondialdehyde Content of P. edulis Sims under Drought Stress
Drought stress activated the antioxidant system of P. edulis Sims. Under drought stress, plants produce many active oxygen-free radicals via various means. In the protective enzyme system, SOD is the first line of defense against the removal of oxygen free radicals and plays a key role in the enzyme system. SOD can catalyze the disproportionation of oxygen free radicals to produce hydrogen peroxide (H2O2). The H2O2 produced is further catalyzed by CAT or POD to ultimately form harmless oxygen molecules and water. Under drought stress, the SOD and POD activities of P. edulis Sims seedlings first increased with increasing drought stress and then decreased under moderate or severe drought stress, which is consistent with the responses of alfalfa and hydrangea to drought stress [51,52]. This study revealed that the activities of SOD and POD in P. edulis Sims varieties XH-BL, XH-TWZ, and TN1 with relatively strong drought resistance increased significantly. When drought stress reached a certain degree, the MDA content resulting from membrane lipid peroxidation continuously increased, and the activity of antioxidant enzymes decreased. Moreover, osmotic adjustment substances regulate osmotic pressure to resist damage caused by drought stress in plant cells. Under drought stress, XH-TWZ exhibited the greatest increase in MDA, suggesting that it possesses a strong self-regulatory capacity when faced with arid conditions. In contrast, GH1 reached its peak MDA levels during moderate drought and showed a decline in severe drought, indicating that GH1’s ability to self-regulate MDA encounters difficulties beyond a certain threshold of drought stress. This reflects that GH1’s drought resistance is lower than that of XH-TWZ. Free radicals can cause damage to many cell components. In the present study, SOD and POD activities increased significantly to eliminate many oxygen free radicals generated in plants under drought stress, preventing free radicals from reacting with biological macromolecules in cells and ensuring the normal transport function and enzyme activity of the membrane, providing a basis for plant survival under adverse conditions. Antioxidant enzyme activities were different among the different P. edulis Sims varieties. For example, compared with that in the control treatment, the enzyme activity in the TN1 and other treatment groups significantly increased under drought stress, but the POD activity in the DH-JW cultivar under drought stress showed little or no significant change. The enzyme activity was related to the stress resistance of the plants. In arid environments, the activities of SOD and POD are low, which prevents the removal of free radicals. Moreover, CAT activity decreases, preventing H2O2 production during metabolism, resulting in H2O2 accumulation oxidation and damage to cells, leading to low drought resistance.
4.4 Changes in Endogenous Hormone Contents under Drought Stress
ABA is known as a stress hormone that acts mainly through the regulation of stomatal movement, enhances the permeability of the root system, promotes root growth, increases cytoplasmic Ca2+, depolarizes the membrane, activates genes responsible for preventing water stress, maintains the water balance in plant tissue, and improves the permeability of water to enhance plant resistance [53,54]. In the present study, the ABA content of the P. edulis Sims varieties except DH-JW increased under drought stress, which indicated that the ABA content of the P. edulis Sims seedlings increased under drought stress to promote root growth, regulate stomatal conductance, promote the redistribution of water and nutrients from source to sink, and thus improve the molecular mechanism of crop drought resistance. However, the change in the ABA content in DH-JW, a variety with poor drought resistance, was different from that in other P. edulis Sims varieties. As drought stress increased, the ABA content did not significantly change and was significantly lower than that in the other treatments under severe drought stress, indicating that the varieties with poor drought resistance had weak self-regulation of ABA under drought conditions and could not maintain the balance of tissue water, which led to significant impacts on plant growth and development. This was also evidenced by the poor height and ground diameter growth of DH-JW under severe stress. These changes are consistent with the changes in endogenous hormone levels in citrus plants under drought stress. The variation amplitude of ABA was greater in the variety with strong drought resistance and lower in the variety with weak drought resistance [55]. Li et al. [56] also reported that ZR and ABA had opposite effects on controlling the opening and closing of leaf stomata. ABA promoted the closing of leaf stomata, while ZR’s growth-promoting effect prevented the rapid closing of stomata when water was lost; thus, ABA alleviated water deficit in plants, while ZR aggravated water loss in plants. This could reasonably explain why the ABA content of the P. edulis Sims seedlings increased while the ZR content decreased, which was a physiological mechanism for maintaining the water balance of P. edulis Sims seedlings under drought stress.
Gaion et al. [57] showed that under drought conditions, the greatest decrease in stomatal conductance was observed in plants with a high GA content, which is beneficial for maintaining a high relative water content, and GA plays an important role in root–shoot communication to induce stomatal closure under water stress. In the present study, the GA content of multiple varieties, except DH-JW and ZJ-MT, significantly decreased under drought stress, indicating that P. edulis Sims did not respond to drought mainly by regulating the hormone GA. The IAA content showed a significant downward trend with the aggravation of drought stress. A.H.A. Farooqi et al. [58] indicated that IAA could partially alleviate the adverse effects of drought on plants. Pustovoitova et al. [59] indicated that IAA also participated in the development of defense responses during drought adaptation. These results indicated that P. edulis Sims had a weak ability to self-regulate IAA to cope with drought conditions.
4.5 Comprehensive Evaluation of Drought Resistance in P. edulis Sims
Principal component analysis, correlation analysis, and cluster analysis all indicate that SOD, POD, ABA, soluble sugar, CAT, free proline, soluble protein, and MDA can be grouped together. These physiological indicators are closely related to drought stress, with their increasing magnitudes indicative of stronger drought resistance. This is similar to the research findings on drought stress in P. edulis Sims conducted by Qi et al. [60]. This suggests that these 8 indicators are key factors in the response of P. edulis Sims to drought stress. Meanwhile, under drought conditions, ZR, IAA, GA, height, and diameter all decrease and are classified into another group. P. edulis Sims reduces ZR to delay water loss in response to drought, while IAA and GA, hormones known for their ability to combat drought, show a sharp decrease during stress, suggesting that P. edulis Sims does not rely on IAA and GA to cope with drought or that its ability to self-regulate IAA and GA under drought conditions is limited. By conducting a comprehensive evaluation of these indicators, the varieties are ranked from strongest to weakest in terms of drought resistance as follows: XH-BL > XH-TWZ > TN1 > GH1 > ZJ-MT > LP-LZ > DH-JW.
This study is original and significant as it systematically assesses a range of key physiological indicators of P. edulis Sims in the karst drought environment for the first time, clarifying the direct relationship between these indicators and the plant’s drought resistance. Based on this, an effective evaluation and ranking of the drought resistance of different varieties have been conducted. These achievements not only broaden our understanding of the strategies P. edulis Sims employs to combat drought stress but also provide a scientific basis for cultivating varieties with greater drought resistance in similar arid environments in the future, holding significant theoretical value and application prospects. Moreover, the methodological framework and findings of this study can serve as a reference for other plant drought-resistance research, promoting further studies into the physiological mechanisms of plants under drought stress and the advancement of drought-resistant crop breeding techniques.
In this study, the changes in the various physiological and biochemical indices of seven P. edulis Sims varieties under drought stress were determined, and a comprehensive evaluation was conducted based on principal component analysis of the determined indicators. The results showed that the drought resistance of the varieties decreased in the order XH-BL > XH-TWZ > TN1 > GH1 > ZJ-MT > LP-LZ > DH-JW. Under drought stress, the height, ground diameter, GA, IAA, and ZR indices of P. edulis Sims showed a downward trend. P. edulis Sims responds to drought by reducing ZR levels, rather than by adjusting the hormones IAA and GA. However, P. edulis Sims can cope with drought stress and maintain their water balance by increasing soluble sugar, soluble protein, free proline, MDA, CAT, ABA, SOD, POD, and other indicators.
Acknowledgement: None.
Funding Statement: This work is supported jointly by the Science and Technology Project of Guizhou Province (Qian-Ke-He Platform Talents [2021]5624), the National Natural Science Foundation of China (31960576), and Science and Technology Project of Guizhou Province (Qian-Ke-He Support [2021] General 228) were funded.
Author Contributions: Study conception and design, Binyang Zhao, Anding Li, Fengchan Wu; Experiment development and results analysis, Binyang Zhao, Fengchan Wu, Peiyu Xi, Yulin Guo; writing—original draft preparation, Binyang Zhao, Fengchan Wu; Project management and inspection, Binyang Zhao, Anding Li, Fengchan Wu, Guojun Cai. All authors reviewed the results and approved the final version of the manuscript.
Availability of Data and Materials: The datasets used and/or analyzed during the current study are available from the author and/or corresponding author on reasonable request.
Ethics Approval: Not applicable.
Conflicts of Interest: The authors declare that they have no conflicts of interest to report regarding the present study.
References
1. Zhang J, Yang T, Zhang C, Zhang T, Pu L, Zhao W. Effects of exogenous zinc on the physiological characteristics and enzyme activities of Passiflora edulis Sims f. edulis seedlings. PeerJ. 2023;11:e16280. doi:10.7717/peerj.16280. [Google Scholar] [PubMed] [CrossRef]
2. Song S, Zhang D, Ma F, Xing W, Huang D, Wu B, et al. Genome-wide identification and expression analyses of the aquaporin gene family in passion fruit (Passiflora edulisrevealing PeTIP3-2 to be involved in drought stress. Int J Mol Sci. 2022;23(10):5720. doi:10.3390/ijms23105720. [Google Scholar] [PubMed] [CrossRef]
3. Ortiz DC, Bohórquez A, Duque MC, Tohme J, Cuéllar D, Mosquera Vásquez T. Evaluating purple passion fruit (Passiflora edulis Sims f. edulis) genetic variability in individuals from commercial plantations in Colombia. Genet Resour Crop Evol. 2011;59(6):1089–99. doi:10.1007/s10722-011-9745-y. [Google Scholar] [CrossRef]
4. Dhawan K, Dhawan S, Sharma A. Passiflora: a review update. J Ethnopharmacol. 2004;94(1):1–23. doi:10.1016/j.jep.2004.02.023. [Google Scholar] [PubMed] [CrossRef]
5. Asande LK, Omwoyo RO, Oduor RO, Nyaboga EN. A simple and fast Agrobacterium-mediated transformation system for passion fruit KPF4 (Passiflora edulis f. edulis × Passiflora edulis f. flavicarpa). Plant Methods. 2020;16:141. doi:10.1186/s13007-020-00684-4. [Google Scholar] [PubMed] [CrossRef]
6. Liu S, Li A, Chen C, Cai G, Zhang L, Guo C, et al. De novo transcriptome sequencing in Passiflora edulis Sims to identify genes and signaling pathways involved in cold tolerance. Forests. 2017;8(11):435. [Google Scholar]
7. Zhang LM, Peng X, Cai GJ, Zhang SJ, Wu YH, Li AD. Comprehensive evaluation and analysis of passion fruit in different frame modes. Sci Technol Food Ind. 2021;42(21):33–40 (In Chinese). [Google Scholar]
8. Bu HY, Song WZ, Cao CG, Li P. Root growth responses to soil water deficit for a water-saving and drought-resistant rice Genotype Hanyou113. Sci Agric Sin. 2017;50(22):4277–89 (In Chinese). [Google Scholar]
9. Liu X, Zhu X, Pan Y, Bai J, Li S. Performance of different drought indices for agriculture drought in the North China Plain. J Arid Land. 2018;10(4):507–16. [Google Scholar]
10. Fensham RJ, Fairfax RJ. Drought-related tree death of savanna eucalypts: species susceptibility, soil conditions and root architecture. J Veg Sci. 2007;18(1):71. [Google Scholar]
11. Sheffield J, Wood EF. Global trends and variability in soil moisture and drought characteristics, 1950–2000, from observation-driven simulations of the terrestrial hydrologic cycle. J Clim. 2008;21(3):432–58. [Google Scholar]
12. Sheffield J, Wood EF, Roderick ML. Little change in global drought over the past 60 years. Nature. 2012;491(7424):435–8. doi:10.1038/nature11575. [Google Scholar] [PubMed] [CrossRef]
13. Dai A. Drought under global warming: a review. WIREs Climate Change. 2010;2(1):45–65. doi:10.1002/wcc.81. [Google Scholar] [CrossRef]
14. Zhang X, Wu N, Li C. Physiological and growth responses of Populus davidiana ecotypes to different soil water contents. J Arid Environ. 2005;60(4):567–79. doi:10.1016/j.jaridenv.2004.07.008. [Google Scholar] [CrossRef]
15. Monneveux P, Rekika D, Acevedo E, Merah O. Effect of drought on leaf gas exchange, carbon isotope discrimination, transpiration efficiency and productivity in field grown durum wheat genotypes. Plant Sci. 2006;170(4):867–72. doi:10.1016/j.plantsci.2005.12.008. [Google Scholar] [CrossRef]
16. Buckley TN, Mott KA. Modelling stomatal conductance in response to environmental factors. Plant Cell Environ. 2013;36(9):1691–9. doi:10.1111/pce.12140. [Google Scholar] [PubMed] [CrossRef]
17. Galmés J, Ribas-Carbó M, Medrano H, Flexas J. Response of leaf respiration to water stress in Mediterranean species with different growth forms. J Arid Environ. 2007;68(2):206–22. doi:10.1016/j.jaridenv.2006.05.005. [Google Scholar] [CrossRef]
18. Hewitt S, Hernández-Montes E, Dhingra A, Keller M. Impact of heat stress, water stress, and their combined effects on the metabolism and transcriptome of grape berries. Sci Rep. 2023;13(1):9907. doi:10.1038/s41598-023-36160-x. [Google Scholar] [PubMed] [CrossRef]
19. de Ollas C, Arbona V, Gómez-Cadenas A. Jasmonoyl isoleucine accumulation is needed for abscisic acid build-up in roots of Arabidopsis under water stress conditions. Plant Cell Environ. 2015;38(10):2157–70. doi:10.1111/pce.12536. [Google Scholar] [PubMed] [CrossRef]
20. Zhang Z, Wang X, Li X, Zhang M. Effect of soil natural drought on growth, physiological characteristics and accumulation of main medicinal components of Uncaria rhynchophylla. Acta Bot Boreali-Occident Sin. 2020;40(4):658–66 (In Chinese). [Google Scholar]
21. Wang S, Ji H, Ouyang Z, Zhou D, Zhen L, Li T. Preliminary study on weathering and pedogenesis of carbonate rock. Sci China D Earth Sci. 1999;42(6):572–81. doi:10.1007/BF02877784. [Google Scholar] [CrossRef]
22. Chen XB, Zheng H, Zhang W, He XY Li L, Wu JS, et al. Effects of land cover on soil organic carbon stock in a karst landscape with discontinuous soil distribution. J Mt Sci. 2014;11(3):774–81. doi:10.1007/s11629-013-2843-x. [Google Scholar] [CrossRef]
23. Dai QH, Yan YJ. Research progress of karst rocky desertification and soil erosion in Southwest China. J Soil Water Conserv. 2018;32(2):1–10 (In Chinese). [Google Scholar]
24. Che JX, Peng X, Su WC. Study on water balance and drought-resistant mechanism of dominant plant in hot-low karst valley. Ecol Econ. 2008;(6):49–51 (In Chinese). [Google Scholar]
25. Dong WP, Li AD, Zhang JL, Long XQ. Characteristics of wild habitat and ecological niche of Passiflora edulis in karst peak cluster depression. Acta Agric Zhejiangensis. 2019;31(8):1305–11 (In Chinese). [Google Scholar]
26. Huang J, Yu H, Guan X, Wang G, Guo R. Accelerated dryland expansion under climate change. Nat Clim Change. 2016;6(2):166–171. [Google Scholar]
27. Fensholt R, Rasmussen K, Nielsen TT, Mbow C. Evaluation of earth observation based long term vegetation trends—Intercomparing NDVI time series trend analysis consistency of Sahel from AVHRR GIMMS, Terra MODIS and SPOT VGT data. Remote Sensing Environ. 2009;113(9):1886–98. doi:10.1016/j.rse.2009.04.004. [Google Scholar] [CrossRef]
28. Kusvuran S, Dasgan HY. Drought induced physiological and biochemical responses in Solanum lycopersicum genotypes differing to tolerance. Acta Sci Pol Hortorum Cultus. 2017;16(6):19–27. doi:10.24326/asphc.2017.6.2. [Google Scholar] [CrossRef]
29. Lozano-Montaña PA, Sarmiento F, Mejía-Sequera LM, Álvarez-Flórez F, Melgarejo LM. Physiological, biochemical and transcriptional responses of Passiflora edulis Sims f. edulis under progressive drought stress. Sci Hortic. 2021;275:109655. doi:10.1016/j.scienta.2020.109655. [Google Scholar] [CrossRef]
30. Oraee A, Tehranifar A. Evaluating the potential drought tolerance of pansy through its physiological and biochemical responses to drought and recovery periods. Sci Hortic. 2020;265:109225. doi:10.1016/j.scienta.2020.109225. [Google Scholar] [CrossRef]
31. Xiong Y, Qu Y, Han H, Chen F, Li L, Tang H, et al. Unraveling physiological and metabolomic responses involved in Phlox subulata L. tolerance to drought stress. Plant Mol Biol Report. 2020;39(1):98–111. doi:10.1007/s11105-020-01238-7. [Google Scholar] [CrossRef]
32. Santner A, Estelle M. Recent advances and emerging trends in plant hormone signalling. Nature. 2009;459(7250):1071–8. doi:10.1038/nature08122. [Google Scholar] [PubMed] [CrossRef]
33. Ross JJ, Weston DE, Davidson SE, Reid JB. Plant hormone interactions: how complex are they? Physiol Plant. 2011;141(4):299–309. doi:10.1111/j.1399-3054.2011.01444.x. [Google Scholar] [PubMed] [CrossRef]
34. Peleg Z, Blumwald E. Hormone balance and abiotic stress tolerance in crop plants. Plant Biotechnol J. 2011;9(7):747–58. doi:10.1111/j.1467-7652.2010.00584.x. [Google Scholar] [CrossRef]
35. Noor M, Fan J, Kaleem M, Akhtar MT, Jin S, Nazir U, et al. Assessment of the changes in growth, photosynthetic traits and gene expression in Cynodon dactylon against drought stress. BMC Plant Biol. 2024;24(1):235. doi:10.1186/s12870-024-04896-x. [Google Scholar] [PubMed] [CrossRef]
36. Wu F. Evaluation and screening of cold and drought resistance of passion fruit rootstock (Master Thesis). Guizhou University: China; 2022 (In Chinese). [Google Scholar]
37. Koide RT, Robichaux RH, Morse SR, Smith CM. Plant water status, hydraulic resistance and capacitance. Plant physiological ecology: Field methods and instrumentation. Dordrecht Springer Netherlands; Springer; 1989. p. 161–83. [Google Scholar]
38. Chen C. Ecophysiological mechanisms of forage shrubs resistant to drought & cold in karst areas (Doctor Thesis). China Agricultural University: China; 2014 (In Chinese). [Google Scholar]
39. Boussakouran A, El Yamani M, Sakar EH, Rharrabti Y. Genetic advance and grain yield stability of moroccan durum wheats grown under rainfed and irrigated conditions. Interna J Agro. 2021;1(2021):5571501. [Google Scholar]
40. Wu Y, Cosgrove DJ. Adaptation of roots to low water potentials by changes in cell wall extensibility and cell wall proteins. J Exp Bot. 2000;51(350):1543–53. doi:10.1093/jexbot/51.350.1543. [Google Scholar] [PubMed] [CrossRef]
41. Quan W. Response mechanism of Masson pine seedlings to drought stress (Doctor Thesis). Guizhou University: China; 2018 (In Chinese). [Google Scholar]
42. Su D, Sun G, Zhang J, Jiang C, Yu Q, Gu D, et al. Effects of soil water stress on growth and biomass distribution of sedum aizoon and Sedum spectabilis. Acta Hortic Sin. 2007;34(5):1317–20 (In Chinese). [Google Scholar]
43. Li Y, Fan C, Lei Z, Zhang L, Lu Y, Wang T. Comparative analysis on drought tolerance of two maize cultivars at germination and seedling stages. J Northwest Univ. 2020;50(5):703–10 (In Chinese). [Google Scholar]
44. Furlan AL, Bianucci E, Giordano W, Castro S, Becker DF. Proline metabolic dynamics and implications in drought tolerance of peanut plants. Plant Physiol Biochem. 2020;151:566–78. doi:10.1016/j.plaphy.2020.04.010. [Google Scholar] [PubMed] [CrossRef]
45. Crespo SC, Moreno-Chacón AL, Rojas A, Melgarejo LM. Principal component analysis of changes due to water stress for some osmolytes, pigments and antioxidant enzymes in Gmelina arborea Robx. Leaves from trees planted in northern Colombia. J Braz Chem Soc. 2011;22(12):2275–80. doi:10.1590/S0103-50532011001200006. [Google Scholar] [CrossRef]
46. He F, Liu P, Wang L, Du Y, Qing J, Du Q, et al. Effect of drought stress and rewatering on physiological characteristics of Eucommia ulmoides seedling. Plant Physiol J. 2021;57(3):661–71 (In Chinese). [Google Scholar]
47. Li K, Zhou Z, Li S, Yao H, Zhou Y, Chou Y, et al. Growth, osmotic adjustment and antioxidant capacity responses of Schizonepeta tenuifolia to drought stress. Acta Prataculturae Sinica. 2020;29(5):150–8 (In Chinese). [Google Scholar]
48. Lv E, Zhou X, Zhou Z, Zhao G. Physiological responses of the desert shrub Hedysarum mongolicum to drought stress. Acta Pratacultur Sinica. 2016;25(6):42–50 (In Chinese). [Google Scholar]
49. Chen C, Xie X, Wang Y, Li J, Xin J, Zhu T, et al. Effects of salt and drought on the physiological characteristics of Elaeagnus angustifolia L. seedlings. Acta Ecol Sin. 2019;39(12):4540–50 (In Chinese). [Google Scholar]
50. Yang H. The effet of drought stress influencein the proline metabolism pathways of seedling period of sugar beet (Master Thesis). Harbin Institute of Technology: China; 2016 (In Chinese). [Google Scholar]
51. Zhang C, Shi S, Liu Z, Yang F, Zhang Z. Effects of drought stress on the root morphology and anatomical structure of alfalfa (Medicago sativa) varieties with differing drought-toleranc. Acta Prataculturae Sin. 2019;28(5):79–89 (In Chinese). [Google Scholar]
52. Ren Q, Sun J, Zhangm D, Ding Y, Zhang Y, Zhang J. Physiological response and drought resistance evaluation of different Hydrangea varieties under drought stress. Acta Agric Zhejiangensis. 2021;33(10):1852–60 (In Chinese). [Google Scholar]
53. Sarfraz A, Vikas B, Sumer Singh P, Manohar R, Dalip, Shyam Singh R, et al. Role of plant secondary metabolites and phytohormones in drought tolerance: a review. Gesunde Pflanz. 2022;75(4):729–46. [Google Scholar]
54. Zhao Y, Zhang Z, Gao J, Wang P, Hu T, Wang Z, et al. Arabidopsis duodecuple mutant of PYL ABA receptors reveals PYL repression of ABA-independent SnRK2 activity. Cell Rep. 2018;23(11):3340–51.e5. doi:10.1016/j.celrep.2018.05.044. [Google Scholar] [PubMed] [CrossRef]
55. Hussain S, Khalid MF, Saqib M, Ahmad S, Zafar W, Rao MJ, et al. Drought tolerance in citrus rootstocks is associated with better antioxidant defense mechanism. Acta Acta Physiol Plant. 2018;40(8):135. doi:10.1007/s11738-018-2710-z. [Google Scholar] [CrossRef]
56. Li J, Huang J, Wang Q, Ruan X. The response of content of endogenous hormones and osmotic regulaters in haloxylon ammodendron leaves to high temperature stress. JN Anjing For Univ. 2005;N/A(6):45–8 (In Chinese). [Google Scholar]
57. Gaion LA, Carvalho RF. Stomatal response to drought is modulated by gibberellin in tomato. Acta Physiol Plant. 2021;43(9):129. doi:10.1007/s11738-021-03286-2. [Google Scholar] [CrossRef]
58. Farooqi AHA, Fatima S, Khan A, Sharma S. Ameliorative effect of chlormequat chloride and IAA on drought stressed plants of Cymbopogon martinii and C. winterianus. Plant Growth Regul. 2005;46(3):277–84. doi:10.1007/s10725-005-8807-6. [Google Scholar] [CrossRef]
59. Pustovoitova TN, Zhdanova NE, Zholkevich VN. Changes in the levels of IAA and ABA in cucumber leaves under progressive soil drought. Russ J Plant Physiol. 2004;51(4):513–7. doi:10.1023/B:RUPP.0000035745.82509.bc. [Google Scholar] [CrossRef]
60. Qi Y, Ma L, Ghani MI, Peng Q, Fan R, Hu X, et al. Effects of drought stress induced by hypertonic polyethylene glycol (PEG-6000) on passiflora edulis sims physiological properties. Plants. 2023;12(12):2296. [Google Scholar] [PubMed]
Appendix A
Cite This Article
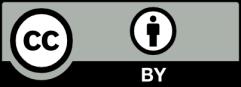
This work is licensed under a Creative Commons Attribution 4.0 International License , which permits unrestricted use, distribution, and reproduction in any medium, provided the original work is properly cited.