Open Access
REVIEW
Ecosystem Services of Grazed Grasslands in the Flooding Pampa
1 Facultad de Agronomía, Área de Agroecología, Universidad de Buenos Aires, Buenos Aires, 1417, Argentina
2 Facultad de Agronomía, Departamento de Producción Animal, Universidad de Buenos Aires, Buenos Aires, 1417, Argentina
* Corresponding Author: Elizabeth J. Jacobo. Email:
(This article belongs to the Special Issue: Ecology of Rangelands in Argentina)
Phyton-International Journal of Experimental Botany 2024, 93(6), 1179-1202. https://doi.org/10.32604/phyton.2024.050928
Received 22 February 2024; Accepted 30 May 2024; Issue published 27 June 2024
Abstract
The Flooding Pampa grasslands are the last remnant of the Rio de la Plata grasslands in Argentina. Anthropogenic interventions have led to severe degradation and, as a result, the ecosystem services provided by the grasslands are declining, in terms of provisioning, regulating, and supporting services. We synthesized the existing literature on the ecosystem goods and services provided by these grasslands under grazing in different conditions and conservation status. We found that plant and animal diversity and primary production are the most studied ecosystem services, while climate regulation, water supply, nutrient cycling, meat production and erosion control, in that order, are less studied. Cultural services are under-researched. Continuous grazing and glyphosate spraying are the main drivers of grassland degradation. Controlled grazing and conservative stocking rates have been shown to reverse degradation and demonstrate that livestock production is compatible with ecosystem conservation by maintaining regulating and provisioning services. As these management strategies are poorly integrated, improving their implementation will require important changes in farmers’ decisions and the development of policies that create the economic conditions for this to happen. Research is needed to understand the conditions that prevent the knowledge generated from being transferred to producers and translated into practices that would improve the provision of ecosystem services.Keywords
The Río de la Plata Grasslands (RPG) region covers approximately 853,000 km2 and includes the extensive plains of Argentina, Uruguay, and southern Brazil that surround the estuary of the Río de la Plata and its major tributaries [1]. The Pampas region, covering 398,966 km2 in central-eastern part of Argentina (Fig. 1), is one of the largest temperate grassland regions globally and the most significant grassland ecosystem in the country [2]. It is a strategic area, accounting for 40% of the agricultural land in the RPG region [3] and is home to almost half of Argentina’s population [4].
Figure 1: Pampa region and its subregions. Insert: location of Pampa region in Argentina
Within the Pampa region, different subregions are recognised based on biophysical characteristics [3] (Fig. 1). The Rolling and Flat Inland Pampa subregions, with deep and fertile soils that are highly suitable for agriculture, have undergone intense land use change, resulting in the replacement of approximately 75% of natural grassland with annual crops [2,5]. The Flooding Pampa subregion (90,000 km2) has an average annual rainfall that varies from 1000 mm in the north to 850 mm in the south, evenly distributed throughout the year, and monthly temperatures that range from 6.8°C in July–August to 21.8°C in January [6]. This sub-region is characterised by shallow soil depth, soil salinity [7] and frequent floods and droughts [8], which severely limit agricultural production, thus preserving a large area of natural or semi-natural grasslands1.
The proportion of natural or semi-natural grasslands area varies from 53% to 89%, depending on the district [10], with an average of 68% [11]. These grasslands are the main source of fodder for livestock, as extensive livestock farming has been the main economic activity since Spanish colonisation to the present day.
Natural grassland ecosystems provide several goods and services to society [12], both directly through the provision of food, and indirectly through supporting and regulating services [13]. However, the provision of ecosystem goods and services is strongly dependent on the state and condition of grasslands, caused by anthropogenic interventions [14–16].
Therefore, the objectives of this review article are (i) to describe the history of use and its effects on the Flooding Pampa grasslands, (ii) to synthesise the existing literature on the ecosystem goods and services provided by the Flooding Pampa grasslands under grazing in different condition and conservation status caused by human interventions, (iii) to analyse and discuss the existing work to complete the state of knowledge and suggest new research directions. The achievement of these objectives will permit the organization and updating of the state of the art regarding the provision of goods and services in the Flooding Pampa grasslands.
We conducted a comprehensive literature search on 05 February 2024 in order to identify ecosystem goods or services provided by the Flooding Pampa grasslands. We first used the free search engines Google Scholar (https://www.google.com/, accessed on 09/04/2024) and Research Gate (https://www.researchgate.net/, accessed on 09/04/2024) to search across a wide range of academic publications. We searched by the terms “Flooding Pampa AND Grazing” and “Flooding Pampa Grassland AND Ecosystem Services”, and the same terms in Spanish “Pampa Deprimida Pastoreo”, and “Pastizales Pampa Deprimida Servicios Ecosistémicos” within the time range from 1980 to 2023. A total of 1140 results were obtained, from which we selected articles, reviews and thesis that explicitly addressed some function, process, or indicator of ecosystem services provided by the Flooding Pampa grasslands. From this selection, 145 results were obtained. We also conducted a second literature search in the scientific database Scopus, Science Direct, Springer and JSTOR using the same terms in English within the same period. From this search we selected 77 scientific articles, all of them were included in the first search.
We considered the spatial scale, so we referred globally to the Rio de la Plata grasslands at the regional scale, to the Flooding Pampa grasslands at the subregional scale, and specifically to the different vegetation communities that make up the landscape of the Flooding Pampa subregion. We have included some references to the grasslands of other subregions of the RPG where relevant.
The main conceptual models related to the ecology and management of grasslands were reviewed in order to discuss their consistency with the empirical results obtained in the grazed grasslands of the Flooding Pampa.
We preceded the results related to some ecosystem goods and services with a brief theoretical framework when it was necessary to improve their interpretation.
We have included several case studies to illustrate the best practices that have led to the successful implementation of conservation strategies.
3.1 Conceptual Models and Evolutionary History
In grassland ecology and management science, the multiple equilibrium, state-and-transition [17] or state-and-threshold model [18] and the generalized model of the effects of grazing on plant community structure and diversity [19] have been widely accepted as conceptual frameworks that were supported by empirical evidence. The generalized model predicted the response of plant diversity to grazing intensity, as a function of rainfall availability (subhumid or semi-arid climate) and evolutionary history of grazing (long or short) and assumed that rangelands in southern South America had a short evolutionary history of grazing [19]. A reworking and combination of these models to include a wider range of real situations concluded that the rangeland systems of southern South America could have a longer evolutionary history of grazing [20]. Several examples supported this conclusion, such as the case of Flooding Pampas grasslands, which did not respond to the prediction of the generalized model because changes in vegetation structure caused by grazing were reversible, as predicted for systems with a long history [20] (Fig. 2). Other studies have confirmed that South America has a long evolutionary history of herbivory by large grazers [21,22]. Although large herbivores became extinct in South America at the end of the Pleistocene and the beginning of the Holocene [23], the period of about 10,000 years without grazing by large herbivores until the introduction of domestic livestock is considered insufficient to substantially change the regional grassland species pool and the absence of large grazers may have been a major factor that increased fire frequency at the end of the Pleistocene in the Rio de la Plata grassland [1]. Thus, in humid ecosystems fire maintained an analogous selective pressure for traits also adapted to grazing, such as rapid regrowth after biomass loss [24].
Figure 2: Diversity as function of grazing intensity for a site with high productivity that have evolved under long history of grazing, such as Flooding Pampa grassland. Vertical arrow indicates the grazing intensity after which irreversible transitions can occur. Adapted from [20,25]
3.2 Grazing and Degradation History
The Spanish colonizers introduced domestic livestock to Argentina in 1549. A second arrival of cattle in the Pampas region between 1570 and 1580 increased the size of the herds [26]. The temperate climate and herbaceous vegetation of the Pampas allowed for a high reproductive rate of cattle, resulting in a population of 20 million head by 1850 [26,27]. Consequently, Argentina became the primary supplier of leather and meat to Western Europe in the second half of the 19th century. Between 1875 and 1930, the land used for agricultural crops increased from 100,000 to 10 million hectares [28]. Since then, agriculture has continued to expand, leading to the conversion of natural grassland to crops. From 2001 to 2018, this process was the primary cause of land use change in the Pampa region, resulting in a decline in ecosystem functions and services [29,30].
Since Spanish colonization cattle grazed freely on the native grasslands until they were confined when the new owners fenced their properties in the early 20th century. The structure and function of Flooding Pampa grasslands were altered by the uncontrolled or continuous grazing of the introduced livestock. Continuous grazing by large, introduced herbivores has increased the relative contribution of annual and forbs, mainly exotic and cool-season species of Eurasian origin and reduced the relative contribution of warm-season native perennial grasses that dominated the original vegetation [31,32]. Although these changes have increased diversity by adding non-native species to the grassland, they have significantly reduced aboveground net primary production [33]. As a result, after almost 100 years of continuous grazing, stocking rates, secondary productivity and economic returns declined towards the end of the last century (1995) from 0.77 to 0.49 animal units ha−1, from 105.2 to 66.4 kg live weight ha−1 and from 14 to −4.4 u$s ha−1 ,respectively [34]. A more recent reassessment conducted in 2017 also showed a similar tendency to decline in stocking rates (from 1 to 0.6 animal units ha−1), secondary productivity (from 182 to 84 kg live weight ha−1) and economic returns (from 130 to −10 u$s ha−1) under continuous grazing [35].
At the beginning of this century, the process of agriculture expansion and intensification in the Pampa region that initiated, led to a drastic change in land use. This change was made possible by the introduction of genetically modified soybeans tolerant to glyphosate [36] and the elimination of soil tillage (no tillage) [37]. As a result, pastures and annual fodder crops have been replaced by wheat–soybean relay cropping and maize crops. Due to the decrease in land allocated for forage production in the fertile areas of the Pampas, cattle were moved to the less fertile areas, such as the Flooding Pampa. This led to an increase in the stocking rate in this subregion, where native grasslands were the dominant land use and were partially replaced by annual forage crops or cultivated pastures [38]. In this subregion, the main change in land cover was from natural grasslands to semi-natural grasslands. This change was attributed to the intensification of livestock activity [5]. Additionally, an increase in stocking rate may exacerbate overgrazing of remnant native grasslands, resulting in negative impacts on aboveground net primary productivity, plant and animal diversity, and soil organic carbon and erosion [11].
To manage with the higher stocking rate, farmers in the Flooding Pampa have adopted a new technology that involves using glyphosate and nitrogen fertilisation to increase forage productivity during winter, when forage production is typically lower. Glyphosate is sprayed in late summer to eliminate vegetation composed mainly of C4 grasses and forbs, and to improve the germination and establishment of cool-season (C3) annual grasses. The main component of these grasses is Lolium multiflorum. Initially, this practice increased winter forage production and allowed for improvements in stocking rates and meat production [39]. However, recurrent application of glyphosate dramatically alters the structure and function of the vegetation community. It greatly reduces species richness and diversity, causes the local extinction of several native perennial species [40], reduces the annual amount of ANPP and changes its seasonal pattern, resulting in a dramatic reduction in spring and summer [41].
Due to more than a century of uncontrolled or continuous grazing in most of the area and more than twenty years of glyphosate application in the most productive communities, natural grasslands have severely degraded. As a result of this degradation process, medium-scale traditional farmers have a low meat production of about 87.5 kg PV ha−1 year−1 [42].
3.3 Controlled Grazing as a Tool to Reverse Degradation
In the late 1990s studies demonstrated the superiority of rotational or Adaptative Multi Paddock Grazing (AMPG) over continuous stocking in maintaining productivity and conserving and restoring native grasslands in North America [43–45], Australia [46] and the Flooding Pampa [47,48]. Furthermore, recent research has found a positive association between AMPG and the physical wellbeing of Canadian ranchers [49].
In the Flooding Pampa, the AMPG was referred to as Controlled Grazing (CG). CG involves dividing the land surface into homogeneous areas based on the type of plant community and applying disturbances, mainly grazing with high instantaneous stocking rates followed by a resting period of variable duration. The grazing intensity, timing and resting period duration are determined based on the plant community, season and objective pursued. This includes restoring the vigour of species with high forage value, controlling selectivity, modifying competitive relationships between functional groups, promoting germination and establishment, as well as flowering and fruiting of desired species [34].
Long-term evaluation at farm scale showed that CG increased the contribution of functional group species of high forage value and reduced the proportion of less valuable forage grasses and forbs [48]. Consequently, the grassland condition index, an indicator of grassland health and productivity, was significantly higher on cattle farms that applied CG (average 75%) than on those that applied continuous grazing (average 20%) [50]. In non-halophytic communities, CG anticipated the establishment and increased the biomass production of winter grasses with high nutritive value [47]. This reduces the winter forage deficit, which is the main limitation for cattle production [34]. In halophytic communities, CG increased vegetation cover, species richness, the contribution of cool-season grasses and forage quality [51].
The statement that these grasslands have a long evolutionary history of herbivory by large grazers and/or fire, which maintained a selective pressure towards traits that are also adapted to grazing (see Section 3.1) is supported by the reversion of the degradation of vegetation structure and function via CG.
3.4 Ecosystem Services Provided by Grasslands at Different Conservation Status
Natural grassland ecosystems provide various goods and services to society [12], both directly through the provision of food, and indirectly through supporting and regulation services [13]. However, the provision of ecosystem goods and services depends heavily on the state and condition of the grassland, which is influenced by anthropogenic interventions [14–16]. The subsequent subsections summarise the primary goods and services offered by the Flooding Pampa grasslands under different conservation statuses resulting from human interventions.
3.4.1 Supporting Ecosystem Services
Supporting services refers to the fundamental ecosystem processes that contribute to human wellbeing and maintain the functions necessary for provisioning, regulating, and cultural services [52].
Habitat Provision: Animal Diversity
The Pampa grasslands currently host an estimated 300 bird species, 65 mammal species, 49 reptile species and 35 amphibian species. Agricultural intensification has had a negative impact on animal diversity because it has homogenised the landscape. Birds and carnivores have been more severely affected than rodents and insects. The geographic range and/or abundance of many native species has been reduced, including those of carnivores, herbivores, and specialists, sometimes leading to regional extinctions. Meanwhile some native species have been unaffected or even benefited, and novel species have been introduced [53].
The Flooding Pampa has a greater richness and abundance of birds compared to other pampa sub-regions where agricultural activity dominates and grassland coverage is less extensive. This highlights that livestock activity provides the best scenario for bird conservation [54]. The greater species richness of the Flooding Pampa is due to the higher presence of habitat-specific bird species, which are specialists in grassland habitat. Generalist species, on the other hand, are adapted to a variety of open habitats [55]. The rapid conversion of natural grasslands into agricultural land has a significant impact on grassland birds, whose reproduction is strictly dependent on these environments. Several threatened grassland specialists in the Pampas, such as Rhea americana and Asthenes hudsoni, are exclusively found in livestock landscapes, and the long-term survival of these species will depend on the conservation of this landscape type [56].
The structure of the vegetation affects bird richness [57]. To promote a diverse range of bird species, it is desirable to have a mosaic of short and tall native grasslands with patches of tussocks. The heterogeneous vegetation structure benefits various functional groups, as different grassland types elicit different responses to grassland birds [58]. The presence of tussocks, consisting of tall grasses such as Andropogon lateralis and Cortadeira selloana, have been shown to increase bird species diversity. This strategy has even been suggested for protecting endangered species [59]. Conversely, habitats with short grasses are necessary for certain migratory shorebird species [60].
Livestock management practices are used to modify vegetation structure, which can affect the abundance and structure of bird assemblages in the Flooding Pampa. Continuous grazing in livestock farming with homogenises grasslands in space and time leading to the replacement of grassland birds that are highly dependent on tall grasses with others that select short grasses [61]. However, the use of glyphosate to promote L. multiflorum has been found to decrease the abundance of short grassland birds due to the low vegetation cover in the lowest layer (less than 10 cm) of the canopy [62]. A recent study compared Grassland Alliance farms managed by CG with neighbouring farms managed by continuous grazing and found that bird species diversity was higher on the former. CG has allowed the establishment of populations of specialist grassland bird species, some of which are threatened with extinction [63]. The greater spatial and temporal variation in grassland height under CG allows for the presence of species with different habitat requirements for nesting and foraging [64].
Agricultural expansion has caused severe habitat loss for mammals. Large carnivores have become locally extinct [64], while populations of medium-sized carnivores and wild ungulates have significantly declined [64,65]. Additionally, rodents and other small mammals are reducing diversity, favouring more common species at the expense of rare or habitat-specific species [53,66]. It is worth noting that grasslands serve as refuges for several native species. The Tandilia mountain system, a grassland relict of the Southern Pampa and a contiguous subregion to the Flooding Pampa, contains 44% of the mammal diversity of the Pampas ecoregion. This includes species that are endemic to the ecoregion, globally declined species, and threatened species [67]. The selection of mammal species appears to be influenced by vegetation structure, with a preference for more complex and diverse habitats associated with extensive livestock production [68,69]. He pampas deer (Ozotocerus bezoarticus) is an endangered species [70] with small and highly isolated populations. There are only 200 individuals protected within the Campos del Tuyú National Park, in the Flooding Pampa. Habitat selection is affected by the presence of cattle, as deer prefer areas without them. However, when cattle are removed from plots, deer occupy them, suggesting that consumption is facilitated [65]. Research has demonstrated that large herbivores can enhance the consumption of small herbivores by consuming significant amounts of forage. This promotes the regrowth of higher quality tissues and improves the quality of the forage supply remaining for smaller herbivores [71]. The floristic composition and forage quality of two communities used by pampas deer were improved by the disturbance regime characteristic of CG, demonstrating the potential of CG to successfully address both productive objectives and the conservation of threatened herbivores [72].
Several farm-scale case studies have shown that CG enhances the diversity of birds and the presence of Pampas deer in livestock farms in the Flooding Pampa [73]. Therefore, CG can be considered a successful strategy for promoting wild fauna biodiversity.
Habitat Provision: Plant Diversity
The RPG are home of thousands of vascular plants species. About 8% of the species are endemic, while slightly more than 10% are naturalised exotic species [74]. The Flooding Pampa contains a total of 430 species, of which 21%–25% are naturalised exotic species, mostly of European origin. The native flora is composed of 141 species of grasses, 172 species of forbs, which only 15% being annuals. The annual species dominated the exotic flora, accounting for 72% of the total. Among them, annual forbs were the most prevalent, making up 58% [75].
Most exotic plants were likely introduced over 100 years ago [76]. The success of their invasion can be explained by their phylogenetic affinity and similarity on life forms to the native flora [77]. It is probably that most invasive species move from their native habitat to a similar one [78]. Invasion patterns are the result of interactions between the invasiveness of the available species, which is related to large seed size, and the invasibility of the community, which is related to its structure and disturbance [79]. Livestock grazing is considered a relevant disturbance that promotes the invasion of exotic species in Flooding Pampa grasslands [80].
The vegetation of the Flooding Pampa is arranged in complex mosaic of herbaceous plant communities. The species composition of these communities varies according to local variations in topography and soil salinity/alkalinity. The main communities are (i) Mesophytic meadows, which develop on positive and convex terrains, highest areas over typic Argiudolls soils; (ii) Humid mesophytic meadows, which develop on flat areas at intermediate topographic position over Natraquoll soils; (iii) Humid prairies, which develop on extended lowlands, with groundwater near the surface over Argialbolls soils; and (iv) Halophytic steppes, which develop on small depressions in flat areas, or rings surrounding humid areas over Mollic Natraqualfs soils [80]. Plant richness is highest in well-drained habitats at elevated topographic positions and declines towards lowland communities, where stressful conditions would determine that only a reduced number of species are able to establish [75].
The introduction of annual and exotic forbs due to continuous grazing by domestic herbivores has led to an increase in plant richness in the Flooding Pampa grassland [31,32]. Grazing prevents the dominance of a few tussock grasses and allows a diverse group of subordinate species to coexist at small scales [33]. Livestock grazing has been found to promote the introduction of exotic plant species and the coexistence of native ones, thereby enhancing plant diversity at the community level [80]. Furthermore, cattle dung serves as a vector for the dispersal of seeds of exotic species [81]. Consequently, certain exotic species such as L. multiflorum and Lotus tenuis have become stable and significant components of this grassland [82,83]. In the Ventania mountain system, which is another grassland relict in the Southern Pampa subregion, the maximum richness of a remnant of upland grassland was recorded at medium grazing intensities. This was due to a high number of forb species and winter grasses [84]. Additionally, at the landscape scale, continuous grazing has reduced the original heterogeneity of the grassland, resulting in a decrease in floristic diversity between communities ([75,80]).
The application of CG had a significant effect on the contribution of different functional groups in the most extensive communities. In the humid mesophytic meadows, CG increased the cover of legumes and C3 native and non-native grasses, both annual and perennial, while decreasing the cover of C4 prostate grasses and dicot forbs. In the humid prairie CG increased the cover of legumes and tussock hygrophytic grasses, while decreasing the cover of dicot forbs [48]. The floristic diversity remained unchanged in these non-halophytic communities. This was due to the reduction of dicots forbs and prostate grasses being offset by the concomitant increment in two non-native species of high forage value: L. multiflorum, a C3 annual grass with a high growing rate, and Lotus tenuis, a spring-summer legume [48]. CG promotes species of high forage value, mostly native, while reducing species of low or no forage value, mostly exotic. It also increases the contribution of cool-season forage species, resulting in a concomitant increase in carrying capacity (see section primary productivity and meat production). Additionally, the separation of areas with homogeneous vegetation (different communities), which is characteristic of this grazing method [34], helps to maintain the spatial heterogeneity of the grassland.
A long-term farm-scale case study demonstrated that in the halophytic communities, CG also caused favourable changes in vegetation structure. It increased vegetation cover and the contribution of warm and cool season native grasses, both annual and perennial, of higher forage value. Additionally, it encouraged the appearance of several grasses adapted to fertile, well-drained, and non-saline soils. As a result of these structural changes, species richness and diversity increased in this community [51]. The changes have been attributed to positive feedback, with increased organic carbon storage in soil resulting from increased fresh plant residue returns. This has led to an improvement in the physical environment of the topsoil due to the process of aggregate stabilisation. Furthermore, these beneficial changes are responsible for increased infiltration, which favours an incipient process of salt leaching and pH decreases [85].
The repeated use of glyphosate significantly changes the structure of vegetation in humid mesophytic meadows. It reduces species richness and diversity, causes the local extinction of several native perennial species, increases the basal cover of exotic cool-season annual grasses, and reduces the basal cover of cool-season perennial grasses, warm-season tussock grasses, warm-season legumes, and the vegetation cover in summer [40]. Furthermore, the intervention resulted in a significant alteration of the seed bank. Specifically, the density of cool-season annual grasses increased while that of cool and warm-season perennial grasses, sedges, legumes, and dicot herbs decreased. As a result, there was a decrease in both richness and diversity, and an increase in dominance [86].
3.4.2 Provision Ecosystem Services
Net Primary Productivity (NPP)
Mean annual precipitation is widely recognized as the primary driver of aerial net primary production (ANPP) in grasslands and shrublands [87,88]. This factor alone accounts for over 75% of the ANPP variation at a regional scale [89–91]. A regional scale estimation of ANPP of the Rio de la Plata grasslands during six years using remote sensing ranged from 5200 to 7860 kg ha−1 year−1, depending on the spatial location (subregions) (Table 1). The temporal variation of ANPP, ranged from 11% to 65% of the mean value of the ANPP for each location [92].
However, the differences among vegetation communities in the Flooding Pampa, which respond to soil traits and topographic position, result in different total annual and spatial patterns of ANPP [93,94]. The total annual ANPP estimated by biomass harvest ranges from 1500–2000 kg DM ha−1 year−1 for the most restricted community, the halophyte steppe, to 7500 kg DM ha−1 year−1 for the richest community, the mesophytic meadow, which is largely replaced by crops [33,93,95,96]. Intermediate values of ANPP are recorded in the humid mesophytic meadows, the most prominent community, ranging from 5800 to 4500 kg DM ha−1 year−1, and in the humid prairies, which reaches 6600 kg DM ha−1 year−1 [93,95,96]. However, estimations via remote sensing in the humid mesophytic meadow ranged from 6400–7200 kg DM ha−1 year−1 [97], and in the humid prairies found values around 4100–4500 kg DM ha−1 year−1 with an interannual variation ranging from 3993 to 4845 kg DM ha−1 year−1 [98] (Table 1).
In well-studied communities, grazing and other agricultural practices such as fertilization or herbicide spraying cause structural changes to vegetation cover, floristic composition, and functional groups. These changes, in turn, affects the quantity and seasonal pattern of primary productivity, as well as other ecosystem processes and services.
Grazing management has been found to modify the annual ANPP and the seasonal pattern of the humid mesophytic meadow. The ANPP for one year was 7200 kg DM ha−1 year−1 under grazing exclusion, 5700 kg DM ha−1 year−1 after mowing the vegetation of the exclosure, and 2250 kg DM ha−1 year−1 under continuous heavy grazing [33]. Two farm-scale experiments have shown that CG either increases annual ANPP or changes the seasonal pattern by increasing winter ANPP. The first experiment compared continuous grazing and CG at the same stocking rate during two consecutive years, one humid and one dry. The results found that CG modified the seasonal pattern of ANPP [47]. Winter forage productivity was 1377 and 1135 kg DM ha−1 year−1 under CG and 610 and 731 kg DM ha−1 year−1 under continuous grazing for the first and second year respectively, which represented an increase of 55%–125%. Summer forage productivity was 2127 and 1088 kg DM ha−1 year−1 under CG and 3250 and 1846 kg DM ha−1 year−1 under continuous grazing for the first and second year respectively. This represents a decrease of 52%–70%. An effective method to increase winter forage production is CG, which involves an intensive grazing period in early autumn to remove almost all available warm season biomass, followed by a resting period to allow seedling development and establishment of C3 grasses. The major contribution to winter forage production was L. multiflorum, which earlier establishment is the result of promoting germination through the perception of high red/far red ratios generated by very intense defoliation [47]. Therefore, it is recognized that CG may mitigate the negative impact of continuous grazing on winter forage availability in these grasslands. In the second experiment ANPP significantly increased in a humid mesophytic meadow that produced 2600 kg DM ha−1 year−1 under continuous grazing. After three years of applying CG, ANPP increased to 4800 kg DM ha−1 year−1 [99]. Both experiments were case studies in which CG was implemented at whole livestock farms, and the results were monitored for several years. Therefore, CG can be considered a successful strategy for increasing winter forage production and enhancing the seasonal pattern of ANPP.
The ANPP of halophytic steppes varied depending on the grazing method employed. Traditional continuous grazing resulted in an ANPP range of 2100 [93] to 2969 kg DM ha−1 year−1 [96]. Heavy continuous grazing decreased the ANPP to 1564 kg DM ha−1 year−1 [100]. After a minimum of seven years of applying CG, the ANPP increased to 4700 ± 797 kg DM ha−1 year−1, while grazing exclusion for seven to seventeen years allowed for an ANPP of 6274 ± 978 kg DM ha−1 year−1 [100] (Table 1). This study-case conducted at farm-scale demonstrated that even in this marginal community with high soil limitations, CG and grazing exclusion increases primary production and triggers positive ecosystem processes that reverses continuous grazing deterioration [51,85].
A two-year experiment demonstrated that spraying glyphosate in the humid mesophytic meadows community in late summer resulted in a reduction of the annual ANPP by approximately 16%–25% (3350 and 2918 kg DM ha−1 year−1 for the first and second year, respectively) compared to the unsprayed community (4005 and 3871 kg DM ha−1 year−1 for the first and second year respectively) [41] (Table 1). Between June and September, ANPP increased by 100% to 500%. However, during spring, summer, and autumn ANPP was 22% to 90% lower than the unsprayed grassland. Glyphosate spraying caused a significant alteration in the seasonal pattern of ANPP. This change in the seasonal pattern resulted from the reduced contribution of warm-season grasses and legumes, which are severely impacted by glyphosate spraying in late summer, when they are actively growing. Therefore, the higher forage supply in winter was unable to compensate for the reduction during the rest of the year. This may explain the recent trend of increasing the area cultivated with summer forage crops in the last years in the Flooding Pampa [41].
Fertilization with phosphate alone or in combination with nitrogen increased ANPP during both the cool and the warm seasons in the humid mesophytic meadows. This effect was observed at both plot-scale without grazing [101] and at farm-scale under CG [102] (Table 1). CG involves an intensive grazing period in early autumn to provide better growing conditions to C3 grasses by removing almost all available warm season biomass [47,48]. The release of competition and the provision of phosphorus alone or with nitrogen resulted in the promotion of C3 annual grasses and a significant increase in legume contribution, particularly Lotus tenuis. The annual ANPP of the humid mesophytic meadows increased by 30% to 100% compared to the control treatment, depending on the dose of fertilizer and the interannual variation of precipitation [102]. This community was fertilized for four years with 10 gm−2 year−1 NPK + micronutrients. It was then compared between seasonal grazing (rest during winter and spring and grazing during summer and autumn) and the grazing exclusion. The results showed that fertilization did not affect ANPP under exclosure, while it increased the ANPP of the seasonal grazing treatment by up to 103% [103].
Meat and Wool Production
ANPP is the primary factor determining of livestock biomass, particularly cattle and sheep, in South America [104]. Therefore, secondary production, including meat, milk, and wool, is also reliant to ANPP. While the conversion of photosynthetically active solar energy into net secondary production is inefficient, it is the sole means of converting the energy contained in rangelands vegetation, which is not suitable for human consumption, into animal products that are highly nutritious for humans. Globally, rangeland ecosystems provide 75 trillion MJ of animal products annually, assuming a utilization efficiency of 20% and a conversion efficiency of 2% of the consumed biomass into animal products. This value could potentially increase with improved management strategies that enhance energy capture, utilization efficiency, or conversion efficiency of consumed biomass to animal products [105].
The estimated production of meat equivalent (meat + milk + wool) in the RPG is 78 kg live weight ha−1 year−1, with a spatial range from 92.5 to 35.2 kg live weight ha−1 year−1 [1]. These estimations are consistent with historical data on meat production in the Flooding Pampa subregion, which ranged from 60 to 80 kg live weight ha−1 year−1 [106,107]. In 2019, the cattle stock in the Flooding Pampa was 8727.092 animals, with 6.674.346 falling under the cow-calf operation categories (cows, heifers, calves, and bulls). This represents 16% of the country’s stock and 46% of the Buenos Aires province [108]. We estimated the total volume of meat equivalent production in the Flooding Pampa subregion applying the procedure proposed in [1] which yields a value of 4560.000 t of live weight year−1. Multiplying the number of weaned calves derived from backgrounding per year in the subregion yields a similar value of 460.000 t of live weight year−1. This volume represents 15% of the total slaughtered bovine in 2022 [109], which feeds 9 million of Argentinian people per year, based on a per capita beef meat consumption of 51 kg year−1 [109]. In 2022, the sheep livestock stock of Flooding Pampa was around 781,000 animals [108], which produced 2132 tons of greasy wool [110].
Meat production in the Flooding Pampa is highly dependent on the livestock production model, including livestock system, inputs level, and grazing method. The suitability of the environment, as indicated by the relative proportions of the different communities, determines the achievable meat production. The model characterised by the predominant use of natural grassland in good condition through CG (low input farms) significantly increases meat production compared to Continuous Grazing. This is a consequence of higher PPNA and/or higher zootechnical value of the grassland under CG [50,99]. In pure cow-calf farming systems with no agricultural environment (mesophytic meadows) and therefore with grassland covering the entire area, CG allows for the achievement of 100 kg live weight ha−1 year−1. In backgrounding farming systems where up to 25% of the agricultural environment is replaced by forage crops and the CG allows the achievement of 200 kg live weight ha−1 year−1. The conversion of a small area to pasture on suitable soils not only results in the provision of higher-quality forage but also allows for more effective management of the natural grassland, as it affords greater flexibility in determining rest periods. Full cycle farming systems, which have a larger area of pasture and forage crops and use feedlot finishing, while continuously grazing the remaining grassland, achieve a live weight of 360 kg ha−1 year−1. However, it has been found that increased meat production in systems that replace more than 25% of the grassland (high input farms) significantly increases fossil energy consumption and reduces energy use efficiency [50]. On a farm undergoing agroecological conversion, meat production increased from 91 to 182 kg. live weight ha−1 year−1 after 3 years of being implemented CG and sowing 15% of the area with forage crops, resulting in a significant increase in gross margin [99]. The CG model yields higher profit, income stability and gross margin compared to the continuous grazing model. The latter lacks economic viability, even under different input-output price ratio scenarios [35]. These models are based on real farming systems and supported by empirical data, making them valuable case studies to highlight the CG as a suitable strategy for enhancing several provision services such as primary productivity and meat production.
Genetic Resources
The Flooding Pampa grasslands provide native genetic resources, allowing the identification of variability in different plant species traits. These are sources of genes for breeding programmes and to restore degraded habitats.
Two grass species, Elymus scabrifolius and Stapfochloa berroi (formerly Chloris berroi), are being studied for their potential to tolerance to saline–alkaline conditions [111,112]. These grasses are native to South America and have high forage value. E. scabrifolius is a perennial cool-season grass while S. berroi is a perennial warm-season grass. They are rarely found under continuous grazing due to their highly preference by herbivores. S. berroi is a significant component of the halophytic steppes under CG or grazing exclosure [51]. Meanwhile, E. scabrifolius is found in the upland communities of the Samboromboy Bay under seasonal grazing exclusion [73].
Two other native cool-season grasses which belong to the mesophytic and humid mesophytic meadows, Bromus catharticus and Bromus auleticus, are highly productive and digestible, and have been extensively studied due to their widespread use as forage resources. Bromus catharticus is an annual/biennial native fodder grass. Its high genetic diversity has allowed for the development of breeding programmes to obtain diverse commercial cultivars [113]. Bromus auleticus is a perennial native grass with high genetic diversity and potential for use in breeding cultivars and restoring degraded grasslands [114]. Both species were promoted in the upland communities of the Samborombom Bay under seasonal grazing exclusion [73].
Paspalum dilatatum is a perennial warm-season C4 grass native to South America. It is a primary component of the humid mesophytic meadows and humid prairies communities of the Flooding Pampa grasslands, providing high-quantity forage in large quantities during the warm-season [41]. P. dilatatum is more tolerant to frosts than other C4 grasses and can adapt to various edaphic and environmental conditions. Additionally, it is resistant to defoliation and has excellent regrowth capacity. Several cultivars were developed through conventional breeding in Argentina [115–117] and Australia. Currently, efforts are focused on transgenic and genome editing approaches [118].
3.4.3 Regulating Ecosystem Services
Climate regulation
Research on climate regulation in the Flooding Pampa grassland region has focused on estimating the carbon footprint, modelling strategies to reduce greenhouse gas emissions, estimating the greenhouse gas balance, and measuring changes in soil SOC stocks due to management practices.
SOC stocks are considered an intermediate ecosystem service that contributes to climate regulation. Grasslands are a crucial ecosystem for carbon storage, complementing forests in the fight against climate change [119]. However, grasslands’ contributions to human and planetary well-being have been widely overlooked, and their preservation, conservation, and restoration are of utmost importance. The global success of carbon sequestration depends on the complete and effective restoration of grassland ecosystems [120]. The RPG are an important carbon sink, estimated to store 5% of Latin America’s total soil carbon, despite covering less than 3% of the subcontinent’s total area [121].
Management practices change soil stocks in the Flooding Pampa grasslands. Soil organic matter levels were significantly higher in healthy native tall tussock grasslands compared to sown pastures [122]. Additionally, the application of glyphosate to promote L. multiflorum resulted in a reduction of SOC by 1,058 t ha−1 year−1 [41]. In a review at global level, grazing has been shown to have a negative effect on soil organic carbon (SOC) compared to non-grazed situations [123]. However, in the Flooding Pampa grazing promotes carbon accumulation compared to non-grazed systems [124,125]. The method of grazing significantly affects SOC stocks. CG has been found to increase soil C stocks by 1.48 t ha−1 year−1 in the lowland alkaline sites occupied by the halophytic steppe community [100] and by 0.539 t ha−1 year−1 in the highland or intermediate sites, occupied by the humid mesophytic meadow community [41] when compared to continuous grazing.
Using whole-farm modelling, the carbon footprint of cow-calf systems in the Flooding Pampa was estimated to be between 19–22 t CO2-eq/t LW [126–128]. When estimating the carbon footprint of real farms values ranged from 12.66 to 16.89 t CO2-eq/t LW [126]. Mitigation strategies for Flooding Pampa have been proposed through production intensification. The modelling of GHG emissions from cow-calf farms revealed that systems that include backgrounding with grain supplementation emit less GHG per unit of product than pure cow-calf systems [128]. Additionally, incorporating 10% of the farm area into fescue pasture and advancing the first service of heifers (from 27 to 15 months) reduced GHG intensity emissions by up to 17% [126].
Two models of livestock systems were found in the Flooding Pampas based on empirical data obtained from several real livestock farming systems used as case studies. The estimation of GHG balance showed a significant difference between the two models. The model characterized by the predominant use of natural grassland through CG (grassland system) emitted 2273 kg CO2 eq·ha−1 year−1 and sequestered carbon at a rate of 1851 kg CO2 eq·ha−1 year−1. However, the model associated with a greater expanse of pasture and forage crops as well as a higher animal stocking rate (intensive system), emitted 4500 kg CO2 eq·ha−1 year−1 and reduced SOC at a rate of 601 kg CO2 eq·ha−1 year−1. The lower GHG emissions of the grassland system were attributed to the lower emissions of CH4 and N2O resulting from the lower stocking rate. The elevated carbon sequestration observed in the grassland system was attributed to an increase in carbon sequestration capacity of the grassland in good condition, resulting from the implementation of CG. Consequently, the GHG balance was approximately ten times more negative in the intensive system than in the CG grassland system, which was nearly neutral [129].
A single measurement experiment was conducted to compare N2O winter emissions in soils with and without grazing in different patches of grazed Flooding Pampa grasslands. The emissions of N-N2O from the urine patches were higher than those from faeces and control treatments, with average values of 26.13, 5.35 and 2.88 µg N-N2O/m2h, respectively. Additionally, the N-N2O emissions increased after rainfall [130].
Nutrient Cycling and Erosion Control
Soil nitrogen (N) content is influenced by both plant community and grazing treatment. In the lowland community, where high C content is likely due to the export of dissolved nutrients from upland to lowland sites, continuous grazing increased N content with respect to exclosure conditions [124]. The significantly lower amount of C and N measured in this community soil under exclosure reflected a major change in the pattern of element cycling, which resulted in large quantities of C and N flowing through the aboveground vegetation pathway in absence of domestic herbivores [124]. However, in the upland community continuous grazing either had no effect on the mineral N content of the soil [124] or decreased it [131]. The extractable phosphorus (P) content did not change under continuous grazing [127] or decreased with respect to the grazing exclusion [131].
Continuous grazing affects nutrient cycling by altering both the floristic composition of the vegetation and the soil conditions for decomposition. Forbs dominate continuously grazed grasslands and have higher litter quality than grasses that dominate ungrazed grasslands [132]. Grazed soil environments increase decomposition rates relative to those without grazing [133]. The application of glyphosate significantly decreased the total soil P content and had a tendency to reduce soil N content when compared to unsprayed grasslands. This is due to changes in vegetation function and structure, which may alter litter quantity and quality [41].
The application of CG significantly improved soil physical properties related to erosion control. In the upland community, topsoil had 16% lower bulk density and 54% lower surface bearing capacity compared to continuous grazing [134]. A long-term case study of a livestock farming system with a high proportion of alkaline lowland soils demonstrated that CG improved the physical properties of this community by reducing bulk density, structural instability, salinity and bearing capacity of the topsoil [85].
An experiment was conducted to estimate the sediment concentration of runoff in grasslands of different conditions in a catchment of Rolling Pampa subregion using simulated rainfall. The results showed that sediment concentration was 3.5 times higher in degraded grasslands than in healthy ones [135].
Water Regulation
Changes in land use, such as cropping or afforestation, have affected the regulating services provided by Flooding Pampa grasslands at the landscape scale. A catchment study that includes part of the Flooding Pampa found a strong direct relationship between the loss of grasslands to cropping and the reduction in in lentic water bodies [136]. Afforestation resulted in increased water uptake, which lowered groundwater levels by about 0.5 m compared to the surrounding grassland [137].
At a plot scale, the vegetation structure had an impact on the water dynamic of the grassland. The infiltration rate was significantly higher, and the bulk density was lower in the native tall tussock grasslands compared to the native short-grass grasslands of the Flooding Pampa. Furthermore, the simulation of water dynamics under drought conditions indicated that transpiration from native tall tussock was higher and evaporation was lower than that from native short grass. This reflects the high productivity of native tall tussock grasslands under drought conditions. Conversely, under surplus water conditions, transpiration and evaporation were higher from native short grass than from native tall tussock, suggesting a lower capacity of native tall tussock to remove surplus water [122].
The grazing method affects plant and litter cover, which in turn affect water dynamics through evapotranspiration fluxes. In the Flooding Pampa, litter cover is significantly increased by CG compared to continuous grazing in all the communities studied [48,51]. In the upland community, CG significantly increased the gravimetric water content [134]. In the halophyte steppe, the community with fewer resource, CG reduced bare soil from 71% under continuous grazing to 43%, due to a significant increase in plant cover [51]. Higher litter and plant cover in these halomorphic soils lead to a reduction in water loss by evaporation, which improves the hydric and thermal regime of the soil and thus reduces the risk of salinization [138]. This triggers a positive feedback loop between improvements in soil and plant condition.
Cultural ecosystem services are defined as the multiple intangible values and non-material benefits provided by ecosystems through experiences, recreation and tourism that enhance human well-being by satisfying physiological, psychological and emotional needs [139]. Grasslands provide important cultural services such as cultural heritage, spiritual, aesthetic and social cohesion; tourism and recreation; education and scientific study [14]. However, relevant studies on cultural ecosystem services are relatively scarce and mainly cover Europe and North America [140].
Protected areas of varying conservation status have been established in the Flooding Pampa to ensure the long-term conservation of the grassland pampa biome and its cultural diversity. These public protected areas cover 32,534 ha and are visited for recreational activities such as bird watching and hiking, for educational purposes and for scientific studies [141]. However, only a very small percentage, 0.36%, of the grasslands in the Flooding Pampa are under public protection [73].
In recent years, the increasing preference of local tourists by natural and semi-natural environments for recreation has coincided with the interest of livestock farmers to conserve native grasslands under grazing for livestock production and, at the same time, to include rural tourism as an alternative source of economic income [142]. Several examples of livestock farms in the Flooding Pampa that have been incorporated into the Wildlife Refuge Network, conserve almost 8000 hectares of native animals and plants of the grassland communities [73,143].
Livestock farming in Pampa grassland is an ancient and deeply rooted cultural heritage, dating back to the origin of Argentina. The social cohesion around this activity and the traditional celebrations related to animal husbandry are relevant in each location of the Flooding Pampa. The traditional herding system for cattle keeping is an integral part of social cohesion in rural landscapes [144,145].
Anthropogenic interventions such as land use change, continuous grazing, and glyphosate spraying, as well as increased stocking rates, have caused severe degradation of the Flooding Pampa grasslands. Consequently, the ecosystem services provided by the grasslands are declining, including provisioning services (despite increased inputs, current meat production is low) and regulating and supporting services. The study of ecosystem services in this region has been primarily concerned with the impact of drivers on floristic and animal diversity as well as on primary production. There has been considerably less research conducted on other services, including climate regulation, water provision, nutrient cycling, meat production, and soil erosion. Furthermore, there is a significant lack of research on the cultural services that connect society and nature and promote a comprehensive public understanding and protection of ecosystems.
Controlled Grazing, AMPG’s system design for managing Flooding Pampa grasslands, and conservative stocking rates has been shown to reverse degradation and to make compatible production and economic profits with ecosystem conservation. Nevertheless, further research is required to ascertain the circumstances that impede the transmission of such insights to producers, with the objective of implementing them in practical applications. Moreover, future research directions should focus on the impact of livestock farming management practices, such as grazing methodology and stocking rate, as well as those applied to enhance grassland production or quality, such as fertilization, weed control, burning, interseeding, water systematization, and afforestation, on C sequestration, water dynamics, nutrient cycling, and plant and animal diversity. This research should be conducted at the farm-scale and over the long term.
Acknowledgement: The authors thank the anonymous reviewers, whose comments improved the quality of the paper.
Funding Statement: The authors received no specific funding for this study.
Author Contributions: Both authors confirm equal contribution to the paper: designed the literature search, wrote and organization of the manuscript. All authors reviewed the results and approved the final version of the manuscript.
Availability of Data and Materials: Not applicable.
Ethics Approval: Not applicable.
Conflicts of Interest: The authors declare that they have no conflicts of interest to report regarding the present study.
1We named “natural grassland” to those old-growth grasslands, which are ancient ecosystems characterized by high herbaceous species richness, high endemism, and unique species compositions, which biodiversity is maintained by frequent fires or grazing. While “seminatural grassland” are those “secondary grassland”, which are novel, anthropogenic young ecosystems derived from agriculture or other anthropogenic intervention that modified their original composition [9].
References
1. Paruelo JM, Oesterheld M, Altesor A, Piñeiro G, Rodríguez C, Baldassini P, et al. Herbivores and fires: their role in shaping the structure and functioning of the grasslands of the Río de la Plata. Ecol Austral. 2022;32(2):784–805 (In Spanish). doi:10.25260/EA.22.32.2.1.1880. [Google Scholar] [CrossRef]
2. Oyarzabal M, Andrade B, Pillar VD, Paruelo J. Temperate subhumid grasslands of southern South America. In: Goldstein MI, DellaSala DA, editors. Encyclopedia of the World’s Biomes. New York: Elsevier. 2019;3:577–93. [Google Scholar]
3. Soriano A, León RJC, Sala OE, Lavado RS, Deregibus VA, Cahuepe MA, et al. Río de la Plata grasslands. In: Coupland RT, editor. Ecosystems of the world 8A. Natural grasslands. Introduction and western hemisphere. New York: Elsevier; 1992. p. 367–407. [Google Scholar]
4. Sokolowski AC, Álvarez VE, Mangiarotti A, Rodríguez HA, Barrios MB, Prack McCormick B, et al. Multidimensional performance of periurban horticulture: assessing agroecological transition and soil health. Agroecol Sustain Food Syst. 2024;48(2):281–310. doi:10.1080/21683565.2023.2279972. [Google Scholar] [CrossRef]
5. Lara B, Gandini M. Quantifying the land cover changes and fragmentation patterns in the Argentina Pampas, in the last 37 years (1974–2011). GeoFocus. 2014;14:163–80. [Google Scholar]
6. Fernández LM, Della CT, Peretti M, Lúgato T, Figueiras E, Calabrese L, et al. Agroclimatic and Environmental Information Center (In Spanish). Available from: https://ciag.agro.uba.ar/. [Accessed 2024]. [Google Scholar]
7. Lavado RS. Río de la Plata Grasslands. Soils. In: Couplan RT, editor. Ecosystems of the world 8A. Natural grasslands. Introduction and western hemisphere. New York: Elsevier; 1992. p. 377–80. [Google Scholar]
8. Batista WB, Taboada MA, Lavado RS, Perelman S, León RJC. Association between plant communities and soils in the Flooding Pampa grassland. In: Oesterheld M. Aguiar MR, Ghersa CM, Paruelo JM, editors. The heterogeneity of the vegetation of agroecosystems. Buenos Aires: Editorial Faculty of Agronomy; 2005. p. 113–29 (In Spanish). [Google Scholar]
9. Veldman JW, Buisson E, Durigan G, Fernandes GW, Le Stradic S, Mahy G, et al. Toward an old-growth concept for grasslands, savannas, and woodlands. Front Ecol Environ. 2015;13(3):154–62. doi:10.1890/140270. [Google Scholar] [CrossRef]
10. Vázquez P, Rojas MDC. Agroecological zoning of the area of influence of the EEA Cuenca del Salado. Buenos Aires: INTA Editions; 2006. p. 14 (In Spanish). Technical Publication No. 2, INTA EEA Cuenca del Salado. [Google Scholar]
11. Modernel P, Rossing WAH, Corbeels M, Dogliotti S, Picasso V, Tittonell P. Land use change and ecosystem service provision in Pampas and Campos grasslands of southern South America. Environ Res Lett. 2016;11(11):3002. doi:10.1088/1748-9326/11/11/113002. [Google Scholar] [CrossRef]
12. Sala OE, Paruelo JM. Ecosystem services in grasslands. In: Daily G, editor. Nature’s services: societal dependence on natural ecosystems. Washington: Island Press; 1997. p. 237–51. [Google Scholar]
13. Fisher B, Turner RK, Morling P. Defining and classifying ecosystem services for decision making. Ecol Econ. 2009;68(3):643–53. doi:10.1016/j.ecolecon.2008.09.014. [Google Scholar] [CrossRef]
14. Bengtsson J, Bullock JM, Egoh B, Everson C, Everson T, O’Connor T, et al. Grasslands—more important for ecosystem services than you might think. Ecosphere. 2019;10(2):e02582. doi:10.1002/ecs2.2582. [Google Scholar] [CrossRef]
15. Teague R, Kreuter U. Managing grazing to restore soil health, ecosystem function, and ecosystem services. Front Sustain Food Syst. 2020;4:534187. doi:10.3389/fsufs.2020.534187. [Google Scholar] [CrossRef]
16. Xiao B, Zhao LJ, Zheng LP, Tan L, Zheng FL, Siya·Man Like A. Review of the impact of grassland degradation on ecosystem service value. Open J Appl Sci. 2022;12(7):1083–97. doi:10.4236/ojapps.2022.127074. [Google Scholar] [CrossRef]
17. Westoby M, Walker B, Noy-Meir I. Opportunistic management for rangelands not at equilibrium. J Range Manag. 1989;42(4):266–74. doi:10.2307/3899492. [Google Scholar] [CrossRef]
18. Laycock WA. Stable states and thresholds of range condition on North American rangelands: a viewpoint. J Range Manag. 1991;44(5):427–33. doi:10.2307/4002738. [Google Scholar] [CrossRef]
19. Milchunas DG, Sala OE, Lauenroth WK. A generalized model of effects of grazing by large herbivores on grassland community structure. Am Nat. 1988;132(1):87–106. doi:10.1086/284839. [Google Scholar] [CrossRef]
20. Cingolani AM, Noy Meir I, Díaz S. Grazing effects on rangeland diversity: a synthesis of contemporary models. Ecol Appl. 2005;15(2):757–73. doi:10.1890/03-5272. [Google Scholar] [CrossRef]
21. MacFadden BJ. Diet and habitat of toxodont megaherbivores (Mammalia, Notoungulata) from the late Quaternary of South and Central America. Quat Res. 2005;64(2):113–24. doi:10.1016/j.yqres.2005.05.003. [Google Scholar] [CrossRef]
22. Strömberg CAE. Evolution of grasses and grassland ecosystems. Annu Rev Earth Planet Sci. 2011;39(1):517–44. doi:10.1146/annurev-earth-040809-152402. [Google Scholar] [CrossRef]
23. Prado J, Martínez-Maza C, Alberdi MT. Megafauna extinction in South America: a new chronology from the Argentine pampas. Palaeogeogr Palaeoclimatol Palaeoecol. 2015;425(2):41–9. doi:10.1016/j.palaeo.2015.02.026. [Google Scholar] [CrossRef]
24. Díaz S, Lavorel S, McIntyeare S, Falczuk V, Casanoves F, Milchunas DG, et al. Plant trait responses to grazing—a global synthesis. Glob Chang Biol. 2007;13(2):313–41. doi:10.1111/j.1365-2486.2006.01288.x. [Google Scholar] [CrossRef]
25. Cingolani AM, Noy Meir I, Renison D, Cabido M. Is extensive livestock farming compatible with the conservation of biodiversity and soils? Austral. 2008;(3):253–71 (In Spanish). [Google Scholar]
26. Martínez RD, Fernández EN, Género ER, Rumiano FJL. Creole cattle in Argentina. Archi Zootec. 2000;49(187):353–61 (In Spanish). [Google Scholar]
27. Lebedinsky M. Problems of our time. Buenos Aires: Quipo; 1969. p. 172 (In Spanish). [Google Scholar]
28. Hall AJ, Rebella CM, Ghersa CM, Culot JP. Field-crop systems of the Pampas. In: Pearson CJ, editor. Ecosystems of the wrld. Amsterdam: Elsevier; 1992. p. 413–49. [Google Scholar]
29. Baeza S, Vélez-Martin E, De Abelleyra D, Banchero S, Gallego F, Schirmbeck J, et al. Two decades of land cover mapping in the Río de la Plata grassland region: The MapBiomas Pampa initiative. Remote Sens Appl: Soc Environ. 2022;28:100834. [Google Scholar]
30. Lara B, Gandini M, Salese S. Changes in regulation ecosystem services and their relationship with changes in land use in the Pampas region (Argentina). Rev Cha Se Cie For y del Amb. 2024;29(3):3–16 (In Spanish). [Google Scholar]
31. Sala OE, Oesterheld M, León RJC, Soriano A. Grazing effects upon plant community structure in subhumid grasslands of Argentina. Vegetatio. 1986;67(1):27–32. doi:10.1007/BF00040315. [Google Scholar] [CrossRef]
32. Facelli JM. Response to grazing after nine years of cattle exlusion in a Flooding Pampa grassland. Argentina Vegetatio. 1988;78(1–2):21–5. doi:10.1007/BF00045635. [Google Scholar] [CrossRef]
33. Rusch GM, Oesterheld M. Relationship between productivity, and species and functional group diversity in grazed and non-grazed Pampas grassland. Oikos. 1997;78(3):519–26. doi:10.2307/3545613. [Google Scholar] [CrossRef]
34. Deregibus VA, Jacobo EJ, Rodríguez AM. Improvement in rangeland condition of the Flooding Pampa of Argentina through controlled grazing. Afr J Range Forage Sci. 1995;12(2):92–6. doi:10.1080/10220119.1995.9647873. [Google Scholar] [CrossRef]
35. Martínez Ortiz U, Jacobo EJ, Cañada P, Sobredo M. Economic analysis of the management of natural grasslands in the Salado Basin. Buenos Aires: Technical Bulletin of the Argentine Wildlife Foundation; 2017 Dec (In Spanish). Available from: https://wwfar.awsassets.panda.org/downloads/kit_pampas___manual_analisis_economico_del_manejo_de_pastizales.pdf. [Accessed 2023]. [Google Scholar]
36. Trigo EJ, Cap EJ. The impact of the introduction of transgenic crops in Argentinean agriculture. AgBioForum. 2003;6:87–94. [Google Scholar]
37. Satorre EH. Technological changes in current Argentine agriculture. Ciencia Hoy. 2005;15(87):24–31 (In Spanish). [Google Scholar]
38. Paruelo JM, Guerschman JP, Verón SR. Agricultural expansion and changes in land use. Ciencia Hoy. 2005;15(87):14–23 (In Spanish). [Google Scholar]
39. Bilello G, Zeberio G. Technological incorporation in family-type livestock farms in the Salado Basin. Control of red straw (Paspalum quadrifarium) and rejuvenation of rye-grass in natural grasslands. Rev Fac Agron. 2002;22:107–20 (In Spanish). [Google Scholar]
40. Rodríguez AM, Jacobo EJ. Glyphosate application changes plant functional groups proportion and reduces floristic richness and diversity in flooding pampa rangeland (Argentina). Agric Ecosyst Environ. 2010;138:222–31. [Google Scholar]
41. Rodríguez AM, Jacobo EJ, Golluscio RA. Glyphosate alters aboveground net primary production, soil organic carbon, and nutrients in pampean grasslands (Argentina). Range Ecol Manag. 2018;71(1):119–25. doi:10.1016/j.rama.2017.07.009. [Google Scholar] [CrossRef]
42. Faverin C, Machado C. Typologies and characterization of cow-calf system the flooding pampas. Chilean J Agric Anim Sci. 2019;35(1):3–13. [Google Scholar]
43. Biondini ME, Manske L. Grazing frequency and ecosystem processes in a northern mixed prairie, USA. Ecol Appl. 1996;6(1):239–56. doi:10.2307/2269567. [Google Scholar] [CrossRef]
44. Teague WR, Dowhower SL, Baker SA, Haile N, DeLaune PB, Conover DM. Grazing management impacts on vegetation, soil biota and soil chemical, physical and hydrological properties in tall grass prairie. Agric Ecosyst Environ. 2011;141(3–4):310–22. doi:10.1016/j.agee.2011.03.009. [Google Scholar] [CrossRef]
45. Dahl KM, Bork EW, Parkins JR, Sherren K. Assessing variation in range health across grazed northern temperate grasslands. Rangel Ecol Manag. 2021;74:135–46. doi:10.1016/j.rama.2020.08.007. [Google Scholar] [CrossRef]
46. Sanjari G, Ghadiri H, Ciesiolka CA, Yu B. Comparing the effects of continuous and time-controlled grazing systems on soil characteristics in Southeast Queensland. Soil Res. 2008;46(4):348–58. doi:10.1071/SR07220. [Google Scholar] [CrossRef]
47. Jacobo EJ, Rodríguez AM, Rossi JL, Salgado LP, Deregibus VA. Rotational stocking and production of Italian ryegrass on Argentinean rangelands. J. Range Manage. 2000;53(5):483–8. doi:10.2307/4003648. [Google Scholar] [CrossRef]
48. Jacobo EJ, Rodríguez AM, Bartoloni N, Deregibus VA. Rotational grazing effects on rangeland vegetation at a farm scale. Range Ecol Manage. 2006;59(3):249–57. doi:10.2111/05-129R1.1. [Google Scholar] [CrossRef]
49. Sherren K, Hodbod J, MathisonSlee M, Chappell E, King M. Adaptive multi-paddock grazing and wellbeing: uptake, management practices and mindset among Canadian beef producers. Agroecol Sustain Food Syst. 2022;46(9):1304–29. doi:10.1080/21683565.2022.2107597. [Google Scholar] [CrossRef]
50. Jacobo EJ, Rodríguez AM, González JH, Golluscio RA. Effects of livestock intensification on fossil energy use efficiency and rangeland conservation in the lower basin of the Salado River, Buenos Aires province, Argentine. Agriscientia. 2016;33(1):1–14. doi:10.31047/1668.298x.v33.n1.16567. [Google Scholar] [CrossRef]
51. Vecchio MC, Bolaños VA, Golluscio RA, Rodríguez AM. Rotational grazing and exclosure improves grassland condition of the halophytic steppe in flooding pampa (Argentina) compared with continuous grazing. Rangel J. 2019;41(1):1–12. doi:10.1071/RJ18016. [Google Scholar] [CrossRef]
52. Costanza R, de Groot R, Braat L, Kubiszewski I, Fioramonti L, Sutton P, et al. Twenty years of ecosystem services: how far have we come and how far do we still need to go? Ecosyst Serv. 2017;28(A):1–16. [Google Scholar]
53. Medan D, Torretta JP, Hodara K, de la Fuente EB, Montaldo NH. Effects of agriculture expansion and intensification on the vertebrate and invertebrate diversity in the Pampas of Argentina. Biodivers Conserv. 2011;20:3077–100. [Google Scholar]
54. Codesido M, González Fischer C, Bilenca D. Land use patterns and bird assemblages in agroecosystems of the Pampean region, Argentina. Ornitol Neotrop. 2008;19:575–85. [Google Scholar]
55. Gavier-Pizarro GI, Calamari NC, Thompson JJ, Canavelli SB, Solari LM, Decarre J, et al. Expansion and intensification of row crop agriculture in the Pampas and Espinal of Argentina can reduce ecosystem service provision by changing avian density. Agricul Ecosyst Environ. 2012;154:44–55. [Google Scholar]
56. Codesido M. Birds of the pampas and fields. El Hornero. 2013;28(2):90–101 (In Spanish). [Google Scholar]
57. Isacch JP, Cardoni DA, Iribarne OO. Diversity and habitat distribution of birds in coastal marshes and comparisons with surrounding upland habitats in southeastern South America. Estuaries Coast. 2014;37:229–39. [Google Scholar]
58. Isacch JP, Cardoni DA. Different grazing strategies are necessary to conserve endangered grassland birds in short and tall salty grasslands of the flooding pampas. Condor. 2011;113(4):724–34. doi:10.1525/cond.2011.100123. [Google Scholar] [CrossRef]
59. Pretelli MG, Isacch JP, Cardoni DA. Year-round abundance, richness and nesting of the bird assemblage of tall grasslands in the south-east Pampa’s region, Argentina. Ardeola. 2013;60(2):327–43. doi:10.13157/arla.60.2.2013.327. [Google Scholar] [CrossRef]
60. Isacch JP, Martínez MM. Habitat use by non-breeding shorebirds in flooding pampas grasslands of Argentina. Waterbirds. 2003;26(4):494–500. doi:10.1675/1524-4695(2003)026[0494:HUBNSI]2.0.CO;2. [Google Scholar] [CrossRef]
61. Codesido M, González Fischer C, Bilenca D. Agricultural land-use, avian nesting and rarity in the Pampas of central Argentina. Emu. 2012;112(1):46–54. doi:10.1071/MU11049. [Google Scholar] [CrossRef]
62. Codesido M, Bilenca D. Avian assemblages associated with different grasslands managements in cattle production systems in the pampas of Argentina. Perspect Ecol Conserv. 2021;19(4):464–74. doi:10.1016/j.pecon.2021.07.003. [Google Scholar] [CrossRef]
63. Vaccaro AS, Dodyk L, Lapido R, Miguel AD, Grilli P. How does the grassland alliance contribute to the conservation of birds in the Flooding Pampa? El Hornero. 2020;35(2):95–110 (In Spanish). [Google Scholar]
64. Pereira J, Varela D, Fracassi N. The Pampas cat in Argentina: is it absent from the Pampas? Cat News. 2002;36:20–2. [Google Scholar]
65. Vila AR, Beade MS, Barrios Lamunière D. Home range and habitat selection of pampas deer. J Zool. 2008;276(1):95–102. doi:10.1111/j.1469-7998.2008.00468.x. [Google Scholar] [CrossRef]
66. Serafini VN, Priotto JW, Gomez MD. Effects of agroecosystem landscape complexity on small mammals: a multi-species approach at different spatial scales. Landsc Ecol. 2019;34(5):1117–29. doi:10.1007/s10980-019-00825-8. [Google Scholar] [CrossRef]
67. Aranguren MF, Velasco MA, Trofino-Falasco C, Pizzarello MG, Vera DG, Berkunsky I. Mammals of the Tandilia Mountain system, current species inhabiting Pampean highland grasslands. Neotropical Biol Conserv. 2023;18(1):13–29. doi:10.3897/neotropical.18.e98374. [Google Scholar] [CrossRef]
68. Gantchoff MG, Belant JL, Masson DA. Land use affects mammal community composition in a multiple use protected area, east-central Argentina. Latin Am J Conserv. 2014;4:7–13. [Google Scholar]
69. Luza AL, Trindade JPP, Maestri R, da Silva DL, Hartz SM. Rodent occupancy in grassland paddocks subjected to different grazing intensities in South Brazil. Perspect Ecol Conserv. 2018;16(3):151–7. doi:10.1016/j.pecon.2018.06.006. [Google Scholar] [CrossRef]
70. Ojeda RA, Chillo V, Díaz Isenrath GB. Red book of endangered mammals of Argentina. Buenos Aires: Argentine Society for the Study of Mammals; 2012 (In Spanish). [Google Scholar]
71. McNaughton SJ. Grazing as an optimization process: grass-ungulate relationships in the Serengeti. Amer Nat. 1979;11(5):691–703. doi:10.1086/283426. [Google Scholar] [CrossRef]
72. Rodríguez AM, Jacobo EJ, Roitman G, Miñarro F, Preliasco P, Beade M. Management of forage offer in the National Park Campos del Tuyú and neighboring cattle farms for Pampas’s deer conservation. Ecol Austral. 2016;26(2):150–65 (In Spanish). [Google Scholar]
73. Bilenca DN, Codesido M, Abba AM, Agostini MG, Corriale MJ, Gonzalez Fischer CM, et al. Conservation of biodiversity in grasslands systems: good practices for sustainable grassland livestock farming. Extension kit for the pampas and fields. Buenos Aires: Argentine Wildlife and Argentine Birds Foundation; 2018 (In Spanish). [Google Scholar]
74. Andrade BO, Marchesi E, Burkart S, Setubal RB, Lezama F, Perelman S, et al. Vascular plant species richness and distribution in the Río de la Plata grasslands. Bot J Linn Soc. 2018;188(3):250–6. doi:10.1093/botlinnean/boy063. [Google Scholar] [CrossRef]
75. Chaneton EJ, Perelman SB, Omacini M, León RJC. Grazing, environmental heterogeneity, and alien plant invasions in temperate Pampa grasslands. Biol Invas. 2002;4:7–24. [Google Scholar]
76. Rapoport EH. The flora of Buenos Aires: low richness or mass extinction? Inter J Ecol Environ Sci. 1996;22:217–42. [Google Scholar]
77. Fonseca CR, Guadagnin DL, Emer C, Masciadri S, Germain P, Zalba SM. Invasive alien plants in the Pampas grasslands: a tri-national cooperation challenge. Biol Invas. 2013;15:1751–63. [Google Scholar]
78. Arabind NA, Shaanker MU, Bhat HNP, Charles B, Shaanker RU, Shah MA. Niche shift in invasive species: is it a case of home away from home or finding a new home? Biodivers Conserv. 2022;31(11):2625–38. [Google Scholar]
79. Herrera LP, Laterra P. Do seed and microsite limitation interact with seed size in determining invasion patterns in flooding Pampa grasslands?. In: Valk AG, editor. Herbaceous plant ecology: recent advances in plant ecology. Netherlands: Springer; 2009. p. 93–105. [Google Scholar]
80. Perelman SB, León RJC, Oesterheld M. Cross-scale vegetation patterns of Flooding Pampa grasslands. J Ecol. 2001;89:562–77. [Google Scholar]
81. Vignolio OR, Fernández ON. Cattle dung as vector of spreading seeds of exotic species in the Flooding Pampa grasslands (Buenos Aires, Argentina). Annales Botanici Fennici. 2010;47(1):14–22. [Google Scholar]
82. Poggio SL, Perelman SB, Mollard FPO, Leon RJC. Guests and gatec rashers in a New World’s banquet: old World plant species introduced from the Mediterranean Basin enriched the flora of grasslands and croplands in the Pampas of Argentina. Fl Medit. 2015;25:39–54. [Google Scholar]
83. Nieva AS, Ruiz OA. Lotus spp.: A foreigner that came to stay forever: economic and environmental changes caused by its naturalization in the Salado River Basin (Argentina). In: Taleisnik E, Lavado RS, editors. Saline and Alkaline soils in Latin America: natural resources, management and productive alternatives. Netherland: Springer; 2021. p. 431–46. [Google Scholar]
84. Loydi A, Distel RA. Floristic diversity under different intensities of large herbivore grazing in mountain grasslands of the Ventania System, Buenos Aires. Ecol Austral. 2010;20:281–91. [Google Scholar]
85. Vecchio MC, Golluscio RA, Rodríguez AM, Taboada MA. Improvement of saline-sodic grassland soils properties by rotational grazing in Argentina. Rangeland Ecol Manage. 2018;71(6):807–14. doi:10.1016/j.rama.2018.04.010. [Google Scholar] [CrossRef]
86. Rodríguez AM, Jacobo EJ. Glyphosate effects on seed bank and vegetation composition of temperate grasslands. Appl Veget Sc. 2013;16(1):51–62. doi:10.1111/j.1654-109X.2012.01213.x. [Google Scholar] [CrossRef]
87. Sala OE, Gherardi LA, Reichmann L, Jobbágy E, Peters P. Legacies of precipitation fluctuations on primary production: theory and data synthesis. Philos Trans R Soc B. 2012;367(1606):3135–44. doi:10.1098/rstb.2011.0347. [Google Scholar] [PubMed] [CrossRef]
88. Ma R, Xia CL, Liu YW, Wang YJ, Zhang JQ, Shen XJ, et al. Spatiotemporal change of net primary productivity and its response to climate change in temperate grasslands of China. Front Plant Sci. 2022;13:899800. doi:10.3389/fpls.2022.899800. [Google Scholar] [PubMed] [CrossRef]
89. Sala OE, Parton W, Joyce L, Lauenroth W. Primary production of the central grassland region of the United States. Ecology. 1988;69(1):40–5. doi:10.2307/1943158. [Google Scholar] [CrossRef]
90. McNaughton SJ, Sala OE, Oesterheld M. Comparative ecology of African and South American arid to subhumid ecosystems. In: Goldbarat P, editor. Biological relationships between Africa and South America. New Haven: Yale University Press; 1993. p. 548–67. [Google Scholar]
91. Paruelo JM, Lauenroth WK, Burke IC, Sala OE. Grassland precipitation use efficiency varies across a resource gradient. Ecosystems. 1999;2(1):64–8. doi:10.1007/s100219900058. [Google Scholar] [CrossRef]
92. Paruelo JM, Piñeiro G, Baldi G, Baeza S, Lezama F, Altesor A, et al. Carbon stocks and fluxes in Rangelands of the Río de la Plata Basin. Rangeland Ecol Manage. 2010;63(1):94–108. doi:10.2111/08-055.1. [Google Scholar] [CrossRef]
93. Hidalgo LG, Cahuépé MA. Forage production of foraging communities in the flooding pampas. Revista CREA. 1991;149:58–62 (In Spanish). [Google Scholar]
94. Aragón R, Oesterheld M. Linking vegetation heterogeneity and functional attributes of temperate grasslands through remote sensing. Appl Veget Sci. 2008;11:115–28. [Google Scholar]
95. Sala OE, Deregibus VA, Schlichter T, Alippe H. Productivity dynamics of a native temperate grassland in Argentina. J Range Manag. 1981;34(1):48–51. doi:10.2307/3898453. [Google Scholar] [CrossRef]
96. Vecchio MC, Golluscio RA, Cordero MI. Estimation of the carrying capacity at paddock scale in natural grasslands of the Flooding Pampa, Argentina. Ecol Austral. 2008;18:213–22 (In Spanish). [Google Scholar]
97. Irisarri JGN, Gundel PE, Clavijo MP, Durante M, Sosa P. ANPP and carrying capacity estimation through remote sensing data at the ranch level resolution in the flooding pampas. Rev Arg Prod An. 2013;33:1–10 (In Spanish). [Google Scholar]
98. Sciortino G. Variability of net aerial primary productivity of grasslands of the Salado Basin (Prov. Bs. As.) and its relationship with temperature and precipitation (Thesis). Faculty of Agronomy, University of Buenos Aires; 2021 (In Spanish). Available from: http://ri.agro.uba.ar/files/download/tesis/especializacion/2021sciortinogonzalo.pdf [Accessed 2023]. [Google Scholar]
99. Pérez E, Casal AV, Jacobo EJ. Evaluation of the agroecological transition through indicators of a livestock establishment based on native grasslands of the Salado basin. Rev Fac Cienc Agrar, Univ Nac Cuyo. 2019;51(1):295–307 (In Spanish). [Google Scholar]
100. Basili CJ, Sanchez SS. Behavior of aerial net primary production, soil organic carbon and total nitrogen in halophyte steppes under grazing (Thesis). National University of La Plata: Argentina; 2018 (In Spanish). Available from: http://sedici.unlp.edu.ar/bitstream/handle/10915/66562/Documento_completo__.pdf?sequence=1&isAllowed=y [Accessed 2023]. [Google Scholar]
101. Collantes MB, Stoffella SL, Ginzo HD, Kade M. Productivity and divergent botanical composition of two floristic variants of a natural grassland of the Flooding Pampa fertilized with N and P. Revista de la Facultad de Agronomía—Universidad Nacional de La Plata. 1998;103(1):45–59 (In Spanish). [Google Scholar]
102. Rodríguez AM, Jacobo EJ, Deregibus VA. Effect of phosphate fertilization on Flooding Pampa grasslands (Argentina). Rangeland Ecol Manage. 2007;60:471–8. [Google Scholar]
103. Campana S, Yahdjian L. Plant quality and primary productivity modulate plant biomass responses to the joint effects of grazing and fertilization in a mesic grassland. Appl Veg Sci. 2021;24(2):e12588. [Google Scholar]
104. Oesterheld M, Sala OE, McNaughton SJ. Effect of animal husbandry on herbivore carrying capacity at a regional scale. Nature. 1992;356(6366):234–6. [Google Scholar] [PubMed]
105. Briske DD, Heitschmidt RK. An ecological perspective. In: Heitschmidt RK, Stuth JW, editors. Grazing management: an ecological perspective. Portland: Timber Press; 1991. p. 11–26. [Google Scholar]
106. Vázquez P, Rojas MDC, Burges J. Characterization and trends of cattle farming in the Salado Basin. Rev Vet Argentina. 2008;248:572–84 (In Spanish). [Google Scholar]
107. Rearte DH, Pordomingo AJ. The relevance of methane emissions from beef production and the challenges of the Argentinean beef production platform. Meat Sci. 2014;98:35–360. [Google Scholar]
108. National Agri-Food Health and Quality Service (SENASAStatistics—Animal Chain. Cattle and Buffaloes (In Spanish). Available from: https://www.argentina.gob.ar/senasa/mercados-y-estadisticas/estadisticas/animal-estadisticas/bovinos/bovinos-y-bubalinos-sector-primario. [Accessed 2023]. [Google Scholar]
109. Institute for the Promotion of Argentine Beef (IPCVA). Statistics (In Spanish). Available from: https://www.ipcva.com.ar/vertext.php?id=964. [Accessed 2023]. [Google Scholar]
110. Argentinean Lanera Federation (FLA). Annual statistics. (In Spanish). Available from: https://www.flasite.com/index.php/es/publicaciones/estadistica-anual-2018. [Accessed 2023]. [Google Scholar]
111. Zabala JM, Taleisnik E, Giavedoni JA, Pensiero JF, Schrauf GE. Variability in salt tolerance of native populations of Elymus scabrifolius (Döll) J. H. Hunz from Argentina. Grass Forage Sci. 2011;66:109–22. [Google Scholar]
112. Porto N, Andrade NA, Entio LJ, Lissarrague MI, Bezus R, Altamirano R. Variability in characters linked to the implantation in spontaneous populations of Stapfochloa berroi (Arechav.) P. M. Peterson of the Flooding Pampa. Ecol Austral. 2023;33:427–38 (In Spanish). [Google Scholar]
113. Martinez ES, Rimieri P. The genetic improvement of prairie grass (Bromus catharticus Vahl) in Argentina: synthesis of achievements and advances. J Basic Appl Genet. 2023;4(1):41–6. [Google Scholar]
114. Condón F, Jaurena M, Reyno R, Otaño C, Lattanzi FA. Spatial analysis of genetic diversity in a comprehensive collection of the native grass Bromus auleticus Trinius (ex Nees) in Uruguay. Grass Forage Sci. 2017;72(4):723–33. [Google Scholar]
115. Schrauf GE, Blanco MA, Cornaglia PS, Pacheco MG, Madia M, Padilla JM. Ergot resistance in plants of Paspalum dilatatum incorporated by hybridisation with Paspalum urvillei. Trop Grasslands. 2003;37:182–6. [Google Scholar]
116. INaSe 2003. Registration of “RELINCHO” cultivar of Dallisgrass (Paspalum dilatatum) inscribed in the property of cultivar registration and in the National Register of Cultivars of the National Institute of Seeds-Argentina. Register Number 7774. Available from: https://gestion.inase.gob.ar/consultaGestion/gestiones/index/page:2. [Accessed 2024]. [Google Scholar]
117. INaSe 2013. Registration of “PRIMO” cultivar of Dallisgrass (Paspalum dilatatum) inscribed in the property of cultivar registration and in the National Register of Cultivars of the National Institute of Seeds-Argentina. Register Number 12252. Available from: https://gestion.inase.gob.ar/consultaGestion/gestiones/index/page:2. [Accessed 2024]. [Google Scholar]
118. Schrauf GE, Voda L, Zelada AM, García AM, Giordano A, Peralta Roa P, et al. Development of protocols for regeneration and transformation of apomitic and sexual forms of Dallisgrass (Paspalum dilatatum Poir.). Front Plant Sci. 2022;12:787549. [Google Scholar] [PubMed]
119. Yang Y, Tilman D, Furey G, Lehman C. Soil carbon sequestration accelerated by restoration of grassland biodiversity. Nat Commun. 2019;10:718. [Google Scholar] [PubMed]
120. Lyons KG, Török P, Hermann JM, Kiehl K, Kirmer A, Kollmann J. Challenges and opportunities for grassland restoration: a global perspective of best practices in the era of climate change. Glob Ecol Conserv. 2023;46:e02612. Available from: https://www.sciencedirect.com/science/article/pii/S2351989423002470 [Google Scholar]
121. Bernoux M, Volkoff B. Soil carbon stocks in soil ecoregions of Latin America. In: Lal R, Cerri C, Bernoux M, Etchevers J, Cerri E, editors. Carbon sequestration in soils of Latin America. New York, London, Oxford: Food Products; 2006. p. 65–78. [Google Scholar]
122. Sirimarco X, Villarino S, Barral MP, Puricelli M, Laterra P. Transformation of tall-tussock grasslands and soil water dynamics in the Flooding Pampa. Sci Total Environ. 2023;896:165362. [Google Scholar] [PubMed]
123. Bai Y, Cotrufo MF. Grassland soil carbon sequestration: current understanding, challenges, and solutions. Science. 2022;377:603–8. [Google Scholar] [PubMed]
124. Chaneton EJ, Lavado RS. Soil nutrients and salinity after long-term grazing exclusion in a flooding Pampa grassland. Rangeland Ecol Manage. 1996;49(2):182–7. [Google Scholar]
125. Piñeiro G, Paruelo JM, Jobbágy EG, Jackson RB, Oesterheld M. Grazing effects on belowground C and N stocks along a network of cattle exclosures in temperate and subtropical grasslands of South America. Glob Biogeochem Cy. 2009;23:1–14. [Google Scholar]
126. Faverin C, Bilotto F, Fernández Rosso C, Machado C. Productive, economic and greenhouse gases modelling of typical beef cow-calf systems in the flooding pampas. Chilean J Agric Anim Sci. 2019;35(1):14–25. [Google Scholar]
127. Arrieta EM, Cabrol DA, Cuchietti A, González AD. Biomass consumption and environmental footprints of beef cattle production in Argentina. Agric Syst. 2020;85(2):102944. doi:10.1016/j.agsy.2020.102944. [Google Scholar] [CrossRef]
128. Bilotto F, Recavarren P, Vibart R, Machado CF. Backgrounding strategy effects on farm productivity, profitability and greenhouse gas emissions of cow-calf systems in the flooding pampas of Argentina. Agric Syst. 2019;176(2):102688. doi:10.1016/j.agsy.2019.102688. [Google Scholar] [CrossRef]
129. Jacobo EJ, Cadaviz N, Vecchio MC, Rodriguez AM. Estimation of the balance of greenhouse gases in livestock production systems in the Salado river basin. Agriscientia. 2020;37(1):15–32. doi:10.31047/1668.298x.v37.n1.27514. [Google Scholar] [CrossRef]
130. Perez MG, Romaniuk RI, Cosentino VRN, Busto M, González FA, Taboada MA, et al. Winter soil N2O emissions from a meat production system under direct grazing of Argentine Pampa. Anim Prod Sci. 2021;61:156–62. [Google Scholar]
131. Lavado RS, Sierra JO, Hashimoto PN. Impact of grazing on soil nutrients in a Pampean grassland. Rangeland Ecol Manage. 1996;49(5):452–7. doi:10.2307/4002929. [Google Scholar] [CrossRef]
132. Garibaldi LA, Semmartin MG, Chaneton EJ. Grazing induced changes in plant composition affect litter quality and nutrient cycling in Flooding Pampa grasslands. Oecologia. 2007;151(4):650–62. doi:10.1007/s00442-006-0615-9. [Google Scholar] [PubMed] [CrossRef]
133. Semmartin MG, Garibaldi LA, Chaneton EJ. Grazing history effects on above-and below-ground litter decomposition and nutrient cycling in two co-occurring grasses. Plant Soil. 2008;303(1–2):177–89. doi:10.1007/s11104-007-9497-9. [Google Scholar] [CrossRef]
134. Taboada MA, Micucci SN. Response of the physical properties of three soils from the Flooding Pampa to rotational grazing. Cienc del Suelo. 2009;27(2):147–57 (In Spanish). [Google Scholar]
135. Chagas CI, Piazza MV, de Siervi M, Santanatoglia OJ, Morettón J, Paz M, et al. Overland run-off water quality in extensive and intensive farming systems of Argentina. Agrochimica. 2007;51(2–3):130–6. [Google Scholar]
136. Booman GC, Calandroni M, Laterra P, Cabria F, Iribarne O, Vázquez P. Areal changes of lentic water bodies within an agricultural basin of the Argentinean Pampas. Disentangling land management from climatic causes. Environ Manag. 2012;50(6):1058–67. doi:10.1007/s00267-012-9943-1. [Google Scholar] [PubMed] [CrossRef]
137. Engel V, Jobbágy EG, Stieglitz M, Williams M, Jackson RB. Hydrological consequences of Eucalyptus afforestation in the Argentine Pampas. Water Resour Res. 2005;41(10):W10409. doi:10.1029/2004WR003761. [Google Scholar] [CrossRef]
138. Lavado RS, Taboada MA. Soil salinization as an effect of grazing in a native grassland soil in the Flooding Pampa of Argentina. Soil Use Manag. 1987;3(4):143–8. doi:10.1111/j.1475-2743.1987.tb00724.x. [Google Scholar] [CrossRef]
139. Hernandez-Morcillo M, Plieninger T, Bielinget C. An empirical review of cultural ecosystem service indicators. Ecol Indic. 2013;29(2):434–44. doi:10.1016/j.ecolind.2013.01.013. [Google Scholar] [CrossRef]
140. Wang L, Huang L, Cao W, Zhai J, Fan J. Assessing grassland cultural ecosystem services supply and demand for promoting the sustainable realization of grassland cultural values. Sci Total Environ. 2024;912(1):169255. doi:10.1016/j.scitotenv.2023.169255. [Google Scholar] [PubMed] [CrossRef]
141. Sistema Federal de Áreas Protegidas (SiFAP) sifap.gob.ar. Available from: https://sifap.gob.ar/areas-protegidas [Accessed 2024]. [Google Scholar]
142. Weyland F, Colacci P, Cardoni A, Estavillo C. Can rural tourism stimulate biodiversity conservation and influence farmer’s management decisions? J Nat Conserv. 2021;64:126071. doi:10.1016/j.jnc.2021.126071. [Google Scholar] [CrossRef]
143. WWF Argentina. vidasilvestre.org.ar. Available from: https://www.vidasilvestre.org.ar/ [Accessed 2024]. [Google Scholar]
144. Perez Winter C, Troncoso C. The tourist image of the pampas countryside (Argentina) through official promotion. Cuadernos de Antropología Soc. 2019;50:85–106. [Google Scholar]
145. Rodríguez GF. Return to the countryside: rural tourism in the revaluation of rural space. In: Fernández SP, Rodríguez GF, Canale A, Gallo G, editors. Rural tourism in debate: 10 years of experience in training technicians at FAUBA: 2009−2019. Buenos Aires: Editorial Faculty of Agronomy; 2019. p. 12–23 (In Spanish). [Google Scholar]
Cite This Article
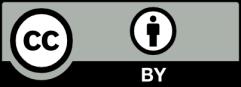