Open Access
REVIEW
Microbial Fertilizer: A Sustainable Strategy for Medicinal Plants Production
1 Hunan Academy of Chinese Medicine, Hunan University of Chinese Medicine, Changsha, 410036, China
2 Institute of Chinese Medicine Resources, Hunan Academy of Chinese Medicine, Changsha, 410013, China
* Corresponding Author: Jian Jin. Email:
(This article belongs to the Special Issue: Microbial Biotechnology Applications on Plant Performance and Physiology for Sustainable Production )
Phyton-International Journal of Experimental Botany 2024, 93(6), 1221-1236. https://doi.org/10.32604/phyton.2024.050759
Received 16 February 2024; Accepted 09 May 2024; Issue published 27 June 2024
Abstract
Medicinal plants have aroused considerable interest as an alternative to chemical drugs due to the beneficial effects of their active secondary metabolites. However, the extensive use of chemical fertilizers and pesticides in pursuit of yield has caused serious pollution to the environment, which is not conducive to sustainable development in the field of medicinal plants. Microbial fertilizers are a type of “green fertilizer” containing specific microorganisms that can improve the soil microbial structure, enhance plant resistance to biological and abiotic stresses, and increase the yield of medicinal plants. The root exudates of medicinal plants attract different microorganisms to the rhizosphere, which then migrate further to the plant tissues. These microbes can increase the levels of soil nutrients, and improve the physical and chemical properties of soil through nitrogen fixation, and phosphorus and potassium solubilization. In addition, soil microbes can promote the synthesis of hormones that increase plant growth and the accumulation of active compounds, eventually improving the quality of medicinal plants. In 2022, the total value of the global microbial fertilizer market was $4.6 billion and is estimated to reach $10.36 billion by 2030. In this review, we have summarized the types of microbial fertilizers, the colonization and migration of microorganisms to plant tissues, and the beneficial effects of microbial fertilizers. In addition, the prospects of developing microbial fertilizers and their application for medicinal plants have also been discussed. It aims to provide a reference for the rational application of microbial fertilizers in the field of medicinal plants and the green and sustainable development of medicinal plant resources.Keywords
Supplementary Material
Supplementary Material FileMedicinal plants occupy an important position in the whole history of human development. The active ingredients of some medicinal plants, such as artemisinin in Artemisia annua, acetylsalicylic acid in willow bark, quinine in cinchona bark, paclitaxel in taxane bark, and ginsenoside in ginseng, are of inestimable importance in maintaining human health and promoting disease treatment. However, medicinal plants often have long growth cycles and slow accumulation of active ingredients, while excessive use of fertilizers leads to environmental pollution, soil acidification and hardening, decreased crop quality, and increased production costs, aggravating the degradation of agricultural ecosystems. Microbial fertilizers containing specific living microorganisms are increasingly considered a viable alternative to chemical fertilizers. The enzymatic activities of the microbes can increase the supply of nutrients to the plants, thereby enhancing plant growth, crop yield, and the quality of agricultural products, as well as improving the ecological balance. Microbial fertilizers cannot only improve soil structure and its physical and chemical properties, and increase fertilizer efficiency, but also improve the disease and pest resistance and quality of crops [1], thus offering a sustainable green production strategy for medicinal plants.
Microbial fertilizers can alter the species composition of the rhizosphere microbiome and endophytes of medicinal plants and increase the abundance of beneficial microorganisms [2,3], which promotes plant growth as well as the accumulation of active compounds, eventually improving the yield and quality of medicinal plants. In addition to live microorganisms, microbial fertilizers also contain organic matter that not only provides energy for microbial growth but is also the source of major nutrients such as nitrogen, phosphorus, and potassium for the plants. The microorganisms in these fertilizers degrade the soil organic matter into smaller carbon compounds, of which some are directly absorbed by plants to form hormones and metabolites that are beneficial to crop quality, resistance, and yield. In addition, microbial fertilizers also enhance the availability of medium and trace elements in the soil. In this review, we have discussed the types of microbial fertilizers, the international market of microbial fertilizers, the registered microbial fertilizer products in China and their main strains, and their dosage forms and applications for medicinal plants. In addition, the effects of microbial fertilizer products and microorganisms on the yield and quality of some medicinal plants have also been summarized. This review aims to provide some references for the research and application of microbial fertilizers in the cultivation of medicinal plants.
2 Current Development of Microbial Fertilizers
2.1 Types of Microbial Fertilizers
Microbial fertilizers are mainly classified according to the types of microorganisms. In China, three types of microbial fertilizers (Fig. S1) are currently used: (1) Microbial inoculants consisting of one or more target microorganisms and a small amount of carrier. The microbes are either used directly after industrial-scale production, or concentrated further, or adsorbed on carriers. (2) Compound fertilizers that consist of live microbes with additional nutrients. (3) Organic fertilizers that are composed of target microorganisms and organic materials derived from animal and plant residues (such as animal manure and crop straw). The production strains for these fertilizers include Rhizobium, silicate bacteria, and phosphate solubilizing microbes among others.
The International Fertilizer Industry Association [4] classifies fertilizers into four categories: mineral or inorganic fertilizers, organo-mineral fertilizers, organic fertilizers, and beneficial substances, while microbial fertilizers are classified into the fourth category-beneficial substances. Furthermore, microbial fertilizers have been subdivided into five categories according to the types of microorganisms: (1) Bacterial fertilizers (Rhizobium, nitrogen-fixing bacteria, phosphate solubilizing bacteria, potassium solubilizing bacteria, and photosynthetic bacteria); (2) Actinomycete fertilizer (antibiotic-producing bacteria); (3) Fungal fertilizer (mycorrhizae including ectomycorrhizal and endomycorrhizal agents); (4) Algal fertilizer (nitrogen-fixing cyanobacteria); (5) Compound microbial fertilizer consisting of two or more microorganisms in a certain proportion.
2.2 The Market for Microbial Fertilizers
The growing population worldwide has concomitantly increased the demand for food. While chemical fertilizers have helped countries achieve self-sufficiency in food production, their adverse effects on the environment and ecological balance have spurned the development of organic agriculture to improve crop yield and quality. According to the “global microbial fertilizer market survey and forecast report” (hereafter referred to as “the report”) released by research and market [5] in 2023 (Fig. 1), bacterial fertilizers account for 42.9% of the microbial fertilizer market, ahead of fungal, algal, and other fertilizers. In particular, nitrogen-fixing bacterial fertilizers are most commonly used, accounting for 27.45% of the total market share. In terms of dosage forms, solid preparations are most common, followed by liquid preparations. Fruits, vegetables, and cereals are still the main targets of microbial fertilizers, and beans and oilseeds account for the largest market share of 30.34%. Europe, the Middle East, and Africa are the main markets of microbial fertilizers, followed by the Americas. The total value of the global microbial fertilizer market was $4.6 billion in 2022 and reached $5.07 billion in 2023 with a growth rate of 10.6%. By 2030, the estimated value of the microbial fertilizer market will be $10.36 billion.
Figure 1: Key data on the development of the global microbial fertilizer market. Data source: research and markets website
2.3 Impacts of Microbial Fertilizers on Environmental Sustainability
The massive use of chemical fertilizers has indeed alleviated the food crisis with the explosive growth of the population, but the negative impact on the environment is also obvious [6]. The long-term excessive use of chemical fertilizers can destroy the microecological balance of soil, and lead to soil hardening, salinization, and water eutrophication. Nitrous oxide, a powerful greenhouse gas released by nitrogen fertilization, has a warming potential of 298 times that of carbon dioxide, which may cause serious climate problems and bring huge environmental pressure [7]. It is well known that soil microorganisms play an important role in maintaining the structure and function of the soil ecosystem. After the application of microbial fertilizer, the increase of soil microbial abundance not only increases the absorption of important nutrients such as nitrogen, phosphorus, and potassium by medicinal plants, improves the yield and quality of medicinal plants, but also improves the rhizosphere soil environment. Through the secretion of various secondary metabolites, proteins, mucus, and other substances by the developing plant roots, suitable microorganisms are recruited for the roots of various medicinal plants, and soil particles are combined and wound together through extracellular polysaccharides to fix the soil, enhance the stability of soil aggregates, and improve soil compaction [8], help to improve soil health, and continuously enhance long-term agricultural productivity.
3 Factors Contributing to Medicinal Plant Microbes’ Interaction
3.1 Medicinal Plant Microbes Interaction at Rhizosphere Level
The rhizosphere is the nutrient-rich zone of the soil that surrounds plant roots [9]. It is a highly dynamic region influenced by microbes and root exudates and is indispensable for plant growth and development. Root exudates include low molecular weight compounds such as sugars, amino acids, organic acids, etc., and high molecular weight compounds like polysaccharides, proteins, etc. (Fig. 2). These substances can alter the biological and abiotic factors in the surrounding soil, which in turn affect the growth and development of plants [10]. Rhizosphere microorganisms detect and track chemical gradients in the environment through transmembrane chemoreceptors called methyl receptor chemotactic proteins (MCPs) [11]. MCPs form a ternary signal complex with histidine autokinase CheA and coupling protein chew, which combines the activity of CheA with receptor control [12]. Root exudates interact with the chemoreceptor ligand binding domain (MCP LBD) to produce a molecular stimulus [13]. After membrane conduction, it regulates the activity of CheA autokinase, changes the flux of the CheA phosphorylation group to Cheb and CheY, and the phosphorylated CheY binds to the whiplash motor and induces clockwise (CW) rotation, eventually leading to chemotaxis [14]. For example, Bacillus subtilis, which is widely used in microbial fertilizers at present, shows chemotactic attraction to Arabidopsis root exudates in vitro through the MCPB and MCPC, and the orphan receptor TLPC [15]. Likewise, Bacillus isolated from cucumber rhizosphere responds to 44 of the 98 root secretory components [16]. Furthermore, the sugar, amino acids, and organic acids present in corn root exudates can significantly induce the chemotaxis, colony movement, and biofilm formation of Bacillus in the roots [17].
Figure 2: Chemotaxis of microorganisms to medicinal plant rhizosphere and main ways of entering plant cells
The root exudates of medicinal plants mainly include organic acids (benzoic acid, phenylacetic acid, butanediol acid, tetradecanoic acid, etc.), fatty acids (linolenic acid, eicosanoic acid, hexadecanoic acid, etc.) and phenolic acids (salicylic acid, syringic acid, ferulic acid, vanilla, cinnamic acid, etc.) [18], which modulate the composition of rhizosphere microorganisms. The differences in the metabolite profiles of the root exudates determine the species composition of endophytes in plant tissues. Studies have shown that some products of photosynthesis or root exudates can attract specific microorganisms to colonize different tissues. In addition, the physiological features, growth, and health status of plants also affect the species and number of endophytes [19].
3.2 Medicinal Plant-Endophytes’ Interaction
Endophytes usually refer to microorganisms that reside in the plant tissues for most of the host life cycle and exert beneficial effects [20]. The exogenous microbes enter plant tissues through horizontal transmission. Briefly, the microorganisms present in the soil and fertilizer first attach to the surface of plant roots or aerial parts and then enter the tissues through the openings in the hairy roots and lateral roots, as well as the stomata, water holes, wounds, and other open structures on stems or buds. The cellulose hydrolases secreted by microorganisms then degrade the cell wall of plant cells, thus allowing the microbes to further migrate through the xylem vessels of the entire plant [13,21,22]. On the other hand, endophytes are vertically transmitted from the parent plant to the seeds, pollen, or vegetative propagules [23]. Previous studies have reported the presence of bacteria in the epidermis, endosperm, and embryo of plant seeds, as well as on the surface and inside of wind-borne and insect-borne pollen. In addition, pollen is also an excellent vehicle for horizontal microbial transmission between different plants [24–27].
4 Beneficial Effects of Microbial Fertilizer on Medicinal Plants
4.1 Improvement in Plant Growth
The most common objective of using microbial fertilizers is to improve plant growth (Fig. 3). Microorganisms synthesize indoleacetic acid through the lysine-dependent pathway to promote plant growth [28,29]. Likewise, phosphate-solubilizing microorganisms can significantly increase the fresh weight, dry weight, plant height, root and crown length, and other growth parameters of mint plants. In addition, these microorganisms are mixed with different phosphorus sources to enhance the performance of the fertilizer [30]. Plant growth-promoting root bacteria (PGPRs) are a large group of root bacteria including Rhizobia, Bradyrhizobium, Bacillus, Azotobacter, and so on [31]. They can enhance plant growth by dissolving insoluble phosphate, fixing atmospheric nitrogen, and secreting growth hormones [32]. Following inoculation of a PGPR strain, the seedling height of Pinus sylvestris var. mongolica increased significantly by 27.6%, and the root length increased by 47.88% (p < 0.05). In addition, the available nitrogen, phosphorus, and potassium in the soil increased by 19.05%–47.62%, 26.63%–83.32%, and 87.70%–98.1% (p < 0.05), respectively [33], indicating that PGPR is a promising microbial fertilizer.
Figure 3: Beneficial effects and mechanisms of microorganisms on medicinal plants. In the figure, P and K represent phosphorus and potassium
4.2 Increased Accumulation of Bioactive Compounds in the Plants
The active compounds accumulate in medicinal plants over an extended period. For example, the duration between planting and harvest of ginseng is usually 4–6 years to allow the ginsenoside content to reach medicinal levels. Appropriate microbial fertilizers can effectively increase the accumulation rate of active products. Fertilizing with Bacillus altiplanus can enhance the content of ginsenoside in ginseng root culture while shortening the growth cycle by increasing the abundance of the endophyte LB 5-3, which is known to increase the biomass of adventitious roots and promote ginsenoside accumulation [34]. In addition, endophytic microbes produce a variety of active secondary metabolites, such as alkaloids [35], organic acids [36], sesquiterpenes [35,37,38], lactones [39,40], saponins [41], and even promote the synthesis of these metabolites in host cells by regulating metabolic pathways. For example, the endophytes of Papaver capsular promote the synthesis and accumulation of benzylisoquinoline alkaloids (BIA) by upregulating the key genes involved in their biosynthesis [42]. Furthermore, the endophytic bacteria ALEB7B of Atractylodes macrocephala significantly enhanced the accumulation of photosynthetic products and altered the metabolic flux in host cells, which increased the synthesis of sesquiterpenes in the latter [43]. Panax notoginseng contains a variety of ginsenosides, such as Rg1, Rh1, RB1, and RE, which have antioxidant, anti-inflammatory, vasodilatory, anti-allergy, anti-diabetes, and other therapeutic effects [44]. Studies show that some endophytes of P. notoginseng can directly participate in the biotransformation of ginsenosides, although the mechanism is not clear. For instance, the conversion rate of Rg1 to F1 by Enterobacter Chengdu is 13.24%, Rb1 to Rd by Trichoderma koningii is 40%, that of Ginsenoside Rg3 is 32.31%, and of Rb1 to Rd by Penicillium chermesinum is 74.24% [45].
4.3 Improvement in the Nutritional, Physical, and Chemical Properties of Soil
The gradual reduction in soil nutrients is an inevitable process in agriculture. In addition to being absorbed by the planted crops, soil nutrients are also lost due to rainfall. The flow of nutrients into water bodies causes eutrophication and pollution, which poses a threat to the ecosystem. Microbial fertilizers containing nitrogen fixing, and potassium and phosphorous solubilizing microorganisms can restore soil nutrients, and improve the growth, yield, and quality of medicinal plants. The beneficial effects of the fertilizer can be further enhanced by including biochar, a porous carrier that provides a surface as well as energy for the microorganisms to grow [46]. A biochar-based microbial fertilizer consisting of Bacillus and exogenous N-P-K fertilizer significantly reduces nutrient leaching from the soil, improves the nutrient profile and quality of the soil, and increases the abundance of bacteria related to carbon and nitrogen metabolism [47]. In addition, microbial fertilizer significantly improves physical and chemical indices such as moisture content (MC), organic matter (OM), alkali hydrolyzable nitrogen (AN), and available phosphorus (AP), which is conducive to maintaining the ecological health of the soil [48].
4.4 Regulation of the Soil Microbiome and Enhanced Biological Resistance
Medicinal plants are often grown as continuous crops since most cannot grow and mature in a year, especially the perennial plants bearing fruits or rhizomes (such as Schisandra chinensis and ginseng). In addition, many active ingredients are either not synthesized or present at low concentrations in immature plants. As already discussed, the root exudates of medicinal plants attract specific microorganisms. Given that most of medicinal plants are perennial, their cultivation methods significantly affect the microbial community structure of the rhizosphere. For example, the diversity of bacteria in the rhizosphere of Pseudostellaria heterophylla grown for two years is significantly lower than that of plants cultivated for one year [49]. Similar results have been reported for American ginseng [50].
Diseases and pests can significantly reduce the yield and quality of medicinal plants, especially the perennial varieties that are continuously planted on the same land. Studies show that continuous cropping can disrupt the microbial community [51,52], and transform the highly fertile bacterial soil to less fertile “fungal” soil. In addition, the decrease in the abundance of beneficial bacteria and the increase in that of harmful bacteria has elevated the risk of diseases [18] during the cultivation of ginseng [53], P. notoginseng [54,55], Angelica sinensis [56], and other medicinal plants. For example, the accumulation of Fusarium oxysporum has been shown to cause root rot in Atractylodes macrocephala and P. notoginseng. The use of eco-friendly microbial fertilizers can improve soil microbial diversity [57,58]. Furthermore, crop rotation [59,60] is known to improve the soil microbial community, and increase the abundance of Bacillus and Lysobacterium. Inoculation of the probiotic Pseudomonas into the roots of P. notoginseng cultivated continuously for three years reduced the relative abundance of potentially pathogenic bacteria in the rhizosphere and increased that of other bacteria and fungi [61], resulting in a lower risk of diseases. In addition, some endophytic bacteria may protect plants from pathogens through a variety of mechanisms, such as replacing pathogens in plant tissues and producing antibiotics that directly inhibit their growth. Some species of Pseudomonas can also hinder the growth of soil pathogens [62]. Microbial fertilizers consisting of relevant probiotics and appropriate carriers can improve the biological resistance of medicinal plants and reduce the risk of diseases.
4.5 Improved Abiotic Resistance
Soil-related abiotic stresses include drought, soil salinization, mineral nutrition deficiency, heavy metal pollution, etc. Plants often resist these environmental stresses by producing hormones [63,64]. Studies show that abiotic stress can induce the synthesis and accumulation of ethylene in plants [65], which triggers various physiological reactions and inhibits plant growth [66]. Several microbes produce 1-aminocyclopropane-1-carboxylate (ACC) deaminase, which can cleave the ethylene precursor ACC into ammonia and α-ketobutyrate and reduce ethylene levels in stressed plants [67,68]. The resulting improvement in the root system enhances the absorption of water and nutrients from the soil depths, which promotes plant growth and prolongs survival under stress conditions [69]. Several ACC deaminase-producing bacteria have been isolated from different habitats [65]. Symbiotic and non-symbiotic nitrogen-fixing bacteria convert atmospheric nitrogen into ammonia that can be utilized by plants. The microbes further enhance nitrogen absorption by the plant roots by mineralizing the organic ammonia in the soil or stimulating hormone production [70]. Likewise, PGPRs such as phosphate-and potassium-solubilizing bacteria can promote the absorption of potassium and phosphorus through chelation, generation of organic ligands, exchange reaction, and demineralization [65]. Excessive levels of heavy metals in the soil can directly affect plant growth, metabolism, and aging, and induce plant cell apoptosis. Plants have evolved mechanisms to reduce the absorption of heavy metals [71]. Some PGPRs can transform the bioavailable forms of heavy metals into unavailable forms [72]. For instance, the soluble phosphorus released by phosphate-solubilizing bacteria can precipitate heavy metals via the formation of insoluble phosphates. In addition, some microorganisms can produce extracellular polymeric substances to adsorb heavy metals. Organic acids produced by soil microorganisms, such as succinic acid and oxalic acid, also form complexes with heavy metals that cannot be easily absorbed by the plant roots [65]. Overall, these strategies can reduce the absorption of heavy metals and minimize plant stress. Therefore, suitable microorganisms can be incorporated into microbial fertilizers to rescue medicinal plants from abiotic stresses.
5 Applications of Microbial Fertilizers in Medicinal Plants
5.1 Main Strains Contained in Existing Microbial Fertilizer Products in China
At present, microbial fertilizers are mainly used for vegetables, tobacco, melons, and fruits. The medicinal plants that are currently cultivated in China are listed in Table S1, and include P. notoginseng, Codonopsis pilosula, A. sinensis, Astragalus membranaceus, Zanthoxylum bungeanum, A. macrocephala, Salvia miltiorrhiza, P. heterophylla, Ophiopogon japonicus, etc. According to the data from the Quality Supervision, Inspection and Testing Center for Microbial Fertilizers and Edible Fungi of the Ministry of Agriculture and Rural Areas of the People’s Republic of China, 109 microbial fertilizer products were registered for Medicinal plant materials, including 65 microbial agents, 33 microbial organic fertilizers and 11 compound microbial fertilizers. The strains include B. subtilis, Bacillus gelatinosus, Bacillus amyloliquefaciens, Penicillium, Streptomyces, Trichoderma, Lactobacillus, and yeast.
5.2 Effect of Microbial Fertilizer on Yield and Quality of Medicinal Plants in Practical Application
Unlike traditional crops, quality is a more important parameter for medicinal plants compared to yield. The quality of medicinal plants is determined by the amount of secondary metabolites. Chemical fertilizers promote the growth of medicinal plants increase their yield, and decrease the production of secondary metabolites, leading to a decline in their quality [73]. In contrast, long-term use of microbial fertilizers can significantly improve the yield and quality of medicinal plants. The effects of some microbial fertilizer products on the quality of medicinal plants are summarized in Table 1. Polysaccharides are the main active compounds in goji berries that mediate the therapeutic effects [74]. Yin et al. showed that microbial agents and composite microbial fertilizers increased the total sugar content in goji berries by 25% and 14%, respectively [75]. Li et al. also found that two types of microbial fertilizers increased the content of goji berry polysaccharides by 75% and 25.76%, respectively, and also improved that of other medicinal components by varying degrees [76]. As shown in Table 1, microbial fertilizers significantly increased the content of fumaric acid in Corydalis yanhusuo [77], peimucin [78] in Fritillaria hunbergiai, curcumin [79] in Curcuma longa, ginsenosides [80] in ginseng, and tanshinone [81] in S. miltiorrhiza.
5.3 Applications of New Technologies on Microbial Fertilizer
In recent years, the development strategy of microbial fertilizer products has shifted from single-strain to multi-strain joint inoculation. This method is conducive to the strains having a greater chance of surviving in the competitive exclusion [85]. The most classic application is co-inoculation of rhizobia and arbuscular mycorrhizal fungi. Many studies have reported their synergistic effects on plant growth promotion [86,87]. The separation of functional strains is also the mainstream of microbial fertilizer research. Furthermore, the innovation of microbial fertilizer carriers is also a big help to promote the development of microbial fertilizer. To keep microorganisms in a dynamic state, commercially available carrier materials are usually based on ground peat, clay, rice, bran, seeds, or other complex organic substrates [88]. Activated carbon has aroused great interest of researchers because of its porous and loose structure, and studies have shown that microbial fertilizer with activated carbon as the carrier is better than other substrates in promoting host growth [89]. The microbial fertilizer nanoparticles encapsulated in chitosan [90] or coated with silicon, zinc, copper, iron, and silver [91] are easier to transport and use, and have better effects and longer effective time. It can be said that the formation and application of nano biological fertilizer is a practical step towards intelligent fertilizer.
Microbial fertilizer is a green substitute for chemical fertilizer, promoting sustainable agricultural practices. It improves the yield and quality of medicinal plants, rarely with an adverse effect on the environment. However microbial fertilizer also faces many challenges. For example, strains in microbial fertilizer might be medicinal plant selectivity and region climate specificity. Regarding its current development status and shortcomings, the possible directions for the future development of microbial fertilizers for medicinal plants are proposed.
6.1 Screening of Suitable Microbial Strains
Since microorganisms are the core of microbial fertilizers, screening suitable microbial strains is a major challenge for the development of microbial fertilizers, especially rhizosphere microorganisms and endophytes. In addition, the complex interactions between medicinal plants, and the rhizosphere microorganisms and endophytes warrant further research since these microbes directly or indirectly affect the growth, development, and quality of medicinal plants [92]. Endophytes are known to synthesize secondary metabolites similar to that of their host plants. For example, some endophytic fungi in Peucedanum praeruptorum can be used as a micro factory for the production of coumarin and improve its medicinal value [93]. In some cases, the pharmacological activity of the substances produced by the endophytes may be stronger than that produced by host plants [94–96]. Therefore, a greater understanding of the rhizosphere microorganisms and endophytes of medicinal plants can aid in the development of more effective microbial fertilizers suitable, and improve their application in cultivated medicinal plants.
6.2 Optimization of the Preparation Process of Microbial Fertilizers
At present, the bulk of the research on microbial fertilizers is focused on improving the yield or quality of the target medicinal plants, and only a few systematic studies have been conducted on the optimal preparation methods of these fertilizers, the mechanisms of action of the microorganisms, the survival of microbes in the rhizosphere soil and the secretion of metabolites, and the material exchange between microorganisms and medicinal plants, which is also a major limitation of the development of microbial fertilizer. The mechanisms underlying the interactions between the microorganisms, soil, and rhizosphere need to be elucidated to select more suitable microbial fertilizers and dosage forms for specific medicinal plants and develop special strains suitable for different regions, maturation stages, and plant species. This may promote a more large-scale application of microbial fertilizers by expanding the varieties of medicinal plants that can be treated with these fertilizers, and that of microbial strains that can be used as fertilizers.
6.3 Promoting the Application of Microbial Fertilizers for Medicinal Plants
Microbial fertilizers are an eco-friendly option for improving the growth and quality of medicinal plants. Nowadays, several varieties of medicinal plants are registered in the Quality Supervision, Inspection, and Testing Center for Microbial Fertilizers and Edible Fungus Strains of the Ministry of Agriculture and Rural Areas of China. Nevertheless, there is still no special microbial fertilizer for different species of medicinal plants. The research and development of microbial fertilizers need to be accelerated to promote the cultivation of medicinal plants on an industrial scale. Furthermore, the technical standards and usage guides of the microbial fertilizers have to be reasonably formulated to popularize their application and promote the organic cultivation of medicinal plants.
In summary, microbial fertilizer can improve the yield and quality of medicinal plants and has little adverse impact on the environment. It is a sustainable strategy for the production of medicinal plants. In this paper, the interactions between microbial fertilizers and medicinal plants and their effects on the yield and quality of medicinal plants were reviewed, and some suggestions on the future development of microbial fertilizers were put forward, hoping to provide a new perspective for the development of microbial fertilizers in the sustainable production of medicinal plants in the future.
Acknowledgement: The authors would like to extend their sincere appreciation to the researchers. Supporting Project Number (2022RC1224, kq2305028).
Funding Statement: This research was funded by the Science and Technology Innovation Program of Hunan Province (2022RC1224) and the Training Program for Excellent Young Innovators of Changsha (kq2305028).
Author Contributions: Data collection and analysis: C. Liu, J. Xie, H. Liu, C. Zhong, G. Pan, and S. H. Zhang; writing draft: C. Liu; project management and funding acquisition: J. Jin. All authors have read and approved the submitted manuscript version.
Availability of Data and Materials: All the data and materials supporting the findings of this study are included in this article.
Ethics Approval: Not applicable.
Conflicts of Interest: The authors declare that they have no conflicts of interest to report regarding the present study.
Supplementary Materials: The supplementary material is available online at https://doi.org/10.32604/phyton.2024.050759.
References
1. Huang Q, Wei GF, Chang RX, Xia CL, Dong LL. The current development status of microbial fertilizers and their application in the cultivation of traditional Chinese medicine. Mod Chinese Med. 2022;24(1):153–9 (In Chinese). [Google Scholar]
2. Li S, Fan W, Xu G, Cao Y, Zhao X, Hao S, et al. Bio-organic fertilizers improve Dendrocalamus farinosus growth by remolding the soil microbiome and metabolome. Front Microbiol. 2023;14:1117355. doi:10.3389/fmicb.2023.1117355. [Google Scholar] [PubMed] [CrossRef]
3. Liang JP, Xue ZQ, Yang ZY, Chai Z, Niu JP, Shi ZY. Effects of microbial organic fertilizers on Astragalus membranaceus growth and rhizosphere microbial community. Annals Microbiol. 2021;71:1–15. [Google Scholar]
4. The International Fertilizer Association. Available from: https://www.fertilizer.org/ [Accessed 2024]. [Google Scholar]
5. Research and Markets. Available from: https://www.researchandmarkets.com/ [Accessed 2024]. [Google Scholar]
6. Wang T, Xu J, Chen J, Liu P, Hou X, Yang L, et al. Progress in microbial fertilizer regulation of crop growth and soil remediation research. Plants. 2024;13:346. doi:10.3390/plants13030346. [Google Scholar] [PubMed] [CrossRef]
7. Abbey JK. Bridging the gap between agriculture and climate: mitigation of nitrous oxide emissions from fertilizers. Environ Progress Sustain Energy. 2022;42(2):e14069. [Google Scholar]
8. Wu L, Wang Y, Zhang S, Wei W, Kuzyakov Y, Ding X. Fertilization effects on microbial community composition and aggregate formation in saline-alkaline soil. Plant Soil. 2021;463:523–35. doi:10.1007/s11104-021-04909-w. [Google Scholar] [CrossRef]
9. Mendes R, Garbeva P, Raaijmakers JM. The rhizosphere microbiome: significance of plant beneficial, plant pathogenic, and human pathogenic microorganisms. FEMS Microbiol Rev. 2013;37(5):634–63. doi:10.1111/1574-6976.12028. [Google Scholar] [PubMed] [CrossRef]
10. van der Putten WH, Bardgett RD, Bever JD, Bezemer TM, Casper BB, Fukami T, et al. Plant-soil feedbacks: the past, the present and future challenges. J Ecol. 2013;101(2):265–76. doi:10.1111/jec.2013.101.issue-2. [Google Scholar] [CrossRef]
11. Salah Ud-Din AIM, Roujeinikova A. Methyl-accepting chemotaxis proteins: a core sensing element in prokaryotes and archaea. Cell Mol Life Sci. 2017;74:3293–303. doi:10.1007/s00018-017-2514-0. [Google Scholar] [PubMed] [CrossRef]
12. Parkinson JS, Hazelbauer GL, Falke JJ. Signaling and sensory adaptation in Escherichia coli chemoreceptors: 2015 update. Trends Microbiol. 2015;23(5):257–66. doi:10.1016/j.tim.2015.03.003. [Google Scholar] [PubMed] [CrossRef]
13. Feng H, Fu R, Hou X, Lv Y, Zhang N, Liu Y, et al. Chemotaxis of beneficial rhizobacteria to root exudates: the first step towards root-microbe rhizosphere interactions. IJMS. 2021;22(13):6655. doi:10.3390/ijms22136655. [Google Scholar] [PubMed] [CrossRef]
14. Sourjik V, Wingreen NS. Responding to chemical gradients: bacterial chemotaxis. Curr Opin Cell Biol. 2012;24(2):262–8. doi:10.1016/j.ceb.2011.11.008. [Google Scholar] [PubMed] [CrossRef]
15. Allard-Massicotte R, Tessier L, Lécuyer F, Lakshmanan V, Lucier JF, Garneau D, et al. Bacillus subtilis early colonization of Arabidopsis thaliana roots involves multiple chemotaxis receptors. mBio. 2016;7(6). [Google Scholar]
16. Feng H, Zhang N, Du W, Zhang H, Liu Y, Fu R, et al. Identification of chemotaxis compounds in root exudates and their sensing chemoreceptors in plant-growth-promoting rhizobacteria bacillus amyloliquefaciens SQR9. Mol Plant Microbe Interact. 2018;31(10):995–1005. doi:10.1094/MPMI-01-18-0003-R. [Google Scholar] [PubMed] [CrossRef]
17. Jin Y, Zhu H, Luo S, Yang W, Zhang L, Li S, et al. Role of maize root exudates in promotion of colonization of bacillus velezensis strain S3-1 in rhizosphere soil and root tissue. Curr Microbiol. 2019;76:855–62. doi:10.1007/s00284-019-01699-4. [Google Scholar] [PubMed] [CrossRef]
18. Yuan Y, Zuo J, Zhang H, Zu M, Liu S. The Chinese medicinal plants rhizosphere: metabolites, microorganisms, and interaction. Rhizosphere. 2022;22:100540. doi:10.1016/j.rhisph.2022.100540. [Google Scholar] [CrossRef]
19. Vives-Peris V, de Ollas C, Gómez-Cadenas A, Pérez-Clemente RM. Root exudates: from plant to rhizosphere and beyond. Plant Cell Rep. 2020;39(1):3–17. doi:10.1007/s00299-019-02447-5. [Google Scholar] [PubMed] [CrossRef]
20. Fagorzi C, Mengoni A. Endophytes: improving plant performance. Microorganisms. 2022;10:1777. doi:10.3390/microorganisms10091777. [Google Scholar] [PubMed] [CrossRef]
21. Sun H, Jiang S, Jiang C, Wu C, Gao M, Wang Q. A review of root exudates and rhizosphere microbiome for crop production. Environ Sci Pollut Res. 2021;28:54497–510. doi:10.1007/s11356-021-15838-7. [Google Scholar] [PubMed] [CrossRef]
22. Wang W, Jia T, Qi T, Li S, Degen AA, Han J, et al. Root exudates enhanced rhizobacteria complexity and microbial carbon metabolism of toxic plants. iScience. 2022;25(10):1–19. [Google Scholar]
23. Frank AC, Saldierna Guzmán JP, Shay JE. Transmission of bacterial endophytes. Microorganisms. 2017;5(4):70. doi:10.3390/microorganisms5040070. [Google Scholar] [PubMed] [CrossRef]
24. Dharampal PS, Carlson C, Currie CR, Steffan SA. Pollen-borne microbes shape bee fitness. In: Proceedings of the Royal Society B: Biological Sciences; 2019. vol. 286, no. 1904, 20182894. [Google Scholar]
25. Dharampal PS, Hetherington MC, Steffan SA. Microbes make the meal: oligolectic bees require microbes within their host pollen to thrive. Ecol Entomol. 2020;45(6):1418–27. doi:10.1111/een.v45.6. [Google Scholar] [CrossRef]
26. Friedle C, D’Alvise P, Schweikert K, Wallner K, Hasselmann M. Changes of microorganism composition in fresh and stored bee pollen from Southern Germany. Environ Sci Pollut Res. 2021;28:47251–61. doi:10.1007/s11356-021-13932-4. [Google Scholar] [PubMed] [CrossRef]
27. Obersteiner A, Gilles S, Frank U, Beck I, Häring F, Ernst D, et al. Pollen-associated microbiome correlates with pollution parameters and the allergenicity of pollen. PLoS One. 2016;11(2):e0149545. doi:10.1371/journal.pone.0149545. [Google Scholar] [PubMed] [CrossRef]
28. Fouda A, Eid AM, Elsaied A, El-Belely EF, Barghoth MG, Azab E, et al. Plant growth-promoting endophytic bacterial community inhabiting the leaves of Pulicaria incisa (Lam.) DC inherent to arid regions. Plants. 2021;10(1):76. doi:10.3390/plants10010076. [Google Scholar] [PubMed] [CrossRef]
29. Gravel V, Antoun H, Tweddell RJ. Growth stimulation and fruit yield improvement of greenhouse tomato plants by inoculation with Pseudomonas putida or Trichoderma atroviride: possible role of indole acetic acid (IAA). Soil Biol Biochem. 2007;39(8):1968–77. doi:10.1016/j.soilbio.2007.02.015. [Google Scholar] [CrossRef]
30. Tariq MR, Shaheen F, Mustafa S, Ali S, Fatima A, Shafiq M, et al. Phosphate solubilizing microorganisms isolated from medicinal plants improve growth of mint. PeerJ. 2022;10:e13782. doi:10.7717/peerj.13782. [Google Scholar] [PubMed] [CrossRef]
31. Fasusi OA, Cruz C, Babalola OO. Agricultural sustainability: microbial biofertilizers in rhizosphere management. Agriculture. 2021;11(2):163. doi:10.3390/agriculture11020163. [Google Scholar] [CrossRef]
32. Domenico P, Roberto F, Damiano S. Microbial biofertilisers in plant production and resistance: a review. Agriculture. 2023;13(9):1666. doi:10.3390/agriculture13091666. [Google Scholar] [CrossRef]
33. Song Q, Song X, Deng X, Luo J, Wang J, Min K, et al. Effects of plant growth promoting Rhizobacteria microbial on the growth, rhizosphere soil properties, and bacterial community of Pinus sylvestris var. mongolica seedlings. Scand J For Res. 2021;36(4):249–62. doi:10.1080/02827581.2021.1917649. [Google Scholar] [CrossRef]
34. Song X, Wu H, Yin Z, Lian M, Yin C. Endophytic bacteria isolated from Panax ginseng improves ginsenoside accumulation in adventitious ginseng root culture. Molecules. 2017;22(6):837. doi:10.3390/molecules22060837. [Google Scholar] [PubMed] [CrossRef]
35. Wang SS, Liu JM, Sun J, Huang YT, Jin N, Li MM, et al. Analysis of endophytic bacterial diversity from different dendrobium stems and discovery of an endophyte produced dendrobine-type sesquiterpenoid alkaloids. Front Microbiol. 2022;12:775665. doi:10.3389/fmicb.2021.775665. [Google Scholar] [PubMed] [CrossRef]
36. Waqas M, Khan AL, Shahzad R, Ullah I, Khan AR, Lee IJ. Mutualistic fungal endophytes produce phytohormones and organic acids that promote japonica rice plant growth under prolonged heat stress. J Zhejiang Univ-SCIENCE B. 2015;16(12):1011 (In Chinese). doi:10.1631/jzus.B1500081. [Google Scholar] [PubMed] [CrossRef]
37. Ding L, Görls H, Hertweck C. Plant-like cadinane sesquiterpenes from an actinobacterial mangrove endophyte. Magn Reson Chem. 2021;59(1):34–42. doi:10.1002/mrc.v59.1. [Google Scholar] [CrossRef]
38. He J, Yang MS, Wang WX, Li ZH, Elkhateeb WAM, Wen TC, et al. Anti-phytopathogenic sesquiterpenoid-xanthone adducts from potato endophytic fungus Bipolaris eleusines. RSC Adv. 2019;9(1):128–31. doi:10.1039/C8RA09861A. [Google Scholar] [PubMed] [CrossRef]
39. Bodede O, Kuali M, Prinsloo G, Moodley R, Govinden R. Anti-Pseudomonas aeruginosa activity of a C16-terpene dilactone isolated from the endophytic fungus Neofusicoccum luteum of Kigelia africana (Lam.). Sci Rep. 2022;12(1):780. doi:10.1038/s41598-021-04747-x. [Google Scholar] [PubMed] [CrossRef]
40. Li N, Xu D, Huang RH, Zheng JY, Liu YY, Hu BS, et al. A new source of diterpene lactones from Andrographis paniculata (Burm. f.) Nees—Two endophytic fungi of Colletotrichum sp. with antibacterial and antioxidant activities. Front Microbiol. 2022;13:819770. doi:10.3389/fmicb.2022.819770. [Google Scholar] [PubMed] [CrossRef]
41. Jin Z, Gao L, Zhang L, Liu T, Yu F, Zhang Z, et al. Antimicrobial activity of saponins produced by two novel endophytic fungi from Panax notoginseng. Nat Prod Res. 2017;31(22):2700–3. doi:10.1080/14786419.2017.1292265. [Google Scholar] [PubMed] [CrossRef]
42. Pandey SS, Singh S, Babu CSV, Shanker K, Srivastava NK, Kalra A. Endophytes of opium poppy differentially modulate host plant productivity and genes for the biosynthetic pathway of benzylisoquinoline alkaloids. Planta. 2016;243:1097–114. doi:10.1007/s00425-016-2467-9. [Google Scholar] [PubMed] [CrossRef]
43. Zhou JY, Sun K, Chen F, Yuan J, Li X, Dai CC. Endophytic Pseudomonas induces metabolic flux changes that enhance medicinal sesquiterpenoid accumulation in Atractylodes lancea. Plant Physiol Biochem. 2018;130:473–81. doi:10.1016/j.plaphy.2018.07.016. [Google Scholar] [PubMed] [CrossRef]
44. Kim JH. Pharmacological and medical applications of Panax ginseng and ginsenosides: a review for use in cardiovascular diseases. J Ginseng Res. 2018;42(3):264–9. doi:10.1016/j.jgr.2017.10.004. [Google Scholar] [PubMed] [CrossRef]
45. Wei G, Chen Z, Wang B, Wei F, Zhang G, Wang Y, et al. Endophytes isolated from Panax notoginseng converted ginsenosides. Microb Biotechnol. 2021;14(4):1730–46. doi:10.1111/mbt2.v14.4. [Google Scholar] [CrossRef]
46. Maftuah E, Saleh M, Pratiwi E. The potentials of biochar from agriculturalwaste as a carrier material of biofertilizer for swamplands. IOP Conf Series: Mat Sci Eng. 2021;980:012064. doi:10.1088/1757-899X/980/1/012064. [Google Scholar] [CrossRef]
47. Wang K, Hou J, Zhang S, Hu W, Yi G, Chen W, et al. Preparation of a new biochar-based microbial fertilizer: nutrient release patterns and synergistic mechanisms to improve soil fertility. Sci Total Environ. 2023;860:160478. doi:10.1016/j.scitotenv.2022.160478. [Google Scholar] [PubMed] [CrossRef]
48. Liu Y, Yang SQ, Zhang WF, Lou S. Effects of microbial fertilizer on physicochemical properties and bacterial communities of saline soil under brackish water irrigation. Huanjing Kexue. 2023;44(8):4585–98 (In Chinese). [Google Scholar] [PubMed]
49. Wu L, Chen J, Wu H, Qin X, Wang J, Wu Y, et al. Insights into the regulation of rhizosphere bacterial communities by application of bio-organic fertilizer in Pseudostellaria heterophylla monoculture regime. Front Microbiol. 2016;7:218466. [Google Scholar]
50. Liu N, Shao C, Sun H, Liu Z, Guan Y, Wu L, et al. Arbuscular mycorrhizal fungi biofertilizer improves American ginseng (Panax quinquefolius L.) growth under the continuous cropping regime. Geoderma. 2020;363:114155. doi:10.1016/j.geoderma.2019.114155. [Google Scholar] [CrossRef]
51. Huang LF, Song LX, Xia XJ, Mao WH, Shi K, Zhou YH, et al. Plant-soil feedbacks and soil sickness: from mechanisms to application in agriculture. J Chem Ecol. 2013;39:232–42. doi:10.1007/s10886-013-0244-9. [Google Scholar] [PubMed] [CrossRef]
52. Wu L, Wang J, Huang W, Wu H, Chen J, Yang Y, et al. Plant-microbe rhizosphere interactions mediated by Rehmannia glutinosa root exudates under consecutive monoculture. Sci Rep. 2015;5(1):15871. doi:10.1038/srep15871. [Google Scholar] [PubMed] [CrossRef]
53. Miao X, Wang E, Zhou Y, Zhan Y, Yan N, Chen C, et al. Effect of ginsenosides on microbial community and enzyme activity in continuous cropping soil of ginseng. Front Microbiol. 2023;14:1060282. doi:10.3389/fmicb.2023.1060282. [Google Scholar] [PubMed] [CrossRef]
54. Bao L, Liu Y, Ding Y, Shang J, Wei Y, Tan Y, et al. Interactions between phenolic acids and microorganisms in rhizospheric soil from continuous cropping of panax notoginseng. Front Microbiol. 2022;13:791603. doi:10.3389/fmicb.2022.791603. [Google Scholar] [PubMed] [CrossRef]
55. Bao L, Liu Y, Chen J, Ding Y, Shang J, Li J, et al. Different effects of six saponins on the rhizosphere soil microorganisms of Panax notoginseng. Plant Soil. 2023;487:389–406. doi:10.1007/s11104-023-05934-7. [Google Scholar] [CrossRef]
56. Zhang XH, Lang DY, Zhang EH, Wang ZS. Effect of autotoxicity and soil microbes in continuous cropping soil on angelica sinensis seedling growth and rhizosphere soil microbial population. Chinese Herbal Med. 2015;7(1):88–93 (In Chinese). doi:10.1016/S1674-6384(15)60025-9. [Google Scholar] [CrossRef]
57. Li L, Tong L, Lv Y. Influence of bio-fertilizer type and amount jointly on microbial community composition, crop production and soil health. Agronomy. 2023;13(7):1775. doi:10.3390/agronomy13071775. [Google Scholar] [CrossRef]
58. Nurhafizhoh Z, Mohd Fahmi K, Sharifah Azura Syed I, Mohamed Mazmira Mohd M. Effect of integrated biofertilizers with chemical fertilizers on the oil palm growth and soil microbial diversity. Biocatal Agric Biotechnol. 2022;39:102237. doi:10.1016/j.bcab.2021.102237. [Google Scholar] [CrossRef]
59. He Z, Mao R, Dong J, Liang Z, Zhang H, Liu L. Remediation of deterioration in microbial structure in continuous Pinellia ternata cropping soil by crop rotation. Can J Microbiol. 2019;65(4):282–95. doi:10.1139/cjm-2018-0409. [Google Scholar] [PubMed] [CrossRef]
60. Wang F, Zhang X, Wei M, Wang Y, Liang Z, Xia P. Appropriate crop rotation alleviates continuous cropping barriers by changing rhizosphere microorganisms in Panax notoginseng. Rhizosphere. 2022;23:100568. doi:10.1016/j.rhisph.2022.100568. [Google Scholar] [CrossRef]
61. Zhang J, Wei L, Yang J, Ahmed W, Wang Y, Fu L, et al. Probiotic consortia: reshaping the rhizospheric microbiome and its role in suppressing root-rot disease of panax notoginseng. Front Microbiol. 2020;11:513301. [Google Scholar]
62. Wu W, Chen W, Liu S, Wu J, Zhu Y, Qin L, et al. Beneficial relationships between endophytic bacteria and medicinal plants. Front Plant Sci. 2021;12:646146. doi:10.3389/fpls.2021.646146. [Google Scholar] [PubMed] [CrossRef]
63. Souza LA, Monteiro CC, Carvalho RF, Gratão PL, Azevedo RA. Dealing with abiotic stresses: an integrative view of how phytohormones control abiotic stress-induced oxidative stress. Theor Exp Plant Physiol. 2017;29:109–27. doi:10.1007/s40626-017-0088-8. [Google Scholar] [CrossRef]
64. Waadt R, Seller CA, Hsu P-K, Takahashi Y, Munemasa S, Schroeder JI. Plant hormone regulation of abiotic stress responses. Nat Rev Mol Cell Biol. 2022;23(10):680–94. doi:10.1038/s41580-022-00479-6. [Google Scholar] [PubMed] [CrossRef]
65. Etesami H, Maheshwari DK. Use of plant growth promoting rhizobacteria (PGPRs) with multiple plant growth promoting traits in stress agriculture: action mechanisms and future prospects. Ecotoxicol Environ Saf. 2018;156:225–46. doi:10.1016/j.ecoenv.2018.03.013. [Google Scholar] [PubMed] [CrossRef]
66. Chen H, Bullock DA, Alonso JM, Stepanova AN. To fight or to grow: the balancing role of ethylene in plant abiotic stress responses. Plants. 2021;11(1):33. doi:10.3390/plants11010033. [Google Scholar] [PubMed] [CrossRef]
67. Gamalero E, Lingua G, Glick BR. Ethylene, ACC, and the plant growth-promoting enzyme ACC deaminase. Biology. 2023;12(8):1043. doi:10.3390/biology12081043. [Google Scholar] [PubMed] [CrossRef]
68. Naing AH, Maung TT, Kim CK. The ACC deaminase-producing plant growth-promoting bacteria: influences of bacterial strains and ACC deaminase activities in plant tolerance to abiotic stress. Physiol Plant. 2021;173(4):1992–2012. doi:10.1111/ppl.v173.4. [Google Scholar] [CrossRef]
69. Glick BR. Bacteria with ACC deaminase can promote plant growth and help to feed the world. Microbiol Res. 2014;169(1):30–9. doi:10.1016/j.micres.2013.09.009. [Google Scholar] [PubMed] [CrossRef]
70. Aasfar A, Bargaz A, Yaakoubi K, Hilali A, Bennis I, Zeroual Y, et al. Nitrogen fixing azotobacter species as potential soil biological enhancers for crop nutrition and yield stability. Front Microbiol. 2021;12:628379. doi:10.3389/fmicb.2021.628379. [Google Scholar] [PubMed] [CrossRef]
71. Ghori NH, Ghori T, Hayat MQ, Imadi SR, Gul A, Altay V, et al. Heavy metal stress and responses in plants. Int J Environ Sci Technol. 2019;16:1807–28. doi:10.1007/s13762-019-02215-8. [Google Scholar] [CrossRef]
72. Etesami H. Bacterial mediated alleviation of heavy metal stress and decreased accumulation of metals in plant tissues: mechanisms and future prospects. Ecotoxicol Environ Saf. 2018;147:175–91. doi:10.1016/j.ecoenv.2017.08.032. [Google Scholar] [PubMed] [CrossRef]
73. Guo LP, Wang TL, Yang WZ, Zhou LY, Chen NF, Han BX, et al. Ecological agriculture—The only way for traditional Chinese medicine agriculture. Chin J Chin Mater Med. 2017;42(2):231–8 (In Chinese). [Google Scholar]
74. Wang MJ, Long L. Research progress on extraction, purification, structure identification and pharmacological effects of Lycium barbarum polysaccharides. Food Ferment Technol. 2022;58(1):131–5+46 (In Chinese). [Google Scholar]
75. Ying ZR, Huang JC, Gui LG, Lei JY, Zhao Y. Effects of microbial fertilizer on physical and chemical properties of primary saline alkali soil and growth of Lycium barbarum. Ningxia Agric For Sci Technol. 2018;59(12):48–51+75 (In Chinese). [Google Scholar]
76. Li XG, Zhou QQ, Li J, Wang YK. Effects of two microbial fertilizers on yield and quality of Lycium barbarum in Jingdian Irrigation area. J Sichuan Agric Univ. 2013;31(2):6 (In Chinese). [Google Scholar]
77. Xiong CX, Zhang J, Jiang FT, Cui JB, Niluo ZJ, Zhang J, et al. Effects of different microbial fertilizer treatments on yield and quality of Corydalis yanhusuo. J Southern Agric. 2020;51(5):1159–68 (In Chinese). [Google Scholar]
78. Dong TW. Effects of combined application of nitrogen and phosphorus fertilizer and microbial fertilizer on growth and quality of 2-year-old Fritillaria thunbergii (Master Thesis). Northwest A&F University; 2018 (In Chinese). [Google Scholar]
79. Wu XF, Zhao GX, Lu M, Yang KF. Effect of a new microbial fertilizer woyiduo on the yield and quality of turmeric. Sci Technol Sichuan Agric. 2017;(9):36–40 (In Chinese). [Google Scholar]
80. Zhang YY, Sun H, Wu LJ, Xu ST, Tan YF. Effects of multifunctional microbial agents on soil bulk density and Ginsenosides of cultivated ginseng in farmland. Spec Res. 2010;32(1):35–7+48 (In Chinese). [Google Scholar]
81. Tan JF, Chen WX, Li Q. Study on increasing tanshinone content by bio organic fertilizer. Agric Technol. 2019;39(24):52–5 (In Chinese). [Google Scholar]
82. Chen QD. Effect of microbial fertilizer on growth and development of Lycium barbarum (Master ThesisGansu Agricultural University; 2014 (In Chinese). [Google Scholar]
83. Guo ZX, Niu YY, Xu SH, Sun XR, Li LZ, Li J. Effects of microbial fertilizer on growth, yield and quality of continuous cropping Rehmannia glutinosa. Northern Hortic. 2022;(19):100–6 (In Chinese). [Google Scholar]
84. Li Q, Wang YQ, Peng T, Yao YY, Ling QR, Dang SR. Effect of compound microbial fertilizer on quality of Angelica sinensis. Jiangsu Agric Sci. 2021;49(9):117–22 (In Chinese). [Google Scholar]
85. Rivett DW, Jones ML, Ramoneda J, Mombrikotb SB, Ransome E, Bell T. Elevated success of multispecies bacterial invasions impacts community composition during ecological succession. Ecol Lett. 2018;21(4):516–24. doi:10.1111/ele.12916. [Google Scholar] [PubMed] [CrossRef]
86. Xavier LJC, Germida JJ. Selective interactions between arbuscular mycorrhizal fungi and Rhizobium leguminosarum bv. viceae enhance pea yield and nutrition. Biol Fertil Soils. 2003;37:261–7. doi:10.1007/s00374-003-0605-6. [Google Scholar] [CrossRef]
87. Wang X, Fang L, Beiyuan J, Cui Y, Peng Q, Zhu S, et al. Improvement of alfalfa resistance against Cd stress through rhizobia and arbuscular mycorrhiza fungi co-inoculation in Cd-contaminated soil. Environ Pollut. 2021;277:116758. doi:10.1016/j.envpol.2021.116758. [Google Scholar] [PubMed] [CrossRef]
88. Yardin MR, Kennedy IR, Thies JE. Development of high quality carrier materials for field delivery of key microorganisms used as bio-fertilisers and bio-pesticides. Radiat Phys Chem. 2000;57(3–6):565–8. [Google Scholar]
89. Bai Y, Feng P, Chen W, Xu S, Liang J, Jia J. Effect of three microbial fertilizer carriers on water infiltration and evaporation, microbial community and alfalfa growth in saline-alkaline soil. Commun Soil Sci Plant Anal. 2021;52(20):2462–70. doi:10.1080/00103624.2021.1928176. [Google Scholar] [CrossRef]
90. Isabel JB, Balamurugan A, Devi PR, Selvakumar P. Chitosan-encapsulated microbial biofertilizer: a breakthrough for enhanced tomato crop productivity. Int J Biol Macromol. 2024;260:129462. doi:10.1016/j.ijbiomac.2024.129462. [Google Scholar] [PubMed] [CrossRef]
91. Akhtar N, Ilyas N, Meraj TA, Pour-Aboughadareh A, Sayyed RZ, Mashwani ZUR, et al. Improvement of plant responses by nanobiofertilizer: a step towards sustainable agriculture. Nanomater. 2022;12(6):965. doi:10.3390/nano12060965. [Google Scholar] [PubMed] [CrossRef]
92. Zhu L, Yan H, Liu P, Zhang ZY, Zhang S, Guo S, et al. Research progress on the effect of rhizosphere microorganisms of medicinal plants on their quality formation and its mechanism. Chinese Herbal Med. 2021;52(13):4064–73 (In Chinese). [Google Scholar]
93. Liu L, Wang X, Chen S, Liu D, Song C, Yi S, et al. Fungal isolates influence the quality of Peucedanum praeruptorum Dunn. Front Plant Sci. 2022;13:1011001. doi:10.3389/fpls.2022.1011001. [Google Scholar] [PubMed] [CrossRef]
94. Wha KJ, Gyu CH, Hoon SJ, Sung KK, Hee SS. Bioactive secondary metabolites from an endophytic fungus Phoma sp. PF2 derived from Artemisia princeps Pamp. J Antibiot. 2019;72(3):174–7. doi:10.1038/s41429-018-0131-2. [Google Scholar] [PubMed] [CrossRef]
95. Patrycja G, Magdalena W, Gauravi A, Dnyaneshwar R, Hanna D, Mahendra R. Endophytic actinobacteria of medicinal plants: diversity and bioactivity. Antonie van Leeuwenhoek. 2015;108(2):267–89. doi:10.1007/s10482-015-0502-7. [Google Scholar] [PubMed] [CrossRef]
96. Qi C, Gao W, Wang J, Liu M, Zhang J, Chen C, et al. Terrusnolides A-D, new butenolides with anti-inflammatory activities from an endophytic Aspergillus from Tripterygium wilfordii. Fitoterapia. 2018;130:134–9. doi:10.1016/j.fitote.2018.08.021. [Google Scholar] [PubMed] [CrossRef]
Cite This Article
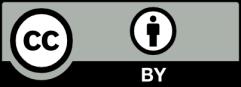
This work is licensed under a Creative Commons Attribution 4.0 International License , which permits unrestricted use, distribution, and reproduction in any medium, provided the original work is properly cited.