Open Access
ARTICLE
The NAC Transcription Factor ANAC089 Modulates Seed Vigor through the ABI5-VTC2 Module in Arabidopsis thaliana
Shanghai Key Laboratory of Bio-Energy Crops, School of Life Sciences, Shanghai University, Shanghai, 200444, China
* Corresponding Authors: Ping Li. Email: ; Xiangyang Hu. Email:
Phyton-International Journal of Experimental Botany 2024, 93(6), 1101-1116. https://doi.org/10.32604/phyton.2024.050220
Received 31 January 2024; Accepted 23 April 2024; Issue published 27 June 2024
Abstract
Seed viability is an essential feature for genetic resource conservation as well as sustainable crop production. Long-term storage induces seed viability deterioration or seed aging, accompanied by the accumulation of toxic reactive oxygen species (ROS) to suppress seed germination. Controlled deterioration treatment (CDT) is a general approach for mimicking seed aging. The transcription factor ANAC089 was previously reported to modulate seed primary germination. In this study, we evaluated the ability of ANAC089 to control seed viability during aging. Compared with that in the wild-type line, the mutation of ANAC089 significantly increased HO, thereby reducing seed viability after CDT, while the overexpression of ANAC089 reduced HO and improved seed longevity, indicating a critical role for ANAC089 in maintaining seed viability through HO signaling. A series of studies have shown that ANAC089 targets and negatively regulates the level of ABI5, an important transmitter of abscisic acid (ABA) signals, to affect seed viability after CDT. Furthermore, ABI5 negatively regulated the expression of VTC2, which is involved in the biosynthesis of the antioxidant ascorbic acid and HO scavenging. As a result, ANAC089 attenuates the generation of HO, thereby enhancing seed viability through the ABI5-VTC2 module during the seed aging process. Taken together, our results reveal a novel mechanism by which ANAC089 enhances seed viability by coordinating ABI5 and VTC2 expression, ultimately preventing the overaccumulation of HO, which would have led to reduced seed viability.Keywords
Supplementary Material
Supplementary Material FilePlant seeds contain plentiful carbohydrates (as in rice seeds) and protein and lipids (as in soybeans), and they are used as staple foods to provide humans with the necessary energy for building human civilization [1,2]. Seeds carry plant genetic information and sexual reproduction to mediate the generational alternation of flowering plants; thus, good germination status after long-term conservation is important for ecological and agronomic value; however, seeds gradually lose their germination viability during storage, a phenomenon called seed aging or deterioration [3–5]. Seed aging is caused by oxidative damage and is influenced by environmental and genetic factors [6,7]. This process is inevitable and irreversible; thus, attenuating seed aging or deterioration can efficiently prolong seed longevity and sustain germination vigor in a dry state. Identifying genes or regulatory networks involved in seed longevity and understanding the mechanism of seed deterioration is important for germplasm resource preservation and crop safety.
Seed viability gradually increases during seed development, reaches a high seed germination capacity during seed maturation, and then decreases during the postharvest period; therefore, seed maturation status determines seed viability or longevity. Four master genes, namely, ABSCISIC ACID INSENSITIVE 3 (ABI3), LEAFY COTYLEDON 1 (Lec1), LEC2, and FUSCA3 (FUS3), play critical roles in seed maturation, and mutation of any of these four genes causes a deficiency in seed maturation, consequently impairing seed viability [8]. For example, both the abi3 and lec1 mutants prevent proper seed storage protein accumulation; thus, they lose their germination viability during the first few weeks of the postharvest period, the abi3 mutant shows ABA insensitivity during seed germination [9,10], and the lec1 mutant shows an abnormal embryo phenotype, lacks protein and oil accumulation and prefers to undergo vivipation. The plant hormone abscisic acid (ABA) also significantly contributes to the seed maturation process and seed vigor [9,11]. The bZIP transcription factor ABI5, an ABA transmitter, reportedly controls seed maturation and vigor in Arabidopsis, legumes, and Medicago [12,13]. Other bZIP transcription factors, such as bZIP23 and bZIP42, also regulate ride seed vigor [14]. In addition to plant hormones and genetic factors, environmental stress induces the accumulation of reactive oxygen species, which are often believed to be key factors for regulating seed vigor. An appropriate level of ROS precisely controls the seed germination status and degree of deterioration, and a low concentration of ROS can trigger seed germination, but the overaccumulation of ROS is highly toxic to seed proteins, DNA, and lipids [4,15–17]. Accordingly, plants have also evolved sophisticated strategies for ROS detoxification to avoid oxidative damage and sustain seed vigor; for example, tocopherols (vitamin E) are lipophilic antioxidants that are particularly abundant in mature seeds and are important for seed longevity because they prevent lipid peroxidation during germination [18]. Vitamin C, or ascorbic acid (AsA), is also an efficient nonenzymatic antioxidant that scavenges ROS for seed germination under salt stress [19]. Arabidopsis VITAMIC DEFECTIVE 2 (VTC2) encodes the key product of GDP-L-galactose phosphorylation for AsA biosynthesis, and a mutant of vtc2 induces more H2O2 after salt stress [20]. PER1 encodes the seed-specific antioxidant 1-Cys peroxiredoxin, which scavenges ROS to improve seed viability in rice [14], and even ectopic expression of NnPER1 from lotus also enhances seed vigor and longevity in Arabidopsis [21,22]; however, the detailed mechanism underlying seed vigor or longevity needs further investigation.
Arabidopsis ANAC089 is an NAC transcription factor and is also a redox-responsive protein that regulates seed sensitivity to ABA [23–26]. ANAC089 and its two homologs, ANAC060 and ANAC040, are reported to control seed primary germination and dormancy [27]. ANAC089 is also involved in flowering time regulation by affecting the expression of FLC, an important negative factor for initiating flowering [28]. In this study, we investigated the function of ANAC089 in controlling seed vigor and found that ANAC089 attenuated H2O2 accumulation, thereby enhancing seed viability, as characterized by a high percentage of seed germination after seed aging. Furthermore, we found that ANAC089 can bind to the promoter of ABI5, an important transmitter of ABA signals, to suppress its expression. Consistent with these findings, the mutation of ABI5 suppressed the generation of H2O2 to increase seed vigor, but the overexpression of ABI5 increased H2O2 to decrease seed vigor. Further study revealed that ABI5 orchestrates endogenous ROS levels by negatively regulating VTC2. Thus, our data reveal the novel function of ANAC089 in controlling seed vigor and longevity through the targeting of ABI5 and downstream VTC2, which ultimately scavenges toxic H2O2. This study expands our knowledge of the use of ANAC089 for seed conservation and sustainable agricultural production.
2.1 Plant Materials and Growth
All Arabidopsis (Arabidopsis thaliana) materials, including abi5 and ABI5-MYC provided by Chuanyou Li’s laboratory (Institute of Genetics, Chinese Academy of Sciences, Beijing, China) [29] and the vtc2 mutant provided by Kai Shu’s laboratory (Northwestern Polytechnical University, Xi’an, China) [19], were surface-sterilized with bleach, plated on half-strength Murashige and Skoog (1/2 MS) media containing 0.8% (w/v) agar and 1% (w/v) sucrose (pH 5.7) and grown at 22°C under long days (16-h light [100 μmol−2s−1]/8-h darkness) or intermediate days (12-h light [100 μmol−2s−1]/12-h darkness). Adult plants were grown under long-day conditions at 22°C in a growth chamber with a 16-h white light (50 μmol m−2s −1)/8-h dark cycle. Homozygous seeds in each batch were harvested at the same time and dried in an incubator at 22°C for approximately 2 months before the germination assays.
2.2 Generation of Gene-Editing Lines
The target sequence with the PAM structure (NGG) was predicted and selected by target design online software (http://skl.scau.edu.cn/targetdesign/) according to a previously reported method [30]. The oligonucleotide containing this target sequence was synthesized and digested by the BsaI enzyme and then inserted into the pCAMBIA-CAS9 vector to generate the gene-editing construct. The resulting construct was subsequently transformed into Agrobacterium GV3101 for Arabidopsis transformation. The resulting gene-editing lines were screened on MS media supplemented with 35 mg/L hygromycin, and the nucleotide deletion pattern around the target site within the ANAC089 genome locus was determined via PCR and Sanger sequencing.
To obtain the 35S: ANAC089-Flag or 35S: VTC2-Flag construct, the full-length fragment of ANAC089 or VTC2 was linked with a Flag tag through PCR amplification using the PrimeSTAR enzyme (Takara, Japan), and then recombined into the pRI101-AN vector through the In-Fusion cloning kit (Clontech, Dalian, China). The construct was transformed into Arabidopsis thaliana (Col-0) plants through Agrobacterium (Agrobacterium tumefaciens)-mediated floral-dipping transformation. The transgenic seeds were collected and sowed on the 1/2 MS medium, and the transgenic line was screened with 50 mg L−1 kanamycin. After three generations of screening on MS media supplemented with kanamycin, the homozygous seeds were used for seed viability analysis.
2.4 Seed Viability Measurement by Aged Treatment and Germination Analysis
Seed viability was evaluated by controlled deterioration treatment (CDT) as previously described [24,31]. In brief, the mature seeds were collected and dried in paper bags at room temperature for two months. The dried seeds were loaded in tubes without lids, and the tubes were placed on a copper wire mesh tray in a desiccator. Approximately 1 L of saturated NaCl solution with 0.01% NaClO was kept below the tray, and then the lid of the desiccator was sealed to ensure that the relative humidity inside was approximately 80%. After 2 days of water equilibration at room temperature, the seeds were aged by placing the desiccator in an incubator at 42°C for 4 days (4 d CDT) and then removed from the desiccator and dried at 25°C for 2 days before the germination assay. Seeds were considered to have completed germination when they showed a >2 mm radicle. The percentage of germinated seeds was recorded after 5 days of germination on 1/2 MS solid media.
2.5 Seed Viability Measurement by Tetrazolium Assay
To measure seed viability after aging, a tetrazolium (TTZ, 2,3,5-triphenyl tetrazolium chloride) assay was used as described previously [31]. Briefly, the aged seeds were gently scratched on an emery cloth, incubated in 1% TTZ solution at 25°C for 48 h, and then washed three times with double distilled water. Pictures were taken using a Zeiss stereomicroscope.
The H2O2 content was measured according to a previous method [14]. Approximately 10 mg of Arabidopsis seeds were ground in 1 mL of precooled acetone. The homogenate was centrifuged for 10 min at 15,000 × g. A total of 1 mL of supernatant, 0.1 mL of titanium reagent, and 0.2 mL of ammonium solution were mixed to precipitate the titanium–hydroperoxide complex. After centrifugation at 5,000 × g for 10 min, the precipitate was dissolved in 1 mL of 2 M H2SO4. H2O2 was measured using an ultraviolet (UV)--visible spectrophotometer and the absolute amount was calculated according to a standard curve.
Total RNA was extracted from hydrated seeds using TRIzol reagent (Invitrogen, Waltham, MA, 02451, USA). RT–qPCR was performed as the used method by Li et al. [32]. Briefly, first-strand cDNA was synthesized from 1.5 µg of DNase-treated RNA in a 20 µL reaction volume using M-MuLV reverse transcriptase (Fermentas, Visalia, CA, 93291, USA) with oligo (dT)18 primers. For qPCR, cDNA samples were diluted to 2–10 ng μL−1, and PCR was performed in the presence of SYBR Green I Master Mix on a Roche LightCycler 480 real-time PCR machine according to the manufacturer’s instructions. All RT–qPCR experiments were independently performed in triplicate, and representative results are shown. PROTEIN PHOSPHATASE 2A (PP2A) was used as the internal control. The prprimerairs used for quantitative RT–qPCR are listed in Table S1.
2.8 Protein Immunoblot Analysis
Total protein was extracted from the seedlings using the method of Li et al. [32,33]. Extracted proteins (15 μg aliquot) were separated with a 12% SDS–polyacrylamide gel, and then the gel was transferred onto polyvinylidene difluoride (PVDF) membranes. After washing with 10 mM PBS buffer (pH = 7.5) three times, the membrane was incubated with the appropriate primary antibody for 30 min at room temperature, and then washed three times with PBS buffer for 15 min each time to remove the unbound antibody, and then the washed membrane was incubated with a secondary horseradish peroxidase-conjugated goat anti-mouse or anti-rat secondary antibody (1:3,000; Promega, Madison, WI, 53711, USA). Signals were detected using the ECL Kit (GeneScript, Nanjing, China). The primary antibody, including anti-ABI5 (1:1,000, Abcam, Shanghai, China), anti-GST (1:3,000, Invitrogen), anti-FLAG (1:3,000; Sigma–Aldrich, St. Louis, MO, 63178, USA), or anti-Actin (1:1,000; Sigma–Aldrich) antibody was used.
2.9 Protoplast Transient Expression Assay
For the transient expression assay, the 2.4-kb ABI5 fragment upstream of the start codon of ABI5 was used as the promoter, such fragment was inserted into the pGreenII 0800-LUC vector to generate the pABI5-LUC reporter construct. The coding sequence of ANAC089-Flag was inserted into the pGreenII 62-SK vector under the control of the 35S promoter as the effecter construct. All primer information for generating these constructs is provided in Table S1. The mesophyll protoplasts were produced from Arabidopsis leaves as the method of Li et al. [32], the purified protoplast was then subsequently transfections with the constructs as above. A dual-luciferase reporter assay system (Promega) was performed, and the activities of firefly Luc and Renilla luciferase (REN) were measured. Relative LUC/REN ratios were used to compare the transcriptional activity of corresponding promoters.
2.10 Electrophoretic Mobility Shift Assay (EMSA)
The ability of ANAC089 to bind to the ABI5 promoter was measured by EMSA as described previously [32,33]. Briefly, oligonucleotide probes containing the G-box motif were synthesized, annealed, and labeled using a Biotin-DNA labeling kit (Pierce, Invitrogen, Waltham, MA, 02451, USA). The E. coli BL21 strain transformed with pGST-ANAC089 was induced with 0.1 mM isopropyl β-d-1-thiogalactopyranoside at 28°C when the OD600 was 1.5. The recombination GST-ANAC089 protein was purified from the crude bacterium protein by GST resin affinity chromatography (Invitrogen). The oligonucleotide probes containing the wild-type or mutated CACGTG motif were labeled with biotin and synthesized. These unmutated or mutated oligonucleotides without biotin labeling were used as cold probes or cold mutated probes, respectively. The binding ability of the labeled probe with the purified protein was checked on the nitrocellulose membrane, and the chemiluminescence of the biotin-labeled DNA was detected using a Light Shift Chemiluminescent EMSA Kit (Pierce).
The data is presented as the means ± SD of three or five biological replicates for each sample. Using the acquired data, a two-way analysis of variance (ANOVA) following Tukey’s test was performed among different groups, or a student’s t-test was used to compare the difference between only two groups. The statistical analyses were carried out using IBM SPSS Statistics 22 (IBM SPSS, Chicago, IL, USA).
3.1 ANAC089 Controls Seed Viability
A previous study showed that ANAC089 controls the cytosolic redox status during seed germination under ABA stress [26], and we wondered whether ANAC089 also regulates seed viability. To test this hypothesis, we first applied a gene-editing approach to generate a series of ANAC089 mutants. As shown in Figs. 1A and 1B, we successfully deleted one nucleotide (deletion A) in the secondary exon of ANAC089, causing a codon shift to inactive ANAC089; this mutant was named anac089-1. We also obtained another gene-editing mutant by deleting a nucleotide (GCAA) in the secondary exon; this line was named anac089-2. The ANAC089 locus in these two lines was sequenced, and successful deletion of this locus was confirmed (Fig. 1B). Moreover, we generated a transgenic line overexpressing the ANAC089-Flag fusion gene under the control of the constitutive 35S promoter. A strong immunoblot signal was also successfully detected in several individual transgenic lines by western blot analysis using an anti-Flag antibody (Fig. 1C).
Figure 1: ANAC089 regulates seed viability (A) Identification of the anac089-1 mutant. A T-DNA fragment is inserted in the first exon to disrupt the functional transcript of ANAC089. (B) Sequencing of the gene editing site of the anac089-2 mutant. One nucleotide was deleted in the ANAC089 genomic locus by a gene editing approach, and this deletion was confirmed by Sanger sequencing. (C) Verification of the transgenic ANAC089-Flag line by western blot analysis. (D and E) Different seed germination percentages among Col, anac089-1, anac089-2, and ANAC089-Flag after 4 days of CDT aging treatment. The seeds were stained with TTC to determine seed viability. Red indicates seeds with high viability, and light yellow indicates that the seeds lost viability. An image was taken after staining (C). After 4 days of CDT treatment, the seeds were placed under normal conditions at 22°C for 5 days, after which the percentage of germinated seeds was recorded. The experiment was performed in triplicate, and the data are presented as the mean ± SD. Bars with different letters are significantly different at p < 0.05 (two-way ANOVA with Tukey’s test)
To determine the effect of ANAC089 on seed viability, we applied the artificial aging approach (CDT) to simulate the course of seed aging and used the tetrazolium (TDZ) staining method to evaluate seed viability. As shown in Figs. 1D and 1E, after artificial aging treatment, TDZ staining revealed slightly red staining in the wild-type Col seeds and the anac089-1 and anac089-2 gene-edited seeds, suggesting decreased seed viability; in contrast, the seed staining for the ANAC089-Flag line was very strong, suggesting high seed viability. We also tested the seed germination potential of these lines after different durations. After 6 months, 1 or 2 years of conservation, both the Col and ANAC089-Flag still showed high seed germination, but only approximately 44.5% of the anac089-1 mutant seeds and 47.8.2% of the anac089-2 mutant seeds germinated after 1 year of storage, and only 24.3% of the anac089-1 and 21.4% of the anac089-2 mutant seeds germinated after 2 years of storage; However, approximately 76.45% of the ANAC089-Flag seeds germinated well, which was greater than that of the anac089-1 and anac089-2 lines (Fig. S1). Based on these data, we propose that ANAC089 negatively regulates seed viability.
3.2 ANAC089 Controls ROS Levels
A previous study showed that the ROS level, mainly the H2O2 level, affects seed viability [19,20]. Here, we measured the endogenous H2O2 level in the seeds after artificial aging treatment. The artificial aging process increased the endogenous H2O2 content in wild-type Col seeds on the first day, after which the high level of H2O2 was maintained during the following three days of CDT treatment (Fig. 2A), suggesting that a high level of ROS is toxic to seed viability after CDT treatment. We further compared the different H2O2 contents among Col, the anac089 mutant, and its transgenic overexpressing line after 3 days of CDT treatment and found that the H2O2 content in the ANAC089-Flag line was lower than that in the Col or anac089 mutant lines (Fig. 2B). It is possible that the relatively lower level of H2O2 attenuated the damage caused by CDT to seed viability.
Figure 2: ANAC089 affects the generation of H2O2 to control seed viability. (A) The effect of CDT treatment on the transcriptional level of ANAC089 in wild-type Col seeds. The freshly harvested seeds were dried for two weeks and then treated with CDT for the indicated times, after which the treated seeds were subjected to total RNA extraction. The transcriptional level of ANAC089 was measured by RT–qPCR analysis, and PP2A was used as the internal control. The experiment was performed in triplicate, and the bars indicate ±SD. (B) ANAC089 affected the accumulation of H2O2 after seed aging treatment. Seeds of Col, anac089, vtc2, and transgenic ANAC089-Flag line were treated with CDT for 4 days, after which the H2O2 content was measured. Seeds not subjected to CDT treatment were used as controls. The experiment was performed in triplicate, and the bars indicate ±SD. Bars with different letters are significantly different at p < 0.05 (two-way ANOVA with Tukey’s test). (C) Effects of H2O2 and CDT treatment on the percentage of germinated seeds among the Col, anac89, and vtc2 mutant and ANAC089-Flag lines. These seeds were treated with 100 nM H2O2 for 12 h or CDT for 4 days, germinated under normal conditions at 22°C for 5 days, and the percentage of germinated seeds was recorded. The experiment was performed in triplicate, and the bars indicate ±SD. Bars with different letters are significantly different at p < 0.05 (two-way ANOVA with Tukey’s test)
VTC2 is an important antioxidant enzyme that scavenges ROS accumulation in Arabidopsis [20]. We also found that the vtc2 mutant showed low seed viability, accompanied by increased H2O2 accumulation after CDT treatment (Fig. 2B), but additional exogenous AsA enhanced the seed germination of vtc2 after CDT (Fig. S2). These data suggest that VTC2 may be responsible for H2O2 accumulation after CDT treatment. Furthermore, we crossed vtc2 with ANAC089-Flag to obtain the ANAC089-Flag/vtc2 line. Unlike the ANAC089-Flag line, the ANAC089-Flag/vtc2 line exhibited lower seed germination after 3 days of CDT treatment, similar to that of vtc2 (Fig. 2C); the H2O2 content in the ANAC089-Flag/vtc2 seeds was also greater than that in the ANAC089-Flag line. However, the addition of AsA increased seed germination and reduced H2O2 production in ANAC089-Flag/vtc2 seeds after CDT treatment (Fig. S3). Meanwhile, we also direct H2O2 treatment to suppress the seed germination (Fig. S4). These data further support the hypothesis that high levels of ROS impair seed viability through ANAC089 and VTC2.
3.3 ANAC089 Binds to the Promoter of ABI5
It has been reported that the ANAC089 homolog ANAC060 can directly bind to the promoter of ABI5 to suppress ABI5 expression in Arabidopsis [34]. Here we found that CDT induced the expression of ABI5 in the wild-type Col seeds, such induction was attenuated in the ANAC089-Flag line, but increased in the anac89 mutant line (Fig. 3A), suggesting that ANAC089 negatively regulated the expression of ABI5 during CDT. It is possible that ANAC089 also binds to the promoter of ABI5 to suppress its expression.
Figure 3: ANAC089 binds to the promoter of ABI5. (A) RT–qPCR analysis of the transcriptional level of ABI5 in the Col, anac089-1, and ANAC089-Flag lines before or after CDT treatment for 4 days. Seeds treated with or without CDT were hydrated for 12 h, after which total RNA was extracted for RT–qPCR analysis. PP2A was used as the internal control. The experiment was performed in triplicate, and the bars indicate ±SD. Bars with different letters are significantly different at p < 0.05 (two-way ANOVA with Tukey’s test). (B) Chromatin immunoprecipitation (ChIP)-qPCR analysis of the association of ANAC089 with the ABI5 promoter in vivo. Hydrated Col-0 and ANAC089-Falg seeds were used for analysis. Upper panel, diagram of the ABI5 promoter showing the positions of G-boxes (blue rectangles) and the five regions (the P1, the P2, the P3, P4, and E1) used for ChIP–qPCR amplification, as indicated by black lines. Lower panel, fold enrichment of six amplified fragments, as quantified by qPCR. An anti-FLAG antibody was used for ChIP. PP2A served as an internal control, and enrichment values were normalized to the level of input DNA. The values are shown as the means ± standard deviation of triplicate experiments. Asterisks indicate significant differences according to the student’s t-test (**p < 0.01). (C) EMSA assay of the direct binding of the ANAC089-GST protein to probes with a G-box elements in vitro. A biotin-labeled probe with a G-box motif. Such probe sequence information is provided on top, highlighted codons with yellow color indicate the binding motif by ANAC089, while the red color indicates the mutated sequence by replacing the CTCGTG with AAAAAA; cold probe means unlabeled probe (un-labeled cold probe at 10 fold or 50 fold was loaded to compete for the specific binding between the labeled probe and the G-box elements); labeled mProbe means biotin-labeled probe with a mutated G-box (highlighted in red); cold mProbe means unlabeled mutated probe. The black arrows point to DNA/protein complexes and free probes, as indicated
To test this hypothesis, we first performed ChIP to test the ability of ANAC089 to bind to the ABI5 promoter. Using the imbibed seeds of the ANAC089-Flag line and anti-Flag, we found that the P3 fragment containing the NAC-recognizing G-box motif (CACGTG) could be specifically enriched by ANAC089-Flag. As a control, other fragments within the reference gene locus could not be efficiently enriched (Fig. 3B). Furthermore, we used probes containing such motifs as probes and performed EMSAs to test the ability of the ANAC089 protein to bind to these probes. The EMSA results showed that ANAC089 indeed strongly bound to this labeled probe, while the unlabeled cold probe could compete with the bind labeled probe to ANAC089, reducing the accumulation of DNA-protein complex (Fig. 3C). As a control, the mutated cold probe could bind the ANAC089 protein to this probe. These data suggest that ANAC089 also specifically recognizes the G-box within the ABI5 promoter.
3.4 ANAC089 Negatively Regulates ABI5 Expression to Modulate H2O2 Accumulation and Seed Viability
Because ANAC089 can bind to the promoter of ABI5, we next wanted to determine the relationship between ANAC089 and ABI5. Here, we performed transient protoplast transformation to test the effect of ANAC089 on the expression of ABI5. We constructed a reporter vector containing the luciferase gene driven by the ABI5 promoter (ABI5p: Luc) and an effector containing the coding region sequence of ANAC089 (35S: ANAC089, abbreviated as ANAC089) and then co-transformed the effector and reporter into the isolated protoplast. The empty effector was used as the control. Compared with the protoplasts co-expressing the empty vector, the protoplasts co-expressing the ANAC089 effector suppressed the expression of LUC in the ABI5p: Luc reporter. We also constructed a mutated ABI5p: Luc reporter (mABI5p: Luc) by deleting the G-box within the promoter of ABI5 to abolish the binding of ANAC089 to this promoter. As expected, ANAC089 did not efficiently suppress the expression of LUC in the mABI5p: Luc reporter (Fig. 4A). Based on these data, we propose that ANAC089 suppresses the expression of ABI5 by recognizing and binding the G-box within the promoter of ABI5.
Figure 4: ANAC089 suppresses the expression of ABI5 to increase seed viability. (A) Transient transformation analysis of the suppressive effect of ANAC089 on ABI5 expression. The schematic diagram indicates the effector and reporter constructs for the transient transformation assay. The G-boxes are indicated with blue rectangles in the reporter construct, and the reporter with the truncated ABI5 promoter lacking the G-box is also shown. Full-length or truncated fragment of the ABI5pro: LUC reporter was co-expressed with ANAC089-Flag effectors overnight, and the firefly luciferase and Renilla luciferase (LUC/REN) ratio was calculated to present the ABI5pro: LUC activity. An empty effector without a promoter fragment was used as the control. The data are presented as the means ± SDs of three biological replicates. Bars with different letters are significantly different at p < 0.05 (Tukey’s test). (B and C) ANAC089 and ABI5 antagonistically control H2O2 generation and seed viability during aging treatment. The seeds of Col, anac089, abi5, ANAC089-Flag, and ABI5-MYC and their crossed seeds were treated with CDT for 4 days, and the H2O2 content in the imbibed seeds was measured (B). The seeds were germinated under normal conditions at 22°C for 5 days, and the percentage of germinated seeds was determined. The experiment was performed in triplicate, and the bars indicate ±SD. Bars with different letters mean significantly different at p < 0.05 (two-way ANOVA with Tukey’s test)
Given that ANAC089 affected the accumulation of H2O2 during the aging process, we wanted to determine the effect of ABI5 on the accumulation of H2O2. Compared with that in the Col line, CDT decreased the H2O2 content in the abi5 mutant but increased H2O2 accumulation in the ABI5-MYC line, suggesting that ABI5 negatively regulates the accumulation of H2O2 during the aging process (Fig. 4B). Accordingly, the seed germination of the abi5 mutant was greater than that of the ABI5-MYC transgenic line and the Col line after aging treatment (Fig. 4C). Furthermore, we crossed the abi5 mutant with the anac089 mutant or transgenic ANAC089-overexpressing line and found that both anac089/abi5 and ANAC089-Flag/abi5 showed lower H2O2 accumulation and a greater percentage of seed germination, similar to the abi5 mutant (Figs. 4B and 4C). These results are consistent with our findings that ANAC089 targets and binds to the promoter of ABI5 to negatively regulate ABI5 expression and indicate that the ability of ANAC089 to control H2O2 accumulation and seed germination after aging treatment is dependent on ABI5.
3.5 ABI5 Targets VTC2 to Control H2O2 Metabolism and Seed Viability
As our above results showed that the vtc2 mutant showed lower seed viability after CDT treatment because of increased accumulation of H2O2, we speculate that VTC2 is involved in the seed aging process and that VTC2 modulates redox status to sustain seed viability during the seed conservation process. To test this hypothesis, we generated a transgenic line overexpressing VTC2 (overexpressing the VTC2-Flag fusion gene under the control of the 35S promoter, 35S: VTC2-Flag, abbreviated VTC2-Flag) and compared the viability of the seeds after CDT treatment. As shown in Fig. 5A, compared with that in the Col line, CDT induced lower H2O2 accumulation in the VTC2-Flag line but more H2O2 accumulation in the vtc2 mutant line (Fig. 5A). Consistently, the percentage of germinated VTC2-Flag seeds was also greater than that of the vtc2 mutant line after the aging process (Fig. 5B), which suggested that VTC2 attenuates CDT-induced H2O2 accumulation to enhance seed germination.
Figure 5: ABI5 affects seed viability through VTC2. (A) The differences in H2O2 levels among Col, ABI5-MYC, VTC2-Flag, and the double transgenic ABI5-MYC/VTC2-Flag line after 4 days of CDT treatment. The experiment was performed in triplicate, and the bars indicate ±SD. The different letters on the top of the bars mean significantly different at p < 0.05 (two-way ANOVA following Tukey’s test). (B) ABI5 controls seed germination after aging via VTC2. The seeds of Col, abi5, ABI5-MYC, vtc2, VTC2-Flag, and their crossed lines were treated with CDT for 4 days, followed by germination under normal conditions at 22°C for 5 days, after which the percentage of germinated seeds was recorded. Seeds not subjected to CDT treatment were used as controls. The experiment was performed in triplicate, and the bars indicate ±SD. The different letters on the top of the bars mean significantly different at p < 0.05 (two-way ANOVA following Tukey’s test). (C) CDT treatment increased the protein accumulation of ABI5. WT Col seeds were treated with CDT for the indicated times, and the protein abundance of ABI5 was measured by western blotting analysis using an anti-ABI5 antibody. Anti-Actin was used as a loading control. (D) ABI5 suppresses the expression of VTC2. After the imbibed Col, abi5, and ABI5-MYC were treated with CDT for 4 days, the transcriptional level of VTC2 was measured by RT−qPCR analysis. Seeds not subjected to CDT treatment were used as controls. PP2A was used as the internal control. The experiment was performed in triplicate, and the bars indicate ±SD. The different letters on the top of the bars mean significantly different at p < 0.05 (two-way ANOVA following Tukey’s test)
To understand the relationship between ABI5 and VTC2, we first compared the different transcriptional levels of VTC2 between the abi5 mutant and ABI5-MYC lines before or after aging treatment by RT–qPCR analysis. As shown in Fig. 5D, overexpressing ABI5 suppressed the transcription of VTC2, whereas the loss-of-function abi5 mutant contained more VTC2 transcripts after aging, suggesting that ABI5 negatively regulates the expression of VTC2 after aging. Moreover, we found that aging increased the protein abundance of ABI5 in the ABI5-MYC line (Fig. 5C). A high level of ABI5 may suppress the expression of VTC2, thus increasing H2O2 accumulation to decrease seed viability. We then crossed the ABI5-MYC or abi5 mutant with the vtc2 mutant to obtain ABI5-MYC/vtc2 and abi5/vtc2. Genetic analysis revealed that both ABI5-MYC/vtc2 and abi5/vtc2 contained higher levels of H2O2 after aging treatment, similar to the vtc2 mutant (Fig. 5A). Similarly, the seed germination of ABI5-MYC/vtc2 and abi5/vtc2 was also lower than that of Col, similar to that of vtc2 (Fig. 5B). These data suggest that ABI5 controls H2O2 accumulation and seed viability through VTC2 during aging treatment.
4.1 ANAC089 Acts as a New Modulator to Control Seed Viability through H2O2 Signaling
A previous study showed that ANAC089 controls the cytosolic redox status to regulate ABA signaling and control seed germination [25,26]. Additionally, ANAC089 accelerated the expression of FLC to delay flowering [28]. Here, we reported that ANAC089 controls seed viability. Using the artificial CDT method to simulate the process of seed deterioration, we observed a rapid increase in the ANAC089 transcript during the early stage of CDT, after which the ANAC089 transcript decreased quickly during the subsequent long-term treatment with CDT (Fig. 2A), suggesting that ANAC089 may function during the seed aging process. Furthermore, overexpressing ANAC089 enhanced seed viability, whereas silencing ANAC089 reduced seed viability, as determined by tetrazolium staining analysis (Figs. 1D and 1E). A previous study also confirmed that a higher level of ROS, mainly H2O2, promoted seed deterioration and decreased seed germination after long-term conservation [14,21,22]. Our previous study also showed that high accumulation of H2O2 impaired seed viability during the desiccation process for recalcitrant seeds [35,36]. Here, we also revealed that ANAC089 affects the biosynthesis of H2O2 after CDT treatment. In agreement with the seed germination phenotype, the H2O2 content decreased in the transgenic ANAC089-Flag line but increased in the anac089 mutant line (Figs. 1B and 1C), which suggested that increased H2O2 may be the main factor affecting the viability of anac089 seeds during CDT. As vitamin C, or ascorbic acid (AsA), is an efficient antioxidant for removing H2O2, VTC2 is the critical enzyme for AsA biosynthesis, and the vtc2 mutant cannot scavenge H2O2 after stress treatment. Here, we also found a high H2O2 content and decreased seed germination of vtc2 after CDT treatment. Moreover, direct treatment of wild-type Col seeds with H2O2 also suppressed seed germination, while the addition of AsA to the vtc2 mutant partially rescued the decreased seed germination of vtc2 after CDT treatment (Fig. S2). These data support the hypothesis that a high concentration of H2O2 is the main factor affecting seed viability after CDT. Further genetic analysis revealed that the ANAC089-Flag/vtc2 crossed line presented greater H2O2 content and lower seed germination than did the ANAC089 line, which presented relatively lower H2O2 content and greater seed germination (Figs. 2B and 2C). Moreover, compared with low H2O2, additional AsA partially increased the germination of ANAC089-Flag/vtc2 seeds after CDT treatment (Fig. S3). Based on these results, we propose that ANAC089 enhances the deterioration of seed viability after long-term storage by attenuating the toxic effects of H2O2.
4.2 The ABI5-VTC2 Module Mediates ANAC089-Mediated Regulation of ROS Signaling during the Deterioration of Seed Viability
A previous study demonstrated that ANAC060 can bind the G-box within the promoter of ABI5 to suppress the transcription of ABI5 [34]. Here, we further elucidated the relationship between ANAC089 and ABI5 and found that ANAC089, which is homologous to ANAC060, can also negatively regulate the expression of ABI5 during CDT, as the transcriptional level of ABI5 decreased in the ANAC089-Flag line but increased in the abi5 mutant line after CDT treatment (Fig. 3A). Furthermore, ChIP and EMSA confirmed that ANAC089 can recognize the G-box within the promoter of ABI5 to suppress the expression of ABI5 (Figs. 3B and 3C). Protoplast transient transformation experiments showed that ANAC089 obviously suppressed the expression of ABI5, but deletion of the G-box within the promoter of ABI5 dramatically reduced this inhibitory effect (Fig. 4A), suggesting that ANAC089 specifically binds the G-box within the promoter of ABI5 to suppress the expression of ABI5. Genetic experiments showed that both ANAC089-Flag/vtc2 and anac089/vtc2 showed lower seed viability and lower seed germination after CDT treatment, similar to vtc2 (Fig. 4C), suggesting that ANAC089 required VTC2 to control the deterioration of seed viability during long-term storage.
ABI5 acts as an important regulator to control ABA signaling [37]. Our previous results also showed that ABI5 interacts with its partner protein AFP2 to control seed germination under high-temperature treatment by binding to the SOMNUS gene [38]. In this study, we found that simulating the seed aging process using the CDT method induced the accumulation of the ABI5 protein in the stored seeds (Fig. 5C). Accordingly, the abi5 mutant showed high seed germination, but overexpressing ABI5 reduced seed germination after CDT (Fig. 4C), suggesting that ABI5 negatively regulates seed viability during the seed aging process. In agreement with our above discussion, the level of H2O2 was also greater in the ABI5-overexpressing line but lower in the abi5 mutant line (Fig. 4B), suggesting that ABI5 controls seed viability through ROS metabolism. Previous studies reported that ABI4 can bind to the promoter of VTC2 to control its expression during saline stress. As both ABI4 and ABI5 are important transmitters of ABA signaling [39], we also found that ABI5 affects the expression of VTC2 during CDT treatment, and the transcriptional level of VTC2 was greater in the abi5 mutant but lower in the ABI5-overexpressing line after CDT (Fig. 5D), suggesting that ABI5 negatively regulates the transcriptional level of VTC2, similar to ABI4, after environmental stress. This result also coincides with our above results showing the accumulation pattern of H2O2 and seed viability in the abi5 or ABI5-MYC lines after CDT and suggests that ABI5 affects the expression of VTC2 to control H2O2 levels and seed viability during the seed aging process. Moreover, unlike in the anac089 mutant line, which had a relatively high H2O2 content after CDT, the H2O2 level in both the ANAC089-Flag and abi5 lines was relatively lower than that in the transgenic ABI5-Myc line after CDT. These data further confirm our above conclusion and suggest that ANAC089 enhances seed viability during seed aging by suppressing ABI5, thereby upregulating VTC2 levels to scavenge ROS.
In summary, we reported the novel function of ANAC089 in controlling seed viability. A series of physiological, biochemical, and genetic studies showed that ANAC089 can specifically bind the G-box region within the promoter of ABI5 to negatively regulate the expression of ABI5, while ABI5 further negatively regulates the expression of VTC2, thereby scavenging H2O2 during the seed aging process. As a result, ANAC089 suppresses H2O2 signaling to enhance seed viability or longevity through the ABI5-VTC2 module. Thus, our findings reveal the mechanism by which the ANAC089-ABI5-VTC2 axis controls seed viability through ROS metabolism, providing a possible strategy for the genetic engineering of ANAC089 to increase seed viability and prolong seed conservation in the field of seed biology.
Acknowledgement: We thank Professor Kai Shu from Northwestern Polytechnical University (Xi’an, China) and Professor Chuanyou Li from the Institute of Genetics (Chinese Academy of Sciences, Beijing, China) for sharing the experimental materials, and thank Arabidopsis Resource Centre (ABRC) for providing the relative mutant seeds.
Funding Statement: This work was supported by the National Natural Science Foundation of China (31970289 to X.H. and 32170562 to P.L.).
Author Contributions: Study conception and design: Xiangyang Hu and Ping Li. Data collection: Yuan Tian. Analysis and interpretation of results: Yuan Tian and Lulu Zhi. Draft manuscript preparation: Xiangyang Hu. All authors reviewed the results and approved the final version of the manuscript.
Availability of Data and Materials: All data used for this study are available within the text and its supplementary files, more information can be requested by contacting the corresponding authors.
Ethics Approval: Not applicable.
Conflicts of Interest: The authors declare that they have no conflicts of interest to report regarding the present study.
Supplementary Materials: The supplementary material is available online at https://doi.org/10.32604/phyton.2024.050220.
References
1. Finch-Savage WE, Bassel GW. Seed vigour and crop establishment: extending performance beyond adaptation. J Exp Bot. 2016;67(3):567–91. [Google Scholar] [PubMed]
2. Leprince O, Pellizzaro A, Berriri S, Buitink J. Late seed maturation: drying without dying. J Exp Bot. 2017;68(4):827–41. [Google Scholar] [PubMed]
3. Vidigal DD, Willems L, van Arkel J, Dekkers BJW, Hilhorst HWM, Bentsink L. Galactinol as marker for seed longevity. Plant Sci. 2016;246:112–8. [Google Scholar]
4. Rehmani MS, Aziz U, Xian BS, Shu K. Seed dormancy and longevity: a mutual dependence or a trade-off? Plant Cell Physiol. 2022;63(8):1029–37. [Google Scholar] [PubMed]
5. Li P, Ni HH, Ying SB, Wei JL, Hu XY. Teaching an old dog a new trick: multifaceted strategies to control primary seed germination by DELAY OF GERMINATION 1 (DOG1). Phyton-Int J Exp Bot. 2020;89(1):1–12. doi:10.32604/phyton.2020.09817 [Google Scholar] [CrossRef]
6. Moothoo-Padayachie A, Varghese B, Pammenter NW, Govender P, Sershen. Germination associated ROS production and glutathione redox capacity in two recalcitrant-seeded species differing in seed longevity. Botany. 2016;94(12):1103–14. [Google Scholar]
7. Salvi P, Saxena SC, Petla BP, Kamble NU, Kaur H, Verma P, et al. Differentially expressed galactinol synthase(s) in chickpea are implicated in seed vigor and longevity by limiting the age induced ROS accumulation. Sci Rep. 2016;6:35088. doi:10.1038/srep35088. [Google Scholar] [PubMed] [CrossRef]
8. Sano N, Rajjou L, North HM, Debeaujon I, Marion-Poll A, Seo M, et al. Staying alive: molecular aspects of seed longevity. Plant Cell Physiol. 2016;57(4):660–74. [Google Scholar] [PubMed]
9. Sugliani M, Rajjou L, Clerkx EJ, Koornneef M, Soppe WJ. Natural modifiers of seed longevity in the Arabidopsis mutants abscisic acid insensitive3-5 (abi3-5) and leafy cotyledon1-3 (lec1-3). New Phytol. 2009;184(4):898–908. [Google Scholar] [PubMed]
10. Pellizzaro A, Neveu M, Lalanne D, Ly Vu B, Kanno Y, Seo M, et al. A role for auxin signaling in the acquisition of longevity during seed maturation. New Phytol. 2020;225(1):284–96. [Google Scholar] [PubMed]
11. Bueso E, Ibanez C, Sayas E, Munoz-Bertomeu J, Gonzalez-Guzman M, Rodriguez PL, et al. A forward genetic approach in Arabidopsis thaliana identifies a RING-type ubiquitin ligase as a novel determinant of seed longevity. Plant Sci. 2014;215–216:110–6. [Google Scholar] [PubMed]
12. Zinsmeister J, Lalanne D, Terrasson E, Chatelain E, Vandecasteele C, Vu BL, et al. ABI5 is a regulator of seed maturation and longevity in legumes. Plant Cell. 2016;28(11):2735–54. doi:10.1105/tpc.16.00470. [Google Scholar] [PubMed] [CrossRef]
13. Dekkers BJ, He H, Hanson J, Willem LA, Jama DC, Cueff G, et al. The Arabidopsis DELAY OF GERMINATION 1 gene affects ABSCISIC ACID INSENSITIVE 5 (ABI5) expression and genetically interacts with ABI3 during Arabidopsis seed development. Plant J. 2016;85(4):451–65. [Google Scholar] [PubMed]
14. Wang WQ, Xu DY, Sui YP, Ding XH, Song XJ. A multiomic study uncovers a bZIP23-PER1A-mediated detoxification pathway to enhance seed vigor in rice. Proc Natl Acad Sci U S A. 2022;119(9):e2026355119. doi:10.1073/pnas.2026355119. [Google Scholar] [PubMed] [CrossRef]
15. Shvachko NA, Khlestkina EK. Molecular genetic bases of seed resistance to oxidative stress during storage. Vavilovskii Zh Genet. 2020;24(5):451–8. doi:10.18699/VJ20.637. [Google Scholar] [CrossRef]
16. Ying SB, Jing SS, Cheng LH, Sun HQ, Tian Y, Zhi LL, et al. Allantoin alleviates seed germination thermoinhibition in Arabidopsis. Phyton-Int J Exp Bot. 2022;91(9):1893–904. doi:10.32604/phyton.2022.022679 [Google Scholar] [CrossRef]
17. Lu S, Hu YL, Chen YL, Yan YR, Jin Y, Li P, et al. Putrescine enhances seed germination tolerance to heat stress in Arabidopsis thaliana. Phyton-Int J Exp Bot. 2022;91(9):1879–91. doi:10.32604/phyton.2022.022605 [Google Scholar] [CrossRef]
18. Sattler SE, Gilliland LU, Magallanes-Lundback M, Pollard M, DellaPenna D. Vitamin E is essential for seed longevity, and for preventing lipid peroxidation during germination. Plant Cell. 2004;16(6):1419–32. doi:10.1105/tpc.021360. [Google Scholar] [PubMed] [CrossRef]
19. Luo X, Dai Y, Zheng C, Yang Y, Chen W, Wang Q, et al. The ABI4-RbohD/VTC2 regulatory module promotes reactive oxygen species (ROS) accumulation to decrease seed germination under salinity stress. New Phytol. 2021;229(2):950–62. doi:10.1111/nph.16921. [Google Scholar] [PubMed] [CrossRef]
20. Kakan X, Yu YW, Li SH, Li XY, Huang RF, Wang J. Ascorbic acid modulation by ABI4 transcriptional repression of VTC2 in the salt tolerance of Arabidopsis. BMC Plant Biol. 2021;21(1):112. doi:10.1186/s12870-021-02882-1. [Google Scholar] [PubMed] [CrossRef]
21. Chen HH, Chu P, Zhou YL, Ding Y, Li Y, Liu J, et al. Ectopic expression of NnPER1, a Nelumbo nucifera 1-cysteine peroxiredoxin antioxidant, enhances seed longevity and stress tolerance in Arabidopsis. Plant J. 2016;88(4):608–19. doi:10.1111/tpj.13286. [Google Scholar] [PubMed] [CrossRef]
22. Chen HH, Ruan JX, Chu P, Fu W, Lian ZW, Li Y, et al. AtPER1 enhances primary seed dormancy and reduces seed germination by suppressing the ABA catabolism and GA biosynthesis in seeds. Plant J. 2020;101(2):310–23. doi:10.1111/tpj.14542. [Google Scholar] [PubMed] [CrossRef]
23. Kim SG, Lee S, Seo PJ, Kim SK, Kim JK, Park CM, et al. Genome-scale screening and molecular characterization of membrane-bound transcription factors in Arabidopsis and rice. Genomics. 2010;95(1):56–65. doi:10.1016/j.ygeno.2009.09.003. [Google Scholar] [PubMed] [CrossRef]
24. Wang DX, Yu YC, Liu ZH, Li S, Wang ZL, Xiang FN. Membrane-bound NAC transcription factors in maize and their contribution to the oxidative stress response. Plant Sci. 2016;250:30–9. doi:10.1016/j.plantsci.2016.05.019. [Google Scholar] [PubMed] [CrossRef]
25. Klein P, Seidel T, Stöcker B, Dietz KJ. The membrane-tethered transcription factor ANAC089 serves as redox-dependent suppressor of stromal ascorbate peroxidase gene expression. Front Plant Sci. 2012;3(27):247. doi:10.3389/fpls.2012.00247. [Google Scholar] [PubMed] [CrossRef]
26. Albertos P, Tatemats K, Mateos I, Sánchez-Vicente I, Fernández-Arbaizar A, Nakabayashi K, et al. Redox feedback regulation of ANAC089 signaling alters seed germination and stress response. Cell Rep. 2021;35(11):109263. doi:10.1016/j.celrep.2021.109263. [Google Scholar] [PubMed] [CrossRef]
27. Song S, Willem LAJ, Jiao A, Zhao T, Schranz ME, Bentsink L. The membrane associated NAC transcription factors ANAC060 and ANAC040 are functionally redundant in the inhibition of seed dormancy in Arabidopsis thaliana. J Exp Bot. 2022;73(16):5514–28. [Google Scholar] [PubMed]
28. Li JQ, Zhang JA, Wang XC, Chen J. A membrane-tethered transcription factor ANAC089 negatively regulates floral initiation in Arabidopsis thaliana. Sci China Life Sci. 2010;53(11):1299–306. [Google Scholar] [PubMed]
29. Chen R, Jiang H, Li L, Zhai Q, Qi L, Zhou W, et al. The Arabidopsis mediator subunit MED25 differentially regulates jasmonate and abscisic acid signaling through interacting with the MYC2 and ABI5 transcription factors. Plant Cell. 2012;24(7):2898–916. [Google Scholar] [PubMed]
30. Xie X, Ma X, Zhu Q, Zeng D, Li G, Liu YG. CRISPR-GE: a convenient software toolkit for CRISPR-based genome editing. Mol Plant. 2017;10(9):1246–9. [Google Scholar] [PubMed]
31. He W, Wang R, Zhang Q, Fan M, Lyu Y, Chen S, et al. E3 ligase ATL5 positively regulates seed longevity by mediating the degradation of ABT1 in Arabidopsis. New Phytol. 2023;239(5):1754–70. [Google Scholar] [PubMed]
32. Li P, Zhang QL, He DN, Zhou Y, Ni HH, Tian DG, et al. AGAMOUS-LIKE67 cooperates with the histone mark reader EBS to modulate seed germination under high temperature. Plant Physiol. 2020;184(1):529–45. doi:10.1104/pp.20.00056. [Google Scholar] [PubMed] [CrossRef]
33. Deng G, Sun H, Hu Y, Yang Y, Li P, Chen Y, et al. A transcription factor WRKY36 interacts with AFP2 to break primary seed dormancy by progressively silencing DOG1 in Arabidopsis. New Phytol. 2023;238(2):688–704. doi:10.1111/nph.18750. [Google Scholar] [PubMed] [CrossRef]
34. Yu B, Wang Y, Zhou H, Li P, Li C, Chen S, et al. Genome-wide binding analysis reveals that ANAC060 directly represses sugar-induced transcription of ABI5 in Arabidopsis. Plant J. 2020;103(3):965–79. doi:10.1111/tpj.14777. [Google Scholar] [PubMed] [CrossRef]
35. Bai XG, Chen JH, Kong XX, Todd CD, Yan YP, Hu XY, et al. Carbon monoxide enhances the chilling tolerance of recalcitrant Baccaurea ramiflora seeds via nitric oxide-mediated glutathione homeostasis. Free Radic Biol Med. 2012;53(4):710–20. doi:10.1016/j.freeradbiomed.2012.05.042. [Google Scholar] [PubMed] [CrossRef]
36. Bai X, Yang L, Tian M, Chen J, Shi J, Yang Y, et al. Nitric oxide enhances desiccation tolerance of recalcitrant Antiaris toxicaria seeds via protein S-nitrosylation and carbonylation. PLoS One. 2011;6(6):e20714. doi:10.1371/journal.pone.0020714. [Google Scholar] [PubMed] [CrossRef]
37. Li Z, Luo X, Wang L, Shu K. ABSCISIC ACID INSENSITIVE 5 mediates light-ABA/gibberellin crosstalk networks during seed germination. J Exp Bot. 2022;73(14):4674–82. doi:10.1093/jxb/erac200. [Google Scholar] [PubMed] [CrossRef]
38. Chang G, Wang C, Kong X, Chen Q, Yang Y, Hu X. AFP2 as the novel regulator breaks high-temperature-induced seeds secondary dormancy through ABI5 and SOM in Arabidopsis thaliana. Biochem Biophys Res Commun. 2018;501(1):232–8. doi:10.1016/j.bbrc.2018.04.222. [Google Scholar] [PubMed] [CrossRef]
39. Chandrasekaran U, Luo X, Zhou W, Shu K. Multifaceted signaling networks mediated by abscisic acid insensitive 4. Plant Commun. 2020;1(3):100040. doi:10.1016/j.xplc.2020.100040. [Google Scholar] [PubMed] [CrossRef]
Cite This Article
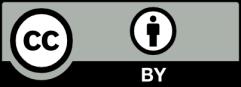