Open Access
ARTICLE
Effect of Light Emitting Diodes (LEDs) on Growth, Mineral Composition, and Nutritional Value of Wheat & Lentil Sprouts
1 Department of Botany, Kohat University of Science & Technology, Kohat, 26000, Pakistan
2 Department of Food Engineering, Faculty of Agriculture, Hatay Mustafa Kemal University, Hatay, 31000, Türkiye
3 Department of Botany, University of Science & Technology, Bannu, 28100, Pakistan
4 Department of Biotechnology and Genetic Engineering, Kohat University of Science & Technology, Kohat, 26000, Pakistan
5 Department of Biology, University of Haripur, Khyber Pakhtunkhwa, 22620, Pakistan
6 School of Science, Western Sydney University, Penrith, New South Wales, 2151, Australia
7 Department of Crop and Animal Production, Sason Vocational School, Batman University, Batman, 72060, Türkiye
8 Department of Plant Sciences, Quaid-i-Azam University, Islamabad, 45320, Pakistan
9 Department of Botany, Islamia College Peshawar, Peshawar, 25120, Pakistan
10 Department of Horticulture, Agricultural Faculty, Ataturk University, Erzurum, 25240, Türkiye
11 HGF Agro, Ata Teknokent, Erzurum, TR-25240, Türkiye
12 Department of Zoology, College of Science, King Saud University, Riyadh, 11451, Saudi Arabia
* Corresponding Authors: Amana Khatoon. Email: ; Muhammad Nauman Khan. Email:
(This article belongs to the Special Issue: Integrated Nutrient Management in Cereal Crops)
Phyton-International Journal of Experimental Botany 2024, 93(6), 1117-1128. https://doi.org/10.32604/phyton.2024.048994
Received 23 December 2023; Accepted 16 April 2024; Issue published 27 June 2024
Abstract
Sprouts are ready-to-eat and are recognized worldwide as functional components of the human diet. Recent advances in innovative agricultural techniques could enable an increase in the production of healthy food. The use of light-emitting diode (LED) in indoor agricultural production could alter the biological feedback loop, increasing the functional benefits of plant foods such as wheat and lentil sprouts and promoting the bioavailability of nutrients. The effects of white (W), red (R), and blue (B) light were investigated on the growth parameters and nutritional value of wheat and lentil sprouts. In the laboratory, seeds were sown under three different LED treatments: white, red, and blue light, while normal incandescent light served as a control. Percentage seed germination improved by 18.34% and 12.67% for wheat and 18.34% and 12.67% for lentil sprouts under LED treatments R and B, respectively. An increase in total soluble protein and sugar by 33.4% and 9.23% in wheat and by 31.5% and 5.87% in lentils was observed under the R LED treatment. Vitamin C concentrations in wheat and lentils were significantly increased by R LED compared to all other treatments. Other parameters, including potassium and sodium concentrations, were significantly increased under red and blue light compared to the control; white light, on the other hand, significantly decreased all these parameters. According to the experimental data, red and blue LED light could be beneficial in the production of functional wheat and lentil sprouts with high nutrient concentrations.Keywords
Indoor agricultural techniques are generally used to shorten the period of crop cultivation and accelerate crop improvement by artificially controlling agricultural conditions so that crops can grow repeatedly regardless of seasonal climate patterns [1]. The difficulties in placing fluorescent and metal halide lights near crops due to excessive temperatures caused by radiant heat prevent the crop canopy from receiving adequate light intensity. In addition, the generation of heat wastes electrical energy. Light-emitting diode (LED) applications improve the productivity of indoor growing plants [2]. Solid-state lighting with LEDs can improve light intensity and spectral distribution while providing high light output with minimal radiant heat [3]. Although LEDs are very promising as a radiation source for plant growth, it is important to further investigate how plants respond morphologically, biochemically, and genetically to LED light. Throughout the life cycle of a plant, light signals- especially those related to light intensity and duration- influence, almost all elements of growth and developmental activities [4]. By providing the energy required for plant growth and development, and controlling seedling photomorphogenesis, stomatal conductance, seedling improvement, chloroplast activity, signal transduction, and inductive reasoning through the photoreceptor transmitter, spectral distribution patterns, especially in the red (R) and blue (B) wavelength ranges, are involved in plant photosynthetic activity [5]. In crop plants, the impacts of light quality on plant growth and production have already been studied in detail [6]. For instance, it was found that B leads to larger leaves than R and that both together produce sweet basil with a medium total leaf area [7]. In cucumber seedlings exposed to a mixture of R and B radiation, leaf area decreased with increasing B photon flux, while seedlings raised with B radiation alone developed larger plants than those irradiated with R radiation alone or with both types of radiation together [8]. The penetration of light into the shoots of plants alters the characteristics of shoot growth and the development of the subsurface root system [9]. Red decreased the development of lateral roots but increased the quantity of 1° lateral roots and the density of 2° lateral roots in tobacco plants [10]. According to certain theories, the photoreceptor cells regulate how much carbon is made available for the formation of biomass in a developing plant [11]. The shoot biomass, root biomass, and root-to-shoot ratio of Mesembryanthemum crystallinum cultivated under R-light conditions were the lowest, while the plants growing under R-to-B conditions showed better photosynthetic performance [12]. Chlorophyll production failed under R irradiation alone while B and R irradiation are required for the synthesis of pigments [13]. Red light increased the total amount of chlorophyll in wheat [14]. Numerous plant functions, including stomata opening, photomorphogenesis, phototropism, and photosynthetic activity of leaves, are influenced by blue light. Blue light is associated with the expression of “sun-like” characteristics in the chloroplasts, such as high photosynthetic activity [15].
Recently, sprouts have gained popularity as a way to design the “Mediterranean diet,” especially in plant-based and vegan diets. Observant customers benefit from including fresh plant foods such as sprouts in their diet as they contain high amounts of easily digestible nutrients [16]. Sprouts are produced by the germination of seeds, which is associated with physiological and biochemical disorders as polysaccharides and proteins are converted into sugars and amino acids. Germination of certain legumes leads to an increase or decrease in vitamins and nutritional variables [17], such as an increase in natural antioxidants [18], and angiotensin-transmitting enzyme [16]. The sprouting process improves the nutritional content of sprouts by increasing nutrient content, decreasing phytic acid, and increasing protein and vitamin C content [19]. Furthermore, the essential utilizable properties of the nutrient content of sprouts have attracted the interest of researchers as potential future health-promoting functional foods [20]. Numerous antihypertensive, antihyperlipidemic, and antidiabetic substances have also been discovered in sprout seeds [21].
More than half of the oilseeds produced come from the commercially significant crops wheat and lentil, which are the subject of much research to understand their physiological molecular processes and stress responses [19]. For many studies, it is essential to cultivate the plants in controlled environments, mainly in phytotrons, which emit different intensity levels or spectral distributions of radiation that are inadequate for legumes. The impacts of LED light on seedlings have only been investigated in a few studies. R-LED light dramatically reduced the growth of chickpea and wheat sprouts in both daylight and dark periods [22]. However, current knowledge of how lentils and wheat seedlings respond to light quality is rather inconsistent. In this work, three different LED light qualities were used to investigate the growth responses of wheat and lentil sprouts. This study aims to learn more about how wheat and lentil sprouts respond to spectral distribution. By specifically addressing the timing of germination under different light qualities, this study aims to provide a more nuanced understanding of how light influences the growth stages and subsequent nutrient composition of sprouts.
The analyses were carried out on wheat (Var KD/2017) and lentil (Punjab Mansoor-2019) seeds collected from Barani Research Centre, Kohat, KP. The seeds were stored at 4°C for further use.
The seeds were planted in petri dishes and treated with distilled water in a growth room (25°C). The Petri dishes were kept under three different light spectra, namely white LED (HT100-5700), red LED (660 nm, HT080-Red), and blue LED (450 nm, HT080-Blue). These LEDS lights were manufactured by Zam LED Co. Limited, Chungcheongbuk-do, South Korea. Three Petri dishes with seeds grown under normal incandescent light were used as controls. Seedlings were then harvested after 12 days and stored for various parameters. To achieve a randomized arrangement of growth conditions, the experiment was conducted twice separately. The experiments were conducted in a growth chamber at a temperature of 22 ± 3°C (day and night), a humidity of 75%, and 900 mole CO2 for each treatment.
The germination of wheat and lentil seeds, the shoot/root length, the fresh weight, and the number of secondary roots were measured.
The total soluble sugar was calculated using the Fairbairn technique [23]. A test tube containing 0.05 g of fresh shoot was added mixed with 5 mL of distilled water and placed in a water bath at 85°C for 30 min. After 30 min, the supernatant was collected. This procedure was carried out three times, adding 10 mL of distilled water. Using the sulfuric acid enthronement method, the amount of total soluble sugar was measured at a wavelength of 620 nm.
2.5 Soluble Protein Concentration
The Bradford technique was used to calculate the amount of total soluble proteins in the sample [24]. In a mortar, the materials (0.05 g of the fresh weight of the shoots) were crushed with liquid nitrogen gas before mixing with 3 mL of a phosphate-buffered liquid (pH 7.0). The extraction was centrifuged at 13,000 rpm for 15 min at 4°C. The supernatant was collected and 0.1 mL of Coomassie Brilliant Blue G-250 solution was added. The total soluble proteins were calculated at a wavelength of 595 nm wavelength for two min.
2.6 Measurement of Vitamin-C Level
The modified Gahler approach was used to determine the vitamin C concentration [25]. For this purpose, 15 mL of 4.5 diluted phosphoric acid was added to 0.2 g of the fresh lentil and wheat sample and placed in a shaker. The samples were then centrifuged at 16,000 rpm for 10 minutes. The vitamin C concentration in the supernatant was measured using high-performance liquid chromatography (Agilent, Model-1100, USA) with column chromatography at 254 nm against the ascorbate standard.
2.7 Measurement of Mineral Level
The macro and micronutrients were calculated as ground sprouts of wheat lentil sprouts. For the measurement of sodium (Na) and potassium (K), an acid method with HNO3:HClO4 (2:1, v:v) was performed and the measurement was carried out with a flame photometer (ICP-OES 725-ES; Agilent; Santa Clara, CA, USA) [26].
The data were analyzed using analysis of variance (ANOVA) and least significant difference (LSD) test at a level of p ≤ 0.05. SAS software, version 9.1 was used for all data analyses (SAS Institute; Cary, NC, USA).
3.1 Growth, Morphology, and Pigmentation of Plants
The results in Fig. 1 show that LED light treatments had different effects on germination, germination rate, and seed viability. Compared to the control, the germination percentage improved by 18.34% and 12.67% for wheat and 18.34% and 12.67% for lentil sprouts under the R and B LED light treatments, respectively. The R-LED light significantly increased the germination potential compared to the white (W) and blue light treatments and decreased the germination rate of wheat and lentil sprouts by 13.3% and 6.67%, respectively, compared to the W-LED treatment. This indicates that blue light improves the germination capacity of bitter gourd, while red light increases the germination capacity of seeds. Fig. 2 shows the results of the mass evaluation of wheat and lentil sprouts affected by the LED light spectra. The plants responded to the different light treatments with different growth patterns. The shoot/root length and fresh weight of the plants were highest when grown under red and blue light and lowest under white light. Compared to the control, shoot length and fresh weight increased by 19.3% and 9.59% for wheat and 18.76% and 8.68% for lentils when treated with R light from the LED (Fig. 3). Plants treated with B light of LED had significantly greater root length and fresh weight (FW) than those treated with the control, i.e., 20.88% and 5.27%, for wheat and 17.66% and 5.76% for lentil shoots, respectively. The R- and B-light treatments had higher shoot length and weight than the control; however, the W-light LED treatment was detrimental to these shoots (Fig. 4).
Figure 1: Effects of white, blue, and red light from LEDs on germination percentage of wheat (a) and lentils (b) sprouts, respectively. Seeds were sown under white, blue, and red LED treatments. Normal light served as a control. Values are means of three replicates, with standard errors represented by vertical bars. Using the least significant difference test (as a reference for the LSD test), different lowercase letters show significant differences at a level of p ≤ 0.05
Figure 2: Effects of white, blue, and red light from LEDs on shoot/root length (mass evolution) in cm of wheat (a, b) and lentil (c, d) sprouts, respectively. The seeds were sown under white, blue, and red LED treatments. The weight of the sprouts/roots of wheat and lentils was measured on the 10th day. Normal light served as a control. Values are means of three replicates of standard errors represented by vertical bars. Using the test for least significant differences (various range tests), different lowercase letters show significant differences at a level of p ≤ 0.05
Figure 3: Effects of white, blue, and red light from LEDs on shoot/root fresh weight in g of wheat (a, b) and lentil (c, d) sprouts. Seeds were sown under white, blue, and red LED treatments respectively. The shoot/root weight of wheat and lentil sprouts was determined on the 10th of sowing. Normal light served as a control. Values are means of three replicates standard errors are represented by vertical bars. Using the least significant difference test (p ≤ 0.05, n = 3), various lowercase letters show significant differences
Figure 4: Effects of white, blue, and red light from LEDs on total soluble protein and sugar concentrations (‰) of wheat (a, c) and lentil (b, d) sprouts. In panel b, TSP and not TSS are indicated. Seeds were sown under white, blue, and red LED treatments, respectively. The shoot/root weight of wheat and lentil sprouts was determined on the 10th day after sowing. Normal light served as a control. Values are means of three replicates of standard errors, represented by vertical bars. Using the least significant difference test (p ≤ 0.05, n = 3), various lowercase letters show significant differences.
3.2 The Nutritive Concentration of Lentil and Wheat Sprouts
Fig. 5 depicts the change in the total concentration of soluble sugars in the sprouts on the 10th day of sowing. The results show that the soluble sugar concentration showed an upward trend from W, B to R. Compared with the control treatment, the R treatment significantly increased the amount of soluble protein and sugar in the wheat and lentil sprouts by 33.4% and 9.23%, and 31.5% and 5.87%, respectively. The soluble sugar and protein concentration decreased in the W-LED treatment compared to B and R, but was still higher than in the control.
Figure 5: Effects of white, blue, and red light from LEDs on vitamin-C concentrations (‰) on wheat (a) and lentil (b) sprouts, respectively. Seeds were sown under white, blue, and red LED treatments. The shoot/root weights of wheat and lentil sprouts were determined on the 10th day of sowing. Normal light served as a control. Values are means of three replicates standard errors are represented by vertical bars. Using the least significant difference test (p ≤ 0.05, n = 3), various lowercase letters show significant differences
3.3 Vitamin-C, K, and Na Concentrations
The vitamin C concentration of wheat and lentil sprouts changed with the different R, B, or W light of the LEDs, as depicted in (Fig. 5). This could indicate that the vitamin C concentration responded to the light spectrum. The wheat and lentil sprouts treated with R had the highest vitamin C concentration, which was significantly higher than on the plants exposed to the other treatments. These findings showed that R-LEDs stimulate vitamin C synthesis in wheat and lentil sprouts. The levels of K and Na differed significantly between the various light treatments, indicating that light quality has a significant effect on K and Na concentrations (Fig. 6). Plants exposed to the R treatment had the highest K and Na concentrations in the wheat and lentil sprouts followed by the B light and LED treatments in the wheat and lentil shoots, respectively. The wheat and lentil sprouts treated with W had lower nitrate concentrations than the plants treated with R and B and the control plants.
Figure 6: Effects of white, blue, and red light from LEDs on mineral concentrations (‰) of wheat (a) and lentil (b) sprouts, respectively. Seeds were sown under white, blue, and red LED treatments. The shoot/root weights of wheat and lentil sprouts were determined on the 10th day of sowing. Normal light served as a control. Values are means of three replicates standard errors are represented by vertical bars. Using the least significant difference test (p ≤ 0.05, n = 3), various lowercase letters show significant differences
In general, plants can use light to control their morphological growth and to provide them with energy for their development, and all these activities work together. Light affects some developmental and growth processes, including seed germination, seed size, and flower formation [22]. The percentage of germination of bitter gourd was promoted by red light, which also maintained a high level of germination. This indicates that bitter gourd seed germination responds differently to different types of light.
Both wheat and lentils are important crops that are grown all over the world. Lentils are sensitive to different light conditions and are therefore, grown in greenhouses worldwide [22,27] The current study, we investigated how different LED lights affect the morphological growth and biochemical properties of wheat and lentil sprouts. Wheat and lentil sprouts cultivated under R, B, and W LED treatments showed significantly higher biomass of roots and leaves than the control plants. Furthermore, both wheat and lentil plants treated with R-LEDs had the highest shoot/root length and weight than those treated with B- and W-LEDS (Fig. 3). Overall, the increased promotion of wheat and lentil sprouts indicates that this LED illumination can affect growth, increase the mean weight of wheat and lentil sprouts and, keep the market price of the vegetables high. W-LED lighting caused wheat and lentil sprouts to grow longer with a lower yield [28]. B-LED lighting is crucial for leaf growth and promotes leaf area (LA) and biomass production [29]. Previous studies have shown that sprouts cultivated under R light with the addition of B LED light accumulate more mass than sprouts produced under R light only [30]. Furthermore, the leaves of wheat and lentil sprouts irradiated with B-light showed higher fresh weight of sprouts than plants irradiated with W-LED light [31]. In the current studies, it was concluded that the R-light of the LED treatment outperformed the B-light of the LED treatment as an irradiation source for the development and growth of wheat and lentil shoots. Similar outcomes were obtained for the in vitro growth of tomatoes [32]. Compared to the R and W light treatments, the shoot/root biomass and length of wheat and lentil shoots increased significantly after the R and B LED treatments compared to the W and control treatments (Fig. 2). The considerable increase in fresh weight may have been caused by the greater ability of the leaves to absorb light. Previous studies have shown that the larger photosynthetic area per unit effort in leaf tissue is significantly higher under R-light LED [33]. Plants require a continuous supply of water and nutrients to strengthen the shoot and root systems. Sprouting seedlings, on the other hand, have short roots that cannot absorb enough water or minerals, which slow down plant growth and cause the stems to expand rapidly when there is little sunlight or too much water. Crops with a high shoot-to-root ratio are not ideal for vigorous growth, as their poor roots cannot provide enough water for the huge branches [34].
The absorption bands of plant pigments are different at different wavelengths. The action spectra are the frequencies used in the biosynthesis and synthesis of plant pigments [35]. The best light absorption occurs in the range of 400–500 and 630–680 nm for chlorophyll “a” and “b” and carotenoids, respectively, while low absorption occurs in the 530–610 nm. The plants showed identical absorption spectra of the photosynthetic pigments, i.e., chlorophyll a, b, a + b, and carotenoids, despite the application of light of different quality for all treatments with the same photon flux density (PFD). It is possible that the applied PFD level (210 µmol m−2 s−1) reached a certain PFD minimum required for adequate production and activation of chlorophyll activity. Plants with lower chlorophyll concentrations appeared to utilize chlorophyll more effectively than plants with higher chlorophyll levels [36]. It is possible to use a specific type of light to increase the yield and nutritional quality of vegetable production. The metabolic activities of the investigated vegetable species were influenced differently by the selected LED lights. The main products of photosynthesis, the sugars, and their accumulation in the leaves reacted the most strongly [25]. Consequently, light variations affect sugar and protein concentrations and also measure the signaling molecules that contribute to the control of important morphological functions of plants [37,38]. In terms of food quality, total soluble sugar concentration can be a useful trait. The findings indicated that wheat and lentil sprouts had the highest concentrations of soluble sugars and proteins under R-light from LEDs, suggesting that this light source may contribute to wheat and lentil sprouts storing soluble sugars and proteins (Fig. 4). Furthermore, there were significant differences in the concentration of proteins and soluble sugars in the treated plant leaves. This suggests that the light spectra could be useful for sugar and protein biosynthesis and that the amount of soluble sugar and protein could be a reliable indicator of the nutritional value of wheat and lentil sprouts.
Previous studies have shown that supplemental B-light increases the amount of vitamin C that accumulates in sprouts [25]. In this study, red light treatment was shown to significantly increase vitamin C synthesis in wheat and lentil sprouts compared to the other light treatments. It was also found that the vitamin C concentration increased in plants irradiated with R and B light and LED light and in the control.
The use of favorable light conditions for seed germination has attracted much attention in recent decades. In this study, we investigated how different light spectra (white, blue, and red) affect the growth parameters and nutrient concentrations of two sprout species. The findings presented here showed that the growth, development, and nutrient concentration of sprouts can be influenced by changes in light color. Three general trends emerged from the findings for the two sprout species studied:
(i) Blue LED light had a positive effect on the growth parameters and nutrient concentration of wheat and lentil sprouts.
(ii) Red LED light proved to be more effective in increasing the growth characteristics and nutrient concentration of wheat and lentil sprouts.
(iii) White LED light harmed the growth parameters and nutrient concentration of wheat and lentil sprouts.
Acknowledgement: The authors would like to extend their sincere appreciation to the Researchers Supporting Project Number (RSP2024R410), King Saud University, Riyadh, Saudi Arabia.
Funding Statement: Supported by Researchers Supporting Project Number (RSP2024R410), King Saud University, Riyadh, Saudi Arabia.
Author Contributions: Conceptualization: Abdul Momin, Amana Khatoon; Data Curation: Wajahat Khan, Abdul Momin; Formal analysis: Muhammad Nauman Khan, Baber Ali, Alevcan Kaplan; Funding acquisition: Mohammad Khalid Al-Sadoon, Sezai Ercisli, Dilsat Bozdoğan Konuşkan; Investigation: Muhammad Mudasar Aslam, Muhammad Jamil; Methodology: Muhammad Nauman Khan, Sana Wahab; Resources: Shafiq Ur Rehman; Software: Baber Ali, Mohammad Khalid Al-Sadoon; Validation: Abdul Momin, Amana Khatoon, Mohammad Khalid Al-Sadoo, Muhammad Mudasar Aslam; Writing–original draft: Baber Ali, Abdul Momin; Writing–review & editing: Dilsat Bozdoğan Konuşkan, Mohammad Khalid Al-Sadoon, Baber Ali, Sezai Ercisli. All authors reviewed the results and approved the final version of the manuscript.
Availability of Data and Materials: All the data supporting the findings of this study are included in this article.
Ethics Approval: Not applicable.
Conflicts of Interest: The authors declare that they have no conflicts of interest to report regarding the present study.
References
1. Morrow RC. LED lighting in horticulture. HortScience. 2008;43:1947–50. doi:10.21273/HORTSCI.43.7.1947. [Google Scholar] [CrossRef]
2. Jung WS, Chung IM, Hwang MH, Kim SH, Yu CY, Ghimire BK. Application of light-emitting diodes for improving the nutritional quality and bioactive compound levels of some crops and medicinal plants. Molecules. 2021;26:1–18. [Google Scholar]
3. Singh D, Basu C, Meinhardt-Wollweber M, Roth B. LEDs for energy efficient greenhouse lighting. Renew Sustain Energ Rev. 2015;49:139–47. doi:10.1016/j.rser.2015.04.117. [Google Scholar] [CrossRef]
4. Jiao J, Wang B. High-efficiency reflector optics for LED automotive forward lighting. In: Proceedings of the Nonimaging Optics and Efficient Illumination Systems IV; 2007. [Google Scholar]
5. Rédei GP. Encyclopedia of genetics, genomics, proteomics, and informatics. Springer Science & Business Media; 2008. [Google Scholar]
6. Paradiso R, Proietti S. Light-quality manipulation to control plant growth and photomorphogenesis in greenhouse horticulture: the state of the art and the opportunities of modern LED systems. J Plant Growth Regul. 2022;41:742–80. doi:10.1007/s00344-021-10337-y. [Google Scholar] [CrossRef]
7. Carvalho SD, Schwieterman ML, Abrahan CE, Colquhoun TA, Folta KM. Light quality dependent changes in morphology, antioxidant capacity, and volatile production in sweet basil (Ocimum basilicum). Front Plant Sci. 2016;7:1–14. [Google Scholar]
8. Hernández R, Kubota C. Physiological responses of cucumber seedlings under different blue and red photon flux ratios using LEDs. Environ Exp Bot. 2016;121:66–74. doi:10.1016/j.envexpbot.2015.04.001. [Google Scholar] [CrossRef]
9. Lee HJ, Park YJ, Ha JH, Baldwin IT, Park CM. Multiple routes of light signaling during root photomorphogenesis. Trends Plant Sci. 2017;22:803–12. doi:10.1016/j.tplants.2017.06.009. [Google Scholar] [PubMed] [CrossRef]
10. Meng L, Song W, Liu S, Dong J, Zhang Y, Wang C, et al. Light quality regulates lateral root development in tobacco seedlings by shifting auxin distributions. J Plant Growth Regul. 2015;34:574–83. doi:10.1007/s00344-015-9491-z. [Google Scholar] [CrossRef]
11. Klem K, Gargallo-Garriga A, Rattanapichai W, Oravec M, Holub P, Veselá B, et al. Distinct morphological, physiological, and biochemical responses to light quality in barley leaves and roots. Front Plant Sci. 2019;10:1–19. [Google Scholar]
12. He J, Qin L, Chong EL, Choong TW. Plant growth and photosynthetic characteristics of Mesembryanthemum crystallinum grown aeroponically under different blue-and red-LEDs. Front Plant Sci. 2017;8:1–13. [Google Scholar]
13. Yang F, Feng L, Liu Q, Wu X, Fan Y, Raza MA, et al. Effect of interactions between light intensity and red-to-far-red ratio on the photosynthesis of soybean leaves under shade condition. Environ Exp Bot. 2018;150:79–87. doi:10.1016/j.envexpbot.2018.03.008. [Google Scholar] [CrossRef]
14. Bartucca ML, Guiducci M, Falcinelli B, Del Buono D, Benincasa P. Blue: Red LED light proportion affects vegetative parameters, pigment content, and oxidative status of einkorn (Triticum monococcum L. ssp. monococcum) wheatgrass. J Agric Food Chem. 2020;68:8757–63. doi:10.1021/acs.jafc.0c03851. [Google Scholar] [PubMed] [CrossRef]
15. Hogewoning SW, Trouwborst G, Maljaars H, Poorter H, van Ieperen W, Harbinson J. Blue light dose-responses of leaf photosynthesis, morphology, and chemical composition of Cucumis sativus grown under different combinations of red and blue light. J Exp Bot. 2010;61:3107–17. doi:10.1093/jxb/erq132. [Google Scholar] [PubMed] [CrossRef]
16. Bamdad F, Dokhani S, Keramat J, Zareie R. The impact of germination and in vitro digestion on the formation of angiotensin converting enzyme (ACE) inhibitory peptides from lentil proteins compared to whey proteins. Heart Fail. 2009;5:52–62. [Google Scholar]
17. Mbithi S, van Camp J, Rodriguez R, Huyghebaert A. Effects of sprouting on nutrient and antinutrient composition of kidney beans (Phaseolus vulgaris var. rose coco). Eur Food Res Technol. 2001;212:188–91. doi:10.1007/s002170000200. [Google Scholar] [CrossRef]
18. Donkor O, Henriksson A, Vasiljevic T, Shah NP. Probiotic strains as starter cultures improve angiotensin-converting enzyme inhibitory activity in soy yogurt. J Food Sci. 2005;70:m375–81. [Google Scholar]
19. Sallam A, Alqudah AM, Dawood MF, Baenziger PS, Börner A. Drought stress tolerance in wheat and barley: advances in physiology, breeding and genetics research. Int J Mol Sci. 2019;20:1–36. [Google Scholar]
20. Li T, Luo L, Kim S, Moon SK, Moon B. Trans-resveratrol extraction from peanut sprouts cultivated using fermented sawdust medium and its antioxidant activity. J Food Sci. 2020;85:639–46. doi:10.1111/jfds.v85.3. [Google Scholar] [CrossRef]
21. Nakamura K, Naramoto K, Koyama M. Blood-pressure-lowering effect of fermented buckwheat sprouts in spontaneously hypertensive rats. J Funct Foods. 2013;5:406–15. doi:10.1016/j.jff.2012.11.013. [Google Scholar] [CrossRef]
22. Fan X, Zang J, Xu Z, Guo S, Jiao X, Liu X, et al. Effects of different light quality on growth, chlorophyll concentration and chlorophyll biosynthesis precursors of non-heading Chinese cabbage (Brassica campestris L.). Acta Physiol Plant. 2013;35:2721–6. doi:10.1007/s11738-013-1304-z. [Google Scholar] [CrossRef]
23. Liu H, Fu Y, Hu D, Yu J, Liu H. Effect of green, yellow and purple radiation on biomass, photosynthesis, morphology and soluble sugar content of leafy lettuce via spectral wavebands knock out. Sci Hortic. 2018;236:10–7. doi:10.1016/j.scienta.2018.03.027. [Google Scholar] [CrossRef]
24. Rekowski A, Langenkämper G, Dier M, Wimmer MA, Scherf KA, Zörb C. Determination of soluble wheat protein fractions using the Bradford assay. Cereal Chem. 2021;98:1059–65. doi:10.1002/cche.v98.5. [Google Scholar] [CrossRef]
25. Chen XL, Li YL, Wang LC, Guo WZ. Red and blue wavelengths affect the morphology, energy use efficiency and nutritional content of lettuce (Lactuca sativa L.). Sci Rep. 2021;11:1–13. [Google Scholar]
26. Turan MA, Elkarim AHA, Taban N, Taban S. Effect of salt stress on growth, stomatal resistance, proline and chlorophyll concentrations on maize plant. Afr J Agric Res. 2009;4:893–7. [Google Scholar]
27. Dougher TA, Bugbee B. Differences in the response of wheat, soybean and lettuce to reduced blue radiation. Photochem Photobiol. 2001;73:199–207. doi:10.1562/0031-8655(2001)073<0199:DITROW>2.0.CO;2. [Google Scholar] [PubMed] [CrossRef]
28. Park YR, Kwon SJ, Kim JH, Duan S, Eom SH. Light-induced antioxidant phenolic changes among the sprouts of lentil cultivar. Antioxidants. 2024;13:1–15. [Google Scholar]
29. Rahman MM, Field DL, Ahmed SM, Hasan MT, Basher MK, Alameh K. LED illumination for high-quality high-yield crop growth in protected cropping environments. Plants. 2021;10:1–23. [Google Scholar]
30. Lin KH, Huang MY, Huang WD, Hsu MH, Yang ZW, Yang CM. The effects of red, blue, and white light-emitting diodes on the growth, development, and edible quality of hydroponically grown lettuce (Lactuca sativa L. var. capitata). Sci Hortic. 2013;150:86–91. doi:10.1016/j.scienta.2012.10.002. [Google Scholar] [CrossRef]
31. Ohashi-Kaneko K, Takase M, Kon N, Fujiwara K, Kurata K. Effect of light quality on growth and vegetable quality in leaf lettuce, spinach and komatsuna. Environ Control Biol. 2007;45:189–98. doi:10.2525/ecb.45.189. [Google Scholar] [CrossRef]
32. Fan XX, Xu ZG, Liu XY, Tang CM, Wang LW, Han XL. Effects of light intensity on the growth and leaf development of young tomato plants grown under a combination of red and blue light. Sci Hortic. 2013;153:50–5. doi:10.1016/j.scienta.2013.01.017. [Google Scholar] [CrossRef]
33. Kim HH, Goins GD, Wheeler RM, Sager JC. Green-light supplementation for enhanced lettuce growth under red-and blue-light-emitting diodes. HortScience. 2004;39:1617–22. doi:10.21273/HORTSCI.39.7.1617. [Google Scholar] [CrossRef]
34. Johkan M, Shoji K, Goto F, Hashida SN, Yoshihara T. Blue light-emitting diode light irradiation of seedlings improves seedling quality and growth after transplanting in red leaf lettuce. HortScience. 2010;45:1809–14. doi:10.21273/HORTSCI.45.12.1809. [Google Scholar] [CrossRef]
35. Peters RD, Noble SD. Spectrographic measurement of plant pigments from 300 to 800 nm. Remote Sens Environ. 2014;148:119–23. doi:10.1016/j.rse.2014.03.020. [Google Scholar] [CrossRef]
36. Sæbø A, Krekling T, Appelgren M. Light quality affects photosynthesis and leaf anatomy of birch plantlets in vitro. Plant Cell, Tissue Organ Cult. 1995;41:177–85. doi:10.1007/BF00051588. [Google Scholar] [CrossRef]
37. Dong F, Wang C, Sun X, Bao Z, Dong C, Sun C, et al. Sugar metabolic changes in protein expression associated with different light quality combinations in tomato fruit. Plant Growth Regul. 2019;88:267–82. doi:10.1007/s10725-019-00506-1. [Google Scholar] [CrossRef]
38. Bhandari R, Sharma PK. High-light-induced changes on photosynthesis, pigments, sugars, lipids and antioxidant enzymes in freshwater (Nostoc spongiaeforme) and marine (Phormidium corium) cyanobacteria. Photochem Photobiol. 2006;82:702–10. doi:10.1562/2005-09-20-RA-690. [Google Scholar] [PubMed] [CrossRef]
Cite This Article
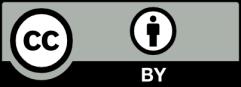
This work is licensed under a Creative Commons Attribution 4.0 International License , which permits unrestricted use, distribution, and reproduction in any medium, provided the original work is properly cited.