Open Access
ARTICLE
Monitoring Xylem Transport in the Stem of Lilium lancifolium Using Fluorescent Dye 5(6)-Carboxyfluorescein Diacetate
1 College of Horticulture, Shanxi Agricultural University, Jinzhong, 030801, China
2 State Key Laboratory of Vegetable Biobreeding, Institute of Vegetables and Flowers, Chinese Academy of Agricultural Sciences, Beijing, 100081, China
3 College of Urban and Rural Construction, Shanxi Agricultural University, Jinzhong, 030801, China
* Corresponding Authors: Fang Du. Email: ; Jun Ming. Email:
(This article belongs to the Special Issue: Botany, Phytochemistry and Biological Properties of Medicinal and Aromatic Plants )
Phyton-International Journal of Experimental Botany 2024, 93(5), 1057-1066. https://doi.org/10.32604/phyton.2024.051197
Received 29 February 2024; Accepted 12 April 2024; Issue published 28 May 2024
Abstract
The xylem undergoes physiological changes in response to various environmental conditions during the process of plant growth. To understand these physiological changes, it is extremely important to observe the transport of xylem. In this study, the distribution and structure of vascular bundle in Lilium lancifolium were observed using the method of semithin section. Methods for introducing a fluorescent tracer into the xylem of the stems were evaluated. Then, the transport rule of 5(6)-Carboxyfluorescein diacetate (CFDA) in the xylem of the stem of L. lancifolium was studied by fluorescence dye in live cells tracer technology. The results showed that the vascular bundles of L. lancifolium were scattered in the basic tissue, the peripheral vascular bundles were smaller and densely distributed, and the closer to the center, the larger the volume of vascular bundles and the more sparsely distributed. The vascular bundles of L. lancifolium are limited external tenacity vascular bundles, which are composed of phloem and xylem. The most suitable method for CFDA labeling the xylem of isolated stem segments of L. lancifolium was solution soaking for 24 h. The running speed of CF in the isolated stem was 0.3 cm/h, which was consistent with the running speed of the material in the field. CF could be transported between the xylem and parenchyma cells, indicating that the material transport in the xylem could be through the symplastic pathway. The above results laid a foundation for the study of the xylem transport mechanism and the xylem pathogen disease of lily.Keywords
In vascular plants, vascular bundles are necessary structures for transporting substances, which consist of xylem and phloem. The xylem provides the mechanical support and stability that plants need to sustain themselves and expand their growth and carries substances such as water, minerals, inorganic salts, and countless signaling molecules over long distances from the roots to all above-ground parts [1–4]. The study of xylem has attracted many scholars for more than a century [5–7]. Some studies have found that the xylem of monocotyledonous plants has stronger plasticity, and the size and quantity of the xylem will change under different water conditions [8,9]. The high plasticity of xylem development is very important for the environmental adaptation of plant growth. To cope with the change in environmental conditions, the xylem will produce physiological changes accordingly, so it is very important to observe the transport of the xylem.
Lilies have high requirements for the physical and chemical properties of the growth environment, and the growth of lilies varies greatly in unsuitable acid-base environments. Under saline-alkali stress, it will be difficult to absorb water which reduces the absorption capacity of minerals, resulting in unhealthy growth and even death of plants [10]. The studies conducted by Carlquist have demonstrated that the xylem structure significantly influences water transport [8,9]. Moreover, it has been observed that xylem development varies among different plant species and even within the same plant under diverse stress conditions. Therefore, the study of the xylem structure and water transport of lily will be of great significance for the analysis of physiological changes in response to stress.
Some scholars have studied xylem transport and developed some methods to monitor it; the dye method is the most popular [11,12]. In the Arabidopsis xylem study, ink containing agar was applied to the roots to observe the shootward transport of ink in Arabidopsis seedlings, but it was not confirmed that the ink was only transported in the xylem [13]. Rhodamine B and fluorescein sodium salt have been applied to Arabidopsis roots to trace the transport of substances in the xylem from root to leaf, making it possible to measure the transport velocity [14]. Texas Red (sulforhodamine 101 acid chloride) has also been used to observe xylem connectivity in Arabidopsis [15]. Carboxyfluorescein diacetate (CFDA) is a fluorescent dye, which is broken down into CF by enzymes. The advantage of using CFDA is that it fluoresces strongly only after entering living tissue [16]. It was widely used in monitoring xylem transport in Arabidopsis, Populus, Aceraceous, and other plants [16,17], and widely used to observe the recovery of xylem transport capacity in plant grafting [18–21]. In addition, CFDA is often introduced through leaves as a phloem tracer [22]. However, CFDA introduced from the root can only be transported upward through the xylem [23].
In this study, the distribution and structure of the vascular bundle of L. lancifolium were observed using the method of semithin section, and CFDA was used as a fluorescent tracer to simulate the xylem transport of lily stems. The rate of xylem transport was measured, which provides new ideas for studying the growth and development of lily plants in the process of adapting to the environment. It also provides new ideas for understanding the response of xylem to fusarium wilt pathogens to prevent and control these diseases.
The autotriploid species L. lancifolium was used as material in this study. L. lancifolium bulbs of uniform size (about 12 cm in circumference) harvested in September 2022 were embedded with substrate and stored at 4°C at the Institute of Vegetables and Flowers, Chinese Academy of Agricultural Sciences, Beijing, China. When the stems of the bulbs grew to 15 cm in length, they were cut into segments of 1.5 cm according to He et al. [24] after surface sterilization for the CFDA labeling test. L. lancifolium bulbs of uniform size with 1–2 cm buds were grown in soil in a greenhouse in early April of 2023. The CFDA labeling test was carried out when the length reached 40 cm.
2.2 Observation of the Microscopic Structure of L. lancifolium Stem
The microscopic structure of L. lancifolium stem was observed using the method described by Zhang et al. [25], with minor modifications. The stem tissue of 1 mm3 was cut and fixed at 4°C for 24 h using a 3% glutaraldehyde fixative. The fixed stems were rinsed with Phosphate-buffered PBS six times for 5 min each, and then fixed with 1% fixative Osmium tetroxide at room temperature for 2 h. The samples were rinsed again with Phosphate-buffered PBS six times for 5 min each, and then dehydrated by gradient ethanol. The samples were dehydrated twice for 10 min each with 100% acetone at room temperature and then embedded with Spurrresin. Semi-thin sections were obtained using the ultra-thin microtome (A130373) and double-stained with uranyl acetate and lead citrate.
2.3 CFDA Labeling in Isolated Stem Segment of L. lancifolium
Three CFDA labeling methods were used to label the isolated stem of L. lancifolium. (1) Cutting wound introduction: At 0.7 cm below the stem node, a double-sided blade was used to cut inward from the epidermis, creating a wound with a depth of 1 mm. The wound was wrapped with absorbent cotton covered with 200 μL CFDA solution (1 mg/mL, Beijing Solarbio Science & Technology Co., Ltd., Beijing, China), then wrapped with tinfoil and finally cultured on MS+ 60 g/L sucrose medium (Fig. 1A). (2) Medium introduction: The sterilized single stem segments were directly cultured on MS+ 60 g/L sucrose + 1 g/L CFDA medium (Fig. 1B). (3) Soaking introduction: 200 μL CFDA working fluid was added into a 2 mL centrifuge tube, and the single stem segment was directly inserted into the CFDA working fluid (Fig. 1C). The treated materials were cultured away from light, and samples labeled for 12, 24, 48, 72 h were taken for observation, respectively.
Figure 1: CFDA labeling methods in isolated stem segment of L. lancifolium. (A) Cutting wound introduction, (B) Medium introduction, (C) Soaking introduction. The scale bar is 50 mm
2.4 CFDA Labeling in Field Plants of L. lancifolium
The method of CFDA labeling in field plants of L. lancifolium was performed according to the method of Zhang et al. [25]. At 1 cm below one stem node of the middle-upper stem, a double-sided blade was used to cut inward from the epidermis, creating a wound with a depth of 1 mm. The wound was wrapped with absorbent cotton covered with 200 μL CFDA solution (1 mg/mL), then wrapped with tinfoil to avoid light (Fig. 2). Biology is repeated three times.
Figure 2: CFDA labeling method in field plant of L. lancifolium. The scale bar is 1 cm
2.5 Transport of CFDA in the Stem of L. lancifolium
The introduction position was as the starting point (0 cm). After the introduction of CFDA, cross sections were taken every 1 h at the distance 0, 0.3, 0.6, 0.9, and 1.2 cm from the introduction position of CFDA. The sections (150 μm) were obtained using a vibrating microtome. The operation of CF in the vascular bundle of the stem was observed using a Confocal laser scanning microscope (CLSM, TCS SP8), and the excitation wavelength was 488 nm. The biology experiment was repeated three times.
3.1 Microscopic Structure of L. lancifolium Stem
The cross-section of the L. lancifolium stem is shown in Fig. 3, the L. lancifolium stem was composed of four parts: epidermis, mechanical tissue, basic tissue, and vascular bundle. The epidermis (ep) cells were oval and tightly packed, covered with the stratum corneum. The lower part of the epidermis was mechanical tissue (mt). The finite vascular bundles were scattered in ground tissue (gt) without vascular sheath. The lateral vascular bundles were small and densely distributed, and the central vascular bundles were large and loosely distributed (Fig. 3A). The vascular bundle (VB) consisted of phloem (Ph) and xylem (Xy) (Fig. 3B). The phloem is composed of sieve tubes, companion cells, and parenchyma cells.
Figure 3: Microscopic structure of L. lancifolium stem. (A) The cross-section of L. lancifolium stem, epidermis (ep) cells, mechanical tissue (mt), vascular bundle (VB), ground tissue (gt). The scale bar is 200 μm. (B) Vascular bundle, Xy: Xylem, Ph: Phloem, PP: Phloem parenchyma cell, gt: Ground tissue. The scale bar is 10 μm
3.2 The Optimal CFDA Labeling for L. lancifolium Xylem in Vitro
Three CFDA labeling methods were used to label the stem tissue. The stem tissue located 0.9 cm above the introduction site was cut for observation at 12, 24, 48, and 72 h, respectively. The results implied three CFDA labeling methods showed the same rule at different labeling times. The fluorescence signal showed a trend of first strengthening and then weakening with the extension of labeling time. The fluorescence signal with labeling 24 h was the strongest (Fig. 4). In our study, the use of the wound introduction method might cause some problems, such as the vascular bundle far from the wound having a slower fluorescence signal, or even no fluorescence signal. Therefore, this method of introduction is unstable. Then, we conducted a medium introduction and found that the medium introduction method affected the activity of CFDA due to the high medium temperature. In conclusion, the most suitable method for CFDA labeling of isolated stem segments of L. lancifolium was solution soaking for 24 h. In addition, Texas Red was also used for cross-staining, and the two fluorescence signals were found to overlap, indicating that CFDA was successfully transported upward from the xylem (Fig. 5).
Figure 4: Fluorescence signals of three CFDA labeling methods at different labeling times. (A) Cutting wound introduction, (B) Medium introduction, (C) Soaking introduction. The scale bar is 50 μm
Figure 5: Schematic diagram of two fluorescein pairs. The scale bar is 75 μm
3.3 Transport of CF in Xylem of L. lancifolium
3.3.1 Longitudinal Transport of CF in the Xylem of Field Plants
The introduction position was as the starting point (0 cm). In field plants treated with CFDA, fluorescence signals were not immediately observed in the xylem of stems at the starting point, but were observed after 1 h, indicating that fluorescence was transversely transported from the mechanical tissue to the vascular bundle. Fluorescence began to transport upward from 1 h after the introduction of CFDA, and the fluorescence signal was observed in the xylem of the stem located 0.3 cm above the CFDA introduction site after CFDA introduction for 2 h. A weak fluorescence signal was observed in the xylem of the stem located 0.6 cm above the CFDA introduction site after CFDA introduction for 3 h. A weak fluorescence signal was observed in the xylem of the stem located 0.9 cm above the CFDA introduction site after CFDA introduction for 4 h. A weak fluorescence signal was observed in the xylem of the stem located 1.2 cm above the CFDA introduction site after CFDA introduction for 5 h (Fig. 6). Therefore, it can be concluded that the running speed of CF in the stem was 0.3 cm/h.
Figure 6: Longitudinal transport of CF in the stem of the field plant of L. lancifolium. The scale bar is 25 μm
3.3.2 Longitudinal Transport of CF in the Isolated Stem of L. lancifolium
The introduction of CFDA to the isolated stems of L. lancifolium was performed, no fluorescence signal was observed in the vascular bundle of the transverse section at 0.3 cm when labeled for 0.5 h (Fig. 7). Whereas, when CFDA was introduced for 1 h, the fluorescent signal could be found in the xylem. With the increase of time, the fluorescence signal of phloem was gradually enhanced. The stem located 0.6 cm above the CFDA introduction site was sliced and observed. The results showed that a weak fluorescence signal was found in the xylem after CFDA introduction for 2 h. A weak fluorescence signal was found in the vascular bundle after CFDA introduction for 3 h when the stem was located 0.9 cm above the CFDA introduction site. A weak fluorescence signal was found in the xylem after CFDA introduction for 4 h when the stem was located 1.2 cm above the CFDA introduction site. It can be concluded that the running speed of CF in the isolated stem was 0.3 cm/h, which was consistent with the running speed of the material in the field.
Figure 7: Longitudinal transport of CF in the isolated stem of L. lancifolium. The scale bar is 25 μm
3.3.3 Transversal Transport of CF in the Stem of L. lancifolium
The results showed that fluorescence signals were present in parenchyma cells in both the isolated stem and the field plant (Fig. 8), indicating that CF could be transported between the xylem and parenchyma cells. In our study, it was found that the fluorescence signals were not only found in the xylem but also in parenchyma cells. CF is impenetrable. There is a channel between the cells in the vascular bundle, which enables the transport of CF to be unimpeded. Therefore, we concluded that there was a symplastic pathway for the xylem transport of L. lancifolium.
Figure 8: The distribution of CF in parenchyma cells and xylem in the stem of L. lancifolium. The scale bar is 50 μm
Understanding the structure of vascular bundles is fundamental to the successful introduction of fluorescein. There were no secondary structures in the vascular bundles in L. lancifolium, which was consistent with lilies such as Lilium Asiatic Hybrids [26]. The mechanical tissue gives the stem a certain hardness and lodging resistance [27]. The successful introduction of fluorescein is fundamental to the observation of xylem material transport. In this experiment, three fluorescent dye introduction methods were used: wound introduction method, solution immersion method, and medium introduction method. At present, wound introduction is widely used [28,29]. In our study, the use of the wound introduction method might cause some problems, such as the vascular bundle having no fluorescent signal, which might be due to the wound vascular bundle secreting callose and blocking the wound [30,31]. Then, we conducted a medium introduction and found that the medium introduction method affected the activity of CFDA due to the high medium temperature. This method is mainly used in cells [32] because the liquid medium is used in cells, the fluorescence agent will not be affected by temperature and produce instability, but this experiment can only use a solid medium, so this method is not suitable for this experiment. The solution soaking method was relatively more effective and stable and had been successfully applied to tomatoes [33].
In different species, the vascular structure is different, the number and size of vascular cells are different, and therefore, the transport rate of fluorescein is also different. Péron et al. found that fluorescent signals were found in the root cells of Phelipanche ramosa 2 h after the introduction of CFDA to the leaves of the host plant (Brassica napus) [34]. Zhang et al. found that it took 4 h for CFDA to reach the phloem of apple fruit when it was introduced from the carpopodium of apple, and at least 16 h for CFDA to reach apple fruit when it was applied to the surface of leaves [25]. Ren found that it took 5 h for CFDA to reach the stem vascular bundle after introducing it from the petiole of Neolamarckia cadamba [35]. When the ink was applied to the roots in Arabidopsis thaliana, ink was found in the shoots within a few hours, and Rhodamine B and fluorescein sodium salt were applied to the lower part of the taproot in 14-day-old Arabidopsis seedlings, and fluorescent signals were found in the upper root zone within a few minutes [14]. In our study, the transport rate of CF in the curly stems was 0.3 cm/h. It can be seen that different species, different application sites, and different fluorescent tracers may change the transport speed, the marking time can be reasonably selected according to the speed of species transport. Therefore, the study of xylem transport should continue in the future. In addition, we also found that the fluorescent signal of CF appeared in parenchyma cells, which indicates that there may be a symplastic mode in the transport of xylem substances in the L. lancifolium, which is consistent with the results of Katarzyna. In a study of Aceraceae plants, CFDA was used to trace xylem transport over long distances, and fluorescent signals were found in both xylem and parenchyma cells of branches, confirming that water and minerals transport in trees not only in the form of exosomes but also in the form of symplastic [17].
The finite vascular bundles of L. lancifolium consisted of phloem and xylem. The vascular bundles were smaller on the periphery of the stem, while those near the center were larger and dispersed. The most suitable method for CFDA labeling the xylem of isolated stem segments of L. lancifolium was solution soaking for 24 h. The running speed of CF in the isolated stem was 0.3 cm/h, which was consistent with the running speed of the material in the field. CF could be transported between xylem and parenchyma cells, and could not be transported to the phloem.
Acknowledgement: We thank Jianan Wu and Fan Wang from the Core Facility Platform, Institute of Crop Sciences, Chinese Academy of Agricultural Sciences (CAAS) for their assistance with TEM analysis.
Funding Statement: This study was supported by the National Natural Science Foundation of China (31902043, 32172612).
Author Contributions: Yulin Luo: Methodology, Data curation, Investigation, Visualization, Formal analysis, Writing-original draft. Mengmeng Bi: Resources, Visualization. Leifeng Xu: Resources, Visualization. Fang Du: Resources, Visualization. Panpan Yang: Methodology, Visualization, Formal analysis, Writing-original draft, Project administration. Jun Ming: Conceptualization, Validation, Writing-review & editing, Supervision, Project administration, Funding acquisition. All authors reviewed the results and approved the final version of the manuscript.
Availability of Data and Materials: The datasets analysed during the current study are available from the corresponding author on reasonable request.
Ethics Approval: Not applicable.
Conflicts of Interest: The authors declare that they have no conflicts of interest to report regarding the present study.
References
1. Jacobs WP. The role of auxin in differentiation of xylem around a wound. Am J Bot. 1952;39(5):301–9. doi:10.1002/ajb2.1952.39.issue-5. [Google Scholar] [CrossRef]
2. Mayer U, Ruiz RAT, Berleth T, Miséra S, Jürgens G. Mutations affecting body organization in the Arabidopsis embryo. Nature. 1991;353(6343):402–7. doi:10.1038/353402a0. [Google Scholar] [CrossRef]
3. Turner SR, Somerville CR. Collapsed xylem phenotype of Arabidopsis identifies mutants deficient in cellulose deposition in the secondary cell wall. Plant Cell. 1997;9(5):689–701. [Google Scholar] [PubMed]
4. Yokoyama R, Nishitani K. Identification and characterization of Arabidopsis thaliana genes involved in xylem secondary cell walls. J Plant Res. 2006;119:189–94. doi:10.1007/s10265-006-0261-7. [Google Scholar] [PubMed] [CrossRef]
5. Herud-Sikimić O, Stiel AC, Kolb M, Shanmugaratnam S, Berendzen KW, Feldhaus C, et al. A biosensor for the direct visualization of auxin. Nature. 2021;592(7856):768–72. doi:10.1038/s41586-021-03425-2. [Google Scholar] [PubMed] [CrossRef]
6. Turco GM, Rodriguez-Medina J, Siebert S, Han D, Valderrama-Gómez MÁ, Vahldick H, et al. Molecular mechanisms driving switch behavior in xylem cell differentiation. Cell Rep. 2019;28(2):342–51. doi:10.1016/j.celrep.2019.06.041. [Google Scholar] [PubMed] [CrossRef]
7. McCarthy RL, Zhong R, Ye ZH. MYB83 is a direct target of SND1 and acts redundantly with MYB46 in the regulation of secondary cell wall biosynthesis in Arabidopsis. Plant Cell Physiol. 2009;50(11):1950–64. doi:10.1093/pcp/pcp139. [Google Scholar] [PubMed] [CrossRef]
8. Carlquist S. Ecological strategies of xylem evolution. Berkeley: UC Press; 1975. [Google Scholar]
9. Carlquist S. Monocot xylem revisited: new information, new paradigms. Bot Rev. 2012;78:87–153. doi:10.1007/s12229-012-9096-1. [Google Scholar] [CrossRef]
10. Chang X, Zhu G, Chen S, Sun D, He H, Li G, et al. AtPHB2 regulates salt stress response in Arabidopsis thaliana. Plant Growth Regul. 2021;94:23–32. doi:10.1007/s10725-021-00692-x. [Google Scholar] [CrossRef]
11. Brodersen CR, Roddy AB, Wason JW, McElrone AJ. Functional status of xylem through time. Annu Rev Plant Biol. 2019;70:407–33. doi:10.1146/arplant.2019.70.issue-1. [Google Scholar] [CrossRef]
12. Konrad W, Katul G, Roth-Nebelsick A, Jensen KH. Xylem functioning, dysfunction and repair: a physical perspective and implications for phloem transport. Tree Physiol. 2019;39(2):243–61. doi:10.1093/treephys/tpy097. [Google Scholar] [PubMed] [CrossRef]
13. Li S, Chen M, Yu D, Ren S, Sun S, Liu L, et al. EXO70A1-mediated vesicle trafficking is critical for tracheary element development in Arabidopsis. Plant Cell. 2013;25(5):1774–86. doi:10.1105/tpc.113.112144. [Google Scholar] [PubMed] [CrossRef]
14. Endo S, Iwai Y, Fukuda H. Cargo-dependent and cell wall-associated xylem transport in Arabidopsis. New Phytol. 2019;222(1):159–70. doi:10.1111/nph.2019.222.issue-1. [Google Scholar] [CrossRef]
15. Birschwilks M, Sauer N, Scheel D, Neumann S. Arabidopsis thaliana is a susceptible host plant for the holoparasite Cuscuta spec. Planta. 2007;226:1231–41. doi:10.1007/s00425-007-0571-6. [Google Scholar] [PubMed] [CrossRef]
16. Breeuwer P, Drocourt JL, Bunschoten N, Zwietering MH, Rombouts FM, Abee T. Characterization of uptake and hydrolysis of fluorescein diacetate and carboxyfluorescein diacetate by intracellular esterases in Saccharomyces cerevisiae, which result in accumulation of fluorescent product. Appl Environ Microb. 1995;61(4):1614–9. doi:10.1128/aem.61.4.1614-1619.1995. [Google Scholar] [PubMed] [CrossRef]
17. Sokołowska K, Zagórska-Marek B. Symplasmic, long-distance transport in xylem and cambial regions in branches of Acer pseudoplatanus (Aceraceae) and Populus tremula× P. tremuloides (Salicaceae). Am J Bot. 2012;99(11):1745–55. doi:10.3732/ajb.1200349. [Google Scholar] [PubMed] [CrossRef]
18. Melnyk CW. Monitoring vascular regeneration and xylem connectivity in Arabidopsis thaliana. Xylem: Methods and Protocols. 2017;1544:91–102. doi:10.1007/978-1-4939-6722-3. [Google Scholar] [CrossRef]
19. Bartusch K, Trenner J, Melnyk CW, Quint M. Cut and paste: temperature-enhanced cotyledon micrografting for Arabidopsis thaliana seedlings. Plant Methods. 2020;16(1):1–11. [Google Scholar]
20. Serivichyaswat PT, Bartusch K, Leso M, Musseau C, Iwase A, Chen Y, et al. High temperature perception in leaves promotes vascular regeneration and graft formation in distant tissues. Development. 2022;149(5):dev200079. doi:10.1242/dev.200079. [Google Scholar] [PubMed] [CrossRef]
21. Jiang M, Deng Z, White RG, Liang D. Shootward movement of CFDA tracer loaded in the bottom sink tissues of Arabidopsis. J Vis Exp. 2019;147:e59606. [Google Scholar]
22. Knoblauch M, Vendrell M, de Leau E, Paterlini A, Knox K, Ross-Elliot T, et al. Multispectral phloem-mobile probes: properties and applications. Plant Physiol. 2015;167(4):1211–20. doi:10.1104/pp.114.255414. [Google Scholar] [PubMed] [CrossRef]
23. Bartusch K, Blanco-Touriñán N, Rodriguez-Villalón A, Truernit E. Monitoring xylem transport in Arabidopsis thaliana seedlings using fluorescent dyes. Methods Mol Biol. 2023;2722:3–15. [Google Scholar]
24. He GR, Yang PP, Tang YC, Cao YW, Qi XY, Xu LF, et al. Mechanism of exogenous cytokinins inducing bulbil formation in Lilium lancifolium in vitro. Plant Cell Rep. 2020;39:861–72. doi:10.1007/s00299-020-02535-x. [Google Scholar] [PubMed] [CrossRef]
25. Zhang LY, Peng YB, Pelleschi-Travier S, Fan Y, Lu YF, Lu YM, et al. Evidence for apoplasmic phloem unloading in developing apple fruit. Plant Physiol. 2004;135(1):574–86. doi:10.1104/pp.103.036632. [Google Scholar] [PubMed] [CrossRef]
26. Sun YM, Wang WH, Wang SD, Zhao XY, Zhang K. Analysis on anatomical structure of vegetative organs of different lilium varieties. In: Chinese Society of Horticulture Ornamental Horticulture Professional Committee 2013 Academic Annual Meeting; 2013 Aug 2; Zhengzhou, China. [Google Scholar]
27. Özen FAZIL, Temeltaş HACI, Aksoy ÖZLEM. The anatomy and morphology of the medicinal plant, Lilium candidum L. (Liliaceaedistributed in Marmara region of Turkey. Pak J Bot. 2012;44(4):1185–92. [Google Scholar]
28. Zhou Y, Li K, Wen S, Yang D, Gao J, Wang Z, et al. Phloem unloading in cultivated melon fruits follows an apoplasmic pathway during enlargement and ripening. Hortic Res. 2023;10(8):uhad123. doi:10.1093/hr/uhad123. [Google Scholar] [PubMed] [CrossRef]
29. Walsh KB, Sky RC, Brown SM. The anatomy of the pathway of sucrose unloading within the sugarcane stalk. Funct Plant Biol. 2005;32(4):367–74. doi:10.1071/FP04102. [Google Scholar] [PubMed] [CrossRef]
30. Hao P, Liu C, Wang Y, Chen R, Tang M, Du B, et al. Herbivore-induced callose deposition on the sieve plates of rice: an important mechanism for host resistance. Plant Physiol. 2008;146(4):1810–20. doi:10.1104/pp.107.111484. [Google Scholar] [PubMed] [CrossRef]
31. Kuśnierczyk A, Winge PER, Jørstad TS, Troczyńska J, Rossiter JT, Bones AM. Towards global understanding of plant defence against aphids-timing and dynamics of early Arabidopsis defence responses to cabbage aphid (Brevicoryne brassicae) attack. Plant cell Environ. 2008;31(8):1097–115. doi:10.1111/pce.2008.31.issue-8. [Google Scholar] [CrossRef]
32. Wang WL, Xie B, Meng FR, Wang N, Yang Y, Liu ZH, et al. Experimental study on detection of dead and live Mycobacterium tuberculosis by flow cytometry. J Sun Yat-sen University (Medical Sciences). 2019;40(3):372–8 (In Chinese). [Google Scholar]
33. Chai L, Wang H, Yu H, Pang E, Lu T, Li Y, et al. Girdling promotes tomato fruit enlargement by enhancing fruit sink strength and triggering cytokinin accumulation. Front Plant Sci. 2023;14:1174403. doi:10.3389/fpls.2023.1174403. [Google Scholar] [PubMed] [CrossRef]
34. Péron T, Candat A, Montiel G, Veronesi C, Macherel D, Delavault P, et al. New insights into phloem unloading and expression of sucrose transporters in vegetative sinks of the parasitic plant Phelipanche ramosa L. (Pomel). Front Plant Sci. 2017;7:2048. [Google Scholar]
35. Ren CX. The tracing of pathway for carbohydrate by CFDA in Neolamarckia cadamba. Guangzhou, China: South China Agricultural University; 2017 (In Chinese). [Google Scholar]
Cite This Article
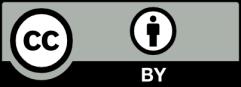
This work is licensed under a Creative Commons Attribution 4.0 International License , which permits unrestricted use, distribution, and reproduction in any medium, provided the original work is properly cited.