Open Access
ARTICLE
Physiological Mechanism of Exogenous Selenium in Alleviating Mercury Stress on Pakchoi (Brassica campestris L.)
1 College of Horticulture and Gardening, Yangtze University, Jingzhou, 434025, China
2 Enshi Se-Run Material Engineering Technology Co., Ltd., Enshi, 445000, China
3 Henry Fok School of Biology and Agricultural, Shaoguan University, Shaoguan, 512005, China
4 National R&D for Se-Rich Agricultural Products Processing Technology, Wuhan Polytechnic University, Wuhan, 430023, China
* Corresponding Author: Feng Xu. Email:
(This article belongs to the Special Issue: Abiotic Stress Impacts on Plant Physiology and Their Alleviation)
Phyton-International Journal of Experimental Botany 2024, 93(5), 951-962. https://doi.org/10.32604/phyton.2024.050893
Received 21 February 2024; Accepted 02 April 2024; Issue published 28 May 2024
Abstract
The objective of this study was to explain the physiological mechanisms through which NaSeO mitigates the growth and developmental inhibition of pakchoi under HgCl stress. The results showed that treatment with HgCl (40 mg L) led to reduced biomass, dwarfing, root shortening, and root tip necrosis in pakchoi. Compared to control (CK), the activities of superoxide dismutase (SOD) and peroxidase (POD) in Hg treatment increased, and the content of malondialdehyde (MDA) also dramatically increased, which negatively impacted the growth of pakchoi. Low concentrations of NaSeO (0.2 mg L) significantly increased the content of soluble sugars compared with control, while chlorophyll, soluble proteins, free amino acids, and vitamin C had no significant changes. The results of the mixed treatments with HgCl and NaSeO suggested that selenium may be able to reduce the toxicity of mercury in pakchoi. The biomass, plant height, root length, chlorophyll content, soluble protein, other physiological indicators, and proline showed significant increases compared with the HgCl treatment. Additionally, the MDA content and mercury accumulation in pakchoi decreased. Our results revealed the antagonistic effects of selenium and mercury in pakchoi. Thus, a theoretical basis for studying pakchoi’s mercury-excreted and selenium-rich cultivation technology was provided.Keywords
Mercury (Hg) is a highly toxic heavy metal known for its neurotoxic and teratogenic properties [1]. Human industrial activities and inadequate management strategies have led to a significant influx of Hg into the Earth’s internal circulation, resulting in approximately 20% of cropland and 50% of agricultural land worldwide being salinity-stressed [2]. Hg pollution has emerged as a major concern worldwide [3]. The average Hg content in China is 0.04 mg kg−1, higher than the global average [4]. Plants’ absorption of elemental Hg and its subsequent enrichment in the food chain seriously threaten food safety. Consuming food with excessive Hg levels leads to the misassembly of Hg2+ within proteins, replacing the original protein binding sites and binding to proteins containing sulfhydryl groups. This process triggers the overproduction of reactive oxygen species (ROS) in organisms, leading to membrane damage and changes in the activity of antioxidant system enzymes in plants [5]. Consequently, Hg becomes a biological and environmental contamination source, leading to potential health risks [6].
Selenium (Se) is a crucial trace element for the human body and is often called the “life protector” [7]. Modern medicine has established that Se deficiency can lead to various diseases, including growth retardation, abnormal thyroid function, Creutzfeldt–Jakob disease, and macrosomia [8]. Humans primarily obtain Se through dietary sources, with plants being the biosphere’s main natural organic Se contributors [9]. Essentiality of Se in plants for plants has not been fully established, but research has demonstrated that appropriate concentrations of exogenous Se can enhance crop yields and improve crop quality [10]. Se also improves antioxidant capacity and stress resistance in plants [11,12]. Moreover, Se has been found to inhibit plant Hg uptake, as evidenced in studies involving rice and garlic [13,14].
Brassica campestris L. (pakchoi), a common vegetable in China, belongs to the family Cruciferae. It is characterized by a short growth cycle, easy cultivation, high nutrient content, and excellent capacity for Se enrichment, making it an ideal Se-rich vegetable [15]. The quality and safety of pakchoi are threatened by Hg contamination. Some studies have indicated that appropriate spray concentrations of Se (≤1.0 mg kg–1) significantly contribute to the growth and detoxification of Hg in Cruciferous vegetables [16,17]. However, research on the physiological metabolism of Se concerning the promotion of Hg detoxification under Hg stress is lacking. Therefore, the present study aimed to utilize sodium selenite (0.2 mg L–1 Na2SeO3) as a Se source to explore its effect on pakchoi-growth quality under mercuric chloride (40 mg L–1 HgCl2) stress. Changes in the growth indices, physiological indices, and antioxidant enzymes of pakchoi under the influence of Se and Hg were analyzed. The findings can serve as a theoretical basis and technical support for exploring Pakchoi’s Se-rich and Hg-resistant cultivation techniques.
Pakchoi seeds were purchased from Jingzhou Agricultural Market (Jingzhou, China). The variety was Golden Cabbage from Hualing Hi-Tech (Jingzhou, China).
2.2 Plant Culture and Treatments
The pakchoi was raised in a hole-tray substrate (soil–vermiculite–perlite = 5:1:1) and sown in late September 2022. It was subsequently transplanted uniformly in hydroponic tanks (410 mm × 260 mm × 140 mm) after growing four to six true leaves, to which pure water without a nutrient solution was added. Seven days after transplanting, plants with uniform growth were selected and planted in new incubators with a medium containing one-fourth concentration of nutrient solution, configured based on Hoagland hydroponics experimental formula and Arnon micronutrient formula [18]. Nine plants were grown in each incubator as a group. Each group was a biological replicate and was repeated three times. Plants were treated with mercuric chloride alone (40 mg L–1) [19], sodium selenite alone (0.2 mg L–1) [20], a combination of mercuric chloride and sodium selenite (HgCl2 40 mg L–1 + Na2SeO3 0.2 mg L–1), and no treatment control (CK) using a three-factor design. The interval for each treatment was one week, with a total of three times. After three weeks, plant materials were harvested and washed. Then, the underground and aboveground were partly frozen in liquid nitrogen and stored in a refrigerator at −80°C for further use.
2.3 Detection of Total Se and Total Hg Contents
Hg content was determined based on the method of Deng et al. [21] through hydride-generation atomic fluorescence spectrometry (Beijing Haiguang Instrument Co., Beijing, China). In a typical procedure, 0.5 g of pakchoi sample was weighed and placed in a digestion jar. After adding 5 mL of nitric acid, the jar was covered with a lid that was tightened. It was left overnight to allow digestion according to the standard operation procedure of the microwave-digestion instrument. It was taken out after cooling, and the lid was slowly opened to exhaust the gas. A small amount of water was used to rinse the inner lid, and the digestion jar was placed on a temperature-controlled hot plate, which was heated at 80°C to drive off the brown gas. The degradation solution was collected and transferred into 25 mL plastic volumetric flasks. The inner jar was washed three times with a small amount of water, and the washing solution was combined in the volumetric flask and fixed to the standard line. After mixing well, it was left to determine the total Hg content.
The total Se content of pakchoi was detected according to our previous report with minimum modification [22]. In a typical procedure, 0.2 g of samples were weighed, placed in a digestion tube, and digested with 10 mL of HNO3 and 2 mL of H2O2 in a micro-wave-digestion system. The solution was added with 5 mL of HCl and continuously heated on a heating plate until it became transparent and white smoke was generated. Its volume was fixed to 10 mL with deionized water for total Se determination.
2.4 Determination of Soluble Protein, Soluble Sugar, Free Amino Acid, Vitamin C, and Chlorophyll Content
The contents of soluble protein, sugar, free amino acid, and vitamin C were determined as described by Gui et al. [20]. Soluble protein was evaluated by Coomassie Brilliant Blue G-250 colorimetry at 595 nm. Soluble sugar was measured using anthrone colorimetry with a UV–visible spectrometer at a wavelength of 620 nm. The total free amino acid was quantified by ninhydrin colorimetry and detected at 570 nm. Vitamin C was measured by the 2,6-dichloro-indophenoltitration method and detected at 525 nm. Chlorophyll was determined by ethanol extraction. Every treatment group contained three biological replicates.
2.5 Determination of Antioxidase Activity and Antioxidants
Referring to the method of Zhu et al. [23], plant enzymes were extracted from pakchoi shoots after treatments with Se and Hg to analyze antioxidant enzyme activities. Specifically, approximately 0.1 g of frozen shoots were ground into powder under ice bath conditions and then added to 0.9 mL of phosphate buffered saline (PBS) (0.1 M, pH 7.4) to make a homogenate. The homogenate was centrifuged (4000×g, 10 min) and the final supernatant was used for the determination of peroxidase (POD), superoxide dismutase (SOD), catalase (CAT), malondialdehyde (MDA), and proline (PRO) according to the instructions of the respective assay kits (A084-3, A001-1, A007-1, A003-1, A107-1, Nanjing Jiancheng Bioengineering Institute, China).
Data were analyzed using SPSS22 (SPSS Inc., Chicago, IL, USA) by one-way ANOVA. All treatments’ data are expressed as the mean values representing three biological triplicates ± standard errors. Comparisons between multiple treatment groups were performed by Duncan’s multiple significant difference test at p < 0.05.
3.1 Changes in Biomass and Cell Morphology of Pakchoi Treated with Hg and Se
Pakchoi growth was affected by exogenous Na2SeO3 or HgCl2 treatments (Fig. 1 and Table 1). Under HgCl2 stress, the aboveground and underground weights of pakchoi were 4.06 and 0.57 g, respectively, which were only 25% and 50% of the control (CK). Likewise, plant height and root length were measured at 7.29 and 8.86 cm, which were 55% and 65% of the CK. Simultaneously, pakchoi growth inhibition and root tip necrosis were observed. On the contrary, the pakchoi growth did not differ significantly under Na2SeO3 treatment. The weight of the aboveground parts and root length decreased, and the average plant height increased slightly, but none of them showed significant differences compared with the CK (Table 1). In the HgCl2 + Na2SeO3 treated group, the average aboveground, root weights, and root length were 6.40 g, 0.70 g, and 11.83 cm, respectively, which were 60%, 23%, and 34% higher than those in HgCl2, but still less than CK. Therefore, it was hypothesized that Se might be responsible for alleviating the growth stress of Hg on pakchoi.
Figure 1: Growth phenotypes of pakchoi under different mercury or selenium treatments. CK, no treatment control; Hg, HgCl2 40 mg L–1 treatment; Se, Na2SeO3 0.2 mg L–1 treatment; Hg + Se, HgCl2 40 mg L–1 + Na2SeO3 0.2 mg L–1
3.2 Changes in Physiological Indicators of Pakchoi under Hg and Se Treatments
To explore the effect of Se and Hg on pakchoi’s nutrient parameters, the contents of soluble protein, soluble sugar, vitamin C, free amino acids, and chlorophyll were measured (Table 2). Under HgCl2 treatment, all detected parameters significantly decreased. The soluble protein, vitamin C, and chlorophyll contents decreased to about half of CK, while the levels of soluble sugars and free amino acids reached about 85% of CK, indicating that Hg caused substantial damage to the growth of pakchoi. Treatment with Na2SeO3 alone obviously increased the soluble sugar content, while the levels of soluble protein, vitamin C, free amino acid, and chlorophyll did not change significantly. In the HgCl2 + Na2SeO3 treatment group, the contents of soluble protein, soluble sugar, and chlorophyll increased significantly compared with the HgCl2 treatment, reaching 81%, 94%, and 78% of that in CK, respectively.
3.3 Effect of Hg and Se Treatments on the Total Hg and Se Contents of Pakchoi
The Se content of pakchoi was affected by different treatments (Fig. 2a). Among them, the Hg treatment led to a decrease in the Se content of pakchoi, which remained at 0.14 μg kg−1. The highest Se content was found in the group with Na2SeO3 treatment alone, which was two-fold higher than that in the CK. The Se content under the co-treatment with HgCl2 + Na2SeO3 reached 0.29 μg kg−1, significantly lower than that in the Se treatment but also significantly higher than that of CK and Hg treatments.
Figure 2: Changes of total Se and total Hg contents in pakchoi under different treatments with Na2SeO3 or HgCl2. (a) Selenium contents in different treatments. (b) Mercury contents in different treatments. Different lowercase letters indicate significant (p < 0.05) differences among treatments
The Hg content of pakchoi varied significantly under different treatments (Fig. 2b). In the HgCl2 treatment, Hg content reached 42 μg kg−1, which was fourteen-fold higher than that in the control. By comparison, no change occurred in Hg content with the Se-only treatment. In the HgCl2 + Na2SeO3 group, Hg content was measured at 30.4 μg kg−1, which was ten-fold higher than that of the control group. However, Hg content was still significantly lower compared to that of the group treated with HgCl2 alone. Accordingly, the decrease in Hg content in pakchoi was assumed to be due to Se.
3.4 Effect of Hg and Se Treatments on MDA and PRO in Pakchoi
Plants accumulate PRO in response to abiotic stresses. As shown in Fig. 3a, Hg treatment dramatically increased PRO levels in pakchoi to resist stress. In the Se-treated group alone, there was no significant change in PRO content. However, treating pakchoi with both Se and Hg resulted in a significant 72% increase in PRO content compared to Hg-treated pakchoi. It could be seen that Se began to promote PRO production in large quantities to protect pakchoi from Hg stress.
Figure 3: Contents of PRO and MDA in pakchoi under different treatments with Na2SeO3 or HgCl2. (a) PRO contents in different treatments. (b) MDA contents in different treatments. Different lowercase letters indicate significant (p < 0.05) differences among treatments
MDA is an end product of the lipid peroxidation reaction in cells, which can cause significant damage to cell membranes. As shown in Fig. 3b, there was a dramatic increase in MDA content under mercury stress, reaching almost twice that of CK. However, the addition of selenium reduced the MDA content by 14% and alleviated the damage in pakchoi. Treatment with selenium alone resulted in a substantial reduction in MDA content, which was found to be beneficial for the growth of pakchoi.
3.5 Effect of Hg and Se Treatments on POD, CAT, and SOD Activities in Pakchoi
Antioxidant enzymes played an important role in plants subjected to abiotic stresses, they showed very different changes in the experiment (Fig. 4). In the Hg-treated group, POD activity was significantly increased to 270% of that in CK. However, it was reduced to 67% in the Se + Hg treatment group compared to the Hg-treated group. In the Se + Hg group, the CAT activity was about 70% of that of CK, but much higher than that of the Hg alone group, which was only 18% of that of the control. In addition, SOD activity in the Hg-treated and Se + Hg treatment groups increased by approximately 12% for CK, and there was no significant difference between these two groups. In Se treatment alone, the contents of all three antioxidant enzymes did not change significantly, demonstrating that Se does not increase the activity of antioxidant enzymes in pakchoi in the absence of Hg stress.
Figure 4: Effect of different treatments with Na2SeO3 or HgCl2 on the activities POD, CAT, and SOD in pakchoi. (a) POD enzyme activities in different treatments. (b) CAT enzyme activities in different treatments. (c) SOD enzyme activities in different treatments. Different lowercase letters indicate significant (p < 0.05) differences among treatments
Mercury (Hg) is a very harmful heavy metal, and high concentrations of it can cause plant-cell structure abnormalities and malfunction, thereby inhibiting plant growth. Selenium (Se) is an essential trace element for the human body and has various effects, such as improving the immune system, preventing cancer, and regulating blood pressure balance. Although selenium is not an essential trace element for plant growth, applying appropriate concentrations of selenium can promote plant growth, thereby improving plant yield and quality. Studies have shown that Se can improve plant resistance, alleviate heavy-metal stress in plants, and reduce their uptake of heavy metals, effectively alleviating heavy-metal pollution in plants [24]. Suitable Se concentrations promote maize growth and significantly increase plant biomass [25]. Conversely, high Se concentrations inhibit maize growth, reduce plant dry-matter accumulation, and decrease seed quality. The plant height, stem thickness, root length, and branch number of Perilla frutescens initially increase and then decrease with increased selenium concentration [26]. The total protein and vigor index of Suaeda salsa under mercury stress conditions increases after Se application [27], indicating that suitable Se concentrations can improve the stress caused by heavy metals such as Hg on plant growth. Selenium can combine with elemental mercury to form insoluble Se-Hg complexes around the plant root system, which promotes the production of phytochelatins (PCs) and thus reduces mercury accumulation in the plant [28,29]. The use of hydroponics in this experiment may have reduced the amount of selenium-mercury complexes during the washing process after collection of the material, resulting in lower measurements. Similar to the above studies, our results revealed that the Na2SeO3 + HgCl2 treatment reduced growth inhibition and increased the biomass of pakchoi compared with HgCl2 treatment alone. This finding indicated that exogenous Se effectively alleviated the stress effect of Hg on pakchoi growth (Fig. 5).
Figure 5: Possible mechanisms of selenium attenuation of mercury stress in pakchoi
Applying Se at appropriate concentrations can improve the nutritional quality of pakchoi. A study on grapes has revealed that the foliar application of exogenous Se significantly improves the fruit quality in soluble solids, total sugars, sugar-to-acid ratio, vitamin C, tannins, and anthocyanins, as well as increases the yield of grapes in three types of grapes [30]. Foliar spraying of sodium selenite also improves intrinsic qualities, such as vitamin C, carotenoid, soluble protein, soluble sugar, and total flavonoids in broccoli sprouts [31]. Vitamin C itself is an antioxidant and is more sensitive to heavy metal stress [32]. Selenium acts more as a protector in antagonizing heavy metal stress, and selenium treatment alone had little effect on changes in vitamin C content [33]. Soluble sugars are involved in physiological activities in plants as osmotic pressure compounds. Under mercury stress, plant cells are damaged, and soluble sugar content is reduced [34]. At the same time, exogenous selenium treatment increased soluble sugar content [35]. This increase in osmotic substances may be a defense mechanism for plants to mitigate the damage caused by exogenous elements through osmoregulation [36]. Selenium has an antagonistic effect on mercury, thus gradually restoring the soluble sugar content to the plant’s own level. The role of selenium in mitigating changes in soluble protein and free amino acid content in Hg-stressed plants remains to be investigated. In the present work, the application of Se was effective in increasing the contents of soluble protein and soluble sugar in Hg-stressed pakchoi, consistent with the results of previous studies on other horticultural plants. This finding indicated that Se treatment was effective in increasing selenium content and improving the multiple nutritional qualities of pakchoi (Fig. 5).
Chloroplasts are the main site of photosynthesis in plants, and chloroplast content can affect the strength of their photosynthesis. Studies have shown that appropriate concentrations of Se can, to some extent, mitigate the structural damage and functional impairment of plant cells caused by heavy-metal stress and thus restore plant-chloroplast damage caused by heavy-metal stress. The mitigation is done by increasing chlorophyll content, reconstructing chloroplast ultrastructure, and reorganizing cystoid and stromal structures [37], as well as increasing chloroplast size, unsaturated fatty acidity, and cell-membrane fluidity [38]. The reactivation of membrane enzymes and the restoration of important metabolite transport can alleviate heavy metal stress in plants [39]. Proline (PRO) is involved in the maintenance of osmotic balance and the stabilization of cell membrane structure and function. The addition of Se under cadmium stress increases PRO metabolism and thus mitigates the effects of cadmium stress on wheat photosynthesis [40]. Similar studies have been reported in rice, where low doses of Se solutions increase photosynthesis in rice plants [41]. Se application to sorghum seedlings significantly increases the photosynthesis rate, stomatal conductance, and transpiration rate [42,43]. The repair of plant cell structures including chloroplasts is primarily regulated indirectly by Se, which promotes the uptake of essential trace elements such as Fe, Cu, Zn, and P. This phenomenon indirectly promotes the synthesis of chlorophyllate reductase and the repair of plant bilayer structures, thereby indirectly reducing heavy-metal stress in plants [44,45]. The current study showed that the chloroplast content under Hg stress increased after Se application, which showed that selenium mitigates the damage caused by Hg to the intracellular chloroplasts of pakchoi. PRO, as one of the regulators of normal plant growth, also varies within the expected range. Therefore, an appropriate amount of Se can exert a protective effect on pakchoi under Hg stress (Fig. 5).
Similar to other abiotic stresses, mercury stress decreases the antioxidant capacity of plants, leading to elevated levels of reactive oxygen radicals (ROS), which cause lipid peroxidation damage and the production of MDA in cells [46,47]. Selenium is an essential micronutrient for antioxidant and metabolic reactions in plants. It can directly or indirectly scavenge ROS, reducing MDA content and protecting plant safety. Experimental studies on wheat [48], rice [49], tomato [50], and canola [51] have shown that Se enhances their antioxidant capacity and stress resistance, ensuring normal growth. In the current research, POD activities in pakchoi significantly increased to resist oxidative damage caused by mercury stress. However, high concentrations of HgCl2 lead to a drastic reduction in CAT activity. After the appropriate increase of exogenous Se, POD activities and MDA content in pakchoi significantly reduced, and CAT content was significantly elevated close to the control. These results indicate that selenium incorporation effectively alleviated the oxidative stress caused by mercury (Fig. 5).
Pakchoi is a prevalent edible vegetable in China. However, it is essential not to ignore the problem of occasional mercury contamination. To find out the way to alleviate the mercury stress in pakchoi, we took the treatment of pakchoi with 0.2 mg L−1 Na2SeO3, 40 mg L−1 HgCl2, and 40 mg L−1 HgCl2 + 0.2 mg L−1 Na2SeO3 combined treatment. The plant weight, height, root length, and physiological indexes such as soluble protein, soluble sugar, vitamin C, free amino acids, chlorophyll, and antioxidant enzymes were detected. We found that pakchoi biomass and height were obviously reduced under mercury stress, severe damage to the root tip, and significant decreases in all nutrient indices. Meanwhile, POD activity and MDA content significantly increased, while the content of CAT dramatically decreased. By contrast, in the Se + Hg co-treated group, pakchoi growth inhibition was alleviated, biomass was enhanced, pakchoi aboveground and root elongated, and the content of proline and physiological indices such as soluble protein and soluble sugar increased significantly. Meanwhile, the MDA content and POD activity also decreased significantly, while CAT activity was significantly elevated close to the control. The results indicate that exogenous selenium supplementation may be an effective method to reduce the accumulation of mercury in pakchoi. Our results have important implications for the management of mercury pollution and provide some technical guidance for the technology of selenium-enriched vegetable cultivation.
Acknowledgement: Not applicable.
Funding Statement: This research was funded by the Key Program of Hubei Province, Grant Number 2023BBA043.
Author Contributions: Conceptualization, C.Q. and F.X.; methodology, C.Q.; validation, Q.C., L.J. and X.Y.; formal analysis, Q.C.; investigation, X.Y.; resources, S.R.; data curation, W.Z.; writing—original draft preparation, C.Q.; writing—review and editing, L.J. and F.X.; project administration, F.X. All authors have read and agreed to the published version of the manuscript.
Availability of Data and Materials: All data are available in this article.
Ethics Approval: Not applicable.
Conflicts of Interest: The authors declare that they have no conflicts of interest to report regarding the present study.
References
1. Méplan C, Hesketh J. The influence of selenium and selenoprotein gene variants on colorectal cancer risk. Mutagenesis. 2012;27(2):177–86. doi:10.1093/mutage/ger058 [Google Scholar] [PubMed] [CrossRef]
2. Gao G, Yan L, Tong K, Yu H, Lu M, Wang L, et al. The potential and prospects of modified biochar for comprehensive management of salt-affected soils and plants: a critical review. Sci Total Environ. 2023;912:169618. doi:10.1016/j.scitotenv.2023.169618 [Google Scholar] [PubMed] [CrossRef]
3. Gustin MS, Bank MS, Bishop K, Bowman K, Branfireun B, Chételat J, et al. Mercury biogeochemical cycling: a synthesis of recent scientific advances. Sci Total Environ. 2020;737(20):139619. doi:10.1016/j.scitotenv.2020.139619 [Google Scholar] [PubMed] [CrossRef]
4. Qiu G, Feng X, Jiang G. Synthesis of current data for Hg in areas of geologic resource extraction contamination and aquatic systems in China. Sci Total Environ. 2012;421–422(5):59–72. doi:10.1016/j.scitotenv.2011.09.024 [Google Scholar] [PubMed] [CrossRef]
5. Riyazuddin R, Nisha N, Ejaz B, Khan MIR, Kumar M, Ramteke PW, et al. A comprehensive review on the heavy metal toxicity and sequestration in plants. Biomol. 2021;12(1):43. doi:10.3390/biom12010043 [Google Scholar] [PubMed] [CrossRef]
6. Yang L, Zhang Y, Wang F, Luo Z, Guo S, Strähle U. Toxicity of mercury: molecular evidence. Chemosphere. 2020;245(4):125586. doi:10.1016/j.chemosphere.2019.125586 [Google Scholar] [PubMed] [CrossRef]
7. Rayman MP. Selenium and human health. Lancet. 2012;379(11):1256–68. doi:10.1016/S0140-6736(11)61452-9 [Google Scholar] [PubMed] [CrossRef]
8. Avery JC, Hoffmann PR. Selenium, selenoproteins, and immunity. Nutr. 2018;10(9):1203. doi:10.3390/nu10091203 [Google Scholar] [PubMed] [CrossRef]
9. Xie M, Sun X, Li P, Shen X, Fang Y. Selenium in cereals: insight into species of the element from total amount. Compr Rev Food Sci Food Saf. 2021;20(3):2914–40. doi:10.1111/1541-4337.12748 [Google Scholar] [PubMed] [CrossRef]
10. Hasanuzzaman M, Nahar K, García-Caparrós P, Parvin K, Zulfiqar F, Ahmed N, et al. Selenium supplementation and crop plant tolerance to metal/metalloid toxicity. Front Plant Sci. 2021;12:792770. doi:10.3389/fpls.2021.792770 [Google Scholar] [PubMed] [CrossRef]
11. Trippe RC, Pilon-Smits EAH. Selenium transport and metabolism in plants: phytoremediation and biofortification implications. J Hazard Mater. 2021;404(285):124178. doi:10.1016/j.jhazmat.2020.124178 [Google Scholar] [PubMed] [CrossRef]
12. Wang Z, Huang W, Pang F. Selenium in soil-plant-microbe: a review. Bull Environ Contam Toxicol. 2022;108(2):167–81. doi:10.1007/s00128-021-03386-2 [Google Scholar] [PubMed] [CrossRef]
13. Zhang H, Feng X, Zhu J, Sapkota A, Meng B, Yao H, et al. Selenium in soil inhibits mercury uptake and translocation in rice (Oryza sativa L.). Environ Sci Technol. 2012;46(18):10040–6. doi:10.1021/es302245r [Google Scholar] [PubMed] [CrossRef]
14. Zhao J, Gao Y, Li YF, Hu Y, Peng X, Dong Y, et al. Selenium inhibits the phytotoxicity of mercury in garlic (Allium sativum). Environ Res. 2013;125:75–81. doi:10.1016/j.envres.2013.01.010 [Google Scholar] [PubMed] [CrossRef]
15. Liu X, Duan B, Xia Q, Jiao W, Guo Z, Hu C, et al. Effects of sulfur on transformation of selenium in soil and uptake of selenium in rape. Huan Jing Ke Xue. 2014;35:3564–71 [Google Scholar] [PubMed]
16. Tran TAT, Zhou F, Yang W, Wang M, Dinh QT, Wang D, et al. Detoxification of mercury in soil by selenite and related mechanisms. Ecotoxicol Environ Saf. 2018;159:77–84. doi:10.1016/j.ecoenv.2018.04.029 [Google Scholar] [PubMed] [CrossRef]
17. Boldrin PF, de Figueiredo MA, Yang Y, Luo H, Giri S, Hart JJ, et al. Selenium promotes sulfur accumulation and plant growth in wheat (Triticum aestivum). Physiol Plant. 2016;158(1):80–91. doi:10.1111/ppl.12465 [Google Scholar] [PubMed] [CrossRef]
18. Feng K, Kan X, Liu Q, Yan Y, Sun N, Yang Z, et al. Metabolomics analysis reveals metabolites and metabolic pathways involved in the growth and quality of water dropwort [Oenanthe javanica (Blume) DC.] under Nutrient solution culture. Plants. 2023;12(7):1459. doi:10.3390/plants12071459 [Google Scholar] [PubMed] [CrossRef]
19. Zuo TT, Jin HY, Zhang L, Liu YL, Nie J, Chen BL, et al. Innovative health risk assessment of heavy metals in Chinese herbal medicines based on extensive data. Pharmacol Res. 2020;159(6):104987. doi:10.1016/j.phrs.2020.104987 [Google Scholar] [PubMed] [CrossRef]
20. Gui JY, Rao S, Gou Y, Xu F, Cheng S. Comparative study of the effects of selenium yeast and sodium selenite on selenium content and nutrient quality in broccoli florets (Brassica oleracea L. var. italica). J Sci Food Agric. 2022;102(4):1707–18. doi:10.1002/jsfa.11511 [Google Scholar] [PubMed] [CrossRef]
21. Deng X, Li R, Deng S. Determination of the total content of arsenic, antimony, selenium and mercury in chinese herbal food by chemical vapor generation-four-channel non-dispersive atomic fluorescence spectrometry. J Fluoresc. 2020;30(4):949–54. doi:10.1007/s10895-020-02569-0 [Google Scholar] [PubMed] [CrossRef]
22. Rao S, Yu T, Cong X, Xu F, Lai X, Zhang W, et al. Integration analysis of PacBio SMRT- and Illumina RNA-seq reveals candidate genes and pathway involved in selenium metabolism in hyperaccumulator Cardamine violifolia. BMC Plant Biol. 2020;20(1):492. doi:10.1186/s12870-020-02694-9 [Google Scholar] [PubMed] [CrossRef]
23. Zhu Y, Dong Y, Zhu N, Jin H. Foliar application of biosynthetic nano-selenium alleviates the toxicity of Cd, Pb, and Hg in Brassica chinensis by inhibiting heavy metal adsorption and improving antioxidant system in plant. Ecotoxicol Environ Saf. 2022;240(1):113681. doi:10.1016/j.ecoenv.2022.113681 [Google Scholar] [PubMed] [CrossRef]
24. Hossain A, Skalicky M, Brestic M, Maitra S, Sarkar S, Ahmad Z, et al. Selenium biofortification: roles, mechanisms, responses and prospects. Mol. 2021;26(4):881. doi:10.3390/molecules26040881 [Google Scholar] [PubMed] [CrossRef]
25. Naseem M, Anwar-ul-Haq M, Wang X, Farooq N, Awais M, Sattar H, et al. Influence of selenium on growth, physiology, and antioxidant responses in maize varies in a dose-dependent manner. J Food Qual. 2021;2021:e6642018. doi:10.1155/2021/6642018. [Google Scholar] [CrossRef]
26. Liao R. Effects of abscisic acid on growth and selenium uptake in medicinal plant Perilla frutescens. PLoS One. 2022;17(10):e0275813. doi:10.1371/journal.pone.0275813 [Google Scholar] [PubMed] [CrossRef]
27. Liu X, Lai Y, Sun H, Wang Y, Zou N. The interactive effects of mercury and selenium on metabolic profiles, gene expression and antioxidant enzymes in halophyte Suaeda salsa. Environ Toxicol. 2016;31(4):440–51. doi:10.1002/tox.22057 [Google Scholar] [PubMed] [CrossRef]
28. Rayman MP. Selenium intake, status, and health: a complex relationship. Hormones. 2020;19(1):9–14. doi:10.1007/s42000-019-00125-5 [Google Scholar] [PubMed] [CrossRef]
29. Tran TAT, Dinh QT, Zhou F, Zhai H, Xue M, Du Z, et al. Mechanisms underlying mercury detoxification in soil-plant systems after selenium application: a review. Environ Sci Pollut Res. 2021;28(34):46852–76. doi:10.1007/s11356-021-15048-1 [Google Scholar] [PubMed] [CrossRef]
30. Yin N, Mu L, Liang YL, Hao WL, Yin HF, Zhu SM, et al. Effects of foliar selenium fertilizer on fruit yield, quality and selenium content of three varieties of Vitis vinifera. Ying Yong Sheng Tai Xue Bao = J Appl Ecology. 2020;31:953–8. doi:10.13287/j.1001-9332.202003.007 [Google Scholar] [PubMed] [CrossRef]
31. He R, Gao M, Shi R, Song S, Zhang Y, Su W, et al. The combination of selenium and LED light quality affects growth and nutritional properties of broccoli sprouts. Mol. 2020;25(20):4788. doi:10.3390/molecules25204788 [Google Scholar] [PubMed] [CrossRef]
32. Valko M, Morris H, Cronin M. Metals, toxicity and oxidative stress. Curr Med Chem. 2005;12(10):1161–208. doi:10.2174/0929867053764635 [Google Scholar] [PubMed] [CrossRef]
33. Moniruzzaman M, Lee S, Park Y, Min T, Bai SC. Evaluation of dietary selenium, vitamin C and E as the multi-antioxidants on the methylmercury intoxicated mice based on mercury bioaccumulation, antioxidant enzyme activity, lipid peroxidation and mitochondrial oxidative stress. Chemosphere. 2021;6(273):129673. doi:10.1016/j.chemosphere.2021.129673 [Google Scholar] [PubMed] [CrossRef]
34. Zhang T, Lu Q, Su C, Yang Y, Hu D, Xu Q. Mercury induced oxidative stress, DNA damage, and activation of antioxidative system and Hsp70 induction in duckweed (Lemna minor). Ecotoxicol Environ Saf. 2017;143(1):46–56. doi:10.1016/j.ecoenv.2017.04.058 [Google Scholar] [PubMed] [CrossRef]
35. Marja T, Helinä H, Mervi MS. Effects of selenium treatments on potato (Solanum tuberosum L.) growth and concentrations of soluble sugars and starch. J Agric Food Chem. 2004;52(17):5378–82. doi:10.1021/jf040077x [Google Scholar] [PubMed] [CrossRef]
36. Khalofah A, Migdadi H, El-Harty E. Antioxidant enzymatic activities and growth response of Quinoa (Chenopodium quinoa Willd) to exogenous selenium application. Plants. 2021;10(4):719. doi:10.3390/plants10040719 [Google Scholar] [PubMed] [CrossRef]
37. Ismael MA, Elyamine AM, Moussa MG, Cai M, Zhao X, Hu C. Cadmium in plants: uptake, toxicity, and its interactions with selenium fertilizers. Metallomics. 2019;11(2):255–77. doi:10.1039/C8MT00247A [Google Scholar] [PubMed] [CrossRef]
38. Filek M, Gzyl-Malcher B, Zembala M, Bednarska E, Laggner P, Kriechbaum M. Effect of selenium on characteristics of rape chloroplasts modified by cadmium. J Plant Physiol. 2010;167(1):28–33. doi:10.1016/j.jplph.2009.07.003 [Google Scholar] [PubMed] [CrossRef]
39. Gupta M, Gupta S. An overview of selenium uptake, metabolism, and toxicity in plants. Front Plant Sci. 2016;7:2074. doi:10.3389/fpls.2016.02074 [Google Scholar] [PubMed] [CrossRef]
40. Khan MIR, Nazir F, Asgher M, Per TS, Khan NA. Selenium and sulfur influence ethylene formation and alleviate cadmium-induced oxidative stress by improving proline and glutathione production in wheat. J Plant Physiol. 2015;173:9–18. doi:10.1016/j.jplph.2014.09.011 [Google Scholar] [PubMed] [CrossRef]
41. Wang C, Cheng T, Liu H, Zhou F, Zhang J, Zhang M, et al. Nano-selenium controlled cadmium accumulation and improved photosynthesis in indica rice cultivated in lead and cadmium combined paddy soils. J Environ Sci. 2021;103(4):336–46. doi:10.1016/j.jes.2020.11.005 [Google Scholar] [PubMed] [CrossRef]
42. Djanaguiraman M, Prasad PVV, Seppanen M. Selenium protects sorghum leaves from oxidative damage under high temperature stress by enhancing antioxidant defense system. Plant Physiol Biochem. 2010;48(12):999–1007. doi:10.1016/j.plaphy.2010.09.009 [Google Scholar] [PubMed] [CrossRef]
43. Liu H, Xiao C, Qiu T, Deng J, Cheng H, Cong X, et al. Selenium regulates antioxidant, photosynthesis, and cell permeability in plants under various abiotic stresses: a review. Plants. 2023;12(1):44. doi:10.3390/plants12010044 [Google Scholar] [PubMed] [CrossRef]
44. Li YY, Yu SH, Zhou XB. Effects of phosphorus on absorption and transport of selenium in rice seedlings. Environ Sci Pollut Res. 2019;26(14):13755–61. doi:10.1007/s11356-018-2690-y [Google Scholar] [PubMed] [CrossRef]
45. Mozafariyan M, Shekari L, Hawrylak-Nowak B, Kamelmanesh MM. Protective role of selenium on pepper exposed to cadmium stress during reproductive stage. Biol Trace Elem Res. 2014;160(1):97–107. doi:10.1007/s12011-014-0028-2 [Google Scholar] [PubMed] [CrossRef]
46. Yan L, Riaz M, Li S, Cheng J, Jiang C. Harnessing the power of exogenous factors to enhance plant resistance to aluminum toxicity; a critical review. Plant Physiol Biochem. 2023;203(12):108064. doi:10.1016/j.plaphy.2023.108064 [Google Scholar] [PubMed] [CrossRef]
47. Yu Y, Yuan S, Zhuang J, Wan Y, Wang Q, Zhang J, et al. Effect of selenium on the uptake kinetics and accumulation of and oxidative stress induced by cadmium in Brassica chinensis. Ecotoxicol Environ Saf. 2018;162(11):571–80. doi:10.1016/j.ecoenv.2018.07.041 [Google Scholar] [PubMed] [CrossRef]
48. Wu C, Dun Y, Zhang Z, Li M, Wu G. Foliar application of selenium and zinc to alleviate wheat (Triticum aestivum L.) cadmium toxicity and uptake from cadmium-contaminated soil. Ecotoxicol Environ Saf. 2020;190(3):110091. doi:10.1016/j.ecoenv.2019.110091 [Google Scholar] [PubMed] [CrossRef]
49. Huang QQ, Liu YY, Qin X, Zhao LJ, Liang XF, Xu YM. Selenite mitigates cadmium-induced oxidative stress and affects Cd uptake in rice seedlings under different water management systems. Ecotoxicol Environ Saf. 2019;168:486–94. doi:10.1016/j.ecoenv.2018.10.078 [Google Scholar] [PubMed] [CrossRef]
50. Alyemeni MN, Ahanger MA, Wijaya L, Alam P, Bhardwaj R, Ahmad P. Selenium mitigates cadmium-induced oxidative stress in tomato (Solanum lycopersicum L.) plants by modulating chlorophyll fluorescence, osmolyte accumulation, and antioxidant system. Protoplasma. 2018;255(2):459–69. doi:10.1007/s00709-017-1162-4 [Google Scholar] [PubMed] [CrossRef]
51. Ashraf MA, Rasheed R, Hussain I, Hafeez A, Adrees M, Rehman MZU, et al. Effect of different seed priming agents on chromium accumulation, oxidative defense, glyoxalase system and mineral nutrition in canola (Brassica napus L.) cultivars. Environ Pollut. 2022;309(9):119769. doi:10.1016/j.envpol.2022.119769 [Google Scholar] [PubMed] [CrossRef]
Cite This Article
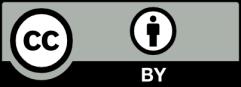
This work is licensed under a Creative Commons Attribution 4.0 International License , which permits unrestricted use, distribution, and reproduction in any medium, provided the original work is properly cited.