Open Access
ARTICLE
Effects of Different Light Qualities on the Growth Characteristics of Populus trinervis
1 Key Laboratory for Forest Genetics and Tree Improvement and Propagation in Universities of Yunnan Province, Southwest Forestry University, Kunming, China
2 Key Laboratory of Biodiversity Conservation in Southwest China, State Forestry Administration, Southwest Forestry University, Kunming, China
3 Key Laboratory for Forestry Resources Conservation and Utilization in the Southwest Mountains of China, Ministry of Education, Southwest Forestry University, Kunming, China
* Corresponding Author: Chengzhong He. Email:
(This article belongs to the Special Issue: Abiotic Stress Impacts on Plant Physiology and Their Alleviation)
Phyton-International Journal of Experimental Botany 2024, 93(5), 1043-1056. https://doi.org/10.32604/phyton.2024.050637
Received 12 February 2024; Accepted 11 April 2024; Issue published 28 May 2024
Abstract
Populus trinervis is native to China and plays an irreplaceable role in maintaining the ecological balance of boreal and temperate forests. P. trinervis mainly grows in high-altitude areas. At present, there are limited studies on the response of P. trinervis to different light qualities, so it is necessary to investigate the photosynthetic physiological changes of P. trinervis in different light environments. In our study, P. trinervis was grown for 8 months under light filtered by three different colored films. The three treatments were blue film, green film, and white plastic film. The effects of blue (B), green (G), and white (W) light on photosynthetic pigment content, absolute growth, photosynthetic parameters, soluble sugar content, and chlorophyll fluorescence parameters were studied, respectively. Compared to the W treatment, the chlorophyll a and b, carotenoids, total chlorophyll content (a+b), absolute growth of seedling height, net photosynthetic rate (P), water use efficiency (WUE), total soluble sugars, sucrose, and nonphotochemical quenching (NPQ) of P. trinervis were significantly increased under B treatment. Meanwhile, chlorophyll a and b, carotenoids, total chlorophyll (a+b), transpiration rate (Tr), intercellular CO concentration (C), stomatal conductance (g), absolute growth of seedling height and leaf length, reducing sugar, total soluble sugar content, and NPQ were significantly increased under G treatment. The results showed that the absolute growth and chlorophyll content of P. trinervis were increased under B light, while the sugar and photosynthetic parameters were increased under G light. Additional studies may look into how B light impacts absolute growth and promotional mechanisms, as well as how G light affects the accumulation of sugar levels.Keywords
Photosynthetic organisms rely on light capture and the conversion of their energy into stored photosynthetic products, light is not only an important environmental signal that affects plant growth but also provides energy for their growth and development [1]. In the first step of photosynthesis, the plant’s photosynthetic pigments intercept the light signal and transfer it to the reaction center of the two light systems, Photosystems II and I (PSII, PSI), which constitute the photosynthetic unit. The quality and wavelength of light play a crucial role in influencing the photosynthetic process in plants [2,3]. Several published studies have revealed that light quality affects seed germination [4], circadian rhythm [5], axillary bud growth [6], morpho-physiological [7], secondary metabolite synthesis network [8], the ultrastructure of chloroplasts and anatomical structure of leaves [9], disease resistance [10], anthocyanin biosynthesis [11] and gene expression [12]. Studies conducted by Eze Pojmann-Ezeonyilo have demonstrated that the quality of light can impact pest biology, thereby suggesting that light quality could be a valuable tool for pest management [13]. Different light qualities can cause different physiological and metabolic responses in plants, leading to different growth states of plants. Blue light increases the photosynthetic rate and promotes stomatal opening [14]. The study of [15] has revealed that green sensors can regulate the growth of plants, in addition to red, far red, blue, and ultraviolet (UV) sensors. In this article, blue, green, white, red, and far red are represented by B, G, W, R, and FR, respectively.
The supplemental lighting and an increase in the photosynthetic daily light integral are often used to improve harvest in the process of agricultural production. Photobiological studies have revealed that the use of blue-violet light with a wavelength of 400–480 nm is conducive to improving the efficiency of photosynthesis and the protein content of crops. Exposure to B or R light, or a combination of both, enhances the expression of potassium transporter genes, leading to increased potassium uptake and accumulation. This results in enhanced lycopene synthesis and improved color of tomato fruits [16]. Exposure to B or R light, or a combination of both, enhances the expression of K transporter genes, leading to increased potassium uptake and accumulation. This results in enhanced lycopene synthesis and improved color of tomato fruits. Several methods have been adopted to mitigate the reduced yield caused by different light conditions, including breeding varieties with altered developmental responses. Compared with these methods, light-emitting diode (LED) lighting is increasingly being used for greenhouse crop cultivation because of its long service life, low energy consumption, and low heat output [17,18]. The role of auxiliary lighting has been demonstrated in numerous studies of watermelon, chrysanthemum, mustard, roses, and Brassica rapa ssp. Chinensis [19–23]. Changes in the ratio of R to B light in irradiated lettuce can increase the contents of sugars and phenols in its leaves and increase its yield [24]. Yu et al. [25] use m-MeOPTCDA converters to convert yellow-green light (510–580 nm) of sunlight into blue-violet light and red-orange light that can be absorbed by plant leaves to improve the photosynthetic efficiency of crops. Adding R light to lettuce and other leafy plants can control their plant structure. The addition of R light can reduce leaf decline and internode elongation, further, slow down plant aging and increase plant yield [26]. Prolonged exposure to certain plants, such as tomatoes and bell peppers, can improve fruit quality and yield [27,28]. At present, the research and application of light-quality control in vegetable cultivation and flower cultivation are more and more extensive, but research on the impact of light quality on tree breeding is scarce. Therefore, it is crucial to investigate how tree seedling growth and photosynthesis respond to different light qualities.
Populus trinervis is native to China and was first found in Sichuan Province. Most of them are found at altitudes between 2100 and 3000 meters. Its leaves are 2.5–5 cm wide and 4–7 cm long [29]. P. trinervis plays an irreplaceable role in the ecological balance of boreal and temperate forests. They can grow in environments such as riparian forests and provide a habitat for wild animals. So, it is very adaptable to the environment. As the altitude increases, UV light enhancement in natural light, the proportion of short wavelengths represented by B light usually has noteworthy effects on plant growth and adaptation. However, there are limited studies on the response of P. trinervis to light quality in high-altitude mountains, the changes in the photosynthetic physiology of P. trinervis under different light-quality environments is not clear, and its adaptability to different light quality is not well understood.
B light, G light, R light, and FR light occupy a large proportion of the relative content of visible light in the middle and high-altitude areas where P. trinervis grows [30]. In the present study, we focused on the influence of B light and G light on the growth of P. trinervis and measured the absolute leaf growth and photosynthetic response parameters of poplar cuttings under different light qualities. The study is expected to provide a theoretical basis and a reference for further exploring the introduction and cultivation of different poplar species in high-altitude areas.
2.1 Plants and Light Treatments
The experiment was conducted in climate chambers at Southwest Forestry University of Yunnan province (25°06′N, 102°76′E) from March to October 2020. P. trinervis plants were collected from different P. trivernis individuals, and maintained in a greenhouse. The 1-year-old trunk with the same growth and no pests and diseases was harvested, and the middle section with full buds was cut to produce cuttings with a length of 25 cm. Cuttings were planted in a 42 cm nursery pot, and the cultivation substrate was rural laterite:peat:humus = 1:2:1. One cutting is inserted into a basin, and 15 cuttings are propagated in each clone, which is placed in the intelligent greenhouse. Three clones were used as three biological replicates. At the end of July, the cuttings with strong and similar growth were selected and transferred to the vault covered by different color films. The arch shed covered with films of different colors was built in an open area and arranged in the east-west direction. The specifications were length * width * height = 3 m * 3 m * 2 m, and the spacing between the arch shed was 2 m, respectively, covered with B, G, and W films. We replace film every 20 d or so, to ensure the stability of light intensity and quality in the arch shed. One arch shed was built for each color film for a treatment. Under each treatment, 3 pots of the same clone cuttings were placed, and 3 replicates were set for each treatment, a total of 9 pots. The related indexes of seedlings were measured after 60 days of growth under different film treatments.
We measured the radiant energy of the spectrum using an HR-550 plant intelligence spectrometer. Related photosynthetic indexes were measured at 20 cm points below the membrane of the vault every 2 h from 8:00 to 18:00. We determined transmission spectrum components like the Photosynthetic Photon Flux Density (PPFD), The PPFD value was used as the representative value of transmission spectrum of different color films. We measured the transmission spectrum values of different color films in one day as shown in the (Fig. 1) below. The average values of the transmission spectra under B, G, and W films are 406.54, 920.37, and 1030.30, respectively.
Figure 1: Diurnal variation curves of photosynthetic photon flux density under different treatments. Each treatment’s transmission spectrum was represented by the average of three measurements of PPFD values taken during each period
2.2 Determination of Photosynthetic Pigment
Fresh leaves of P. trinervis under different treatments were collected, respectively (No. 5~7 from the top canopy). Chlorophyll (Chl) a, Chl b, and carotenoids (Car) were extracted with 80% cold acetone. The absorbance A665, A649, and A470 were determined by visible light spectrophotometer at 665, 649, and 470 nm, respectively. For each treatment, the mean value was measured three times, and three biological replicates were set. The concentrations of Chl a, Chl b, and Car are calculated as follows [31]:
Chl a (mg/g−1 FW) = ((13.95A665−6.88A649) * extracting solution)/(fresh weight of sample * 1000)
Chl b (mg/g−1 FW) = ((24.96A649−7.32A665) * extracting solution)/(fresh weight of sample * 1000)
Car (mg/g−1 FW) = ((1000A470+3.27 * (13.95A665−6.88A649) + 104 * (24.96A649−7.32A665)/
229 * extracting solution))/(fresh weight of sample * 1000)
Chl a/b = Chl a/Chl b
Chl a+b = Chl a+Chl b
2.3 Determination of Growth Parameters
In July and September 2020, tape measures and vernier calipers were used to determine the length (seedling height) and the thickness (ground diameter) at the base 2 cm of the main branch of P. trinervis under different treatments. Under each treatment, the average value of three seedlings from each clone was taken as one replicate, and a total of three biological replicates were determined.
2.4 Determination of Sugar Content
Total soluble sugar, reducing sugar, and amylum were prepared by the anthrone-sulfuric acid method and the 3,5-dinitro salicylic acid method, respectively. The absorbance values were measured by a visible light spectrometer, and the calculation of sucrose and amylum contents was done based on the corresponding calculation method [32]. A biological replicate for each treatment was created by measuring each sample three times and calculating the mean value.
Content of reducing sugar (%) = (C * Vt)/(W * Vs) * 100%
Total soluble sugars content (%) = (C * Vt * n)/(W * Vs *1000) * 100%
Amylum content (%) = (C * Vt * 0.9)/(W * Vs *1000) * 100%
Sucrose content (%) = (Total soluble sugars content − content of reducing sugar) * 0.95
In the above formula: C: The glucose content in the tube of the sample measured or calculated from the standard curve (mg); Vt: Total volume of sample extract (mL); Vs: Sample volume (mL); W: Sample dry weight (mg); n: Dilution ratio; 1000: Conversion factor; 0.9: Conversion coefficient of glucose into starch.
2.5 Determination of Photosynthetic Characters
We monitored the determination of photosynthetic characters in mid-September 2020 using the LI-6800 (LI-COR, USA) XT portable photosynthesis system’s quantum sensor. The gas exchange parameters and environmental factors were measured at 8:00~17:00 every 2 h under different treatments. The index mainly includes the net photosynthetic rate (PN), transpiration rate (Tr), stomatal conductance (gs), intercellular CO2 concentration (Ci), water use efficiency (WUE) (WUE = PN/Tr), and Chl fluorescence parameters. Besides, measurements were made between 8:00 to 9:00 h, minimal fluorescence of dark-adapted state (F0), photochemical quenching coefficient (qp), fresh mass (Fm), the quantum efficiency of PSII (PSII), Variable fluorescence (Fv) (Fv = Fm − F0) and nonphotochemical quenching (NPQ) were measured by using LI-6800, and determined in accordance with the law of van Kooten et al. [33] and Maxwell et al. [34]. The quantity of leaves per plant clones was measured across various treatments.
The SPSS package was utilized for conducting a one-way analysis of variance (ANOVA) to analyze all data sets. Means were then compared using Duncan’s new multiple-range test. Graphs were created using Origin 8.5 (OriginLab Corporation, Northampton, MA, USA) and GraphPad Prism 8.0 (GraphPad Software, San Diego, CA, USA).
3.1 Analysis of Transmission Spectral Ratio with Different Colors Films
There are extremely significant differences between the light-quality components under different color films (Fig. 2). The ratio of UV and red light transmittance of the W film is significantly higher than that of B film and G film; The ratio of B light and FR light transmitted by B film is significantly higher than G film and W film; While under G film, the ratio of G light is much greater than that of B film and W film, and the ratio of R light is significantly higher than that of B film. It can be seen that the wavelength of light passing through the B and G films is the same as the B and G light wavelengths. In addition, we found that B film is conducive to the transmission of FR light, and W film is more conducive to the transmission of R light.
Figure 2: The composition and ratio of ultraviolet light (PFD-UV), blue light (PFD-B), red light (PFD-R), green light (PFD-G), and far-red light (PFD-FR) transmitted spectra under different color films. Different-colored columns represent different treatments. The data are shown as means ± standard deviation, with different letters (a, b, c) above the bars denoting statistically significant differences between treatments as determined by Duncan’s new multiple-range test at p < 0.05
Chlorophyll content was significantly affected by the three different light-quality treatments (Fig. 3). In general, Both treatments B and G led to a significant increase in the levels of Chl a, Chl b, and Car in P. trinervis compared to the W treatment. The B treatment showed the highest levels of Chl a, Chl b, and Car contents, which were notably greater compared to the levels observed in the G and W treatments (p < 0.05). The levels of Chl a+b peaked in the B treatment, showing a significant increase compared to both the G and W treatments (p < 0.05). Conversely, the trend observed for Chl a/b is inverted. The results indicated that Chl a/b was highest under the W treatment, significantly surpassing levels in the B and G treatments (p < 0.05).
Figure 3: Effects of different light qualities on the levels of chlorophyll a, chlorophyll b, carotenoids, chlorophyll a/b, and chlorophyll (a+b), in the leaves of P. trinervis. Different-colored columns represent different treatments. The data are shown as means ± standard deviation, with different letters (a, b, c) above the bars denoting statistically significant differences between treatments as determined by Duncan’s new multiple-range test at p < 0.05
The morphological characteristics of P. trinervis showed significant alterations under the influence of the three distinct light-quality conditions (Fig. 4). The G light accelerated significantly seedling height, the G treatment yielded the tallest seedling height, which was significantly greater than the heights observed in the B and W treatments (p < 0.05). The G light also positively promoted the stem diameter, leaf length, and petiole length of P. trinervis. There was a notable increase in the stem diameter with the G treatment as opposed to the B treatment. (p < 0.05) and slightly larger than the W treatment. The leaf length was considerably longer in the G treatment in comparison to the W treatment (p < 0.05) and slightly longer than the B treatment. Notably, the G treatment had the longest petiole length, which was significantly greater than that of the B treatment. (p < 0.05) and slightly longer than the W treatment. Nevertheless, no significant variances were found in leaf width across the three different light-quality treatments.
Figure 4: Effects of different light qualities on the seedling height, stem diameter, leaf length, leaf width, and petiole length of P. trinervis. Different-colored columns represent different treatments. The data are shown as means ± standard deviation, with different letters (a, b, c) above the bars denoting statistically significant differences between treatments as determined by Duncan’s new multiple-range test at p < 0.05
3.4 Photosynthetic Characteristics
The photosynthetic characteristics of P. trinervis leaves showed significant variations among different treatments (Table 1). It was found that the values of Tr, Ci, and gs were the highest in the G treatment, surpassing those in the B and W treatments (p < 0.05). Conversely, the highest PN value was documented in the B treatment, slightly edging out the G treatment and significantly outperforming the W treatment (p < 0.05). Moreover, the highest WUE value was observed in the B treatment, showing a significant difference compared to both the G and W treatments (p < 0.05). These findings suggest that different light-quality have a direct impact on the photosynthetic performance of P. trinervis leaves, with implications for overall the growth and productivity of plants.
3.5 Accumulation of Sugar Content
There were distinct variations in the concentrations of reducing sugar, total soluble sugar, amylum, and sucrose in the leaves of P. trinervis across the B, G, and W treatments (Fig. 5). In the G treatment, the level of reducing sugar was the highest, significantly surpassing that of both the B and W treatments (p < 0.05). Conversely, the B treatment exhibited the highest levels of total soluble sugar and sucrose, which was noticeably higher than both the G and W treatments (p < 0.05). The W treatment recorded the highest level of amylum, significantly exceeding that of both the B and G treatments (p < 0.05).
Figure 5: Effects of different light qualities on the levels of reducing sugar, total soluble sugar, amylum, and sucrose in the leaves of P. trinervis. Different-colored columns represent different treatments. The data are shown as means ± standard deviation, with different letters (a, b, c) above the bars denoting statistically significant differences between treatments as determined by Duncan’s new multiple-range test at p < 0.05
3.6 Chlorophyll Fluorescence Parameters
There were no notable variations in Chl fluorescence parameters (F0, Fv, qp, Fv/Fm, and ΦPSII) under B, G, and W light-quality treatments (Fig. 6). The W treatment exhibited the highest Fm value, slightly surpassing the G treatment and notably exceeding the B treatment (p < 0.05). Conversely, the highest NPQ standard was recorded under G, slightly surpassing the B treatment and significantly exceeding the W treatment (p < 0.05).
Figure 6: Effects of different light qualities on the levels of the chlorophyll fluorescence of P. trinervis. leaves. Minimal fluorescence of dark-adapted state (F0); maximal fluorescence of dark-adapted state (Fm); variable fluorescence (Fv); nonphotochemical quenching (NPQ); photochemical quenching coefficient (qp); maximum quantum yield of PSII photochemistry (Fv/Fm); quantum efficiency of PSII (ΦPSII). Different-colored columns represent different treatments. The data are shown as means ± standard deviation, with different letters (a, b) above the bars denoting statistically significant differences between treatments as determined by Duncan’s new multiple-range test at p < 0.05
Light is a crucial element for the growth of plants photosynthesis, it has a crucial impact on plant morphogenesis and material synthesis [35–37]. A close relationship has been found between photosynthesis and biosynthesis and the accumulation of photosynthetic products [38,39]. Adaptation of leaf photosynthesis to irradiance is influenced by B light for higher plants. B light affects plant growth in several ways [40–42]. In addition, B light photoreceptors mediate the photomorphogenesis response [43,44]. Therefore, reasonable light conditions are essential to optimize the accumulation of phytochemicals.
Pigments such as chlorophyll, carotenoids found in chloroplasts absorb, convert, and transfer light energy, affect photosynthetic response and the stomatal opening, and regulate plant morphogenesis. The quality of light can influence plant photosynthesis by altering the content and composition of chlorophyll. Kai et al. observed that strawberry cultivated under various colored plastic films showed variations in their growth, the order of treatment was as follows, according to the Chl content from highest to lowest: RF > white film > YF > green film > BF [45]. However, research conducted by Kobayashi demonstrated that exposing lettuce leaves to B light led to a higher chlorophyll content in comparison to leaves exposed to R light [46]. A similar finding was reported at Toona sinensis seeding [47]. We found that P. trinervis plants had lower levels of Chl a, Chl b, and total Chl under W light in comparison to B and G light. The results are consistent with those obtained on peas, grapes, and cotton, in which exposure to light B resulted in a rise in the chlorophyll levels of the plants [39,48,49]. Another study revealed that B light still affected Chl biosynthesis in the absence of any other lights [50]. Research on phalaenopsis also revealed that B light is beneficial for the accumulation of Chlorophyll [51]. Based on our results, B light appeared to motivate the accumulation of Chl and Car in P. trinervis.
Different plant species exhibit varying responses to different qualities of light, B light typically has a more pronounced impact on growth. In this study, the observations revealed a notable growth. in the absolute growth volume of P. trinervis seedling height and leaf length under B light. Heo et al. found that Marigold and Salvia plants had a greater height when exposed to B light compared to plants grown under W light [52]. Moreover, as a result of B light, leaf length grew significantly faster than under W light. According to Macedo and Leal-Costa, when B light was utilized, the light treatments caused Alternanthera brasiliana (L.) Kuntze produced the largest leaf area [53] and Nafiseh found the same phenomenon in Arabidopsis thaliana [54]. Our results proved that P. trinervis seedlings’ height was also significantly affected by light quality in terms of their morphological and photosynthetic features. Compared with W light, the height of plants growing under G light increased significantly. Ke et al. found that using G light resulted in a higher leaf length/width ratio, producing longer and narrower leaves compared to using W light [55]. Furthermore, we noted the following G light promoted the lengthening of leaves in P. trinervis plants. Similarly, the absolute growth of seedling height, stem diameter, leaf length, and petiole length under G light was the greatest, which was contrary to the report of Su that G light had a counteractive effect on plant growth [56]. The results on the impacts of monochromatic wavelength lights, as presented in this study, align with those previously reported by Wang et al. for cucumber plants, and by Yu et al. for Acacia mangium seedlings [56,57].
Our results indicated that Tr increased under G and B light in comparison to the W light, which was in agreement with the PN and gs. Yang observed a decrease in PN under the B light of tobacco [58]. This precisely aligns with the absorption spectra of photosynthetic pigments, Chl absorbs light in the 400–500 nm wavelength range. However, Yu et al. found that the PN level under G light was the lowest [59], contrary to our findings, which may be due to the great diversity among species. Therefore, under the same light intensity, the PN of the plant will be different under different light qualities.
Carbohydrates can regulate plant photosynthesis, growth, and development through a feedback mechanism. Our research showed that the accumulation of total sugar and sucrose content was noteworthily influenced by B light. A significant accumulation of reducing sugars and total sugars was induced by the G light. While amylum showed the highest content under W light. It was in agreement with Wang et al.’s findings [58], that the total soluble sugar content of cucumber reductions was significant in the plants under G. Therefore, our study suggests that B and G light facilitate the biosynthesis and accumulation of soluble sugars. The Calvin cycle enzymes may be more acute under B light due to a higher rate of activity. Ke et al. also discovered that plants exposed to B light exhibited increased Rubisco carboxylase activity and higher expression of genes related to the Calvin cycle enzymes [55,58].
Through regulating the ratio of PSI and PSII, plants can acclimatize to different light environments [59]. Different monochromatic light reduced the Fm of P. trinervis leaves, but increased the NPQ compared to W light. This corresponds to the change in PN of P. trinervis under different light qualities. This may be because plants that grow under B light conditions maintain a higher PN to avoid damage to photosynthetic organs since B light is more energetic. Besides losing more energy by dissipating heat from the PSII reaction centers, plants grown under G light conditions also exhibit lower photosynthetic capacity [60]. Past research has demonstrated a correlation between F0 and Chl content [61]. This study revealed that the various colors of light did not have a notable impact on the F0 of P. trinervis, this suggests that different monochromatic lights do not significantly damage the reaction center of P. trinervis PSII compared to W light. The Fm of plants cultivated in various monochromatic light conditions. was inferior to plants grown on W light. This suggests that populus grown under W light conditions exhibited a greater degree of the Redox Potential of the Primary Plastoquinone Electron Acceptor QA in Photosystem II status than under B and G light conditions. Fv/Fm remained in a stable area when populus were grown in favorable environments, but it decreased when plants were grown in unfavorable environments [62]. No significant difference was observed between Fv/Fm under different monochromatic light and W light. This may be the result of the fact that monochromatic light is not detrimental to the growth of P. trinervis. ΦPSII is usually associated with photosynthetic efficiency and electron transfer efficiency [63], The findings indicated that plants exposed to various monochromatic lights did not exhibit significant differences from those under W light, which is in line with the change in qp.
The findings indicated that various types of lighting had a significant impact on the growth and maturation of P. trinervis. Compared with the W light, Chl and Car accumulation and absolute plant growth of P. trinervis were significantly increased under B and G light. The effect of photosynthetic pigment accumulation was more obvious under B light. However, the absolute growth parameters such as seedling height and ground diameter were more obvious under G light. This study demonstrated that both B and G lights had noteworthy impacts. on the photosynthesis of P. trinervis. Light qualities also had an obvious effect on the accumulation of sugar content. B light mainly encouraged the accumulation of total soluble sugar and sucrose content, the accumulation effect of reducing sugar and total soluble sugar was more obvious under G light. Moreover, the influence of light characteristics on Chl fluorescence parameters of P. trinervis was primarily demonstrated by the rise in NPQ and the decline in Fm. Having insight into how spectral quality influences fundamental poplar growth parameters is crucial for comprehending both the natural and controlled development of plants.
Acknowledgement: The authors appreciate the support provided by the Applied Basic Research Foundation of Yunnan Province (Grant No. 202101AU070144) and the Joint Agricultural Project of Yunnan Province (Grant No. 202101BD070001-127).
Funding Statement: Applied Basic Research Foundation of Yunnan Province (Grant No. 202101AU070144) and the Joint Agricultural Project of Yunnan Province (Grant No. 202101BD070001-127).
Author Contributions: Jiaqi Li conceptualized and designed the experiments, carried out the experimental work, analyzed the resulting data, generated figures and/or tables, contributed to drafting the paper, and gave final approval. Zhensheng Qiao and Dan Zong conducted the experiments, contributed to drafting the paper, and gave final approval. Chengzhong He participated in the experiment conceptualization and design, data analysis, figure/table preparation, and final approval of the draft.
Availability of Data and Materials: The information provided in this research can be obtained by contacting the corresponding author upon request. The data is not accessible to the public for ethical considerations.
Ethics Approval: Not applicable.
Conflicts of Interest: The authors declare that they have no conflicts of interest to report regarding the present study.
References
1. Yavari N. An integrated approach to unravel the physiological and molecular basis of arabidopsis thaliana response to narrow-wavelength light (Master Thesis). McGill University: Canada; 2020. [Google Scholar]
2. McCree KJ. The action spectrum, absorptance and quantum yield of photosynthesis in crop plants. Agric Meteorol. 1971;9:191–216. doi:10.1016/0002-1571(71)90022-7. [Google Scholar] [CrossRef]
3. Belkov V, Garnik EY, Konstantinov YM. Mechanism of plant adaptation to changing illumination by rearrangements of their photosynthetic apparatus. Current Chall Plant Genet, Genom, Bioinform Biotechnol. 2019;24:101–3. [Google Scholar]
4. Roso R, Nunes UR, Müller CA, Paranhos JT, Lopes SJ, Dornelles SHB, et al. Light quality and dormancy overcoming in seed germination of Echium plantagineum L. (Boraginaceae). Braz J Biol. 2020;81:650–6. [Google Scholar]
5. Battle MW, Jones MA. Cryptochromes integrate green light signals into the circadian system. Plant, Cell & Environ. 2020;43(1):16–27. doi:10.1111/pce.v43.1. [Google Scholar] [CrossRef]
6. Schneider A, Godin C, Boudon F, Demotes-Mainard S, Sakr S, Bertheloot J. Light regulation of axillary bud outgrowth along plant axes: an overview of the roles of sugars and hormones. Front Plant Sci. 2019;10:478249. [Google Scholar]
7. Rehman M, Pan J, Mubeen S, Ma W, Luo D, Cao S, et al. Morpho-physio-biochemical, molecular, and phytoremedial responses of plants to red, blue, and green light: a review. Environ Sci Pollut Res. 2024;1–20. [Google Scholar]
8. Du Y, Ma H, Liu Y, Gong R, Lan Y, Zhao J, et al. Major quality regulation network of flavonoid synthesis governing the bioactivity of black wolfberry. New Phytol. 2024;242(2):558–75. doi:10.1111/nph.v242.2. [Google Scholar] [CrossRef]
9. Liu XY, Guo SR, Xu ZG, Jiao XL, Takafumi T. Regulation of chloroplast ultrastructure, cross-section anatomy of leaves, and morphology of stomata of cherry tomato by different light irradiations of light-emitting diodes. HortScience. 2011;46(2):217–21. doi:10.21273/HORTSCI.46.2.217. [Google Scholar] [CrossRef]
10. Shang J, Zhang S, Du J, Wang W, Li K, Yang W. Red and blue light induce soybean resistance to soybean mosaic virus infection through the coordination of salicylic acid and jasmonic acid defense pathways. Viruses. 2023;15(12):2389. [Google Scholar] [PubMed]
11. Zhou Y, Wu W, Sun Y, Shen Y, Mao L, Dai Y, et al. Integrated transcriptome and metabolome analysis reveals anthocyanin biosynthesis mechanisms in pepper (Capsicum annuum L.) leaves under continuous blue light irradiation. BMC Plant Biol. 2024;24(1):210. doi:10.1186/s12870-024-04888-x. [Google Scholar] [PubMed] [CrossRef]
12. Azari R, Tadmor Y, Meir A, Reuveni M, Evenor D, Nahon S, et al. Light signaling genes and their manipulation towards modulation of phytonutrient content in tomato fruits. Biotechnol Adv. 2010;28(1):108–18. doi:10.1016/j.biotechadv.2009.10.003. [Google Scholar] [PubMed] [CrossRef]
13. Pojmann-Ezeonyilo E, Langenhoven P, Ingwell LL. Evaluating the impact of light quality on plant-herbivore interactions using hemp as the model system. Environ Entomol. 2024;53(1):40–9. doi:10.1093/ee/nvad127. [Google Scholar] [PubMed] [CrossRef]
14. Sharkey TD, Raschke K. Effect of light quality on stomatal opening in leaves of Xanthium strumarium L. Plant Physiol. 1981;68(5):1170–4. doi:10.1104/pp.68.5.1170. [Google Scholar] [PubMed] [CrossRef]
15. Folta KM, Maruhnich SA. Green light: a signal to slow down or stop. J Exp Bot. 2007;58(12):3099–111. doi:10.1093/jxb/erm130. [Google Scholar] [PubMed] [CrossRef]
16. Ahammed GJ, Chen Y, Liu C, Yang Y. Light regulation of potassium in plants. Plant Physiol Biochem. 2022;170:316–24. doi:10.1016/j.plaphy.2021.12.019. [Google Scholar] [PubMed] [CrossRef]
17. Bourget CM. An introduction to light-emitting diodes. HortScience. 2008;43(7):1944–6. doi:10.21273/HORTSCI.43.7.1944. [Google Scholar] [CrossRef]
18. Morrow RC. LED lighting in horticulture. HortScience. 2008;43(7):1947–50. doi:10.21273/HORTSCI.43.7.1947. [Google Scholar] [CrossRef]
19. Bantis F, Panteris E, Dangitsis C, Carrera E, Koukounaras A. Blue light promotes vascular reconnection, while red light boosts the physiological response and quality of grafted watermelon seedlings. Sci Rep. 2021;11(1):21754. doi:10.1038/s41598-021-01158-w. [Google Scholar] [PubMed] [CrossRef]
20. Seif M, Aliniaeifard S, Arab M, Mehrjerdi MZ, Shomali A, Fanourakis D, et al. Monochromatic red light during plant growth decreases the size and improves the functionality of stomata in chrysanthemum. Funct Plant Biol. 2021;48(5):515–28. doi:10.1071/FP20280. [Google Scholar] [PubMed] [CrossRef]
21. Lin P, Di H, Li Z, Wang Y, Zhou W, Huang S, et al. Light irradiation maintains the sensory quality, health-promoting phytochemicals, and antioxidant capacity of post-harvest baby mustard. J Food Sci. 2022;87(1):112–23. doi:10.1111/1750-3841.15980. [Google Scholar] [PubMed] [CrossRef]
22. Heidari Z, Noruzi P, Rezapour-Fard J, Jabbarzadeh Z. Different LED light spectra’s and nano-chelated potassium affect the quality traits of Dolce Vita cut roses in soilless culture condition. Sci Rep. 2023;13(1):6769. doi:10.1038/s41598-023-34056-4. [Google Scholar] [PubMed] [CrossRef]
23. Frede K, Schreiner M, Baldermann S. Light quality-induced changes of carotenoid composition in pak choi Brassica rapa ss chinensis. J Photochem Photobiol B. 2019;193:18–30. doi:10.1016/j.jphotobiol.2019.02.001. [Google Scholar] [PubMed] [CrossRef]
24. Wojciechowska R, Długosz-Grochowska O, Kołton A, Żupnik M. Effects of LED supplemental lighting on yield and some quality parameters of lamb’s lettuce grown in two winter cycles. Scientia Horticulturae. 2015;187:80–6. doi:10.1016/j.scienta.2015.03.006. [Google Scholar] [CrossRef]
25. Yu Y, Wang Y, Liu W, Jia X, Ma L, Ren L, et al. Exploration of highly efficient light conversion agents for agricultural film based on the bay-substituted perylene diimides derivatives. Dyes Pigm. 2018;159:483–90. doi:10.1016/j.dyepig.2018.07.015. [Google Scholar] [CrossRef]
26. Zhang G, Shen S, Takagaki M, Kozai T, Yamori W. Supplemental upward lighting from underneath to obtain higher marketable lettuce (Lactuca sativa) leaf fresh weight by retarding senescence of outer leaves. Front Plant Sci. 2015;6:1110. [Google Scholar] [PubMed]
27. Dorais M, Yelle S, Gosselin A. Influence of extended photoperiod on photosynthate partitioning and export in tomato and pepper plants. New Zeal J Crop Hort. 1996;24(1):29–37. doi:10.1080/01140671.1996.9513932. [Google Scholar] [CrossRef]
28. Xu HL, Xu Q, Li F, Feng Y, Qin F, Fang W. Applications of xerophytophysiology in plant production—LED blue light as a stimulus improved the tomato crop. Scientia Horticulturae. 2012;148:190–6. doi:10.1016/j.scienta.2012.06.044. [Google Scholar] [CrossRef]
29. Hou Z, Wang Z, Ye Z, Du S, Liu S, Zhang J. Phylogeographic analyses of a widely distributed Populus davidiana: further evidence for the existence of glacial refugia of cool-temperate deciduous trees in Northern East Asia. Ecol Evol. 2018;8(24):13014–26. doi:10.1002/ece3.2018.8.issue-24. [Google Scholar] [CrossRef]
30. Liu ZR, Zhang GL, Feng SY, Liu W, Feng SH, Ye WP. Light quality distribution in different altitudes and its correlation with fruit quality of golden delicious apple tree. Northern Horticulturae. 2009;7:25–8. [Google Scholar]
31. Dere Ş, Güneş T, Sivaci R. Spectrophotometric determination of chlorophyll-A, B and totalcarotenoid contents of some algae species using different solvents. Turk J Bot. 1998;22(1):13–8. [Google Scholar]
32. Buysse J, Merckx R. An improved colorimetric method to quantify sugar content of plant tissue. J Exp Bot. 1993;44(10):1627–9. doi:10.1093/jxb/44.10.1627. [Google Scholar] [CrossRef]
33. van Kooten O, Snel JF. The use of chlorophyll fluorescence nomenclature in plant stress physiology. Photosynth Res. 1990;25(3):147–50. doi:10.1007/BF00033156. [Google Scholar] [PubMed] [CrossRef]
34. Maxwell K, Johnson GN. Chlorophyll fluorescence—a practical guide. J Exp Bot. 2000;51(345):659–68. doi:10.1093/jexbot/51.345.659. [Google Scholar] [CrossRef]
35. Walters RG. Towards an understanding of photosynthetic acclimation. J Exp Bot. 2005;56(411):435–47. doi:10.1093/jxb/eri060. [Google Scholar] [PubMed] [CrossRef]
36. Hu YB, Sun GY, Wang XC. Induction characteristics and response of photosynthetic quantum conversion to changes in irradiance in mulberry plants. J Plant Physiol. 2007;164(8):959–68. doi:10.1016/j.jplph.2006.07.005. [Google Scholar] [PubMed] [CrossRef]
37. Matsuda R, Ohashi-Kaneko K, Fujiwara K, Kurata K. Analysis of the relationship between blue-light photon flux density and the photosynthetic properties of spinach (Spinacia oleracea L.) leaves with regard to the acclimation of photosynthesis to growth irradiance. Soil Sci Plant Nutr. 2007;53(4):459–65. doi:10.1111/j.1747-0765.2007.00150.x. [Google Scholar] [CrossRef]
38. Sæbø A, Krekling T, Appelgren M. Light quality affects photosynthesis and leaf anatomy of birch plantlets in vitro. Plant Cell, Tissue Organ Cult. 1995;41:177–85. doi:10.1007/BF00051588. [Google Scholar] [CrossRef]
39. Wu MC, Hou CY, Jiang CM, Wang YT, Wang CY, Chen HH, et al. A novel approach of LED light radiation improves the antioxidant activity of pea seedlings. Food Chem. 2007;101(4):1753–8. doi:10.1016/j.foodchem.2006.02.010. [Google Scholar] [CrossRef]
40. Senger H, Bauer B. The influence of light quality on adaptation and function of the photosynthetic apparatus. Photochem Photobiol. 1987;45:939–46. doi:10.1111/php.1987.45.issue-S1. [Google Scholar] [CrossRef]
41. Anderson JM, Chow WS, Park YI. The grand design of photosynthesis: acclimation of the photosynthetic apparatus to environmental cues. Photosynth Res. 1995;46(1–2):129–39. [Google Scholar] [PubMed]
42. Walters RG. Towards an understanding of photosynthetic acclimation. J Exp Bot. 2005 Jan;56(411):435–47. [Google Scholar] [PubMed]
43. Briggs WR, Huala E. Blue-light photoreceptors in higher plants. Annu Rev Cell Dev Biol. 1999;15:33–62. doi:10.1146/cellbio.1999.15.issue-1. [Google Scholar] [CrossRef]
44. Lin C. Plant blue-light receptors. Trends Plant Sci. 2000;5(8):337–42. doi:10.1016/S1360-1385(00)01687-3. [Google Scholar] [PubMed] [CrossRef]
45. Xu K, Guo YP, Zhang SL. Effect of light quality on photosynthesis and chloropyll fluorescence in strawberry leaves. Agric Sci China. 2004;3(9):678–86 (In Chinese). [Google Scholar]
46. Kobayashi K, Amore T, Lazaro M. Light-emitting diodes (LEDs) for miniature hydroponic lettuce. Opt Photonics J. 2013;2013:74–7. [Google Scholar]
47. Zhang L, Liu S, Zhang Z, Yang R, Yang X. Dynamic of different qualities on growth of Toona sinensis seedlings. Acta Agric Boreali Occidentalis Sin. 2010;19:115–9. [Google Scholar]
48. Poudel PR, Kataoka I, Mochioka R. Effect of red-and blue-light-emitting diodes on growth and morphogenesis of grapes. Plant Cell, Tissue and Organ Cult. 2008;92:147–53. doi:10.1007/s11240-007-9317-1. [Google Scholar] [CrossRef]
49. Li H, Xu Z, Tang C. Effect of light-emitting diodes on growth and morphogenesis of upland cotton (Gossypium hirsutum L.) plantlets in vitro. Plant Cell, Tissue and Organ Cult (PCTOC). 2010;103:155–63. doi:10.1007/s11240-010-9763-z. [Google Scholar] [CrossRef]
50. Zhou H, Liu S, Yang Y, Yang A, Liu L, Yu F. Effect of light quality on the growth and photosynthetic characteristics of Cinnamomum camphora rooted cuttings. Scand J For Res. 2021;36(7–8):532–8. [Google Scholar]
51. Ren G, Wang X, Zhu G. Effect of LED in different light qualities on growth of Phalaenopsis plantlets. Chin Bull Bot. 2016;51(1):81–8 (In Chinese). [Google Scholar]
52. Heo J, Lee C, Chakrabarty D, Paek K. Growth responses of marigold and salvia bedding plants as affected by monochromic or mixture radiation provided by a light-emitting diode (LED). Plant Growth Regul. 2002;38(3):25–230. [Google Scholar]
53. Macedo AF, Leal-Costa MV, Tavares ES, Lage CLS, Esquibel MA. The effect of light quality on leaf production and development of in vitro-cultured plants of Alternanthera brasiliana Kuntze. Environ Exp Bot. 2011;70(1):43–50. doi:10.1016/j.envexpbot.2010.05.012. [Google Scholar] [CrossRef]
54. Yavari N, Tripathi R, Wu BS, MacPherson S, Singh J, Lefsrud M. The effect of light quality on plant physiology, photosynthetic, and stress response in Arabidopsis thaliana leaves. PLoS One. 2021;16(3):e0247380. doi:10.1371/journal.pone.0247380. [Google Scholar] [PubMed] [CrossRef]
55. Ke X, Li JY, Li XY, Wu CF, Xu CH, Jing Y, et al. Effects of different light quality on growth and photosynthesis of tobacco (Nicotiana tabacum L.) leaves. Plant Physiol J. 2011;47(5):512–20. [Google Scholar]
56. Yang LY, Wang LT, Ma JH, Ma ED, Li JY, Gong M. Effects of light quality on growth and development, photosynthetic characteristics and content of carbohydrates in tobacco (Nicotiana tabacum L.) plants. Photosynthetica. 2017;55(3):467–77. doi:10.1007/s11099-016-0668-x. [Google Scholar] [CrossRef]
57. Yu H, Ong BL. Effect of radiation quality on growth and photosynthesis of Acacia mangium seedlings. Photosynthetica. 2003;41:349–55. doi:10.1023/B:PHOT.0000015458.11643.b2. [Google Scholar] [CrossRef]
58. Wang H, Gu M, Cui J, Shi K, Zhou Y, Yu J. Effects of light quality on CO2 assimilation, chlorophyll-fluorescence quenching, expression of Calvin cycle genes and carbohydrate accumulation in Cucumis sativus. J Photochem Photobiol B: Biol. 2009;96(1):30–7. doi:10.1016/j.jphotobiol.2009.03.010. [Google Scholar] [PubMed] [CrossRef]
59. Jensen PE, Bassi R, Boekema EJ, Dekker JP, Jansson S, Leister D, et al. Structure, function and regulation of plant photosystem I. BBA-Bioenergetics. 2007;1767(5):335–52. doi:10.1016/j.bbabio.2007.03.004. [Google Scholar] [PubMed] [CrossRef]
60. Badger MR, von Caemmerer S, Ruuska S, Nakano H. Electron flow to oxygen in higher plants and algae: rates and control of direct photoreduction (Mehler reaction) and rubisco oxygenase. Philos Trans Royal Soc London. Series B: Biol Sci. 2000;355(1402):1433–46. doi:10.1098/rstb.2000.0704. [Google Scholar] [PubMed] [CrossRef]
61. Schnettger B, Critchley C, Santore UJ, Graf M, Krause GH. Relationship between photoinhibition of photosynthesis, D1 protein turnover and chloroplast structure: effects of protein synthesis inhibitors. Plant, Cell & Environ. 1994;17(1):55–64. doi:10.1111/pce.1994.17.issue-1. [Google Scholar] [CrossRef]
62. Allen DJ, Ort DR. Impacts of chilling temperatures on photosynthesis in warm-climate plants. Trends Plant Sci. 2001;6(1):36–42. doi:10.1016/S1360-1385(00)01808-2. [Google Scholar] [PubMed] [CrossRef]
63. Miyake C, Amako K, Shiraishi N, Sugimoto T. Acclimation of tobacco leaves to high light intensity drives the plastoquinone oxidation system—relationship among the fraction of open PSII centers, non-photochemical quenching of Chl fluorescence and the maximum quantum yield of PSII in the dark. Plant Cell Physiol. 2009;50(4):730–43. doi:10.1093/pcp/pcp032. [Google Scholar] [PubMed] [CrossRef]
Cite This Article
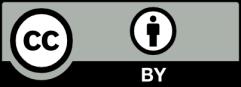
This work is licensed under a Creative Commons Attribution 4.0 International License , which permits unrestricted use, distribution, and reproduction in any medium, provided the original work is properly cited.