Open Access
ARTICLE
Grazing Pressure and Plant Functional Types in Puna Highlands, Northwestern Argentina
1 IPAF NOA—INTA (National Institute for Agricultural Technology); Posta de Hornillos, Jujuy, 4622, Argentina
2 Facultad de Ciencias Naturales, Universidad Nacional de Salta, Salta, 4400, Argentina
3 Instituto de Bio y Geociencias del NOA (IBIGEO), UNSa-CONICET. Laboratorio de Ecología Aplicada a la Conservación (LEAC), Facultad de Ciencias Naturales, Universidad Nacional de Salta, Salta, 4400, Argentina
4 Maestría en Gestión Ambiental, Escuela de Negocios, Universidad Católica de Salta (UCASAL), Salta, 4400, Argentina
* Corresponding Author: Quiroga Mendiola Mariana. Email:
(This article belongs to the Special Issue: Ecology of Rangelands in Argentina)
Phyton-International Journal of Experimental Botany 2024, 93(5), 1067-1080. https://doi.org/10.32604/phyton.2024.050556
Received 10 February 2024; Accepted 16 April 2024; Issue published 28 May 2024
Abstract
The Puna grasslands support grazing systems that produce meat and wool in multi-species herds, especially from llama (Lama glama) and sheep. However, it is yet unknown whether grazing pressure can modify grassland structure and Plant Functional Types diversity and cover in Puna grasslands. We analyzed the relationship between grazing pressure and Plant Functional Types by comparing transects located near stockyards (high grazing pressure) and far from them (low pressure) and by evaluating the relationship between the Plant Functional Types cover to a Grazing Pressure Index (GPI). This index incorporates the heterogeneity of traditional pastoral management. At two ecological sites (Ciénego and Tolar), we sampled paired transects (near-far). Plots near and far from corrals were similar in all the variables measured except for plots in Tolar, which tend to have higher total cover, possibly due to fecal fertilization near stockyards. Furthermore, we recorded an increase in total cover and grass cover at higher GPI in Ciénego, while in Tolar we found lower values of total cover and herbaceous dicotyledonous cover at intermediate intensities of GPI. The only negative relationship found was the cover of clonal and non-clonal shrubs, possibly explained by the greater use of these shrubs as firewood near stockyards. In summary, our study does not show important changes in Plant Functional Types as a function of grazing pressure in the studied grasslands probably because grazing pressure is diluted or diminished when livestock rotates in different areas and grazing durations vary. Finally, the long grazing history with which the Puna has evolved could also contribute to the co-structuration between native vegetation and livestock farming.Keywords
Supplementary Material
Supplementary Material FileGrazing on arid and semi-arid lands is often the only livelihood for many farming families in these adverse environments [1–3]. Although grazing on natural vegetation significantly alters its structure and functionality [4,5], the effects of herder-monitored grazing on ecosystems in high Andean regions, such as the Argentine Puna, remain poorly understood. Therefore, it is crucial to generate data that enriches the global theoretical framework of grazing ecology, enabling the development of management strategies that enhance the sustainability of pastoral systems and can ultimately be integrated into public policies.
Like many high-altitude environments [6], extreme conditions such as intense solar radiation, temperature fluctuations, strong winds, and limited rainfall prevail in the Argentine Puna. Despite these challenges, pastoral communities have long inhabited the area, sometimes by generating exchange relations with other neighboring communities, agro-pastoralists, or pure agriculturalists, within a framework of mutual benefits [7,8]. Their livelihood relies on the mobile and fluctuating breeding of domestic animals, primarily sheep and llamas (L. glama), which graze on the native vegetation. These native pastoralists operate within a production logic that integrates various environmental, socio-economic, and historical factors, probably resulting in highly efficient production systems tailored to these harsh conditions [9,10]. However, the relationship between historical livestock grazing practices and the high Andes plant communities, which form the backbone of pastoral activity, remains uncertain.
Various theoretical and methodological approaches have examined the relationship between natural vegetation and grazing patterns. In arid and semi-arid environments, livestock congregates around water sources and corrals, creating a gradient of grazing pressure known as the piosphere pattern. This pattern entails decreasing grazing pressure as the distance from the focal point increases [11,12]. While the piosphere pattern approach has been used in different rangelands across Argentina, such as the Chaco Seco and the Monte phytogeographical provinces [12,13], its applicability in the Puna remains unexplored. However, given that this approach overlooks other potentially significant factors, such as livestock species, herd size, grazing rotation frequency, duration of grazing, and seasonal variations, it may be necessary to explore the relationship between livestock pressure and vegetation using alternative approaches that incorporate this variability in grazing regimes [14]. To quantify the potential effect of grazing pressure on vegetation, the increasing recognition of species with similar responses to disturbances coexisting in communities has led to a growing use of the functional classification of plants [15,16]. This approach considers that phylogenetically distant organisms subjected to similar selective pressures develop comparable morphological, anatomical, and physiological traits [17]. Consequently, changes in the proportion of different plant functional types are expected to reflect alterations in ecosystem functionality in response to grazing pressure [18–20]. However, research on the response of plant functional types to grazing pressure in Argentine Puna rangelands is currently lacking.
In this study, we investigate the relationship between grazing pressure and plant functional types in two ecological sites within the aboriginal community of Suripujio (Jujuy province, Puna of Argentina). We employed two methodological approaches to address the following questions: (a) How does the plant community (plant functional types richness and cover) vary in proximity to or remoteness from the corrals, which function as high-pressure points? (b) What is the relationship between plant functional types richness and cover and a Grazing Pressure Index that encompasses the diversity of grazing regimes? (c) Do the general outcomes derived from employing the piosphere pattern approach (a) and the Grazing Pressure Index characterizing grazing regimes (b) exhibit consistency? Addressing these questions is essential for enhancing our understanding of the dynamics between grazing pressure and plant functional types, thereby informing more effective management strategies for sustainable pastoral systems in the high Andean region of Argentina.
We conducted the study on the high plateau of Puna in the Andean mountains of Northwest Argentina, specifically in Suripujio (Fig. 1). This area experiences an arid and cold climate, with an average temperature of 8.5°C and an average annual precipitation of 300 mm. The region also exhibits high daily and annual temperature fluctuations and a high altitude of 3500 m above see level (m a.s.l.) Grazing territories are managed year-round, with rotations between lower lands (3500 m a.s.l) and higher altitudes above 4000 m a.s.l.
Figure 1: The study area and transects are located at two distances from the corral in two ecological sites in Suripujio, Jujuy Province, Northwestern Argentina
The inhabitants primarily consist of family farmers from aboriginal communities who raise llamas (L. glama) and sheep, with meat and wool being the main products. Flocks are composed of only llamas, only sheep, or both llamas and sheep and in very different quantities of each one. Adjacent to the main family house is a small vegetable garden containing potatoes, fava beans (Faba sp.), and other horticultural species. Grazing occurs through movements between various grazing sites within the community territory. Each family has the right to use designated family grazing territories determined by the community assembly. These territories are not continuous spatially, as grazing sectors are designated across different ecological sites used by families throughout the year. As a result, families move their flocks 2 to 6 times a year between different ecological sites, remaining at each site for periods ranging from 1 week to 5 months [21]. These movements and durations are influenced by environmental variables (especially water availability), the livestock cycle (breeding and calving), and other factors such as school schedules for children, religious events, fairs, and barter exchanges [7,22]. Hence, the pastoralists use the grazing sites in several different ways, generating a very heterogeneous grazing pressure between territories. Within each ecological site, there is a stall (known as “puesto” or “estancia”) where the pastoralist woman temporarily resides. These structures are tiny houses with adjacent corrals. The pastoralists manage two main ecological grazing sites: a shrub-steppe (locally known as “Tolar”) at 3500 m a.s.l., and a wet grassy pasture (known as “Ciénego”) at 4000–4500 m a.s.l. [21].
2.2 Plant Functional Types in Suripujio
To identify the Plant Functional Types in the area, we created a presence/absence card for each plant species collected, identifying functional traits associated with arid conditions and plant responses to herbivory (Table 1) [23].
We performed a cluster analysis using a final matrix of 71 species and ten plant traits (Table S1). The data were standardized, and we employed the Average linkage method with Euclidean distances. As a result, we identified eight Plant Functional Types. The list of species, their corresponding functional types, and the traits used to define these functional types are presented as supplementary information. Following this, we conducted all further analyses regarding vegetation and its relationship with grazing pressure by transforming floristic data into data on the coverage and richness of plant functional types.
Our sampling occurred during the middle or end of the growing season (summer). Considering the piosphere pattern, we conducted paired samplings along two linear transects, each one 30 m in length, perpendicular to a radial line originating from the corral. This was done at each ecological site (Ciénego: n = 6, and Tolar: n = 7) (Fig. 1). The near transect was positioned at a 100-m distance from the corral (to avoid heavily trampled areas in the vicinity of the corral), and the far transect was set at 700 m away (as far away as possible before entering the territory of an adjacent grazing post).
All transects experience some degree of grazing pressure. During each transect, we recorded all plant species present along the line. We calculated the percentage of line coverage by vegetation to determine total and species-specific cover (line transect intercept). Utilizing this data and the previously established classification into PFT groups, we calculated the richness and cover of each Plant Functional trait.
Given the diverse management strategies employed by families in grazing spaces, we developed a Grazing Pressure Index (GPI). Information for this index was gathered through interviews and participatory observations (Table 2). Since grazing pressure is very heterogeneous along space and time, and because of the different flock sizes and species, we categorized and ranked the qualitative pastoralist’s responses.
GPI=a × b × c × ((d × e llamas) + (d × e sheep))log10 Corral distance × f
The response variables utilized included plant functional type richness, total cover, and cover for each plant functional type. We compared these variables between sectors near (with higher grazing pressure) and far (with lower grazing pressure) from the corrals at each ecological site using a Wilcoxon nonparametric test for paired samples. Additionally, we used generalized linear models to assess the relationship between the response variables and the Grazing Pressure Index. We modeled different cover variables (in %) assuming a binomial distribution (logit link function), while plant functional type richness (count) was modeled using a Poisson distribution (log link function) [24]. We adjusted for linear and quadratic effects and selected the model with the lowest AIC (Akaike Information Criterion). We verified the assumptions of normality and homoscedasticity through residual plots. All tests were conducted at a significance level of 5% using R software, version 4.0.0 [25].
3.1 Floristic and Plant Functional Types Richness
Seventy-one species representing 26 families were identified. Cluster analysis of these species enabled us to discriminate eight plant functional types based on traits related to grazing pressure and frequent droughts (Table 3).
In the Ciénego ecological site, total cover was 70%, dominated by grasses/graminoids and herbaceous dicots with 66% and 33% of total cover, respectively. In the Tolar ecological site, total cover was 50%, and with more diverse and even communities: herbaceous dicots (42%), clonal shrubs (27%), non-clonal shrubs (14%), and grasses and graminoids (11%) (Fig. 2).
Figure 2: Rank-abundance curves of Plant Functional Types (PFTs) in two ecological sites (Ciénego and Tolar) in Suripujio, Jujuy, Northwestern Argentina. Only the names of PFTs with proportional cover greater than 0.1 are shown
3.2 Plant Functional Types and Piosphere Pattern
We did not find any differences when comparing all the response variables between the two distances from the corral at the Ciénego ecological site (Fig. 3). However, in the Tolar ecological site, we observed a trend (p = 0.078; Fig. 4a) towards higher total cover (1.15 times) in sectors closer to the corral compared to distant sectors. The other response variables in Tolar did not show any changes between the two distances (Figs. 4b–4f).
Figure 3: Total cover (a), richness of plant functional types (b), and cover of dominant plant functional types (c and d) in sectors near and far from the corral at the Ciénego ecological site, Suripujio (Jujuy, Northwestern Argentina). The first and third quartiles delimit the box, and the thick line between quartiles represents the median. Vertical whiskers indicate adjacent values located at a distance equal to or less than 1.5 times the interquartile range
Figure 4: Total cover (a), richness of plant functional types (b), and cover of dominant plant functional types (c–f) in sectors near and far from the corral at the Tolar ecological site, Suripujio (Jujuy, Northwestern Argentina). The legend of Fig. 3 provides further details on the box explanation
3.3 Plant Functional Types and Grazing Pressure Index
At Ciénego ecological site, total cover was linearly and positively related to the Grazing Pressure Index (GPI) (Fig. 5). In contrast, in Tolar ecological site, it decreased to intermediate values of this index (Fig. 6). Plant functional types richness showed no relationship with GPI in either of the two ecological sites (Figs. 5b and 6b). Of the two dominant plant functional types at the Ciénego ecological site, only grasses and graminoids showed a lineal cover increase with higher grazing pressure (Fig. 5c). Two out of the four dominant plant functional types were related to GPI in the Tolar ecological sites. Herbaceous dicotyledons cover decreased at intermediate pressures (Fig. 6d), while clonal shrubs’ cover declined linearly with rising GPI.
Figure 5: Total cover, richness of plant functional types, and cover of dominant plant functional types of the Ciénego ecological site as a function of the Grazing Pressure Index (GPI), Suripujio (Jujuy, Northwestern Argentina)
Figure 6: Total cover, richness of plant functional types, and cover of dominant plant functional types of the Tolar ecological site as a function of the Grazing Pressure Index (GPI), Suripujio (Jujuy, Northwestern Argentina)
Our study pioneers the investigation of plant functional type diversity and abundance about grazing in the Puna highland ecosystems. The results varied depending on the ecological site and methodological approach. The piosphere pattern approach showed few vegetation changes about grazing pressure only in the Tolar ecological site. Using the GPI approach, we found more sensitivity, but in general, the results were contrary to expectations.
Based on the results obtained regarding the piosphere pattern and plant functional types, our findings indicate a few minimal differences between sectors near and far from the corral. It suggests that this approach does not robustly detect changes in vegetation cover in response to grazing pressure, although in Tolar, it shows a slightly significant variation. This uniformity in the plant community structure, irrespective of proximity to the grazing point, hints at a certain level of resilience or adaptation to grazing pressure in the Ciénego ecological site. Conversely, in the Tolar ecological site, we noted a trend toward higher total cover in sectors closer to the corral than those farther away. Although this observed increase is marginally significant, it hints at the potential influence of grazing pressure on vegetation composition and distribution within this site. Contrary to what was expected by the piosphere pattern, this result could be explained by a positive effect of fertilization from animal dung, as we found a higher frequency of dung in plots near the corrals in Tolar (Fig. S1). Similar findings support this explanation, as it has been found that the presence of manure from both exotic and native animals, can increase plant diversity and cover [12,26–29]. However, it is important to highlight that while total cover tended to augment in closer sectors, the remaining response variables in Tolar exhibited no discernible changes between distances from the corral.
The relationships became more complex when other factors related to the grazing regime were incorporated into the GPI. At the Ciénego ecological site, grazing pressure seems to be positively correlated with the total cover and the cover of grasses and graminoids. Similar to the explanation provided for the piosphere pattern, these results could be attributed to the effect of organic fertilization provided by animal manure (as higher GPI values are expected to correspond to greater dung frequency) and/or the removal of soil crusts in these deserts or semidesert environments, creating microsites that are subsequently colonized by native vegetation, especially grasses, and graminoids [26]. Conversely, at the Tolar ecological site, total cover and cover of herbaceous dicotyledons decreased towards intermediate values of the GPI, suggesting a potential threshold effect where moderate grazing pressure may lead to reduced vegetation cover. Low vegetation cover values at both ends of the index could be explained by reduced plant consumption by livestock at low GPI values (less pressure), and likely by dung fertilization at higher GPI values [26–30]. The only negative linear response regarding the GPI was for the cover of clonal shrubs at the Tolar ecological site. However, since the shrubs comprising this plant functional type have low grazing preference, especially for sheep, another yet undisclosed mechanism should be responsible for this pattern rather than grazing pressure itself. These shrubs are frequently used as firewood, and it is expected that where there is a higher GPI (ultimately indicating greater use), the harvest of these shrubs would also increase [31]. These findings emphasize the need to enhance the construction of the GPI to include, in future studies, other aspects related to livestock management (such as collection of firewood and dung density estimation, among others).
By incorporating the heterogeneity of the grazing regime through the Grazing Pressure Index (GPI), we identified more associations than with the piosphere pattern approach. Our results highlight the complex interaction between heterogeneous forms of livestock management and vegetation dynamics. Further investigation into the underlying mechanisms driving these variations is needed to enhance our understanding of ecosystem responses to grazing in highland environments.
In summary, our study in the vicinity of Suripujio, in the highlands of the Argentinean Puna, did not find a clear and significant negative impact of grazing pressure on vegetation cover and plant functional type richness. Similarly, no significant differences were found in functional diversity between sites with different grazing intensities in semi-arid Mediterranean grasslands [32]. Although our study did not quantify functional diversity, plant functional type richness also showed no relationship with grazing pressure. This partially contrasts with the findings of other authors, who analyzed plant functional types about stocking rates and detected a decrease in the abundance of dwarf palms and short grasses with higher grazing pressure in arid or semi-arid environments in Spain [18].
The lack of response to grazing pressure for most of the variables studied could be explained by various reasons, not mutually exclusive. Firstly, grazing pressure may be moderated enough not to modify vegetation structure and functionality [5,33–35]. Secondly, given that Puna environments have evolved under a long grazing history [36], it is expected that vegetation diversity is not drastically altered by grazing pressure and that livestock grazing is compatible with biodiversity conservation [32,37–40]. Moreover, arid and semi-arid sites often exhibit minimal responses to herbivory pressure, possibly because the vegetation has evolved traits to escape drought that are often similar to traits that avoid grazing [23,41]. Additionally, the daily grazing pressure away from the corral, monitored by herders, prevents animals from remaining near the corral for extended periods. Managing grazing pressure through daily, seasonal, and inter-annual practices helps avoid the formation of overgrazed or undergrazed areas.
Furthermore, the longstanding co-evolution of pastures and herds in the Puna highlands could also explain the absence of negative responses mentioned above. This ancestral environmental management, livestock husbandry, and pastoral economy, passed down through generations, likely increases the compatibility of pastoral activities and biodiversity conservation by dispersing grazing pressure across space and time [42,43]. In this sense, the subdivision of land into units of management based on inherent landscape productivity and biophysical characteristics seems to be a conservative use of low-productive landscapes in the Puna region as other authors postulate for low-productive grasslands in Australia [44]. Thus, as in traditional Andean grazing management, the intensity and moments of grazing should be varied and utilized in a vast menu of management strategies [45].
Acknowledgement: We thank the Suripujio Aboriginal Community for their hospitality, support, and information provided. To Dr. Alicia Barchuk and Dr. Gabriela Karasik, who directed the doctoral thesis based on which this article was written. Also, to Daniela Liebeskind, Lucas Bilbao, Julio Tolaba, Diego Molina, Luciana Costa, Víctor Aquino, and Juan Pablo Zamora for their companionship during arduous field sampling at high altitude and far from their homes. To the fellow researchers, administrative staff, and authorities of IPAF NOA INTA, who kindly gave me all their support. To the Faculty of Natural Sciences of the National University of Salta because it trained and provided us with tools for our professional life. Finally, we are grateful for the comments and suggestions of reviewers who helped to substantially improve the clarity of this work.
Funding Statement: The authors received no specific funding for this study.
Author Contributions: The authors confirm their contribution to the paper as follows: study conception and design: Mariana Quiroga Mendiola; data collection: Mariana Quiroga Mendiola; analysis and interpretation of results: Mariana Quiroga Mendiola and Andrés Tálamo; draft manuscript preparation: Mariana Quiroga Mendiola and Andrés Tálamo. All authors reviewed the results and approved the final version of the manuscript.
Availability of Data and Materials: The data is available upon request to the authors.
Ethics Approval: Not applicable.
Conflicts of Interest: The authors declare that they have no conflicts of interest to report regarding the present study.
Supplementary Materials: The supplementary material is available online at https://doi.org/10.32604/phyton.2024.050556.
References
1. Wafula WM, Wasonga OV, Koech OK, Kibet S. Factors influencing migration and settlement of pastoralists in Nairobi city, Kenya. Pastoralism. 2022;12:2. doi:10.1186/s13570-021-00204-6. [Google Scholar] [CrossRef]
2. Nori M, Scoones I. Rethinking policies for pastoralists—governing the rangelands. Rangel J. 2023;45:53–66. doi:10.1071/RJ23010. [Google Scholar] [CrossRef]
3. Volkovitskiy A, Terekhina A. A microregional reindeer herding landscape in Yamal: patterns and dynamics of movements. Pastoralism. 2023;13:27. doi:10.1186/s13570-023-00292-6. [Google Scholar] [CrossRef]
4. Cao F, Li W, Jiang Y, Gan X, Zhao C, Ma J. Effects of grazing on grassland biomass and biodiversity: a global synthesis. Field Crops Res. 2024;306:109204. doi:10.1016/j.fcr.2023.109204. [Google Scholar] [CrossRef]
5. Su Y, Dong K, Wang C, Liu X. Grazing promoted plant litter decomposition and nutrient release: a meta-analysis. Agric Ecosyst Environ. 2022;337:108051. doi:10.1016/j.agee.2022.108051. [Google Scholar] [CrossRef]
6. Jacobsen D, Dangles O. The high altitude environment. In: Jacobsen D, Dangles O, editors. Ecol High Alt Waters. UK: Oxford University Press; 2017. p. 272. [Google Scholar]
7. Arzamendia Y, Rojo V, González NM, Baldo JL, Zamar MI, Lamas HE, et al. The Puna pastoralist system: a coproduced landscape in the Central Andes. Mt Res Dev. 2021;41:R38–49. [Google Scholar]
8. Abeledo SH. Las Tasas de trueque en las caravanas de la Puna argentina: Reflexiones sobre sus continuidades y cambios (Santa Rosa de los Pastos Grandes, Departamento de Los Andes). Estud Atacameños. 2023;69:e5366 (In Spanish). doi:10.22199/issn.0718-1043. [Google Scholar] [CrossRef]
9. Flores Ochoa J, Uywamichiq P. Pastores de Puna. Lima: Instituto de Estudios Peruanos; 1977. pp. 305 (In Spanish). [Google Scholar]
10. Quiroga Mendiola M, Llueve sobre mojado. Trashumancia conceptual frente al pastoralismo alto andino. In: Manzanal M, Ponce Y, Organizadoras M, editors. La desigualdad del Desarro? Controversias y disyuntivas enel desarrollo rural del norte argentino. Rural Norte Argent. Buenos Aires: CICCUS; 2013. pp. 253–72 (In Spanish). [Google Scholar]
11. Thrash I, Derry JF. The nature and modelling of piospheres: a review. Koedoe. 1999;42:73–94. [Google Scholar]
12. Calvo DA, Peter G, Gaitán JJ. El clima modula los efectos del pastoreo sobre la productividad primaria en ecosistemas áridos de Argentina. Ecosistemas. 2021;30:2228(In Spanish). doi:10.7818/ECOS. [Google Scholar] [CrossRef]
13. Trigo CB, Tálamo A, Nunez-Regueiro MM, Derlindati EJ, Maras GA, Barchuk AH, et al. A woody plant community and tree-cacti associations change with distance to a water source in a dry chaco forest of Argentina. Rangel J. 2017;39:15–23. doi:10.1071/RJ16014. [Google Scholar] [CrossRef]
14. Adler PB, Morales JM. Influence of environmental factors and sheep grazing on an Andean grassland. J Range Manag. 1999;52:471–81. doi:10.2307/4003774. [Google Scholar] [CrossRef]
15. Maza-Villalobos S, García-Ramírez P, Endress BA, Lopez-Toledo L. Plant functional traits under cattle grazing and fallow age scenarios in a tropical dry forest of Northwestern Mexico. Basic Appl Ecol. 2022;64:30–44. doi:10.1016/j.baae.2022.06.006. [Google Scholar] [CrossRef]
16. Huxley JD, White CT, Humphries HC, Weber SE, Spasojevic MJ. Plant functional traits are dynamic predictors of ecosystem functioning in variable environments. J Ecol. 2023;111:2597–613. doi:10.1111/jec.v111.12. [Google Scholar] [CrossRef]
17. Díaz SM, Gurvich DE, Pérez Harguindeguy N, Cabido MR. ¿Quién necesita tipos funcionales de plantas? Bol Soc Argent Botánica. 2002;37:135–40 (In Spanish). [Google Scholar]
18. Navarro T, Alados CL, Cabezudo B. Changes in plant functional types in response to goat and sheep grazing in two semi-arid shrublands of SE Spain. J Arid Environ. 2006;64:298–322. doi:10.1016/j.jaridenv.2005.05.005. [Google Scholar] [CrossRef]
19. Miedema Brown L, Anand M. Plant functional traits as measures of ecosystem service provision. Ecosphere.2022;13:e3930. doi:10.1002/ecs2.v13.2. [Google Scholar] [CrossRef]
20. Yan P, He N, Yu K, Xu L, van Meerbeek K. Integrating multiple plant functional traits to predict ecosystem productivity. Commun Biol. 2023;6:1–8. [Google Scholar]
21. Quiroga Mendiola M. Donde no se puede sembrar. La triple espacialidad pastoril en Suripujio, Puna de Jujuy, Argentina. In: Benedetti A, Tomasi J, editors. Espac Altoandinas Nuevos Aportes Desde Argent. Facultad de Filosofía y Letras: Universidad Nacional de Buenos Aires; 2021. p. 227–256 (In Spanish). [Google Scholar]
22. Quiroga Mendiola M, Cladera JL. Ganadería en la Puna Argentina. In: Grau HR, Babot MJ, Izquierdo AE, Grau A, editors. Puna Argent Nat Cult. Tucuman, Argentina: Fundación Miguel Lillo; 2018. pp. 387–402 (In Spanish). [Google Scholar]
23. Pérez-Harguindeguy N, Díaz S, Garnier E, Lavorel H, Poorter P, Jaureguiberry M, et al. Corrigendum to: new handbook for standardised measurement of plant functional traits worldwide. Aust J Bot. 2016;64(8):715–6. doi:10.1071/BT12225_CO. [Google Scholar] [CrossRef]
24. Zuur FL, Ieno AF, Walker NJ, Saveliev AA, Smith G. Mixed effects models and extensions in ecology with R. New York: Springer; 2009. [Google Scholar]
25. R Core Team. R: a language and environment for statistical computing; 2022. https://www.R-project.org/time. [Accessed 2024]. [Google Scholar]
26. DeGabriel JL, Albon SD, Fielding DA, Riach DJ, Westaway S, Irvine RJ. The presence of sheep leads to increases in plant diversity and reductions in the impact of deer on heather. J Appl Ecol. 2011;48(5):1269–77. doi:10.1111/j.1365-2664.2011.02032.x. [Google Scholar] [CrossRef]
27. Reider KE, Schmidt SK. Vicuña dung gardens at the edge of the cryosphere. Ecology. 2021;102(2):e03228. doi:10.1002/ecy.3228. [Google Scholar] [PubMed] [CrossRef]
28. Franzluebbers AJ, Stuedemann JA. Surface soil changes during twelve years of pasture management in the Southern Piedmont USA. Soil Sci Soc Am J. 2010;74(6):2131–41. doi:10.2136/sssaj2010.0034. [Google Scholar] [CrossRef]
29. Liang D, Lamb EG, Zhang S. Yak dung deposition affects litter mixing effects on mass loss in Tibetan Alpine Grassland. Rangel Ecol Manag. 2019;72(2):405–10. doi:10.1016/j.rama.2018.11.004. [Google Scholar] [CrossRef]
30. Washaya S, Washaya DD. Benefits, concerns and prospects of using goat manure in Sub-Saharan Africa. Pastoralism. 2023;13(1):28. doi:10.1186/s13570-023-00288-2. [Google Scholar] [CrossRef]
31. Aguirre MG. Combustibles leñosos empleados en la localidad de Antofagasta de la Sierra (Catamarca, Argentina). Bol Soc Argent Botánica. 2020;55:1–10 (In Spanish). [Google Scholar]
32. Papanikolaou AD, Fyllas NM, Mazaris AD, Dimitrakopoulos PG, Kallimanis AS, Pantis JD. Grazing effects on plant functional group diversity in Mediterranean shrublands. Biodivers Conserv. 2011;20(12):2831–43. doi:10.1007/s10531-011-0112-2. [Google Scholar] [CrossRef]
33. Li N, Chang R, Jiang H, Tariq A, Sardans J, Peñuelas J, et al. Combined livestock grazing-exclusion and global warming decreases nitrogen mineralization by changing soil microbial community in a Tibetan Alpine Meadow. CATENA. 2022;219(12-13):106589. doi:10.1016/j.catena.2022.106589. [Google Scholar] [CrossRef]
34. Mu J, Zeng Y, Wu Q, Niklas KJ, Niu K. Traditional grazing regimes promote biodiversity and increase nectar production in Tibetan Alpine Meadows. Agric Ecosyst Environ. 2016;233(1):336–42. doi:10.1016/j.agee.2016.09.030. [Google Scholar] [CrossRef]
35. Niu K, He JS, Zhang S, Lechowicz MJ. Grazing increases functional richness but not functional divergence in Tibetan Alpine Meadow plant communities. Biodivers Conserv. 2016;25(12):2441–52. doi:10.1007/s10531-015-0960-2. [Google Scholar] [CrossRef]
36. Martínez JG. Sociedades prehispánicas de la Puna argentina: desde el poblamiento temprano hasta los inicios de la producción pastoril y agrícola. In: Grau HR, Babot MJ, Izquierdo AE, Grau A, editors. La Puna Argentina: Naturaleza y Cultura. Tucuman, Argentina: Fundación Miguel Lillo; 2018. pp. 273–94 (In Spanish). [Google Scholar]
37. Cingolani AM, Noy-Meir I, Renison DD, Cabido M. La ganadería extensiva, ¿es compatible con la conservación de la biodiversidad y de los suelos? Ecol Austral. 2008;18:253–71 (In Spanish). [Google Scholar]
38. de Knegt HJ, Groen TA, van de Vijver CADM, Prins HHT, van Langevelde F. Herbivores as architects of savannas: inducing and modifying spatial vegetation patterning. Oikos. 2008;117(4):543–54. doi:10.1111/j.0030-1299.2008.16403.x. [Google Scholar] [CrossRef]
39. Price JN, Sitters J, Ohlert T, Tognetti PM, Brown CS, Seabloo EW, et al. Evolutionary history of grazing and resources determine herbivore exclusion effects on plant diversity. Nat Ecol Evol. 2022;6:1290–8. doi:10.1038/s41559-022-01809-9. [Google Scholar] [PubMed] [CrossRef]
40. Zhang M, Delgado-Baquerizo M, Li G, Isbell F, Wang Y, Hautier Y, et al. Experimental impacts of grazing on grassland biodiversity and function are explained by aridity. Nat Commun. 2023;14:5040. doi:10.1038/s41467-023-40809-6. [Google Scholar] [PubMed] [CrossRef]
41. Adler PB, Milchunas DG, Sala OE, Burke IC, Lauenroth WK. Plant traits and ecosystem grazing effects: comparison of US Sagebrush steppe and Patagonian steppe. Ecol Appl. 2005;15:774–92. doi:10.1890/04-0231. [Google Scholar] [CrossRef]
42. Fernandez-Gimenez ME. The role of Mongolian nomadic pastoralists’ ecological knowledge in rangeland management. Ecol Appl. 2000;10:1318–26. doi:10.1890/1051-0761(2000)010[1318:TROMNP]2.0.CO;2. [Google Scholar] [CrossRef]
43. Sharifian A, Gantuya B, Wario HT, Kotowski MA, Barani H, Manzano P, et al. Global principles in local traditional knowledge: a review of forage plant-livestock-herder interactions. J Environ Manage. 2023;328:116966. doi:10.1016/j.jenvman.2022.116966. [Google Scholar] [PubMed] [CrossRef]
44. Dorrough J, Yen A, Turner V, Clark SG, Crosthwaite J, Hirth JR. Livestock grazing management and biodiversity conservation in Australian temperate grassy landscapes. Aust J Agric Res. 2004;55(3):279–95. doi:10.1071/AR03024. [Google Scholar] [CrossRef]
45. Rengifo Vázquez G. La enseñanza es estar contento. In: Educación y afirmación cultural andina. Lima: Proyecto Andino de Tecnologías Campesinas; 2003 (In Spanish). [Google Scholar]
Cite This Article
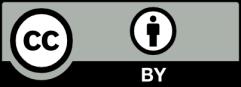
This work is licensed under a Creative Commons Attribution 4.0 International License , which permits unrestricted use, distribution, and reproduction in any medium, provided the original work is properly cited.