Open Access
ARTICLE
Rhizobium Inoculation and Micronutrient Addition Influence the Growth, Yield, Quality and Nutrient Uptake of Garden Peas (Pisum sativum L.)
1 Horticulture Research Centre, Bangladesh Agricultural Research Institute, Gazipur, 1701, Bangladesh
2 Soil Science Division, Bangladesh Agricultural Research Institute, Gazipur, 1701, Bangladesh
3 Irrigation and Water Management Division, Bangladesh Agricultural Research Institute, Gazipur, 1701, Bangladesh
4 Regional Agricultural Research Station, Bangladesh Agricultural Research Institute, Cumilla, 3500, Bangladesh
5 Department of Biology, College of Science, Princess Nourah Bint Abdulrahman University, P. O. Box 84428, Riyadh, 11671, Saudi Arabia
6 Department of Science and Technology, University College‑Ranyah, Taif University, P. O. Box 11099, Taif, 21944, Saudi Arabia
7 Department of Economic Entomology and Pesticides, Faculty of Agriculture, Cairo University, Giza, 12613, Egypt
8 Department of Genetics, Faculty of Agriculture, Cairo University, Giza, 12613, Egypt
9 Department of Biology, College of Science, Taif University, Taif, 21944, Saudi Arabia
10 Division of Soil Science, Bangladesh Wheat and Maize Research Institute, Dinajpur, 5200, Bangladesh
* Corresponding Author: Akbar Hossain. Email:
(This article belongs to the Special Issue: Microbial Biotechnology Applications on Plant Performance and Physiology for Sustainable Production )
Phyton-International Journal of Experimental Botany 2024, 93(5), 901-922. https://doi.org/10.32604/phyton.2024.049226
Received 31 December 2023; Accepted 29 March 2024; Issue published 28 May 2024
Abstract
Garden pea productivity and qualities are hampered in zinc (Zn), boron (B), and molybdenum (Mo) deficient soil. Thus, the combination of micronutrients (i.e., Zn, B, and Mo) and rhizobium is necessary to increase the productivity and quality of garden peas, since this management for garden peas is neglected in Bangladesh. Therefore, the present study was made to assess the effectiveness of rhizobium inoculant singly or in combination with the micronutrients (i.e., Zn, B, and Mo) on growth, yield, nutrient uptake, and quality of garden peas. Treatments were: T = Control, T = Rhizobium inoculation at 50 g/kg seed, T = T + ZnMo, T = T + BMo, T = T + ZnB, T = T + ZnBMo and T = ZnBMo. All treatments were arranged in a randomized complete block design and repeated all treatments in three times. The application of 3 kg Zn, 2 kg B, and 1 kg Mo ha with inoculation of Rhizobium at 50 g kg seed (T) facilitated to increase of 44.8% in the green pod and 29.7% seed yield over control. The same treatment contributed to attaining the maximum nodulation (25.3 plant), Vitamin C (43.5 mg 100 g), protein content (22.2%), and nutrient uptake as well as accumulation in garden peas. Among all treatment combinations, treatment T was found superior to others based on microbial activities, soil fertility, and profitability. The results of the study found that the application of 3 kg Zn, 2 kg B, and 1 kg Mo ha in combination with Rhizobium inoculation (50 g kg seed) can improve the yield and quality of garden peas. The results of the study have the potential for the areas, where there is no use of Rhizobium inoculant or Zn, B, and Mo fertilizer for cultivation of garden pea.Keywords
The increasing global population is a great challenge for ensuring food and nutritional security in the current era of climate change [1]. Additionally, declining soil health with time is another challenge for achieving higher crop productivity [2]. Therefore, it is inevitable that the food demands of the increasing population will be met by increasing agricultural productivity [3]. Garden pea is a legume crop that can play a key role in attaining food and nutritional security [4]. It is enriched with high protein and carbohydrate contents [5] and is also a rich source of minerals and antioxidants [3]. Garden pea cultivation is convenient for conserving soil fertility through biological nitrogen fixation [6]. Legume crops have their own physiological and morphological features to procure nitrogen via symbiotic bacteria [7], which require an appropriate amount of essential micronutrients (Zn, B, and Mo) and biofertilizers for improved growth and development [6]. Mostly, inequity and excess chemical fertilizer, particularly nitrogenous fertilizer application, increase crop production but affect the ecological balance, deteriorating soil, water, and human health [8,9]. Thus, the excessive use of inorganic fertilizers is desirable in advanced technologies to reduce production costs and save the environment and human health [10]. Consequently, the integration of an appropriate amount of micronutrients (zinc, boron, and molybdenum) with Rhizobium inoculant is crucial and has emerged as an innovative technology for ensuring garden pea growth and yield and sustaining soil fertility [11,12].
Rhizobium plays a vital role in improving soil health by influencing the growth and development of plants through the formation of root nodules and N fixation [13–15]. Rhizobium inoculants support the ability of NPK and other plant nutrients to be absorbed by crops, triggering the growth and development of plants and resulting in increased crop yields [16,17]. Several researchers have reported that the yield of several crops can increase by approximately 25% due to the influence of Rhizobium and that the use of N-and P-based fertilizers can decrease the yield by approximately 25% to 50%, respectively [18,19]. Other studies have shown that the use of effective Rhizobia strains can enhance legume productivity [13,20].
Zinc (Zn), boron (B), and molybdenum (Mo) are the three most deficient micronutrients in the acidic and calcareous soils of South Asia, including Bangladesh and India, and their deficiencies in crop productivity have been reported to be approximately 45%, 46%, and 31%, respectively [21]. Earlier findings also reported that Zn, B, and Mo are crucial for enhancing the fixation of N in legumes [22,23]. Among these micronutrients, Zn is also involved in metabolism as well as biological N fixation through the formation of nodules [24]. In addition, Zn influences the water uptake capacity of plants and transports nutrients, leading to increased crop productivity and reducing the adverse effects of various stresses [25,26]. Zn is also required in plants for hormone biosynthesis [27] and physiological processes of protein synthesis [24]. B is involved in plant cell wall formation and stabilizes nodular cell walls and membrane stability [28,29]. B assists in increasing the flowering, pollen germination, and growth of pollen tubes, pod setting, and yield of legumes [30]. It is beneficial for chlorophyll synthesis and carbohydrate metabolism [31]. B stimulates the uptake and acquisition of macronutrients by plants, particularly N, P, and K [32]. On the other hand, Mo has a significant role in proper plant metabolic functioning and is involved in enzymatic activities such as cofactors for the Rhizobium nitrogenase enzyme [33]. It helps in N acclimatization and the synthesis of phytohormones [33]. Mo application increases the canopy, nodulation, and yield of crops [34].
The present study aimed to determine whether the application of a single Rhizobium inoculant or a combination of Rhizobium inoculants with micronutrients (Zn, B, and Mo) enhances the growth, yield, nodulation, nutrient uptake, and quality of garden peas. This study also investigated economic returns and soil microbes.
2.1 Experimental Location, Soil and Climate
The study was carried out during the winter seasons of 2019–20 and 2020–21 at the Horticulture Research Centre (HRC), Bangladesh Agricultural Research Institute (BARI), Joydebpur, Gazipur 1701, Bangladesh. The study site was located in agro-ecological zone 28 (Madhupur tract) (23°98´N latitude and 90°41´E longitude and is 8.4 m above sea level). The soil type of the experimental region was a grey terrace that was chemically acidic and belonged to the Chhiata soil series (Texonomy: Aeric Haplaquepts) [35]. Texturally, the soil was clay loam (30.38% sand, 35.20% silt, and 34.42% clay) with a pH of 6.2%, 1.29% organic matter, 0.073% total N, 12.0 ppm P, 0.14 meq./100 g soil K, 15.0 ppm S, 0.78 ppm Zn, and 0.14 ppm B. The particle density, bulk density, and porosity of the soil were 2.55 g/cc, 1.38 g/cc, and 46.0%, respectively. The field capacity (FC) was 26.8% of the gravimetric water content, and the cation exchange capacity (CEC) was 7.4 Cmol kg−1 soil. The physicochemical properties of the presowing and postharvest soils were analyzed by standard methods [36].
The climate conditions of the study site are characterized as subtropical and humid. The monthly average temperatures during the study period ranged from 13.0°C to 36.1°C, and the monthly rainfall ranged from 0 to 218 mm. The maximum and minimum monthly average temperature, humidity, and rainfall during the experimental periods (2019–20 and 2020–21) were recorded and are presented in Figs. 1a and 1b.
Figure 1: Monthly average minimum and maximum air temperature, rainfall and monthly average humidity during the experimental periods of 2019–20 (a) and 2020–21 (b)
2.2 Land Preparation and Layout
The experimental soils were opened by a tractor-driven plough with 4 passes, and a tractor-driven rotavator was also used for uniform tilling and levelling. The soil was manually cleaned to remove weeds and stubbles. The treatments were T1 = Control (no Zn, B, Mo or Rhizobium inoculant), T2 = Rhizobium inoculation (50 g kg−1 seed), T3 = Zn3Mo1 + Rhizobium inoculant as applied in T2, T4 = B2Mo1 + Rhizobium inoculant as applied in T2, T5 = Zn3B2 + Rhizobium inoculant as applied in T2, T6 = Zn3B2Mo1 + Rhizobium inoculant as applied in T2 and T7 = Zn3B2Mo1. All treatments were arranged in a randomized complete block design and repeated three times.
The other common fertilizers were applied in all plots in elemental form (i.e., N, P, K and S at 40, 24, 50 and 10 kg ha−1, respectively) along with 5 t ha−1 decomposed cow dung. The sources of N, P, K, S, Zn, B and Mo were urea, TSP, MoP, gypsum, zinc sulphate (monohydrate), boric acid, and ammonium molybdate fertilizers, respectively. The peat-based Rhizobium inoculum (rhizobium strain BARI RPs 504) was used at a rate of 50 g kg−1 seed, which was prepared and supplied by the Soil Science Division of BARI. The experimental plots were divided into three equal blocks consisting of seven-unit plots in each block. The size of each unit plot was 3 m × 2 m. The unit plots were separated by 50 cm gaps, and the replicated blocks were separated from each other by a distance of 1 m.
2.3 Isolation of Rhizobium and Biochemical Tests
The Rhizobium inoculum (strain) was isolated from clean and freshly collected healthy root nodules of Garden pea. The selected root nodules were cleaned with distilled water and sterilized several times with sodium hypochlorite (NaOCl) solution. The sterilized nodules were transferred to Petri dishes and then crushed with the help of a sterilized glass rod to obtain a milky suspension, which was subsequently streaked on a yeast extract mannitol agar (YEMA) plate. The isolate was subcultured on a series of YEMA plates for pure cultures of the Rhizobium strain. The pure culture was maintained on YEMA slants and incubated at 28 ± 2°C for 48 h. The slants were preserved in a refrigerator at 4°C after sufficient growth [37] for further investigation.
The identified isolate (Rhizobium) was confirmed by several tests, including Gram staining and growth on YEMA with congo red. Nodulation ability was also tested on homologous hosts by plant infection tests according to the technique of Somasegaran et al. [38]. A sterilized inoculation loop was used for the entire test. The isolate was inoculated into broth media and kept for 3–5 days in a rotary shaker for good growth. The growth culture (20 ml suspension) was inoculated into 100 g of sterilized peat soil made of poly pack, which was subsequently incubated for 5–7 days at 28 ± 2°C before field application.
2.4 Plant Material, Fertilizer Application and Seed Sowing
Seeds of garden pea (BARI Motorshuti-3) were obtained from the Olericulture Division of HRC, BARI, Gazipur 1701, Bangladesh. Cowdung at 5 t ha−1 and other common fertilizers, including half of the urea and half of the MoP, were applied basally during the final plot preparation. Full amounts of zinc sulphate, boric acid and ammonium molybdate were applied plotwise as per prescribed treatments. The applied fertilizers were mixed thoroughly into the soil. The peat soil carrier-based Rhizobium inoculant was coated gently with healthy dry seeds at a rate of 50 g kg−1 seed, and the seeds were placed under shade for a few minutes for air drying before sowing. The plots with uninoculated seeds were sown first to avoid contamination, and then, the plots with inoculated seeds were sown at 120 kg ha−1 with a spacing of 20 cm × 10 cm on 09 December 2019 and 10 December 2020. The remaining half of the urea and MoP was applied between the rows as banding at 25 days after sowing (DAS), and it was mixed properly with the soil.
As the soil was dry, postsowing irrigation was performed immediately after seed sowing to facilitate proper germination. Each plot was irrigated with a hosepipe starting at 7 DAS at regular intervals of 7–10 days based on the soil moisture. The crop was manually weeded twice, at 25 and 40 DAS. The fungicides Provax 200 wp and distance M-45 were sprayed with 2 g/L water at 35 and 45 DAS to control root rot and brown spot disease, respectively. The infestation of insects such as pod borers and aphids was minimized by spraying 0.5 ml/L water with Imitaf 20 SL at 35 and 45 DAS. The test crop was harvested plotwise on two dates at two stages. The first half was harvested at the green pod stage, and the second half was harvested at the dry seed stage. Green pods were harvested at the tender stage on 04 February 2020 and 05 February 2021 from four rows in each plot. The remaining crop in the plot was allowed to grow until maturity, and mature pods were harvested for dry seed yield. The perfect maturity of the garden peas indicated that when the plants and pods turned brown, the seeds became hard.
Active modulation data were calculated from five randomly selected plants in each plot at 50 DAS. Five plants were uprooted smoothly from each plot with the help of a hand hoe and were carefully washed and dried under normal conditions. The lengths of the roots of five plants were measured, and the number of root nodules on each plant was counted and averaged. Ten nodules were randomly selected from five plants of each treatment to measure the individual nodule length, diameter, and individual nodule weight, and the values were averaged. The detached pods of four rows in each plot were weighed by an electric balance to determine the yield of the green pods, which was converted to kg ha−1. The green seeds were separated from the composite pods of four rows to measure the weight of the 100-green seeds. Fresh green pod samples (250 g) from each treatment were collected and preserved in a refrigerator at −30°C in the postharvest laboratory of the HRC of BARI for quality analysis. Total soluble solids (TSSs), titratable acidity, and vitamin C content were recorded following standard methods. The total soluble solid content was assessed by taking a drop of green seed juice on a hand refractometer glass lens (Atago Ltd., PAL-1, Tokyo, Japan), and the results are expressed in 0Brix as described by Anonymous [39]. The titratable acidity was determined according to the accepted method of Ranganna [40]. The level of vitamin C (ascorbic acid) was estimated from a standard method [39]. After the maturity of the remaining crop in each treatment plot, the growth and yield contributing characteristics, viz., plant height, number of branches plant−1, number of pods plant−1, pod length, number of seeds pod−1, and 100-seed weight, were recorded for five randomly selected plants. Data on the dry seed and straw yields were recorded from the remaining mature test crops and converted to kg ha−1.
Treatment basis straw and seed samples were oven-dried at 70°C for 48 h and ground with a CyclotecTM 1093 sample mill (Made in Sweden). The ground straw and seed samples were digested by a diacid mixture (HNO3-HClO4) (5:1) according to the method described by Piper (1966) [41]. The digested mixtures (straw and seed) were utilized to determine the N content following the Micro-Kjeldahl method [42], the P content via the spectrophotometer method, the K content via the atomic absorption spectrophotometer method and the S content via the turbidity method via BaCl2 via the spectrophotometer. The Zn content of the digest was directly measured by atomic absorption spectroscopy (VARIAN SpectrAA 55B, Australia). The B content in the digest was determined by a spectrophotometer through the azomethine-H method [36]. The Mo concentration data are not shown due to a lack of laboratory facilities.
The seed protein content of garden peas was measured after multiplying the N content by the constant food factor of 6.25 [43].
Dry crop yields and nutrient contents in seeds and dry plants (straw) were used to determine nutrient (N, P, K, S, Zn and B) uptake according to the following formula [44]:
2.8 Preparation and Isolation of Microbes
Postharvest soil samples were collected by standard procedures at a soil depth of 0–15 cm. Total Rhizobium, free-living bacteria, phosphate-solubilizing bacteria (PSB), actinomycetes and fungal colonies were grown in different prepared media. After serial dilution, one drop of solution was poured into a Petri dish with different types of media. The Petri plates were incubated for three days to count total bacteria, Rhizobium, free-living bacteria, PSB, actinomycetes and fungal colonies. Bacterial media were prepared as nutrient agar media containing 28 g/L distilled water up to 1.00 L. The ingredients of the media were mixed in a normal glass flask with the required amount of distilled water. The initial pH of the medium was adjusted to 7.0. The medium was dissolved by boiling and autoclaved at 1210°C for 15 min. YEMA (yeast mannitol agar) medium contained the following ingredients: 0.5 g of K2HPO4, 0.2 g of MgSO4·7H2O, 0.1 g of NaCl, 0.2 g of CaCl2·6H2O, 0.01 g of FeCl3·6H2O, 10 g of mannitol, 0.5 g of yeast extract, 15.00 g of agar powder, and 1 L of distilled water. The initial pH of the medium was adjusted to 7.0 by adding 0.1 N HCl solution. The agar used in this medium was dissolved by boiling, and the medium was autoclaved at 1210°C for 15 min. PSB media plates were prepared with Pikovaskya’s medium. Composition of 1 l of Pikovskaya media: 10 g of glucose, 5 g of Ca3(PO4)2, 0.5 g of (NH4)2SO4, 0.2 g of NaCl, 0.1 g of MgSO4·7H2O, 0.2 g of KCl, 0.5 g of yeast extract, and 0.5 g of MnSO4. H2O (0.002 g), FeSO4.7H2O (0.002 g) and agar (15 g) were used [45].
Actinomycete agar media: Plates were prepared with Actinomycete agar media. The composition of the Actinomycetes agar medium in 1 l was 21.7 g of Actinomycetes agar with 5 ml of glycerol. The initial pH of the medium was adjusted to 7.0 by adding 0.1 N HCl solution.
PDA (potato dextrose agar) media: The plates were prepared with PDA media. The amount of PDA medium in 1 litre was 39 g. The initial pH of the medium was adjusted to 7.0 by adding 0.1 N HCl solution. The agar used in this medium was dissolved by boiling, and the medium was autoclaved at 1210°C for 15 min. After autoclaving, 10 ml of lactic acid was mixed per litre of media. Nitrogen-free bacteria (NFB) media: Plates were prepared with nitrogen-free bacterial media. The nitrogen-free bacterial media included 5 g of malic acid, 0.5 g of K2HPO4, 0.1 g of NaCl, 0.2 g of MgSO4.7H2O, 0.02 g of CaCl2, 5% BTB in 2 ml of 0.02 N KOH, 8 ml of 1.64% FE-EDTA and 20 g of agar. The initial pH of the media was adjusted to 7.0 by adding 1 N KOH solution. The agar used in this medium was dissolved by boiling, and the medium was autoclaved at 12°C for 15 min.
2.9 Estimation of the Benefit-to-Cost Ratio
The green pod and dry seed yield of garden peas were used to compute the gross return per hectare of land. The gross return was calculated after multiplying the yield by the farmgate unit price of the green pod and dry seed.
The gross margin was calculated by subtracting the total variable cost from the gross return according to the following formula:
Treatment total variable cost was measured by adding the cost incurred for labourers, ploughing and inputs of each treatment. The shadow prices (land rent, straw cost, etc.) were not considered.
The benefit-cost ratio (BCR) was calculated from the gross return divided by the total variable cost of cultivation. The BCR formula is as follows:
Data on growth, yield and yield attributes, quality characteristics, nutrient content and nutrient uptake were subjected to two-way ANOVA by using SAS software (version 9.4). The microbial population data in postharvest soil were analysed by analysis of variance (ANOVA) using SAS software (version 9.4). The mean separation test for all the data were was performed by using Tukey’s HSD test at the 0.05 (p ≤ 0.05) level of probability.
3.1 Yields of Garden Pea Influenced by Rhizobium and Micronutrients
The year 2020–21 was found to be more favourable for the growth and development of garden peas, resulting in significantly greater green pod and seed yields from 2019–20 (Table 1). The use of a single Rhizobium inoculant or combined with Zn, B and Mo fertilizers had a positive influence on the green pod, seed, and straw yields of garden peas (Table 1). An increase in the green pod yield (8691 kg ha−1) was recorded in the T6 treatment group, which was significantly greater than that in the other treatment groups. Similarly, the T6 treatment resulted in greater seed yield (1869 kg ha−1) than did the T7, T5 and T3 treatments. Both the green pod and seed yields were lowest in the control (T1) treatment (Table 1). The highest straw yield (1770 kg ha−1) was achieved in the T6 treatment, followed by the T7, T5 and T3 treatments (Table 1). However, compared with the control treatment, the combined application of the rhizobium inoculant and micronutrients (Zn, B and Mo) resulted in a 44.8% greater percentage of green pods and a 29.7% greater seed yield (Table 1).
3.2 Growth and Yield Contributing Characteristics of Garden Pea as Influenced by Rhizobium and Micronutrients
The year of cultivation affected the plant height, number of branches per plant and number of pods per garden pea plant (Table 2). The experimental results indicated that the tallest plant (40.4 cm), the greatest number of branches per plant (5.54) and the greatest number of pods per plant (8.05) were recorded in 2020–21, while the lowest values were recorded in 2019–20. The application of Zn, B and Mo with Rhizobium inoculation had a significant influence on the growth and yield of garden peas (Table 2). The tallest plant (42.1 cm) was found in the T6 treatment, which was comparable to the T7, T3 and T4 treatments, while the greatest number of branches per plant (5.69) was also found in the T6 treatment, followed by the T7, T5, T4 and T3 treatments. The lowest values of both parameters were detected in the control treatment (T1) (Table 2). The increase in pod length (6.39 cm) in the T6 treatment was comparable to that in the T5 and T7 treatments, although the greatest number of pods per plant (8.80) was also recorded after the application of 3 kg Zn, 2 kg B and 1 kg Mo ha−1 with Rhizobium inoculation (50 g kg−1 seed) (T6), which was significantly equal to that in the T7 treatment, and the lowest values of both parameters were observed in the control T1 treatment. Significantly, the maximum number of seeds per pod (6.11) was obtained in T6, while the minimum value (4.85) was obtained in the control treatment. The 100 g green seed and dry seed weights reached a maximum of 55.6 and 27.1 g, respectively, in the T6 treatment, both of which were significantly different from those in the other treatments. However, the performance of T6 was statistically identical to that of T7 for 100-green seed weights and that of T7, T5, and T3 for dry seed weights. Both the green pod and dry seed weights were minimal in the control treatment (Table 2).
3.3 Root Nodulation of Garden Pea Plants Influenced by Rhizobium and Micronutrients
The year of cultivation affected only the length of the roots of the garden pea plants. The greatest root length (12.4 cm) was observed in 2020–21, and the lowest (11.7 cm) was in 2019–20 (Table 3). The root length, active nodulation, nodule length and diameter and nodule weight of garden peas significantly responded to the application of Zn, B and Mo combined with Rhizobium inoculation (Table 3). The longest root (12.7 cm) was noted in T6, which was comparable to most of the treatments, while the lowest value was observed in the control plot. In the present study, 50 days after sowing, more active nodules per plant (25.3) were recorded in the T6 treatment than in the other treatments, which was statistically similar to most of the treatments except for the control. However, the nodule length was greater (3.74 mm) in the same T6 treatment group than in the T7, T5 and T3 treatment groups. The greatest nodule diameter (2.67 mm) was found in the T6 treatment group. In terms of nodule weight, the heaviest nodule (0.0076 g) was noted in the T6 treatment group, which was comparable to that in the T7 and T5 treatment groups, but the control treatment group had the lightest (0.004 g) nodule (Table 3).
3.4 Effect of Rhizobium and Micronutrients on the Quality of Garden Pea
The vitamin C and protein contents were significantly different between the two years. The highest content of vitamin C (42.7 mg 100 g−1) and maximum protein content (21.7%) of garden peas were attained in 2020−21, and both were lower in 2019−20 (data not shown). The quality traits, viz., TSS, titratable acidity, vitamin C content and protein content, of garden peas, responded positively to the application of Zn, B and Mo combined with Rhizobium inoculation (Fig. 2).
Figure 2: Total soluble solids (TSSs), titratable acidity, vitamin C content and protein content of garden pea affected by the application of Zn, B and Mo with a rhizobium inoculant. Error bars represent the mean ± standard error of the mean (n = 3). The means indicated by the different letters in the bars are significantly different at the 5% level according to Tukey’s honestly significant difference (HSD) test. T1 = Control, T2 = Rhizobium inoculation, T3 = Zn3Mo1 + T2, T4 = B2Mo1 + T2, T5 = Zn3B2 +T2, T6 = Zn3B2Mo1 + T2, T7 = Zn3B2Mo1
The highest total soluble solid content (0Brix 14.3) was detected in T6, which was comparable to most of the treatments, and the minimum value (0Brix 12.3) was detected in the control treatment (Fig. 2). The value of titratable acidity was highest (1.31%) in the treatments with 3 kg Zn and 2 kg B ha−1 Rhizobium inoculation (T5), which was statistically similar to that in the T6, T4 and T2 treatments (Fig. 2). The highest amount of vitamin C (43.5 mg 100 g−1) was found in the T6 treatment, which was statistically similar to most of the treatments, and the lowest amount (39.7 mg 100 g−1) was found in the T1 treatment (Fig. 2). The same T6 treatment resulted in greater protein content (22.2%), which was statistically identical to that of all other treatments except the control (Fig. 2).
3.5 Rhizobium and Micronutrients Influence the Nutrient Content of Garden Pea Seeds
The year of cultivation affected the nitrogen (N), potassium (K), sulphur (S), zinc (Zn) and boron (B) contents in the seeds of garden peas but not the phosphorus (P) content (Table 4). In the second year (2020-21), relatively high N (34.7 g kg−1), K (11.2 g kg−1), S (3.90 g kg−1), Zn (0.038 g kg−1) and B (0.041 g kg−1) levels were detected in the seeds, while all of these values decreased in the first year (2019–20) (Table 4). The application of Zn, B and Mo combined with Rhizobium inoculation affected the nitrogen, phosphorus, potassium, sulphur, zinc and boron contents in the seeds of the garden peas (Table 4). A significant increase in the nitrogen content (35.5 g kg−1) was detected in T6, similar to most of the treatments, although the minimum nitrogen content was detected in the T1 (control) treatment. The maximum phosphorus content (8.86 g kg−1) was comparable between the T6 treatment and the T5 and T7 treatments. The highest potassium content (13.3 g kg−1) was in the T6 treatment, while the lowest potassium content (6.91 g kg−1) was in the control treatment. The sulphur content measured at the highest level (6.63 g kg−1) in the same T6 treatment was statistically similar to that in the T7 and T5 treatments, whereas it was lower (2.61 g kg−1) in the T1 treatment (control). The maximum zinc content in the seeds (0.042 g kg−1) was noted in T6, which was statistically similar to that in the T7 and T5 treatments. The same T6 treatment exhibited an increase in the content of boron (0.045 g kg−1), which was statistically similar to that in the T7 treatment. Both the Zn and B contents were minimal in the T1 (control) treatment (Table 4).
3.6 Nutrient Contents in Straw Influenced by Rhizobium Inoculant and Micronutrients
The year of cultivation affected the nitrogen, potassium, sulphur and boron contents but not the phosphorus and zinc contents in the straw of garden peas (Table 5). Increased contents of nitrogen (14.3 g kg−1), potassium (15.3 g kg−1) and sulphur (3.04 g kg−1) were detected in 2020–21, while the contents of all nutrients decreased in 2019–20 (Table 5).
Table 5 shows that the increased nitrogen content in straw (14.8 g kg−1) in T6 was comparable to that in most of the treatments, although a lower nitrogen content (12.7 g kg−1) was detected in the control treatment. The T6 treatment resulted in a higher phosphorus content (5.42 g kg−1) that was statistically identical to that of the T7, T5 and T4 treatments, while the control treatment was responsible for the minimum phosphorus content (3.81 g kg−1). The maximum potassium content (16.6 g kg−1) was recorded in the T6 treatment, which was comparable to that in the T7, T5 and T4 treatments, although the minimum potassium content (11.6 g kg−1) was recorded in the T1 treatment. An increase in the sulphur content (3.52 g kg−1) was also detected in the T6 treatment group, followed by the T7, T5 and T4 treatments, while a decrease in the sulphur content (1.81 g kg−1) was detected in the control group (T1). Significantly, the maximum zinc (0.0427 g kg−1) and boron (0.0387 g kg−1) contents were detected in T6, while both were minimal in the control treatment (Table 5).
3.7 Effect of Zn, B and Mo Combined with Rhizobium Inoculant on Total Nutrient Uptake by Garden Pea
Figs. 3a and 3b show the effect of micronutrients (Zn, B & Mo) combined with rhizobium inoculation on the total uptake of different nutrient elements by garden peas. The cultivation year affected the total uptake of N, P, K, S, Zn and B by garden pea (seed + straw). The second year (2020–21) favored the maximum total uptake of N (86.6.0 kg ha−1), P (22.2 kg ha−1), K (45.9 kg ha−1), S (12.2 kg ha−1), Zn (0.132 kg ha−1) and B (0.131 kg ha−1), although the minimum uptake of all nutrients occurred from 2019–20 (data not shown).
Figure 3: (a) Total uptake of N, P, K and S and (b) total uptake of Zn and B by garden pea (seed + straw) as affected by the application of Zn, B and Mo with Rhizobium inoculant. Error bars represent the mean ± standard error of the mean (n = 3). The means indicated by the different letters in the bars are significantly different at the 5% level according to Tukey’s honestly significant difference (HSD) test. T1 = Control, T2 = Rhizobium inoculation, T3 = Zn3Mo1 + Rhizobium, T4 = B2Mo1 + Rhizobium, T5 = Zn3B2 + Rhizobium, T6 = Zn3B2Mo1 + Rhizobium, T7 = Zn3B2Mo1
The highest total uptake of nitrogen (92.5 kg ha−1) was detected in the treatments involving the application of 3 kg Zn, 2 kg B and 1 kg Mo with Rhizobium inoculation (50 g kg−1 seed) (T6), which was statistically similar to the T7 treatment, while the lowest total uptake value (62.7 kg ha−1) was detected in the control treatment. Compared with the T7 treatment, the T6 treatment was more effective at attaining the greatest phosphorus uptake (26.2 kg ha−1) in garden peas, although the control treatment (T1) had the lowest P uptake (Fig. 3a). The highest total potassium uptake (54.3 kg ha−1) was obtained from the T6 treatment, while the lowest was from the T1 treatment (control). The maximum sulphur uptake by garden pea (14.9 kg ha−1) in the T6 treatment was comparable to that in the T7 treatment, while the minimum was in the T1 (control) treatment (Fig. 3a). The greatest zinc (0.154 kg ha−1) and boron uptake (0.153 kg ha−1) were recorded in T6, which were significantly greater than those in the other treatments but were statistically similar to those in T7. Both Zn and B uptake were the lowest in the T1 (control) treatment (Fig. 3b).
3.8 Effect of Zn, B and Mo Combined with Rhizobium Inoculation on the Microbial Population in Soil
Zn, B and Mo combined with Rhizobium inoculation affected Rhizobia, total bacteria, fungi, actinomycetes, PSB and free-living bacteria in the postharvest soil of garden peas (Table 6). In the case of Rhizobia, a significantly increased population (600 × 105 cfu/g soil) was found in T6, while a decreased population was noted in the T1 (control) treatment.
The greatest population of total bacteria (3300 × 105 cfu/g soil) was detected in the T4 treatment, and the lowest population (2.0 × 105 cfu/g soil) was detected in the T1 treatment (control). Significantly, the maximum fungal population (5400 × 105 cfu/g soil) was detected in the T6 treatment, while the minimum fungal population was detected in the T7 and T2 treatments. The population of actinomycetes reached a maximum (1000 × 105 cfu/g soil) in T6, which was significantly greater than that in the other treatments. However, the actinomycete population was least abundant in the T2 treatment (Table 6). The largest population of phosphate-solubilizing bacteria (400 × 105 cfu/g soil) was observed in the T2 and T6 treatments, which was comparable to that in the T3 and T7 treatments, and a smaller population was observed in the T5 treatment. The highest population of free-living bacteria (4200 × 105 cfu/g soil) was recorded in the T6 treatment, followed by the T3 treatment, and the lowest population (11 × 105 cfu/g soil) was registered in the T1 (control) treatment (Table 6).
3.9 Cost and Return Analysis Due to the Application of Rhizobium Inoculant and Micronutrients in Garden Pea
The use of Zn, B, and Mo with Rhizobium inoculation had an encouraging effect on the cost and return analysis of garden pea production (Table 7). The maximum gross returns of USD 4240 ha−1 for green pods and USD 1596 ha−1 for dry seeds of garden peas were recorded for the T6 (Zn3B2Mo1 + Rhizobium) treatment, followed by the T7 treatment. The lowest gross return was recorded in the control treatment group. However, the benefit-cost ratio (BCR) was 5.70 for the green pod treatment and 2.29 for the dry seed from the T2 (only rhizobium inoculation) treatment. The lowest BCR of 3.61 for the green pods of garden peas occurred in the T3 treatment, and the minimum BCR of 1.48 for dry seeds occurred in the T4 treatment (Table 7). The decreasing trend of the benefit-cost ratio might be related to the higher market price of Mo-containing fertilizer.
Zinc, boron, and molybdenum are crucial microelements for plant growth, flowering, fruiting, and quality improvement [46]. These micronutrients are also involved in plant physiological, biochemical, and metabolic activities, and their insufficiency promotes growth abnormalities in plants [22,46]. Improvements in garden pea growth, yield, and quality were amplified by the application of Zn, B, and Mo with Rhizobium inoculant. Rhizobia are good sources of biofertilizers that are widely used to enrich nitrogen in soils and plant roots through biological nitrogen fixation [47,48]. The experimental soil was deficient in micronutrients, viz., Zn, B, and Mo. The present study revealed significantly increased garden pea yields (green pod, seed, and straw yield) in response to the combined application of 3 kg Zn, 2 kg B, and 1 kg Mo per hectare combined with Rhizobium inoculation (50 g kg−1 seed). Micronutrients (Zn, B, and Mo) assisted in the translocation of photosynthates, increasing pod formation and quality, which eventually increased the number of green pods and the seed yield of garden peas. A similar result was corroborated by Hossain et al. [49], who reported that the combined application of 3 kg Zn, 2 kg B, and 1 kg Mo per hectare contributed to achieving greater seed and straw yields of lentils. Quddus et al. [50] also reported that the combined application of 3 kg Zn and 2 kg B resulted in the highest seed yield of field peas. Rhizobium can directly influence plant metabolism by solubilizing phosphates and producing hormones, which consequently promotes plant growth and ultimately improves crop yields [51,52]. In the present study, we compared the performance of Rhizobia singly or in combination with micronutrients (Zn, B, and Mo). However, the combined use of Zn, B, and Mo with Rhizobium inoculant significantly increased the seed yield by approximately 29.7% compared with that of the control group and by 14.7% compared with that of the Rhizobium inoculation group. Yadav et al. [51] reported a similar result in chickpeas in which seed yield significantly increased due to the application of phosphorous, zinc, and Rhizobium inoculation compared with the control. In our study, a single application of Rhizobium inoculant contributed to a 13.0% greater increase in seed yield compared to that of the control. Bhuiyan et al. [20] and Tena et al. [13] noted that the use of effective Rhizobia strains can enhance the yield of legume crops. Kumar et al. [10] also reported that plant-associated microbes play a key role in increasing plant biomass and crop yield.
Growth and yield attributes are very important factors for achieving a higher yield of garden peas. However, the combined use of Zn, B, and Mo along with Rhizobium inoculation was more effective than single Rhizobium inoculation or the application of paired micronutrients (ZnB, ZnMo, or BMo) combined with Rhizobium or three micronutrients, excluding Rhizobium inoculation, in terms of the growth and yield of garden pea. The greatest plant height, the greatest number of branches, the maximum number of pods per plant, and 100 seed weight were achieved by the joint application of 3 kg Zn, 2 kg B, and 1 kg Mo per hectare along with rhizobium inoculation at 50 g kg−1 seed. Similarly, Islam et al. [53] reported that the combined application of micronutrients (Zn, B, and Mo) effectively contributed to increasing plant height, the number of branches, and pods per plant, and the number of lentil seeds. Mohanty et al. [3] reported a similar effect in garden peas: the combination of micronutrients with Rhizobium inoculation, and N, P, and K significantly improved the yield attributes and yield. We observed that the combination of Rhizobium inoculant with micronutrients was more effective than a single application of Rhizobium inoculant. However, the results of the experiment demonstrated that the joint application of Rhizobium inoculant with micronutrients (Zn, B, and Mo) contributed 9.0% more to the number of pods per plant than the single use of Rhizobium inoculant. The treatment combination also contributed 3.0% more to the number of pods per plant than did the combined application of Zn, B, and molybdenum fertilizers. The combined application of Rhizobium inoculant with Zn, B, and molybdenum fertilizers might be more effective in influencing the availability of soil nutrients for plant uptake, leading to the generation of more pods and increasing yields of garden peas. The results of the study also revealed that Rhizobium inoculation alone resulted in better growth and yield of garden peas than no inoculation. This result agreed with the observations of Kumar et al. [10] and Tilman et al. [54]. Sayed et al. [5] reported that the inoculation of legume seeds with associative N2-fixing bacteria resulted in improved growth, yield attributes, and yield.
Micronutrients, especially Mo, are crucial constituents of the nitrogen-fixing enzyme nitrogenase, which assists in atmospheric nitrogen fixation and is converted to NH3, which is assimilated by plants [55]. Zinc is also involved in the process of nodule formation [24]. Boron is also beneficial for increasing root length, nodulation, and the size of root nodules [15]. The inoculation of Rhizobium enhances the content of chlorophyll, protein, and nitrogen fixation, and subsequently the rate of photosynthesis, and thus carbohydrate accumulation in the plant. In our study, the combination of 3 kg Zn, 2 kg B, and 1 kg Mo per hectare with Rhizobium inoculation (50 g kg−1 seed) increased the number of nodules per plant, increased root length, and weight, and improved nodule size. The results of the experiment showed that the increase in nodulation was 21.1% greater than that in the control due to the joint application of 3 kg Zn, 2 kg B, and 1 kg Mo per hectare with Rhizobium inoculant. This result is in agreement with the findings of Das et al. [56], who reported that micronutrients combined with Rhizobium sp. improved the nodule number in chickpea plants by 14.3% compared with that in the uninoculated control group. Gupta et al. [57] also reported in chickpeas that the increase in nodule number per plant was related to sufficient Mo application with Rhizobium, phosphate-solubilizing bacteria, and the recommended dose of other fertilizers. Similarly, Allito et al. [58] reported that inoculation with Rhizobium significantly increased nodulation in faba beans. Bejandi et al. [59] observed that the application of micronutrients and chickpea seed inoculation with rhizobium contributed to an increase in the chlorophyll, and protein content in plants. Wang et al. [60] reported that micronutrients combined with Rhizobium inoculation are beneficial for nitrogen fixation, although they are a basic compound of both chlorophyll and protein.
Micronutrient application combined with Rhizobium inoculation might help improve the nutritional quality of garden peas. In our study, greater TSS in the green seeds of garden peas was found with the application of 3 kg Zn, 2 kg B, and 1 kg Mo per hectare with Rhizobium inoculation (50 g kg−1 seed), but the cause of such an effect is unclear. We found that micronutrient application combined with Rhizobium inoculation improved the vitamin C and protein contents in garden pea seeds. However, inoculation of Rhizobium with micronutrients might be favourable for improving vitamin C and protein contents. Similar findings were verified by the reports of Khiangte et al. [15], and Raj et al. [23].
The experimental soil was deficient in Zn, B, and Mo; thus, the test crop was responsive to the application of those micronutrients, which caused increased accumulation and uptake of several nutrients (N, P, K, S, Zn, and B) by the combined application of 3 kg Zn, 2 kg B, and 1 kg Mo per hectare with Rhizobium inoculation (50 g kg−1 seed). However, the joint application of Zn3B2Mo1 + Rhizobium enhanced the nutrient (N, P, K, S, Zn, and B) content in the plants, causing greater uptake of 23.0% N, 43.9% P, 55.1% K, 58.1% S, 46.6% Zn, and 53.0% B in the aboveground part of the garden pea than did the single application of Rhizobium (data not shown). Hossain et al. [49] reported a similar result in lentils in which the uptake of nutrients increased due to the combined application of Zn, B, and Mo fertilizers. Similar results were also reported by Das et al. [56] in chickpeas, who reported that nutrient uptake was greatest after micronutrient application and rhizobium inoculation. In our study, a single application of Rhizobium resulted in better uptake of all nutrients compared to that in the uninoculated plot. Consequently, the combination of Zn, B, and Mo with Rhizobium inoculation was more efficient than single Rhizobium inoculation or noninoculated treatment. These phenomena might be related to the greater seed and straw yields of garden peas with higher nutrient concentrations. Rihana et al. [46] reported that nutrient acquisition and uptake were greater when appropriate essential micronutrients were supplied to appropriate Rhizobium strains, which also ensured proper microbial activity and a favourable environment.
Microbial populations such as Rhizobium, total bacteria, fungi, actinomycetes, phosphate-solubilizing bacteria (PSB), and free-living bacteria in postharvest soils are influenced by the application of micronutrients combined with Rhizobium inoculation. Most of the treatments in our study exhibited inconsistent variations in the populations of different microbes. Population variations in microbes depend on the soil environment (pH, moisture, etc.) conditions, which influence the survival of microbes in soil [61]. More rhizobium, fungus, and actinomycete populations were isolated in the T6 (Zn3B2Mo1 + Rhizobium) treatment. The highest population of Rhizobium might be favored for increasing yield and nutrient uptake because Rhizobium facilitates better nutrient mobilization and nutrient availability to plants [62]. The bacteria are capable of living in soil as well as inside legume root nodules, which involve atmospheric nitrogen fixation for supplying N to plants [10]. This observation is in agreement with the findings of Purwaningsih et al. [63] in Arachis hypogaea L., who reported that Rhizobium spp. bacteria can increase the host plant’s survival capacity; influence many physiological processes, including cell enlargement and division; and produce more lateral roots, root hairs, and root nodules, which help the plant take up available nutrients and fix biological nitrogen.
In our study, the combined application of Zn, B, and Mo with Rhizobium inoculation improved the postharvest soil organic matter and total N, P, Zn, and B contents (data not shown). Micronutrients and Rhizobium might influence plant biomass production, and increase microbial activities. However, the incorporation of legume biomass into the soil and seed inoculation with Rhizobium might support the availability of nutrients and increase the organic matter content in the soil. Kebede [64] and Yadav et al. [51] also reported that the incorporation of legume crops had a marked effect on rebuilding organic matter and conserving soil quality. In our study, we found a greater gross return with the application of 3 kg Zn, 2 kg B, and 1 kg ha−1 Rhizobium inoculation (50 g kg−1 seed). However, the benefit-cost ratio decreased due to the higher market price of Mo fertilizer in Bangladesh. Hence, the combined contribution of Zn, B, and Mo with Rhizobium inoculation is highlighted by a positive change in soil fertility through biological nitrogen fixation and nutrient availability to plants for maximizing crop productivity and quality.
The results indicated that the application of Zn, B, or Mo at 3, 2 or 1 kg ha−1, respectively, combined with Rhizobium inoculation (50 g kg−1 seed), contributed to enhancing the growth, yield, nodulation, and quality traits of garden pea. The same treatment resulted in the formation of more pods per plant and increased the yield of green pods and dry seeds. The treatment also increased the protein and vitamin C contents and improved the economic benefits. The application of 3 kg Zn, 2 kg B, and 1 kg Mo ha−1 along with Rhizobium inoculation improved postharvest soil fertility. The fertility can be used for the succeeding crop. According to the results and discussion, the recommendation can be made that the application of 3 kg Zn, 2 kg B, and 1 kg Mo ha−1 with Rhizobium inoculation (50 g kg−1 seed) support the maximum yield and quality improvement of garden pea in Zn-, B- and Mo-deficient soil. This result has potential for the region where there is no use of Rhizobium inoculant or imbalanced or no use of Zn, B, or Mo fertilizer in garden pea cultivation. Hence, more comprehensive research is needed in the future to determine the specific involvement of micronutrients and Rhizobium inoculants and to measure the ability of specific microbes to change soil health.
Acknowledgement: The authors are grateful to the authority of the Bangladesh Agricultural Research Institute (BARI) for financial support. The authors are thankful to the respective scientists working at the divisions of the Soil Science, BARI and Postharvest Section of the Horticulture Research Centre for providing the laboratory facilities for analysing the soil, pod, plant and seed samples of our experiment. The authors would like to acknowledge the Princess Nourah bint Abdulrahman University Researchers Supporting Project Number (PNURSP2024R82), Princess Nourah bint Abdulrahman University, Riyadh, Saudi Arabia for supporting the study.
Funding Statement: The research was performed with the financial support of the Bangladesh Agricultural Research Institute (BARI) under the Ministry of Agriculture, Bangladesh. This research was also funded by Princess Nourah bint Abdulrahman University Researchers Supporting Project Number (PNURSP2024R82), Princess Nourah bint Abdulrahman University, Riyadh, Saudi Arabia.
Author Contributions: Conceptualization and setup of the experiments: M.A.Q. and R.A.; methodology, data collection and formal analysis: M.A.Q., M.A.R., M.R., M.E.A., A.H., A.G., L.A.A., S.S. and R.A.; statistical analysis: M.A.Q., A.H., A.G., L.A.A., S.S. and R.A.; writing—original draft preparation: M.A.Q., R.A. and M.A.R.; writing—review and editing: M.A.Q., M.A.R., A.H., K.K.S., A.G., L.A.A., S.S. and M.A.S. Final manuscript approved by all the authors.
Availability of Data and Materials: The data presented in this article are available upon request from the corresponding author.
Ethics Approval: Not applicable.
Conflicts of Interest: The authors declare that they have no conflicts of interest to report regarding the present study.
References
1. FAO, IFAD, UNICEF, WFP, WHO. The state of food security and nutrition in the World 2022. Repurposing food and agricultural policies to make healthy diets more affordable. Rome, Italy; 2022. p. 1–260. doi:10.4060/cc0639en. [Google Scholar] [CrossRef]
2. Aloo BN, Tripathi V, Makumba BA, Mbega ER. Plant growth-promoting rhizobacterial biofertilizers for crop production: the past, present, and future. Front Plant Sci. 2022;13:1002448. doi:10.3389/fpls.2022.1002448 [Google Scholar] [PubMed] [CrossRef]
3. Mohanty K, Nayak DA, Mahapatra P, Jena NK. Effect of rhizobium and micronutrients on yield and yield attributing characters of garden pea (Pisum sativum L.). Int J Curr Microbiol Appl Sci. 2021;10(2):2776–84. doi:10.20546/ijcmas.2021.1002.307. [Google Scholar] [CrossRef]
4. Quilichini TD, Gao P, Yu B, Bing D, Datla R, Fobert P, et al. The seed coat’s impact on crop performance in pea (Pisum sativum L.). Plants. 2022;11(15):2056. doi:10.3390/plants11152056 [Google Scholar] [PubMed] [CrossRef]
5. Sayed EG, Ouis MA. Improvement of pea plant growth, yield, and seed quality using glass fertilizers and biofertilizers. Environ Technol Innov. 2022;26(4):102356. doi:10.1016/j.eti.2022.102356. [Google Scholar] [CrossRef]
6. Akter N, Ali MM, Akter MM, Hossain MM, Hossan MS, Khan MA. Effects of potassium on the growth, yield and physico-chemical properties of three garden pea (Pisum sativum) varieties. Asian J Agric Hort Res. 2020;5(3):22–31. doi:10.9734/AJAHR/2020/v5i330053. [Google Scholar] [CrossRef]
7. Cieslarova J, Hybli M, Griga M, Smykal P. Molecular analysis of temporal genetic structuring in pea (Pisum sativum L.) cultivars breed in the czech republic and former czechoslovakia since the mid-20th century. Czech J Genet Plant Breed. 2012;48:61–73. doi:10.17221/127/2011-CJGPB. [Google Scholar] [CrossRef]
8. Saini P, Nagpal S, Saini P, Kumar A, Gani M, Verma A, et al. Microbial mediated zinc solubilization in legumes for sustainable agriculture. Phytomicrobiome Interact Sustain Agricul. 2021;2(60):254–276. doi:10.1002/9781119644798.ch14. [Google Scholar] [CrossRef]
9. Uddin MR, Harun-Or-Rashid M, Khalid MA, Biswas MA, Kobir MS, Ashrafuzzaman M. Effect of organic and chemical fertilizers on growth and yield of garden pea. Int J Develop Res. 2023;13:63166–72. doi:10.37118/ijdr.26979.06.2023. [Google Scholar] [CrossRef]
10. Kumar S, Diksha, Sindhu SS, Kumar R. Biofertilizers: an ecofriendly technology for nutrient recycling and environmental sustainability. Curr Res Microb Sci. 2022;3(1):1–26. doi:10.1016/j.crmicr.2021.100094 [Google Scholar] [PubMed] [CrossRef]
11. Murgese P, Santamaria P, Leoni B, Crecchio C. Ameliorative effects of PGPB on yield, physiological parameters, and nutrient transporter genes expression in Barattiere (Cucumis melo L.). J Soil Sci Plant Nutr. 2020;20(2):784–93. doi:10.1007/s42729-019-00165-1. [Google Scholar] [CrossRef]
12. Fasusi OA, Cruz C, Babalola OO. Agricultural sustainability: microbial biofertilizers in rhizosphere management. Agricul. 2021;11(2):163. doi:10.3390/agriculture11020163. [Google Scholar] [CrossRef]
13. Tena W, Wolde-Meskel E, Walley F. Symbiotic efficiency of native and exotic Rhizobium strains nodulating lentil (Lens culinaris Medik.) in soils of southern Ethiopia. Agron. 2016;6(1):11. doi:10.3390/agronomy6010011. [Google Scholar] [CrossRef]
14. Pulido-Suárez L, Díaz-Peña F, Notario-del Pino J, Medina-Cabrera A, León-Barrios M. Alteration of soil rhizobial populations by rabbit latrines could impair symbiotic nitrogen fixation in the insular alpine ecosystem of Teide National Park. Appl Soil Ecol. 2021;160(7):103850. doi:10.1016/j.apsoil.2020.103850. [Google Scholar] [CrossRef]
15. Khiangte Z, Kalangutkar A, Sinam V, Siddique A. Impact of rhizobium inoculation and boron application on morphological alterations and biochemical triggers in pea (Pisum sativum L.). J Appl Nat Sci. 2023;15(1):69–74. doi:10.31018/jans.v15i1.4183. [Google Scholar] [CrossRef]
16. Singh JS, Gupta VK. Soil microbial biomass: a key soil driver in management of ecosystem functioning. Sci Total Environ. 2018;634:497–500 [Google Scholar] [PubMed]
17. Basu A, Prasad P, Das SN, Kalam S, Sayyed RZ, Reddy MS, et al. Plant growth promoting rhizobacteria (PGPR) as green bioinoculants: recent developments, constraints, and prospects. Sustain. 2021;13(3):1140. doi:10.3390/su13031140. [Google Scholar] [CrossRef]
18. Khan S, Chattopadhyay N. Effect of inorganic and biofertilizers on chilli. J Crop Weed. 2009;5:191–6. [Google Scholar]
19. Saber Z, Pirdashti H, Esmaeili M, Abbasian A, Heidarzadeh A. Response of wheat growth parameters to coinoculation of plant growth promoting rhizobacteria (PGPR) and different levels of inorganic nitrogen and phosphorus. World Appl Sci J. 2012;16:213–9. [Google Scholar]
20. Bhuiyan MAH, Khanam D, Hossain MF, Ahmed MS. Effect of Rhizobium inoculation on nodulation and yield of chickpea in calcareous soil. Bangladesh J Agric Res. 2008;33(4):549–54. [Google Scholar]
21. Kumar M, Jha AK, Hazarika S, Verma BC, Choudhury BU, Ramesh T, et al. Micronutrients (B, Zn, Mo) for improving crop production on acidic soils of Northeast India. Natl Acad Sci Lett. 2016;39(2):85–89. doi:10.1007/s40009-015-0409-x. [Google Scholar] [CrossRef]
22. Fageria NK, Baligar VC, Elson MK. Zinc requirements of tropical legume cover crops. Am J Plant Sci. 2014;5:1721–32. [Google Scholar]
23. Raj AB, Raj SK. Zinc and boron nutrition in pulses: a review. J Appl Nat Sci. 2019;11(3):673–9. doi:10.31018/jans.v11i3.2157. [Google Scholar] [CrossRef]
24. Alam I, Paul AK, Sultana S, Bithy PA. Effect of Zinc and Molybdenum on the Growth and Yield of Garden Pea (Pisum sativum L.). Int J Bioresour Stress Manag. 2020;11(4):425–31. doi:10.23910/1.2020.2138. [Google Scholar] [CrossRef]
25. Disante KB, Fuentes D, Cortina J. Response to drought of Zn stressed Quercussuber L. Seedlings. Environ Exp Bot. 2011;70:96–103. [Google Scholar]
26. Quddus MA, Anwar MB, Naser HM, Siddiky MA, Hussain MA, Aktar S, et al. Impact of zinc, boron and molybdenum addition in soil on mungbean productivity, nutrient uptake and economics. J Agric Sci. 2020;12(9):115–29. [Google Scholar]
27. Kudi S, Swaroop N, David AA, Thomas T, Hasan A, Rao S. Effect of different levels of sulphur and zinc on soil health and yield of greengram (Vigna radiata L.) Var. Patidar-111. J Pharmac Phytochem. 2018;7(3):2271–4. [Google Scholar]
28. Bolaños L, Esteban E, de Lorenzo C, Fernandez-Pascual M, de Felipe MR, Garate A, et al. Essentiality of boron for symbiotic dinitrogen fixation in pea (Pisum sativum) Rhizobium nodules. Plant Physiol. 1994;104:85–90. [Google Scholar]
29. Quddus MA, Rashid MM, Siddiky MA, Islam MA, Rahman MA. Response of mungbean varieties to boron in calcareous soils of Bangladesh. Bangladesh J Agric Res. 2022;47(1):105–18. [Google Scholar]
30. Qamar J, Rehman A, Ali MA, Qamar R, Ahmed K, Raza W. Boron increases the growth and yield of mungbean. J Adv Agric. 2016;6(2):922–4. [Google Scholar]
31. Laxmi S, Patel R, Singh S, Choudhary B, Gadhwal R, Meena R, et al. Growth and yield response of mungbean as influenced by sulphur and boron application. Int J Curr Microbiol Appl Sci. 2020;9(3):2788–94. [Google Scholar]
32. Das SK. Influence of sulphur and boron on growth and yield of garden pea (Pisum sativum L.) (MS Thesis). Department of Horticulture, Sher-e-Bangla Agricultural University: Bangladesh; 2020. [Google Scholar]
33. Rana MS, Bhantana P, Imran M, Saleem MH, Chengxiao Hu, et al. Molybdenum potential vital role in plants metabolism for optimizing the growth and development. Ann Environ Sci Toxicol. 2020;4(1):032–44. [Google Scholar]
34. Khan QA, Cheema SA, Farooq M, Wakeel A, Haider FU. Monitoring the role of molybdenum and seed priming on productivity of mung bean (Vigna radiata L.). J Res Ecol. 2019;7(1):2417–27. [Google Scholar]
35. Shil NC, Saleque MA, Islam MR, Jahiruddin M. Soil fertility status of some of the intensive crop growing areas under major agro-ecological zones of Bangladesh. Bangladesh J Agric Res. 2016;41(4):735–57. [Google Scholar]
36. Page AL. Methods of soil analysis. Madison, WI; 1982. p. 1159. [Google Scholar]
37. Vincent JM. A manual for the practical study of root-nodule bacteria. In: IBP hand book No. 15. Oxford, England: Blackwell Scientific Publications; 1970. p. 164. [Google Scholar]
38. Somasegaran P, Hoben HJ. Handbook for Rhizobia: Methods in legume-Rhizobium technology. New York: Springer-Verlag; 1994. [Google Scholar]
39. Anonymous. Official methods of analysis. Washington DC: Association of Official Agricultural Chemists; 1994. [Google Scholar]
40. Ranganna S. Handbook of analysis and quality control for fruit and vegetable products. 2nd edNew Delhi, India: Tata McGraw Hill Pub. Co., Ltd.; 1986. [Google Scholar]
41. Piper CS. Soil and plant analysis. Adelaide: Adelaide University Press; 1964. [Google Scholar]
42. Persson JA, Wennerholm M, O’Halloram S. Handbook for kjeldahl digestion. DK-3400 Hilleroed, Denmark: FOSS; 2008. [Google Scholar]
43. Hiller A, Plazin J, Vanslyke DD. A study of conditions of Kjeldhal determination of nitrogen in proteins. J Biol Chem. 1984;176(3):1401–20. [Google Scholar]
44. Sharma NK, Singh RJ, Kumar K. Dry matter accumulation and nutrient uptake by wheat under poplar based agroforestry system. ISRN Agron. 2012:7. doi:10.5402/2012/359673. [Google Scholar] [CrossRef]
45. Pikovskaya RI. Mobilization of phosphorus in soil in connection with vital activity of some microbial species. Microbiol. 1948;17:362–70. [Google Scholar]
46. Rahman R, Sofi JA, Javeed I, Malik TH, Nisar S. Role of micronutrients in crop production. Int J Curr Microbiol App Sci. 2020; (Special Issue-11):2265–87. [Google Scholar]
47. Stagnari F, Maggio A, Galieni A, Pisante M. Multiple benefits of legumes for agriculture sustainability: an overview. Chem Biol Technol Agric. 2017;4(1):2. doi:10.1186/s40538-016-0085-1. [Google Scholar] [CrossRef]
48. Tsyganova AV, Tsyganov VE. Plant genetic control over infection thread development during legume-Rhizobium symbiosis. In: Rigobelo EC, editor. Symbiosis. London: IntechOpen; 2018. p. 23–52. [Google Scholar]
49. Hossain MA, Quddus MA, Alam MK, Naser HM, Anwar B, Khatun F, et al. Application of zinc, boron and molybdenum in soil increases lentil productivity, nutrient uptake and apparent balance. Canadian J Soil Sci. 2021;101(1):113–4. [Google Scholar]
50. Quddus MA, Hossain MA, Naser HM, Anwar B, Akhtar S, Nazimuddin M. Effect of zinc and boron application on productivity, quality and nutrient uptake of fieldpea (Pisum sativum L.) grown in calcareous soils. J Agric Sci Pract. 2018;3(6):132–43. [Google Scholar]
51. Yadav A, Singh D, Kumar R, Pandey SB, Pal S, Sachan R, et al. Effect of phosphorus, zinc and rhizobium on productivity and profitability of chickpea (Cicer arietinum L.) under central plain zone of Uttar Pardesh. Int J Plant Soil Sci. 2022;34(22):1256–66. [Google Scholar]
52. Santos RM, Diaz PAE, Lobo LLB, Rigobelo EC. Use of plant growth-promoting rhizobacteria in maize and sugarcane: characteristics and applications. Front Sustain Food Syst. 2020;4:136. doi:10.3389/fsufs.2020.00136. [Google Scholar] [CrossRef]
53. Islam MM, Karim MR, Oliver MMH, Urmi TA, Hossain MA, Haque MM. Impacts of trace element addition on Lentil (Lens culinaris L.) Agronomy. 2018;8(7):100. [Google Scholar]
54. Tilman D, Balzer C, Hill J, Befort BL. Global food demand and the sustainable intensification of agriculture. Proc Natl Acad Sci U S A. 2011;108:20260–4 [Google Scholar] [PubMed]
55. Alam F, Kim TY, Yeob Kim S, Alam SS, Pramanik P, Kim PJ, et al. Effect of molybdenum on nodulation, plant yield and nitrogen uptake in hairy vetch (Vicia villosa Roth). Soil Sci Plant Nutr. 2015;61(4):664–75. [Google Scholar]
56. Das S, Pareek N, Raverkar KP, Chandra R, Kaustav A. Effectiveness of micronutrient application and Rhizobium inoculation on growth and yield of Chickpea. Int J Agric Environ Biotechnol. 2012;5(4):445–52. [Google Scholar]
57. Gupta SC, Gangwar S. Effect of molybdenum, iron and microbial inoculants on symbiotic traits, nutrient uptake and yield of chickpea. J Food Legumes. 2012;25(1):45–9. [Google Scholar]
58. Allito BB, Ewusi‐Mensah N, Logah NV, Hunegnaw DK. Legume‐rhizobium specificity effect on nodulation, biomass production and partitioning of faba bean (Vicia faba L.). Sci Rep. 2021;11:3678. doi:10.1038/s41598-021-83235-8 [Google Scholar] [PubMed] [CrossRef]
59. Bejandi TK, Sharifii RS, Sedghi M, Namvar A. Effects of plant density, Rhizobium inoculation and microelements on nodulation, chlorophyll content and yield of chickpea (Cicer arietinum L.). Ann Biol Res. 2012;3(2):951–8. [Google Scholar]
60. Wang Q, Liu J, Zhu H. Genetic and molecular mechanisms underlying symbiotic specificity in legume-rhizobium interactions. Front Plant Sci. 2018;9:313. doi:10.3389/fpls.2018.00313 [Google Scholar] [PubMed] [CrossRef]
61. Thilakarathna MS, Raizada MN. A meta-analysis of the effectiveness of diverse rhizobia inoculants on soybean traits under field conditions. Soil Biol Biochem. 2017;105:177–96. [Google Scholar]
62. Dey R, Pal KK, Bhatt DM, Chauhan SM. Growth promotion and yield enhancement of peanut (Arachis hypogaea L.) by application of plant growth-promoting rhizobacteria. Microbiol Res. 2004;159(4):371–94. doi:10.1016/j.micres.2004.08.004 [Google Scholar] [PubMed] [CrossRef]
63. Purwaningsih S, Agustiyani D, Antonius S. Diversity, activity, and effectiveness of Rhizobium bacteria as plant growth promoting rhizobacteria (PGPR) isolated from Dieng, central Java. Iranian J Microbiol. 2021;13(1):130–6. [Google Scholar]
64. Kebede E. Contribution, utilization, and improvement of legumes-driven biological nitrogen fixation in agricultural systems. Front Sustain Food Syst. 2021;5:1–18. doi:10.3389/fsufs.2021.767998. [Google Scholar] [CrossRef]
Cite This Article
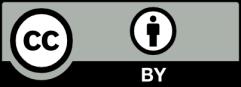
This work is licensed under a Creative Commons Attribution 4.0 International License , which permits unrestricted use, distribution, and reproduction in any medium, provided the original work is properly cited.