Open Access
REVIEW
Research Progress on Economic Forest Water Stress Based on Bibliometrics and Knowledge Graph
1 College of Mechanical and Electrical Engineering, Inner Mongolia Agricultural University, Hohhot, 010018, China
2 College of Energy and Traffic Engineering, Inner Mongolia Agricultural University, Hohhot, 010018, China
3 Key Laboratory of Biopesticide Creation and Resource Utilization of Universities, Inner Mongolia Agricultural University, Hohhot, China
* Corresponding Author: Zheying Zong. Email:
(This article belongs to the Special Issue: Abiotic and Biotic Stress Tolerance in Crop)
Phyton-International Journal of Experimental Botany 2024, 93(5), 843-858. https://doi.org/10.32604/phyton.2024.049114
Received 28 December 2023; Accepted 08 March 2024; Issue published 28 May 2024
Abstract
This study employed the bibliometric software CiteSpace 6.1.R6 to analyze the correlation between thermal infrared, spectral remote sensing technology, and the estimation of economic forest water stress. It aimed to review the development and current status of this field, as well as to identify future research trends. A search was conducted on the China National Knowledge Infrastructure (CNKI) database using the keyword “water stress” for relevant studies from 2003 to 2023. The visual analysis function of CNKI was used to generate the distribution of annual publication volume, and CiteSpace 6.1.R6 was utilized to create network maps illustrating collaboration among authors and institutions. The study also analyzed the hotspots and frontiers of economic forest water stress. As a result, a total of 6664 academic journal articles related to water stress were retrieved. Considerable collaboration networks were observed among scholars and institutions, with a focus on using crown temperature monitoring to diagnose crop water stress. Based on the research findings, it was evident that the primary research trend involved the use of thermal infrared and spectral remote sensing technology for estimating water stress, making it a future research hotspot.Keywords
China possesses abundant natural resources, but the state of its water resources is not optimistic. While the country accounts for 7% of the world’s total water resources, its population constitutes approximately 18% of the global population. The per capita water resources are only 2200 cubic meters, making it one of the 13 countries with the most meager per capita water resources globally. On February 28, 2023, the National Bureau of Statistics released the “Statistical Communiqué of the People’s Republic of China on the National Economic and Social Development in 2022” [1]. According to preliminary calculations, the total water resources in 2022 were 26,634 billion cubic meters, with a total water consumption of 5997 billion cubic meters, representing a 1.3% increase from the previous year. Agricultural water usage increased by 3.7%. Against this backdrop, the issue of water stress for economic crops [2] is becoming increasingly urgent. Economic forests [3], also known as “special-purpose forests”, are characterized by the primary purpose of producing fruits, edible oils, industrial raw materials, and medicinal materials. The cultivation methods involve high density, high efficiency, high returns, and low costs. Simultaneously, economic forests provide ecological protection and social benefits. In recent years, with the intensification of environmental protection efforts in the country, there has been a noticeable improvement in ecological fragility, leading to a significant enhancement in ecological benefits. As the most crucial component of ecosystems, forests play a pivotal role in windbreak and sand fixation, carbon sequestration, and water source conservation. Actively implementing the “Two Mountains” concept and building an ecological economy in China are strategic measures for the social development of contemporary China.
In the realm of crop water stress, thermal infrared and spectral inversion technologies [4–7] can identify the water stress conditions of crops by detecting information such as leaf surface temperature and spectral reflectance. Since their inception, thermal infrared and spectral remote sensing technologies have rapidly developed, particularly with the incorporation of multispectral thermal infrared remote sensing techniques [8–10], becoming crucial research directions and breakthrough points in the field of thermal infrared remote sensing. Thermal infrared and spectral inversion is a technique that involves analyzing the spectral information emitted or reflected by an object to obtain specific properties of the object. When crops face water stress issues, the inability of plants to absorb sufficient water for growth and metabolism leads to a corresponding increase in leaf surface temperature. Simultaneously, there are changes in the spectral reflectance of crops. By utilizing high-spectral-resolution thermal infrared inversion techniques, these changes can be monitored and analyzed, allowing for the identification of crop water stress conditions. Through high-spectral-resolution thermal infrared inversion of crop water stress, it is possible to achieve non-destructive monitoring [11] of crops and provide real-time feedback. This aids farmers in promptly detecting and addressing crop water stress issues, ultimately enhancing crop production efficiency and quality. From a scientific research perspective, it is crucial to comprehensively understand the current state of research and the future development trends of thermal infrared and spectral remote sensing technologies in the field of economic forest water stress research.
In recent years, research in scientometrics has gradually shifted from mathematical and statistical methods [12] to more comprehensive and intelligent knowledge graphs [13]. Some relevant operational software is equipped with visualization capabilities, enabling the analysis of potential knowledge embedded in scientific literature. Through visualization techniques, these tools present the structure, patterns, and distribution of scientific knowledge. CiteSpace 6.1.R6 is a typical and widely used software for visualizing literature analysis [14,15]. On one hand, it can assist researchers in analyzing the overall development processes and trends in a specific research field by ranking certain scientometric indicators, such as the activity of countries, institutions, journals, and researchers within that field. On the other hand, employing network analysis methods from scientometrics can visually and concretely analyze the emphasis and scope of research hotspots by examining the co-occurrence of keywords and topics. Additionally, it can establish co-occurrence networks for journals and disciplines, aiding researchers in analyzing specific research directions within these fields. Moreover, it can investigate the exchange and integration between disciplines, such as in remote sensing technology [16], smart agriculture [17], food safety [18], and intelligent transportation [19]. This software not only objectively and accurately analyzes the past and current development situations in these areas but also helps researchers systematically analyze potential future research hotspots.
Therefore, this study aims to visualize the collected literature data through scientometric methods and knowledge graphs. It will comprehensively analyze the research progress of hyperspectral thermal infrared inversion technology in the monitoring of water stress in economic crops over the past 20 years. This analysis will cover the fundamental principles, current applications, and existing challenges, and provide insights into future development trends. The goal is to offer reference and assistance to researchers in this field.
To gain a deeper understanding of the correlation between hyperspectral thermal infrared remote sensing technology and water stress in economic crops, this study relies on an extensive literature database [20]. The chosen database is the China National Knowledge Infrastructure (CNKI), and advanced literature searches are conducted using subject terms. The searches were performed three times, and the search criteria are outlined in Table 1.
The search was conducted on December 22, 2023, and each search record contained major data information such as authors, institutions, journals, countries/regions, keywords, abstracts, citations, and references cited. From the table, it can be seen that the retrieval results of hyperspectral and thermal infrared technology applied to monitoring crop water stress are very few, indicating that researchers pay less attention to this aspect. It is a weak link in the field of water stress research, and it is more worthy of exploration, research, and analysis. This paper will process and analyze based on these literature data.
First, create a new folder on the PC, and within this folder, create four sub-folders named “data”, “input”, “output” and “project”. Export the literature in Refworks format from CNKI to the “input” sub-folder. Rename the exported “.txt” file in the format “download_xx”. Use the control interface of CiteSpace 6.1.R6 software to perform data conversion, resulting in a new “.txt” format file in the “output” folder. Copy the converted text file from the “output” folder to the “data” sub-folder, making it compatible for use with CiteSpace 6.1.R6. All processed analysis results will be stored in the “project” sub-folder through CiteSpace 6.1.R6 software, facilitating the recording and subsequent analysis of results.
3.1 Basic Statistical Results of Research Field Based on CNKI Database
3.1.1 Analysis of the Number of Publications
In the “Advanced Search” module of the CNKI database, the search was conducted with the following subject terms: “water stress”, “water stress and thermal infrared” and “water stress and spectrum”. The time range for the search was set from “2003–2023”. The visualization analysis feature of CNKI was used to generate trend analysis graphs, including publication volume, main topic distribution, and secondary topic distribution. The annual publication volume is shown in Fig. 1. Over the past 20 years, the literature on the topic of “water stress” has generally exhibited a stable trend, with a slight decline in the last five years. Between 2003 and 2010, the publication volume in this field was quite substantial, with an average of 331 papers per year. The publication volume reached a peak around 2010. Around 2010, with global climate warming, there was an increased focus on the efficient utilization of water resources. Concurrently, the development of new hardware devices such as sensors also propelled the advancement of the discipline. Since 2010, there has been an overall downward trend in annual publications, decreasing by approximately 35 papers per year. By 2022, the annual publication volume is 180 papers, and the data indicates an estimated 160 journal articles to be published in 2023. The search for literature on thermal infrared and spectroscopy in this context yielded relatively fewer results, requiring further comprehensive analysis.
Figure 1: Number of publications
Using the Burst Detection feature of CiteSpace 6.1.R6 software, an analysis was conducted on the earliest publication time and individual publication volume of researchers in the field of water stress. The top six authors by publication volume were selected for a detailed explanation. During this period, Liang et al. had the highest publication volume, with a total of 29 papers. He first published in this field in 2004, focusing on the physiological characteristics of plants under different soil moisture conditions [21–25]. Zhang et al. had a total of 18 papers, with the first publication in 2009, concentrating on the impact of water stress on crop enzyme activity and yield [26–30]. Wang et al. published 15 papers, with the first article in 2007, and his research focused on the influence of wet-dry alternation on crop growth [31–35]. Sun et al. published a total of 15 papers, with the first publication in 2008, and his research centered around monitoring and simulating crop water consumption processes [36–40]. Zhou et al. published 14 papers, with the first publication in 2004, concentrating on plant growth and energy cycles [41–45]. Zhang et al. also published 14 papers, with the first publication in 2005, and focused on the drought resistance physiology of crops and the relationship between water and fertilizer for rain-fed crops [46–50]. See Table 2 for details.
3.1.2 Distribution of Main and Secondary Themes
The primary themes encapsulate the focal points and central topics of the literature. Secondary themes refer to parts generated from the content but do not constitute the main focus of the discussion. Nevertheless, they aid in the auxiliary analysis of the primary themes. Analyzing the quantity of literature related to these themes allows us to discern that heavily researched themes possess relatively limited research space. Researchers may opt to explore themes with moderate or lower publication volumes for subsequent investigations. From the primary theme distribution chart generated in this search, as illustrated in Fig. 2, it is evident that factors such as drought resistance, physiological characteristics, and photosynthetic attributes [51] are indispensable elements in the study of water stress. The secondary theme distribution chart, as depicted in Fig. 3, reveals that physiological indicators like net photosynthetic rate, SOD (Superoxide Dismutase), and stomatal conductance [52], have certain correlations with water stress. These indicators can provide substantial data support for research related to water stress, while also offering researchers new directions and perspectives. By examining both primary and secondary theme distribution charts, researchers can swiftly familiarize themselves with the research content, themes, and keywords in this field. This aids in rapidly comprehending the current state of research within this domain and enables the swift determination of one’s research focus by understanding academic hotspots and research themes.
Figure 2: Main topic distribution
Figure 3: Secondary topic distribution
3.2 Basic Statistical Results Based on Cite Space
In the early 1980s, research on the application of thermal infrared and spectroscopy technologies commenced in China. Inverse techniques based on thermal infrared and spectroscopy technologies rely on the thermal radiation characteristics of crops, utilizing the analysis of relevant crop data to infer their moisture conditions. Throughout the crop growth process, moisture influences parameters such as crop temperature, chlorophyll content, and leaf area index, among others. Changes in these parameters result in variations in the thermal radiation characteristics of crops. Therefore, through the analysis of hyperspectral thermal infrared data, one can obtain parameters such as crop temperature, chlorophyll content, and leaf area index, allowing for the assessment of the crop’s moisture status. To conduct a more in-depth research analysis, literature was specifically searched with “water stress”, “water stress + thermal infrared” and “water stress + spectroscopy” as the main themes. This was done to explore relevant issues in the field of water stress research concerning thermal infrared and spectroscopy technologies.
3.2.1 Analysis of the Cooperation Network of Authors and Institutions
Another significant factor driving the continuous acceleration of scientific research collaboration is the progress in information technology, which has broadened the boundaries of human activities. This has led research groups, scientific institutions, and businesses to establish even closer connections. The increasing convergence and globalization across numerous academic fields and disciplines, along with the interdisciplinary nature of certain research, facilitate scholars’ collaborative efforts. In this section, an author and institution collaboration network was established using CiteSpace and categorized into clusters, as illustrated in Fig. 4. In the field of water stress research, the primary contributors are Northwest A&F University and the University of Chinese Academy of Sciences. Collaboration exists between these two universities. Individuals such as Zongsuo Liang, Wenting Han, and Rui Zhang have made notable contributions to this field. Collaborations have been established between Northwest A&F University and various research institutes. A shared research system has been established among researchers and research units, providing a solid foundation for collaboration, thereby furthering the development of disciplines and research.
Figure 4: Network of co-authors and cooperative institutions
3.2.2 Co-Author Network Analysis
The co-authorship network is a common form of scientific collaboration that provides a powerful tool for studying the structural aspects of academic relationships. The co-authorship network analysis feature of CiteSpace software establishes a network based on the collaborative relationships among paper authors. If two authors co-author one or more papers, a connection is formed between them. Clusters in the author network are identified through CiteSpace software analysis, and each cluster’s key keyword labels are extracted through semantic analysis.
The data on the themes “water stress + spectrum” and “water stress + thermal infrared” were processed and analyzed, yielding the results depicted in Figs. 5 and 6. In Fig. 5, five main clusters are generated, representing five co-authorship networks with a focus on “cotton”, “reflectance spectra”, “inorganic nutrients”, “Dunaliella” and “leaf spectra”. Notably, authors such as Chunjiang Zhao and Dengwei Wang [53] exhibit high activity in these co-authorship networks, indicating extensive collaborative research in the field of using spectral techniques to infer water stress.
Figure 5: Spectral co-author network
Figure 6: Thermal infrared co-author network
In Fig. 6, five main clusters are also generated, representing five co-authorship networks with a focus on “image processing”, “remote sensing”, “vegetation indices”, “eddy covariance techniques” and “itvdi”. Authors like Wenhuan Jie and Yan Li are involved in numerous collaborative research and publication activities, indicating a broad research scope in the domain of using thermal infrared techniques to infer crop water stress.
The keyword timeline graph is constructed based on the keywords of the literature, with keyword co-occurrence relationships forming the foundation for building a keyword co-occurrence network. Each node on the keyword co-occurrence network represents a keyword, and the size of the node is positively correlated with the frequency of its occurrence. Larger nodes correspond to keywords with higher frequencies, and the thickness of the lines connecting nodes indicates the co-occurrence frequency between two keywords. The keyword timeline graph provides a clear and intuitive analysis of the changes and innovations in research hotspots related to water stress over time, with continuous improvements in associated research techniques.
Using the theme “water stress” as the dataset for statistical analysis, as shown in Fig. 7, four main keywords emerge: “yield”, “water stress”, “drought stress” and “wheat”. From Fig. 8, it can be observed that from 2003 to 2006, researchers primarily focused on winter wheat, maize, and rice, studying soil moisture, seedling growth, and nitrogen forms [54]. From 2006 to 2010, attention shifted to spring wheat and soybeans, with research on parameters such as transpiration rate, chlorophyll, and osmotic regulation [55]. The period from 2010 to 2016 saw a transition to peanut research, with experiments on leaf water potential and physiological responses [56]. From 2016 to 2020, researchers turned to the growth and development of sugarcane, primarily observing root-to-shoot ratios [57]. In the years 2020 to 2023, there was a focus on changes in irrigation methods, aided by remote sensing techniques like drones, contributing to the improvement of fruit quality [58]. From the retrieved articles, it is evident that researchers such as Zhitao Zhang and Wenting Han from Northwest A&F University are actively engaged in the field of water stress. They primarily use remote sensing techniques to obtain data parameters such as soil moisture and organic content, applying relevant algorithms for denoising and interference removal to achieve effective inversion and provide valuable guidance for agricultural development [59,60]. Abroad, researchers are focusing on the correlation between water stress and sustainable development, delving specifically into the relationship between water, salt, and crops [61–63]. For example, Castro-Nava et al. studied the drought response of sorghum with different drought-tolerant genotypes at various growth stages [64]. Ahmad employed seed priming and exogenous supplementation of β-aminobutyric acid to alleviate drought stress in flaxseed oil [65]. Ahmad investigated the impact of different plant densities on the growth and seed yield of Camelina under normal and water-deficient conditions [66].
Figure 7: Keyword timeline map
Figure 8: Author, keyword clustering
3.2.4 Cluster Analysis of Authors and Keywords
In the CiteSpace user interface, the “data” subfolder with the theme “water stress” was selected as the dataset. Clustering analysis was performed using “author” and “keywords”, yielding the results shown in Fig. 8. Hua Qi’s research primarily focuses on the drought resistance of crops under water stress. Indicators such as chlorophyll fluorescence characteristics and photosynthetic properties are employed to monitor the water status of crops. These indicators assist farmers and agricultural researchers in understanding the moisture conditions of crops and provide decision support. Researchers like Yi Zhou and Shiwei Guo analyze the impact of water stress on nitrogen forms in crops such as rice, studying water-saving characteristics under different scenarios. Their approach is convenient, computationally simple, and precise.
This study utilized CiteSpace 6.1.R6 software to analyze 6664 journal articles related to water stress retrieved from the CNKI database spanning the last 20 years (2003–2023). The analysis involved creating knowledge network maps based on factors such as authors, publishing institutions, and keywords. Additionally, the study incorporated the distribution of annual publication counts to analyze emergent patterns in research hotspots and advancements in the field. The search results revealed 6664 journal articles with “water stress” as the main subject term. However, when combining “water stress” with “infrared” and “water stress” with “spectroscopy” as subject terms, only 50 and 68 journal articles were retrieved, respectively. This suggests that research explicitly utilizing features like infrared and spectroscopy technologies is limited, and there is relatively less attention from researchers, indicating a potential area of focus for future studies. Examining the distribution of annual publication counts, the average annual publication count was 331 articles during the period from 2003 to 2010, with a peak in 2010. From 2010 onwards, there has been a general decline in the annual publication count, though the decrease has been gradual. By 2022, the average annual publication count had decreased to 180 articles. Simultaneously, with the rapid development of smart agriculture and continuous technological innovation, relevant authorities in China aim to position the water stress field as a robust engine, fostering high-quality development in agriculture and certain industrial sectors. With the support of these policies, it is expected that the publication count in this field will show a growth trend in the coming years. Visualizing the primary and secondary topic distribution through CNKI, the research in this field is primarily focused on biological characteristics such as canopy temperature, transpiration rate, and chlorophyll content, as well as aspects of cultivation such as breeding, selection, and water-saving irrigation. Over the past decade, the predominant research methods have relied on remote sensing to monitor the water stress status of crops, benefiting from the upgrading of remote sensing devices.
The use of CiteSpace software employed various analysis methods such as keyword co-occurrence networks, co-authorship networks, and timeline graphs to conduct a systematic bibliometric analysis and knowledge map research on the research hotspots and distribution in the field. The overall development of the water stress-related field over the past 20 years was outlined. During this period, research efforts were predominantly focused on biological characteristics such as canopy temperature, transpiration rate, and chlorophyll content, as well as aspects of crop cultivation like breeding, selection, water-saving irrigation, and planting patterns. An analysis of CNKI journal literature data from 2020 to 2023 revealed a novel research direction concentrating on techniques such as image processing and feature extraction for variety identification and water stress diagnosis. The co-authorship and institution co-occurrence networks vividly illustrated the well-established collaboration patterns among domestic researchers and institutions in this field. This collaboration has facilitated resource integration, disciplinary diversification, and staying at the forefront, enabling a more comprehensive and in-depth analysis of issues. It revealed information about collaboration patterns, strengths, and directions among different authors and institutions, fostering the emergence of new ideas, technologies, and methods, and providing directional guidance and decision support for collaborators and other researchers. The keyword timeline graph, analyzed from the perspective of temporal development, traced the evolution of multiple research hotspots and their interrelated support in the data. Author and keyword clustering analysis can provide readers with the necessary research information, offering insights into the focal points of authors and allowing for a more profound analysis of research characteristics and implications. For instance, existing researchers have utilized methods such as image collection, image processing, and deep learning for research in the water stress domain.
1. On November 15, 2022, the United Nations announced that the global population had surpassed 8 billion, marking a milestone in human development and a crucial moment for humanity to consider its shared responsibility towards the Earth. The protection of water resources and the improvement of water efficiency are of paramount importance, especially in the agricultural sector, which accounts for 70% of global water consumption. Monitoring water stress in economic crops is significant for water conservation and crop growth. With advancements in science and technology, thermal infrared and spectral remote sensing technologies have become powerful tools for comprehensive, all-weather, multi-angle, and multi-spectral observation of farmland surface temperatures. Thermal infrared and spectral inversion techniques, based on the thermal radiation characteristics of crops, analyze crop thermal infrared and spectral data to infer their water conditions. Throughout the crop growth process, water influences parameters such as temperature, chlorophyll content, and leaf area index, leading to changes in the thermal radiation characteristics of crops. Therefore, analyzing thermal infrared and spectral data allows the acquisition of parameters such as temperature, chlorophyll content, and leaf area index, enabling the assessment of crop water conditions for precise irrigation and management to enhance crop growth and yield. Simultaneously, thermal infrared and spectral inversion techniques can be applied to monitor and assess crop water stress, assisting agricultural producers in promptly identifying and addressing water stress issues in crops. In recent years, these techniques have found widespread application in crop water condition monitoring. Some studies suggest that thermal infrared and spectral inversion techniques can effectively infer crop water conditions, demonstrating stable application results across different crops and growth stages. For example, research indicates that during the growth of maize, thermal infrared, and spectral inversion techniques can accurately infer maize’s leaf area index and transpiration rate, facilitating the monitoring and assessment of maize water conditions. Similarly, studies show that thermal infrared and spectral inversion techniques exhibit high accuracy and stability in monitoring water stress in crops such as wheat and rice.
2. Despite the high accuracy and stability demonstrated by thermal infrared and spectral inversion technologies in monitoring crop water stress, there are still some issues that need to be addressed. The primary concern is the complexity of data acquisition and processing. Collecting thermal infrared and spectral data requires the use of high-resolution thermal infrared sensors, along with data correction and noise reduction processing, increasing the difficulty and complexity of data collection and processing. Additionally, thermal infrared and spectral inversion technologies have some limitations, primarily in the following aspects. Firstly, these technologies are sensitive to the growing environment of economic crops, such as environmental temperature, humidity, light intensity, etc. Changes in these factors can affect the thermal radiation characteristics of crops, thereby impacting the accuracy of the inversion results. Secondly, the application of thermal infrared and spectral inversion technologies needs to consider factors such as the growth stage and variety of characteristics of economic crops. Different crops and growth stages have varying impacts on the inversion results. Lastly, when widely applied, the temporal and spatial resolution issues need to be considered in thermal infrared and spectral inversion technologies. Different time and spatial scales also have different effects on the inversion results.
3. Although thermal infrared and spectral inversion technologies have some issues and limitations, their prospects in the monitoring of crop water stress remain extensive. In the future, the development directions of thermal infrared and spectral inversion technologies will mainly include the following aspects. First, there is a need to further improve the spatial and temporal resolution of data. Currently, the spatial and temporal resolution of thermal infrared and spectral inversion technologies is relatively low and insufficient to meet the demands of widespread, high-precision monitoring of economic crop water stress. Therefore, there is a need to enhance the spatial and temporal resolution of thermal infrared and spectral data to meet the monitoring requirements of economic crop water stress at different regions and scales. Second, research should be conducted on the fusion of multi-source data. Currently, monitoring economic crop water stress requires considering multiple factors such as the growth status of economic crops, environmental factors, and soil moisture conditions. A single data source often cannot meet these demands. In the future, thermal infrared and spectral data can be fused with other multi-source data, such as remote sensing images, meteorological data, ground observation data, etc., to improve the accuracy and reliability of the inversion monitoring of economic crop water stress. Third, integrates machine learning, deep learning, and other technologies for in-depth analysis. While thermal infrared and spectral inversion technologies can infer the water status of crops, the analysis and judgment of factors such as the growth status of crops, environmental factors, and soil moisture conditions are still relatively limited. In the future, combining mature and advanced artificial intelligence technologies can enhance the intelligence of monitoring economic crop water stress.
4. Through the analysis and review of a large number of literature, this paper summarizes the current research hotspots as monitoring crop water stress at the large spatial scale of sky-ground. The focus primarily revolves around thermal infrared remote sensing and multispectral hyperspectral remote sensing monitoring technologies. Additionally, research on water stress at small spatial scales, typified by sunlight greenhouses, is also a significant hotspot due to its unique research value arising from geographical characteristics. In the future, with the impact of global warming and the “dual carbon” goals, there will inevitably be a push for more research technologies and the iterative updating of related sensors and hardware and software equipment. New remote sensing methods and data analysis approaches from these sensors might provide fresh perspectives for research. Consequently, new research hotspots and directions are expected to emerge in the field of water stress, contributing to the sustainable development of agriculture and society.
In conclusion, thermal infrared and spectral inversion technology is an emerging remote sensing monitoring technique with advantages such as high accuracy and stability. It holds vast prospects for application in monitoring economic crop water stress. In the future, further enhancing the application effectiveness and monitoring capabilities of thermal infrared and spectral inversion technology can be achieved through the development of higher spatial and temporal resolution data, multi-source data fusion, and the integration of technologies such as machine learning.
Acknowledgement: The authors sincerely thank the anonymous reviewers who made valuable comments on this paper.
Funding Statement: This work is supported jointly by the Inner Mongolia Natural Science Foundation (2023MS06002), the Scientific Research Project of Higher Education Institutions of Inner Mongolia Autonomous Region (NJZZ22509), the Development Project of Young Scientific and Technological Talents (Innovative Teams) of Inner Mongolia Autonomous Region 2023 (NHGIRT2312), and the Project of Research and Practice on Teaching Reform of Graduate Education of Inner Mongolia Autonomous Region ( JGCG2023049) were funded.
Author Contributions: Conceptualisation, Chunguang Wang and Zheying Zong; writing—original draft preparation, Xin Yin and Shuai Wang; writing—review and editing, Xin Yin, Shuai Wang, Haichao Wang and Zeyu Ban; supervision, Zheying Zong, Haichao Wang and Shuai Wang. All authors have read and agreed to the published version of the manuscript.
Availability of Data and Materials: The data that support the findings of this study are available from the corresponding authors upon reasonable request.
Ethics Approval: Not applicable.
Conflicts of Interest: The authors declared that they have no conflicts of interest to report regarding the present study.
References
1. State Statistical Bureau. 2022 statistical bulletin of the people’s republic of china on national economic and social development. People’s Daily; 2023. [Google Scholar]
2. Yuan GF, Tang DY, Luo Y, Yu Q. Advances in canopy-temperature-based crop water stress research. China: Adv Earth Sci; 2001. p. 49–54 (In Chinese). [Google Scholar]
3. Meng J. Research on ecological economic forest and sustainable development. Hebei Agric; 2022;(12). p. 49–51 (In Chinese). [Google Scholar]
4. Wu H, Li XJ, Li ZL, Duan SB, Qian YG. Hyperspectral thermal infrared remote sensing: current status and perspectives. Nat Remote Sens Bull. 2021;1567–90 (In Chinese). [Google Scholar]
5. Jian Y, Li CL, Ji HZ. Status and prospect of thermal infrared hyperspectralimaging technology. J Infrared Millim Waves. 2015;51–9 (In Chinese). [Google Scholar]
6. Wang XH, Qiu S, Li ZL, Jiang XG. Land surface temperature and emissivity retrieval from hyperspectral thermal infrared data. Arid Land Geogr. 2010;419–26 (In Chinese). [Google Scholar]
7. Camoglu G, Demirel K, Genc L. Use of infrared thermography and hyperspectral data to detect effects of water stress on pepper. Quant InfraRed Thermogr J. 2018;15(1):81–94. doi: 10.1080/17686733.2017.1331008. [Google Scholar] [CrossRef]
8. Feng R, Zhang YS, Wu JW, Ji RP. Advances in drought remote sensing monitoring based on multispectral and hyperspectral data. J Catastrophol. 2019;162–6 (In Chinese). [Google Scholar]
9. Feng ZH, Song L, Zhang SH, Jing YH. Wheat powdery mildew monitoring based on information fusion of multi-spectral and thermal infrared images acquired with an unmanned aerial vehicle. Scientia Agricultura Sinica. 2022;890–906 (In Chinese). [Google Scholar]
10. Zhang WA, Chen BL. Temperature inversion and recognition of ground objects infrared multispectral data. J Infrared Millim W. 2014;172–6 (In Chinese). [Google Scholar]
11. Li ML. Application analysis of non-destructive testing technology for special equipment. Res Exploration. 2022;147–9 (In Chinese). [Google Scholar]
12. Zhang YX, Wu PY, Wang DD. Mathematical statistical methods commonly used in fingerprints of TCM. Shanghai Med Pharm J. 2019;74–7 (In Chinese). [Google Scholar]
13. Xiao M, Qiu XH, Jie H. Comparison of software tools for mapping knowledge domain. China: Libr J. 2013;61–9 (In Chinese). [Google Scholar]
14. Zhang N, Wang JY. Knowledge mapping based on citespace: research hotspots analysis at home and abroad and situation prospects. Inf Doc Serv. 2017;33–41 (In Chinese). [Google Scholar]
15. Chen Y, Chen CM, Liu ZY, Hu ZG. The methodology function of cite space mapping knowledge domains. Studies Sci Sci. 2015;242–53 (In Chinese). [Google Scholar]
16. Zhang XJ, Li DJ, Liu SH, Wang H, Li XH. Application progress of remote sensing technology in the realization of “double carbon” goal. Spacecraft Recovery & Remote Sens. 2022;106–18 (In Chinese). [Google Scholar]
17. Zhao CJ. Current situations and prospects of smart agriculture. J South China Agric Univ. 2021;1–7 (In Chinese). [Google Scholar]
18. Gao BQ. Food safety problems and countermeasures. China Food Saf Magazine. 2023;28–30 (In Chinese). [Google Scholar]
19. Zhang Y, Zhang X. Intelligent transportation planning practice of station-city integration hub in urban core area (In Chinese). In: 2022 China Urban Trans Plan Annual Meeting. [Google Scholar]
20. Yao P, Li KW. Summary of knowledge graph construction technology. Inf Technol. 2020 (In Chinese). [Google Scholar]
21. Wang Y, Liang ZS. Effect of drought on leaf anatomical characteristics of four Artemisia species in the Loess Plateau. Acta Ecologica Sinica. 2014;4535–48 (In Chinese). [Google Scholar]
22. Cao R, Liang ZS. Effect of progressive drought stress and the subsequent re-watering on leaf nitrogen metabolism cotton seedlings. China: J Nuclear Agric Sci. 2013;231–9 (In Chinese). [Google Scholar]
23. Cai M, Guo Y, Han RL, Liang ZS. Effects of drought stress on protective enzyme activities and membrane system of four native gramineous grass in loess plateau. China: Acta Agriculturae Boreali-Occidentalis Sinica. 2012;98–103+145 (In Chinese). [Google Scholar]
24. Zuo XR, Liang ZS. Effect of progressive drying and rewatering on leaf protective enzyme activities of salvia miltiorrhiza. China: Acta Agric Boreali-Occidentalis Sinica. 2011;110–3 (In Chinese). [Google Scholar]
25. Wang ZC, Liang ZS. Effect of water stress on growth and physiological characteristics of isatis tindigotica root. China: Acta Agricu Boreali-Occidentalis Sinica. 2010;98–103 (In Chinese). [Google Scholar]
26. Gao YT, Yang CY, Zhang R. The effect of water stress on carbon metabolism in the rhizospheric microbiome of greenhouse grape. China: J Irrig Drain. 2022;9–18 (In Chinese). [Google Scholar]
27. Gao YT, Zhang R. Effect of water stress on sugar accumulation and sucrose metabolism enzyme activities of greenhouse grape fruit. China: Arid Zone Res. 2021;1713–21 (In Chinese). [Google Scholar]
28. Yang CY, Zhang R. Effects of water stress on soil enzymes and soil microbial community structure in plant rhizosphere. China: Water Res Plan Des. 2020;93–6 (In Chinese). [Google Scholar]
29. Zhang R, Cheng ZY. Effect of regulated deficit drip irrigation on yield and water use efficiency of plastic film mulched corn for seed. China: J South China Agric Univ. 2009;98–101 (In Chinese). [Google Scholar]
30. Zhang R, Cheng ZY. Effect of regulated deficit drip irrigation on growth characteristic and yield of plastic-film-mulched corn for seed. Agricul Res Arid Areas. 2009;125–8 (In Chinese). [Google Scholar]
31. Xu GW, Lu DK, Liu CJ, Wang HZ, Chen MC. Effect of alternate wetting and drying irrigation and nitrogen coupling on endogenous hormones, nitrogen utilization. China: Trans Chinese Soc Agric Eng. 2018;137–46 (In Chinese). [Google Scholar]
32. Guo WX, Song KJ, Lu DK, Wang HZ. Influence of water management and nitrogen application on rice root and shoot traits. Agron J. 2019;111(5):2232–44. doi: 10.2134/agronj2019.02.0096. [Google Scholar] [CrossRef]
33. Wang HZ, Ma J, Li XY, Zhang RP. Effects of water stress on grain filling and activities of enzymes involved in starch synthesis in rice. Scientia Agric Sinica. 2009;1550–8 (In Chinese). [Google Scholar]
34. Wang HZ, Xu GW. Effects of water stress on growth and yield of rice. China Seed Ind. 2009;47–9 (In Chinese). [Google Scholar]
35. Wang HZ, Ma J, Liu HY. Present situations and prospects of study on rice drought resistance. Chin Agric Sci Bull. 2005;110–113+148 (In Chinese). [Google Scholar]
36. Xu JX, Wang Q, Gao Y, Sun JS. Change in hydraulic characteristics of maize in response to water and salinity stresses. J South China Agric Univ. 2009;98–101 (In Chinese). [Google Scholar]
37. Xu JX, Wang Q, Gao Y, Sun JS. Change in hydraulic characteristics of maize in response to water and salinity stresses. J Irrig Drain. 2020;45–51 (In Chinese). [Google Scholar]
38. Song N, Wang JL, Sun JS, Liu ZG. Effect of water stress on the physiology and biochemistry of leaves of potted winter wheat. China Rural Water and Hydropower. 2011;19–23 (In Chinese). [Google Scholar]
39. Li XD. Effect of different water treatments on growth and yield of winter wheat. J Anhui Agric Sci. 2008;11373–5 (In Chinese). [Google Scholar]
40. Zhang JY, Duan AW, Sun JS, Meng ZJ. Advances in automated monitoring and diagnosis of crop water status. China: Trans Chin Soc Agric Eng. 2006;174–8 (In Chinese). [Google Scholar]
41. Yuan XK, Zhou GS, Wang QL, He QJ. Hyperspectral characteristics of chlorophyll content in summer maize under different water irrigation conditions and its inversion. Acta Ecologica Sinica. 2021;543–52 (In Chinese). [Google Scholar]
42. Feng XY, Zhou GS. Relationship of leaf water content with photosynthesis and soil water content in summer maize. Acta Ecologica Sinica. 2018;177–85 (In Chinese). [Google Scholar]
43. Zhou HL, Zhou GS, Song XY, He QJ. Dynamic characteristics of canopy and vegetation water content during an entire maize growing season in relation to spectral-based indices. Remote Sens. 2022;14(3):584. doi: 10.3390/rs14030584. [Google Scholar] [CrossRef]
44. Li BZ, Zhou GS. Advance in the study on drought index. Acta Ecologica Sinica. 2014;1043–52. [Google Scholar]
45. Wang YL, Xu ZZ, Zhou GS. Changes in biomass allocation and gas exchange characteristics of leymus chinensis in response to soil water stress. Acta Phytoecologica Sinica. 2004;803–9 (In Chinese). [Google Scholar]
46. Li YY, Li YP, Wang N, Li HB, Zhang SQ. Influence of water status on characteristics of dry matter accumulation, distribution, transport and its contribution to grain yield in different ploidy wheat species. J Triticeae Crops. 2018;285–92 (In Chinese). [Google Scholar]
47. Li WR, Li XL, Zhang SQ, Shan L. The root system hydraulic conductivity and water use efficiency of alfalfa and sorghum under water deficit. Acta Ecologica Sinica. 2011;1323–33 (In Chinese). [Google Scholar]
48. Yan MF, Zhang C, Li HB, Zhang L, Ren YY, Chen YL, et al. Root pruning improves maize water-use efficiency by root water absorption. Front Plant Sci. 2023;13. [Google Scholar]
49. Li WR, Zhang SQ, Shan L. Effect of water stress on characteristics of root water uptake and photosynthesis in alfalfa seedlings. Acta Agrestia Sinica. 2007;206–11 (In Chinese). [Google Scholar]
50. Zhang SQ, Li JH, Shan L. Regulation of the plant stomatal movement under drought condition. Acta Botanica Boreali-Occidentalia Sinica. 2001;215–22 (In Chinese). [Google Scholar]
51. Zhao H, Ren LW, Zhao FN, Qi Y. The progress on response of potato to soil water stress. J Arid Meteorol. 2018;537–43 (In Chinese). [Google Scholar]
52. Paula P, Pezzato MM, da Cunha-Santino MB, Irineu B. Net photosynthetic rates of Egeria najas and Utricularia breviscapa changes directed by seasonal hydrological variations. Braz J Bot. 2022;45:1129–38. doi: 10.1007/s40415-022-00828-x. [Google Scholar] [CrossRef]
53. Liu XY, Wang DW, Huang CY, Huang KJ. Study on hyperspectral monitoring of water status in cotton full-boll stage based on fluorescence parameters. Xinjiang Agric Sci. 2018;1177–85 (In Chinese). [Google Scholar]
54. Pei D, Sun ZS, Chen SL, Zhang XY, Chen SY, Wang ZH. Effects of water stress on physiological and agronomic features of winter wheat. Trans Chin Soc Agric Eng. 2006;68–72 (In Chinese). [Google Scholar]
55. Zhu L, Liang ZS, Xu X, Li SH. Correlation between flag leaf carbon isotope discrimination and mineral composition in spring wheat genotypes under three types of soil-water conditions. J China Agric Univ. 2009;66–72 (In Chinese). [Google Scholar]
56. Yao ZZ, Xia GM, Wang SJ, Hu JQ, Chi DC. Research progress of the effects of water stress on peanut. Chin J Oil Crop Sci. 2016;699–704 (In Chinese). [Google Scholar]
57. Li HB, Gui YY, Zhang RH, Wei JJ, Yang RZ, Zhang XQ. Research progress on drought resistance and drought-resistant breeding of sugarcane. Mol Plant Breed. 2019;3406–15 (In Chinese). [Google Scholar]
58. Liu Q, Zhang ZT, Liu C, Jia JD, Huang JL, Guo YH. Improved method of crop water stress index based on UAV remote sensing. Trans Chin Soc Agric Eng. 2023;68–77 (In Chinese). [Google Scholar]
59. Zhang ZT, Yu GD, Wu TK, Zhang YX, Bai XQ, Yang S, et al. Temperature extraction of maize canopy and crop water stress monitoring based on UAV remote sensing images. Trans Chin Soc Agric Eng. 2021;82–9 (In Chinese). [Google Scholar]
60. Han WT, Tang JD, Zhang LY, Niu YX, Wang TH. Maize water use efficiency and biomass estimation based on unmanned aerial vehicle remote sensing. China: Trans Chin Soc Agric Mach. 2021;129–41. [Google Scholar]
61. Mahshad F, Mahdi ST, Hossein B. Determining basil production functions under simultaneous water, salinity, and nitrogen stresses. Appl Water Sci. 2023;1–12. [Google Scholar]
62. Ahmad Z, Ahmad WE, Ashar AM, Usman M, Alharby H, Bamagoos A, et al. Foliar application of phosphorus enhances photosynthesis and biochemical characteristics of maize under drought stress. Phyton-Int J Exp Bot. 2021;90(2):503–14. doi:10.32604/phyton.2021.013588. [Google Scholar] [CrossRef]
63. Vinita Y, Zeeshan I. Relationship of water stress and flood damage for sustainable development. Water Resour Manag. 2022;1323–38. [Google Scholar]
64. Castro-Nava. S, Ortiz-Cereceres J, del C Mendoza-Castillo M, Huerta AJ. Biomass production and grain yield of three sorghum lines differing in drought resistance. Phyton-Int J Exp Bot. 2012;81:149–56. doi:10.32604/phyton.2012.81.149. [Google Scholar] [CrossRef]
65. Ahmad YT, Ateeq M, Wasaya A, Hussain M. Seed priming and foliar supplementation with β-aminobutyric acid alleviates drought stress through mitigation of oxidative stress and enhancement of antioxidant defense in linseed (Linum usitatissimum L.). Phyton-Int J Exp Bot. 2023;92(11):3113–31. doi:10.32604/phyton.2023.029502. [Google Scholar] [CrossRef]
66. Ahmad WE, Ahmed Z, Ahmad Z, Ahmad R, Erman M, Cig F, et al. Alterations in growth and yield of camelina induced by different planting densities under water deficit stress. Phyton-Int J Exp Bot. 2020;89(3):587–97. doi:10.32604/phyton.2020.08734. [Google Scholar] [CrossRef]
Cite This Article
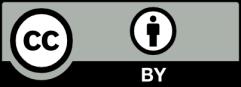
This work is licensed under a Creative Commons Attribution 4.0 International License , which permits unrestricted use, distribution, and reproduction in any medium, provided the original work is properly cited.