Open Access
REVIEW
Research Progress on the Growth-Promoting Effect of Plant Biostimulants on Crops
1 College of Horticulture and Gardening, Hubei Key Laboratory of Waterlogging Disaster and Agricultural Use of Wetland, Yangtze University, Jingzhou, 434020, China
2 Institute of Citrus Research, Zhejiang Academy of Agricultural Sciences, Taizhou, 318026, China
3 Jingzhou Institute of Technology, Jingzhou, 434025, China
* Corresponding Author: Dejian Zhang. Email:
Phyton-International Journal of Experimental Botany 2024, 93(4), 661-679. https://doi.org/10.32604/phyton.2024.049733
Received 16 January 2024; Accepted 07 March 2024; Issue published 29 April 2024
Abstract
A Plant Biostimulant is any substance or microorganism applied to plants to enhance nutrition efficiency, abiotic stress tolerance, and/or crop quality traits, regardless of its nutrient content. The application of Plant biostimulants (PBs) in production can reduce the application of traditional pesticides and chemical fertilizers and improve the quality and yield of crops, which is conducive to the sustainable development of agriculture. An in-depth understanding of the mechanism and effect of various PBs is very important for how to apply PBs reasonably and effectively in the practice of crop production. This paper summarizes the main classification of PBs; The growth promotion mechanism of PBs was analyzed from four aspects: improving soil physical and chemical properties, enhancing crop nutrient absorption capacity, photosynthesis capacity, and abiotic stress tolerance; At the same time, the effects of PBs application on seed germination, seedling vigor, crop yield, and quality were summarized; Finally, how to continue to explore and study the use and mechanism of PBs in the future is analyzed and prospected, to better guide the application of PBs in crop production in the future.Keywords
Plant biostimulants (PBs) refer to any substances (including microorganisms) that can improve plant nutritional efficiency, abiotic stress tolerance, and crop quality traits, and their effects are independent of their nutrient composition or content [1]. PBs are different from traditional fertilizers and pesticides, which directly provide nutrients to improve plant nutrition and alleviate stress. The effect of PBs on plant growth is indirect [2]. By stimulating the natural growth process of plants or improving the growth environment of plants, PBs can improve the ability of plants to absorb and utilize nutrients and their tolerance to abiotic stress [1]. At present, PBs have been widely used in crop production. It effectively reduces the application of chemical fertilizers and pesticides, reduces the damage to the soil environment, and maintains the yield and quality of crops [3]. It is of great significance to the sustainable development of agriculture. Therefore, PBs have a broad market prospect. According to the MarketsandMarkets report, the global market sales of PBs in 2019 were approximately $2.6 billion and are expected to exceed $4 billion in 2025 [4]. In this review, we summarized the classification, biodynamic mechanism, application effect, and future development direction of PBs, to promote the application of PBs in crop production.
2 Main Classification of Plant Biostimulants
2.1 Non-Microbial Plant Biostimulants
Non-microbial PBs mainly include humic substances, seaweed extracts, protein hydrolysates, other nitrogenous substances, chitin and its derivatives, and inorganic compounds with biological stimulatory effects [5]. Humic substances are a class of naturally occurring organic compounds derived from the decomposition and transformation of plant, animal, and microbial residues, which can generally be classified as humin, humic acid, and fulvic acid [6]. Seaweed extracts are naturally active substances extracted from seaweed, which are composed of many different components (including polysaccharides, minerals, phytohormones trace elements, and many other substances) [7]. Moreover, these components will change according to the type of seaweed and collection time as well as the extraction time and method of seaweed extract [8,9]. Protein hydrolysates are mixtures of peptides, oligopeptides, and amino acids formed by partial hydrolysis of various protein sources [10]. Other nitrogen-containing compounds include betaine, polyamines, and non-protein amino acids [1]. Chitin and its derivatives are also a common type of PBs. Chitin is mainly extracted from the shell of crustaceans and the cell wall of fungi. Chitin is insoluble in water and most organic solvents, whereas chitosan is the product of the deacetylation of chitin and has good solubility in acidic solutions [11]. Chitosan oligosaccharides are the products of continuous degradation of chitosan, which have smaller molecular weights, stronger bioactivity, and better water solubility, so they are more convenient to be applied and utilized by plants [12]. Thus, chitosan and chitosaccharides are the main forms of chitin, and its derivatives are used as PBs. The inorganic compounds used as PBs are composed of beneficial elements. Elements that promote plant growth, in addition to those necessary for the plant, are called beneficial elements [13].
2.2 Microbial Plant Biostimulants
Microbial PBs have a great application prospect in the market. Some studies have shown that microbial PBs can help increase crop yield by 10%–40% [14]. They colonize the rhizosphere and plant body to improve the soil environment and plant nutrition, and even protect plants from the effects of pests and diseases [15]. Microbial PBs can be divided into beneficial bacteria and beneficial fungi. Plant growth-promoting bacteria (PGPB), as a representative of PBs in bacteria, can improve nutrient acquisition, growth, and development of plants, and improve the tolerance of plants to abiotic and biotic stresses. Over the years, researchers have isolated and studied a variety of PGPB strains as PBs, among which the most widely studied and used is plant growth-promoting rhizobacteria (PGPR) [16]. Fungi used as PBs mainly include mycorrhizal fungi, yeasts, and fungi of the genus Trichoderma. Mycorrhizal fungi can form mycorrhizae in symbiosis with plant roots to promote plant growth. Arbuscular mycorrhizal fungi (AMF) are the most widely studied and used mycorrhizal fungi. Yeasts and Trichoderma fungi are mainly used to improve plant resistance to biotic stress.
3 Mechanism of Action of Plant Biostimulants to Promote Crop Growth
3.1 Improve Soil Physicochemical Properties
Most PBs are directly applied to soil and have a certain improvement effect on the soil environment. Various PBs can chelate fixed nutrients and decompose mineralized nutrients due to the structural properties or biological activities of their components. Because of its various functional groups and colloidal properties, humus has a high affinity for inorganic and organic ions and many molecules in soil [17]. Therefore, humus can form complexes with metal cations and inorganic phosphorus in soil and improve soil aggregate structure, thereby fixing nutrients to reduce nutrient loss between soils and improving soil physicochemical properties [18–20]. Amino acids have strong chelation ability and can fix metal ions in soil. Amino acids can chelate Fe and other metal ions through their carboxyl groups, such as arginine, glycine, and histidine, to prevent Fe loss and promote Fe absorption by plants [21]. Algal extracts and protein hydrolysates are rich in amino acids. Therefore, these two PBs can also improve soil and improve nutrient availability in soil. Seaweed extracts also contain many kinds of polysaccharides (kelp polysaccharide, rock Ethan, alginic acid, etc.), among which alginic acid is a good chelator, and can form alginate after chelating with metal ions [22]. Alginate has gel properties, which can promote soil aggregate structure, and improve soil physicochemical properties [22]. Other polysaccharides are also important cementing agents, which are conducive to the formation of soil aggregate stability structure [23]. Chitosan is also a polysaccharide. Chitosan could enhance the aggregate structure and permeability of a variety of soils [24]. Chitosan also has very good chelation, and its amino functional group can form a complex with metal ions [25]. PGPR can produce substances that can dissolve minerals, including organic acids and various enzymes, which can improve the availability of elements such as phosphorus and potassium in the soil. In addition, PGPR can produce extracellular siderophores, which can not only complicate with iron ions in soil but also form complexes with other heavy metals, thereby improving the soil environment [26]. The secretion of PGPR and AMF also enhanced the activity of soil phosphatase and promoted the decomposition of organic phosphorus. The extra-root mycelial network formed by mycorrhizal fungi after infection of roots can participate in the formation of microaggregates and maintain the composition of soil aggregates [27]. Soil yeast also can decompose organic matter, dissolve phosphate, and promote soil aggregation [28].
PBs can not only increase the availability of soil nutrients and improve soil physical and chemical properties but also have a positive effect on soil microbial communities. Humus can increase the release of organic acids from the roots of crops such as maize, thereby promoting the activities of microorganisms in the soil, and thus promoting the construction of a good symbiotic relationship between crops and microorganisms [29,30]. Seaweed extracts can also promote the growth of a variety of microorganisms in soil and improve the abundance of soil microorganisms [22].
3.2 Promote Crop Root Growth and Nutrient Uptake
PBs can enhance the endogenous phytohormone content of crop roots to promote root growth. For example, the treatment of cucumber with humic acid can increase the auxin content in its roots [31]; the application of an animal protein hydrolysate to tomato significantly increased endogenous levels of auxin, cytokinin, and jasmonate [32]; the application of kelp extracts to cannabis resulted in an 8-fold increase in auxin content compared to the control [33]. In addition, many PBs also contain phytohormones or phytohormone-like substances that can stimulate root growth and lateral root development. For example, most seaweed extracts contain gibberellins, and protein hydrolysates contain auxin-like and gibberellin-like substances [34,35]. Some microbial PBs can directly produce phytohormones, for example, most PGPR can synthesize and release auxin as secondary metabolites [36]. PBs can not only promote crop root growth but also directly promote crop nutrient absorption. Some studies have shown that humus can induce and stimulate H+-ATPase [37]. H+-ATPase can provide energy for active transport of root cells, thus directly improving the ability of crop roots to acquire nutrients [38]. PBs, such as humus, amino acids, seaweed extracts, and PGPR, can affect the expression of genes and the activity of enzymes related to nutrient transport systems to promote nutrient acquisition [26,39–41]. In addition, most microbial PBs can not only affect the root system by synthesizing secretions but also form symbiotic relationships with roots. For example, commensal nitrogen-fixing bacteria can form a symbiotic relationship with roots to fix nitrogen from the air and provide it to crops, and mycorrhizal fungi can form mycorrhizae in symbiosis with crops to expand the absorption area of crop roots, thereby promoting nutrient absorption of crop roots [26,42].
3.3 Improve the Photosynthetic Efficiency of Crops
Various PBs can improve the photosynthetic efficiency of crops in a variety of ways. Humus can increase the chlorophyll content and leaf photosynthetic rate of crops such as fava beans and peanuts [43,44]. Several amino acid-based PBs have been shown to improve photosynthesis [45]. Seaweed extracts contain substances with cytokine-like activity, which can promote the division and proliferation of chloroplasts [7]. The application of seaweed extract to lettuce resulted in a decrease in the activity of cysteine proteases associated with aging and a significant increase in the expression of genes associated with promoting photosynthesis [46]. Therefore, seaweed extracts can enhance crop chlorophyll content by increasing chloroplast biogenesis, reducing chlorophyll degradation, and delaying chlorophyll senescence. Seaweed extracts, protein hydrolysates, chitosan, and many beneficial elements also enable crops to maintain a good PSII maximum light energy conversion efficiency under various stresses [47,48]. Chitosan can enhance the photosynthetic pigment content of most crops. Wheat seedlings were treated with chitosan and it was found that chitosan may reduce the breakdown of chlorophyll by inhibiting the transcription level of chlorophyllase and thus increase the content of chlorophyll [49]. Spraying chitosan oligosaccharides on leaves increased chlorophyll content and Rubisco activity, thereby accelerating the photosynthetic rate [50]. A beneficial element, titanium (Ti) has also been shown to have this function, and Ti can also induce the expression of crop LHCII-B gene, thereby increasing the content of light-harvesting Complex II (LHCII) in crops [51]. Cerium and lanthanum can not only increase the content of LHCII through the above effects but also promote light energy transfer between LHCII and Photosystem II (PSII) reaction centers [52,53]. In addition, the most beneficial elements can enhance photosynthesis by improving mesophyll conductance and stomatal conductance in crops [48]. Microbial PBs are also able to improve crop photosynthesis Both PGPR and AMF can promote the synthesis of cytokinin to increase chloroplast content, and enhance crop water use efficiency and stomatal conductance to enhance photosynthesis [36,54,55].
3.4 Enhance Crop Tolerance to Abiotic Stresses
Improving the abiotic stress tolerance of crops is one of the main functions of PBs. After suffering from abiotic stress, crops will metabolize different substances to reduce the impact of abiotic stress. PBs can promote and enhance the production and activity of these metabolites [5]. Environmental conditions such as drought and high salt can cause water stress on crops. Crops can produce and accumulate osmoregulatory substances, including inorganic ions such as NA+, K+, and Ga+, and organic solutes such as proline, betaine, and soluble sugar to alleviate water stress [56]. Under abiotic stress conditions, crops can produce a large amount of reactive oxygen species to cause oxidative damage to crops. Crops themselves will produce antioxidant enzymes and non-enzymatic antioxidants to remove reactive oxygen species [57]. Multiple types of PBs can improve the content and activity of the above osmoregulatory substances and antioxidants to alleviate the damage caused by abiotic stress, and thus enhance the tolerance of crops to abiotic stress. For example, seaweed extracts could increase superoxide dismutase activity and proline content in rape under drought conditions [58], and humus increased the activities of superoxide dismutase, peroxidase and catalase and the content of proline in mung bean under both drought and salt stress [59], and inoculation of arbuscular mycorrhizal fungi enhanced the activities of superoxide dismutase, catalase, ascorbate peroxidase and glutathione peroxidase under abiotic stress in crops [60]. Chitin and chitosan could enhance the activities of superoxide dismutase, peroxidase, and catalase in tomatoes [61]. Protein hydrolysates also have this function. In addition, protein hydrolysates can increase the transcription level of genes encoding heat shock proteins and promote the synthesis of heat shock proteins [62]. Heat shock proteins are proteins that are rapidly synthesized by crops under high-temperature stress, and oxidative stress can also stimulate their synthesis. Like functional proteins such as osmoregulatory protein and late embryogenesis abundant protein, it can protect protein structure and enhance membrane stability under abiotic stress conditions [63]. Finally, we summarized the mechanism of action of PBs in Fig. 1.
Figure 1: Schematic diagram of the mechanism of plant biostimulants promoting crop growth
4 Practical Application and Effect of Plant Biostimulant in Production
4.1 Application in Seed Germination and Seedling Cultivation
Higher germination rate, faster seedling emergence rate, good uniformity, and strong seedling growth activity are sought by people in production practice, and the application of PBs can help to achieve such goals. In Table 1, we enumerated the practical applications of various PBs in seed germination and seedling cultivation. The methods used by PBs to promote seed germination are mainly seed priming and seed coating. Seed priming is to allow seeds to absorb water under certain environmental conditions so that they start some important metabolic activities before germination in advance stop the whole process, and dry before the radicle breaks through the seed coat [64]. Seed coating refers to applying a layer of special film to the seed to form coated seeds. Coated seeds can normally absorb and germinate, and coating can slowly release active substances to promote germination and prevent stress [65]. Through these two treatments, PBs increased the activities of biological enzymes and enhanced the plant hormone response of seeds at the early germination stage, thereby increasing germination rate, germination speed, seedling vitality, and controlling seedling emergence time [66,67]. For example, glycine betaine treatment increased α-amylase activity in maize seedlings under both normal and low-temperature stress [68]. On the one hand, the enhancement of phytohormone response in seeds by PBs comes from the regulation of endogenous phytohormone content in seeds [69]. For example, soaking wheat seeds with polyamines under drought conditions can increase the endogenous auxin, abscisic acid, and gibberellin content of seeds. On the other hand, as mentioned above, some PBs contain phytohormones or substances with similar phytohormone activity to provide phytohormone stimulation to seeds.
Treatment of seeds with PBs also improved germination rate and seedling vitality by helping seeds cope with abiotic stresses. Abiotic stresses during seed germination mainly lead to osmotic stress and the accumulation of reactive oxygen species. The mechanism of action of PBs has been described in the previous section PBs regulate plant metabolism to cope with osmotic stress and the accumulation of reactive oxygen species, and also affect seeds. For example, Bacillus was used to soak priming maize seeds can not only increase the activities of superoxide dismutase, catalase, peroxidase, and ascorbic acid peroxidase in maize seeds to reduce the accumulation of reactive oxygen species, but also increase the content of proline to alleviate osmotic stress, and finally improve the emergence rate of maize seeds under salt stress [70], and the use of PBs with lignin derivatives as an active ingredient in soybean seeds can increase the content of non-protein thiol to reduce the content of hydrogen peroxide and alleviate heat stress, thereby improving seed germination rate [71].
In addition, for microbial PBs, the method of seed coating can also increase the colonization efficiency of beneficial microorganisms. For example, on maize, 4860 AMF per plant by soil inoculation and 273 AMF per plant by seed coating resulted in similar colonization rates [72,73].
4.2 Applications in Increasing Crop Yields
Improving crop yield and quality is the main purpose and effect of PBs application in production. In Table 2, we list the practical applications of various PBs in improving crop yield and quality. PBs increased crop yield through the mechanisms described above (improved nutrient uptake capacity and photosynthetic efficiency). For example, the Application of PBs with free amino acids as the main active ingredient in soybean at the leaf development and the beginning of flowering increased soybean yield by 25% and pod and seed number by 32% [95]. Abiotic stress can inhibit plant growth and reduce crop yield. The application of PBs can alleviate stress and improve crop yield. For example, under salt stress at a concentration of 80mM, the growth of tomato was significantly inhibited, and the yield decreased by 33% compared with the normal condition. However, after the application of seaweed extract, the salt stress could be alleviated and the yield of tomato was maintained at the normal level [96].
4.3 Application in Improving Crop Quality
PBs can not only improve crop yield but also improve various qualities of crops. The improvement of crop quality by PBs is mainly reflected in the harvest, that is, PBs are applied during the growing stage of crops to improve various quality indicators of crops. For example, the use of protein hydrolysate in tomatoes can increase the soluble solids, potassium, calcium, magnesium, lycopene, and total ascorbic acid content of tomatoes by 10.7%, 10.5%, 10.7%, 28.3%, 34.9%, and 35.8%, respectively [120]. In addition, PBs can also be applied to improve the quality of crops after harvest, that is, spraying the fruits before harvest or soaking and smearing the fruits after harvest to maintain or improve the quality of crops and prolong the storage time. For example, when stored at 0°C for 60 days, the rot rate of grapes coated with chitosan was reduced by 21.62%, the weight loss rate was reduced by 3.30%, and the content of soluble solids and Vc lost was reduced by 1.54% and 1.43 mg/100g, respectively, compared with grapes without anything applied [121].
The green and sustainable development of agriculture has formed an irreversible trend in the world. People pay more and more attention to the quality and safety of crops, and the development momentum of the PBs industry will be more and more fierce. Therefore, more research and theoretical support are needed for the use of each PB. Future studies on the use and mechanism of PBs can be further explored in the following three aspects.
All kinds of PBs, especially non-microbial PBs, have complex components, and the enhancement of a trait may be the result of single or multiple components in PBs. However, most of the current studies only focus on the effect of overall PBs on plants, and the specific active substances that can trigger plant reactions are not clear. Future studies need to further extract the active substances that may play a role in PBs and verify their effect.
At present, the mechanism of PBs needs to be further studied. The physiological and molecular mechanisms by which PBs regulate biological metabolism remain unclear. In the future, experimental methods such as omics and biotechnology can be used to excavate the plant growth key genes regulated by PBs and analyze the molecular regulatory network of PBs to clarify the action mechanism of PBs.
At present, the combination of different PBs is more and more widely used in practice, especially microbial PBs combined with non-microbial PBs. There are often synergistic and additive effects between different PBs. Studying the effects and reaction mechanisms of multiple PB mixtures can better increase the practical benefits of PBs. This research is likely to become a hot spot for PBS-related research in the future.
Acknowledgement: The authors would like to extend their sincere appreciation to the Researchers Supporting Project Number (32001984).
Funding Statement: This work was supported by the National Natural Science Foundation of China (No. 32001984).
Author Contributions: Conceptualization, D.J.Z. and Q.L.; writing-original draft preparation, Q.L.; writing-review and editing, L.F.J., C.L.T., F.L., and B.H. All authors have read and agreed to the published version of the manuscript.
Availability of Data and Materials: All the data and materials supporting the findings of this study are included in this article.
Ethics Approval: Not applicable.
Conflicts of Interest: The authors declare that they have no conflicts of interest to report regarding the present study.
References
1. Du Jardin P. Plant biostimulants: definition, concept, main categories and regulation. Sci Hortic. 2015;196:3–14. doi:10.1016/j.scienta.2015.09.021. [Google Scholar] [CrossRef]
2. Geelen D, Xu L. The chemical biology of plant biostimulants. Hoboken, NJ, USA: John Wiley & Sons, Ltd.; 2020. [Google Scholar]
3. Calvo P, Nelson L, Kloepper JW. Agricultural uses of plant biostimulants. Plant Soil. 2014;1(2):3–41. doi:10.1007/s11104-014-2131-8. [Google Scholar] [CrossRef]
4. Sible CN, Seebauer JR, Below FE. Plant biostimulants: a categorical review, their implications for row crop production, and relation to soil health indicators. Agron. 2021;11(7):1297. doi:10.3390/agronomy11071297. [Google Scholar] [CrossRef]
5. Franzoni G, Cocetta G, Prinsi B, Ferrante A, Espen L. Biostimulants on crops: their impact under abiotic stress conditions. Horticult. 2022;8(3):189. doi:10.3390/horticulturae8030189. [Google Scholar] [CrossRef]
6. Alessandro P. The supramolecular structure of humic substances. Soil Sci. 2001;166(11):810–32. doi:10.1097/00010694-200111000-00007. [Google Scholar] [CrossRef]
7. Battacharyya D, Babgohari MZ, Rathor P, Prithiviraj B. Seaweed extracts as biostimulants in horticulture. Sci Hortic. 2015;196:39–48. doi:10.1016/j.scienta.2015.09.012. [Google Scholar] [CrossRef]
8. Rioux L, Turgeon SL, Beaulieu M. Effect of season on the composition of bioactive polysaccharides from the brown seaweed Saccharina longicruris. Phytochem. 2009;70(8):1069–75. doi:10.1016/j.phytochem.2009.04.020. [Google Scholar] [PubMed] [CrossRef]
9. Mukherjee A, Patel JS. Seaweed extract: biostimulator of plant defense and plant productivity. Int J Environ Sci Technol. 2020;17(1):553–8. doi:10.1007/s13762-019-02442-z. [Google Scholar] [CrossRef]
10. Schaafsma G. Safety of protein hydrolysates, fractions thereof and bioactive peptides in human nutrition. Eur J Clin Nutr. 2009;63(10):1161–8. doi:10.1038/ejcn.2009.56. [Google Scholar] [PubMed] [CrossRef]
11. Rinaudo M. Chitin and chitosan: properties and applications. Prog Polym Sci. 2006;31(7):603–32. doi:10.1016/j.progpolymsci.2006.06.001. [Google Scholar] [CrossRef]
12. Lodhi G, Kim Y, Hwang J, Kim S, Jeon Y, Je JY. Chitooligosaccharide and its derivatives: preparation and biological applications. Biomed Res Int. 2014;2014(2):1–13. doi:10.1155/2014/654913. [Google Scholar] [PubMed] [CrossRef]
13. Elizabeth AP, Colin FQ, Wiebke T, Mario M, Michela S. Physiological functions of beneficial elements. Curr Opin Plant Biol. 2009;12(3):267–74. doi:10.1016/j.pbi.2009.04.009. [Google Scholar] [PubMed] [CrossRef]
14. Tarigan SI, Toth S, Szalai M, Kiss J, Turoczi G, Toepfer S. Biological control properties of microbial plant biostimulants. A review. Biocontrol Sci Technol. 2022;32(12):1351–71. doi:10.1080/09583157.2022.2129589. [Google Scholar] [CrossRef]
15. Nosheen S, Ajmal I, Song Y. Microbes as biofertilizers, a potential approach for sustainable crop production. Sustain. 2021;13(4):1868. doi:10.3390/su13041868. [Google Scholar] [CrossRef]
16. Pellegrini M, Pagnani G, Bernardi M, Mattedi A, Spera DM, Gallo MD. Cell-free supernatants of plant growth-promoting bacteria: a review of their use as biostimulant and microbial biocontrol agents in sustainable agriculture. Sustain. 2020;12(23):9917. doi:10.3390/su12239917. [Google Scholar] [CrossRef]
17. Rashad M, Hafez M, Popov AI. Humic substances composition and properties as an environmentally sustainable system: a review and way forward to soil conservation. J Plant Nutr. 2022;45(7):1072–122. doi:10.1080/01904167.2021.2005801. [Google Scholar] [CrossRef]
18. García-Mina JM, Antolín MC, Sanchez-Diaz M. Metal-humic complexes and plant micronutrient uptake: a study based on different plant species cultivated in diverse soil types. Plant Soil. 2004;258(1/2):57–68. doi:10.1023/B:PLSO.0000016509.56780.40. [Google Scholar] [CrossRef]
19. Zanin L, Tomasi N, Cesco S, Varanini Z, Pinton R. Humic substances contribute to plant iron nutrition acting as chelators and biostimulants. Front Plant Sci. 2019;10:675. doi:10.3389/fpls.2019.00675. [Google Scholar] [PubMed] [CrossRef]
20. Jindo K, Soares TS, Peres LEP, Azevedo IG, Aguiar NO, Mazzei P, et al. Phosphorus speciation and high-affinity transporters are influenced by humic substances. J Plant Nutr Soil Sci. 2016;179(2):206–14. doi:10.1002/jpln.201500228. [Google Scholar] [CrossRef]
21. Ghasemi S, Khoshgoftarmanesh AH, Hadadzadeh H, Jafari M. Synthesis of iron-amino acid chelates and evaluation of their efficacy as iron source and growth stimulator for tomato in nutrient solution culture. J Plant Growth Regul. 2012;31(4):498–508. doi:10.1007/s00344-012-9259-7. [Google Scholar] [CrossRef]
22. Ali O, Ramsubhag A, Jayaraman J. Biostimulant properties of seaweed extracts in plants: implications towards sustainable crop production. Plants. 2021;10(3):531. doi:10.3390/plants10030531. [Google Scholar] [PubMed] [CrossRef]
23. Martin JP. Decomposition and binding action of polysaccharides in soil. Soil Biol Biochem. 1971;3(1):33–41. doi:10.1016/0038-0717(71)90029-0. [Google Scholar] [CrossRef]
24. Adamczuk A, Jozefaciuk G. Impact of chitosan on the mechanical stability of soils. Molecules. 2022;27(7):2273. doi:10.3390/molecules27072273. [Google Scholar] [PubMed] [CrossRef]
25. Pandey P, Verma MK, De N. Chitosan in agricultural context–A review. Bull Environ Pharmacol Life Sci. 2018;7(4):87–96. [Google Scholar]
26. Ahemad M, Kibret M. Mechanisms and applications of plant growth promoting rhizobacteria: current perspective. J King Saud Univ–Sci. 2014;26(1):1–20. doi:10.1016/j.jksus.2013.05.001. [Google Scholar] [CrossRef]
27. Rillig MC, Mardatin NF, Leifheit EF, Antunes PM. Mycelium of arbuscular mycorrhizal fungi increases soil water repellency and is sufficient to maintain water-stable soil aggregates. Soil Biol Biochem. 2010;42(7):1189–91. doi:10.1016/j.soilbio.2010.03.027. [Google Scholar] [CrossRef]
28. Sarabia M, Cazares S, González-Rodríguez A, Mora F, Carreón-Abud Y, Larsen J. Plant growth promotion traits of rhizosphere yeasts and their response to soil characteristics and crop cycle in maize agroecosystems. Rhizosphere. 2018;6:67–73. doi:10.1016/j.rhisph.2018.04.002. [Google Scholar] [CrossRef]
29. Puglisi E, Fragoulis G, Ricciuti P, Cappa F, Spaccini R, Piccolo A, et al. Effects of a humic acid and its size-fractions on the bacterial community of soil rhizosphere under maize (Zea mays L.). Chemosphere. 2009;77(6):829–37. doi:10.1016/j.chemosphere.2009.07.077. [Google Scholar] [PubMed] [CrossRef]
30. Canellas LP, Olivares FL, Canellas NOA, Mazzei P, Piccolo A. Humic acids increase the maize seedlings exudation yield. Chem Biol Technol Agric. 2019;6(1):3. doi:10.1186/s40538-018-0139-7. [Google Scholar] [CrossRef]
31. Julia I, Oscar M, Analía L, Zocolo Guilherme J, Virginia L. Biofertilization with Macrocystis pyrifera algae extracts combined with PGPR-enhanced growth in Lactuca sativa seedlings. J Appl Phycol. 2020;32(6):4361–71. doi:10.1007/s10811-020-02202-4. [Google Scholar] [CrossRef]
32. Casadesus A, Polo J, Munne-Bosch S. Hormonal effects of an enzymatically hydrolyzed animal protein-based biostimulant (Pepton) in water-stressed tomato plants. Front Plant Sci. 2019;10:758. doi:10.3389/fpls.2019.00758. [Google Scholar] [PubMed] [CrossRef]
33. Wise K, Selby-Pham J, Chai X, Simovich T, Gupta S, Gill H. Fertiliser supplementation with a biostimulant complex of fish hydrolysate, Aloe vera extract, and kelp alters cannabis root architecture to enhance nutrient uptake. Sci Hortic. 2024;323:112483. doi:10.1016/j.scienta.2023.112483. [Google Scholar] [CrossRef]
34. Colla G, Rouphael Y, Canaguier R, Svecova E, Cardarelli M. Biostimulant action of a plant-derived protein hydrolysate produced through enzymatic hydrolysis. Front Plant Sci. 2014;5:1–6. doi:10.3389/fpls.2014.00448. [Google Scholar] [PubMed] [CrossRef]
35. de Hita D, Fuentes M, Fernández V, Zamarreño AM, Olaetxea M, García-Mina JM. Discriminating the short-term action of root and foliar application of humic acids on plant growth: emerging role of jasmonic acid. Front Plant Sci. 2020;11:493. doi:10.3389/fpls.2020.00493. [Google Scholar] [PubMed] [CrossRef]
36. Olanrewaju OS, Glick BR, Babalola OO. Mechanisms of action of plant growth promoting bacteria. World J Microbiol Biotechnol. 2017;33(11):197. doi:10.1007/s11274-017-2364-9. [Google Scholar] [PubMed] [CrossRef]
37. Canellas LP, Olivares FL, Aguiar NO, Jones DL, Nebbioso A, Mazzei P, et al. Humic and fulvic acids as biostimulants in horticulture. Sci Hortic. 2015;196(6):15–27. doi:10.1016/j.scienta.2015.09.013. [Google Scholar] [CrossRef]
38. Zandonadi DB, Santos MP, Caixeta LS, Marinho EB, Peres LEP, Façanha AR. Plant proton pumps as markers of biostimulant action. Sci Agric. 2016;73(1):24–8. doi:10.1590/0103-9016-2015-0076. [Google Scholar] [CrossRef]
39. Elena A, Diane L, Eva B, Marta F, Roberto B, Zamarreño AM, et al. The root application of a purified leonardite humic acid modifies the transcriptional regulation of the main physiological root responses to Fe deficiency in Fe-sufficient cucumber plants. Plant Physiol Biochem. 2009;47(3):215–23. doi:10.1016/j.plaphy.2008.11.013. [Google Scholar] [PubMed] [CrossRef]
40. Jannin L, Arkoun M, Ourry A, Laîné P, Goux D, Garnica M, et al. Microarray analysis of humic acid effects on Brassica napus growth: involvement of N, C and S metabolisms. Plant Soil. 2012;359(1–2):297–319. doi:10.1007/s11104-012-1191-x. [Google Scholar] [CrossRef]
41. Halpern M, Bar-Tal A, Ofek M, Minz D, Muller T, Yermiyahu U. The use of biostimulants for enhancing nutrient uptake. Adv Agron. 2015;130:141. [Google Scholar]
42. Mark CB. Coevolution of roots and mycorrhizas of land plants. New Phytol. 2002;154(2):275–304. doi:10.1046/j.1469-8137.2002.00397.x. [Google Scholar] [PubMed] [CrossRef]
43. Roudgarnejad S, Samdeliri M, Mirkalaei AM, Moghaddam MN. Improving faba bean seed yield, protein and chlorophyll content by foliar application of humic acid. Acta Scientiarum Polonorum Hortorum Cultus. 2022;21(2):115–21. doi:10.24326/asphc.2022.2.10. [Google Scholar] [CrossRef]
44. Li Y, Fang F, Wei J, Cui R, Li G, Zheng F, Tan D. Physiological effects of humic acid in peanut growing in continuous cropping soil. Agron J. 2020;113(1):550–9. doi:10.1002/agj2.20482. [Google Scholar] [CrossRef]
45. Sun W, Shahrajabian MH, Kuang Y, Wang N. Amino acids biostimulants and protein hydrolysates in agricultural sciences. Plants. 2024;13(2):210. doi:10.3390/plants13020210. [Google Scholar] [PubMed] [CrossRef]
46. Jannin L, Arkoun M, Etienne P, Laîné P, Goux D, Garnica M, et al. Brassica napus growth is promoted by Ascophyllum nodosum (L.) Le Jol. seaweed extract: microarray analysis and physiological characterization of N, C, and S metabolisms. J Plant Growth Regul. 2013;32(1):31–52. doi:10.1007/s00344-012-9273-9. [Google Scholar] [CrossRef]
47. Colla G, Nardi S, Cardarelli M, Ertani A, Lucini L, Canaguier R, et al. Protein hydrolysates as biostimulants in horticulture. Sci Hortic. 2015;196(592):28–38. doi:10.1016/j.scienta.2015.08.037. [Google Scholar] [CrossRef]
48. Lo Piccolo E, Ceccanti C, G. L, Landi M. Role of beneficial elements in plants: implications for the photosynthetic process. Photosynthetica. 2021;59(2):349–60. doi:10.32615/ps.2021.032. [Google Scholar] [CrossRef]
49. Mukhtar Ahmed KB, Khan MMA, Siddiqui H, Jahan A. Chitosan and its oligosaccharides, a promising option for sustainable crop production–a review. Carbohyd Polym. 2020;227(C):115331. doi:10.1016/j.carbpol.2019.115331. [Google Scholar] [PubMed] [CrossRef]
50. Li KC, Zhang XQ, Yu Y, Xing RE, Liu S, Li PC. Effect of chitin and chitosan hexamers on growth and photosynthetic characteristics of wheat seedlings. Photosynthetica. 2020;58(3):819–26. doi:10.32615/ps.2020.021. [Google Scholar] [CrossRef]
51. Hussain S, Iqbal N, Brestic M, Raza MA, Pang T, Langham DR, et al. Changes in morphology, chlorophyll fluorescence performance and Rubisco activity of soybean in response to foliar application of ionic titanium under normal light and shade environment. Sci Total Environ. 2019;658(11):626–37. doi:10.1016/j.scitotenv.2018.12.182. [Google Scholar] [PubMed] [CrossRef]
52. Liu XQ, Hao H, Chao L, Min Z, Hong FS. Physico-chemical property of rare earths—effects on the energy regulation of photosystem II in Arabidopsis thaliana. Biol Trace Elem Res. 2009;130(2):141–51. doi:10.1007/s12011-009-8321-1. [Google Scholar] [PubMed] [CrossRef]
53. Liu X, Su M, Liu C, Zhang L, Si W, Hong F. Effects of CeCl3 on energy transfer and oxygen evolution in spinach photosystem II. J Rare Earth. 2007;25(5):624–30. doi:10.1016/S1002-0721(07)60575-4. [Google Scholar] [CrossRef]
54. Zhang J, Bi Y, Song Z, Xiao L, Christie P. Arbuscular mycorrhizal fungi alter root and foliar responses to fissure-induced root damage stress. Ecol Indic. 2021;127(3):107800. doi:10.1016/j.ecolind.2021.107800. [Google Scholar] [CrossRef]
55. Efthimiadou A, Katsenios N, Chanioti S, Giannoglou M, Djordjevic N, Katsaros G. Effect of foliar and soil application of plant growth promoting bacteria on growth, physiology, yield and seed quality of maize under Mediterranean conditions. Sci Rep. 2020;10(1):21060. doi:10.1038/s41598-020-78034-6. [Google Scholar] [PubMed] [CrossRef]
56. Singh M, Kumar J, Singh S, Singh VP, Prasad SM. Roles of osmoprotectants in improving salinity and drought tolerance in plants: a review. Rev Environ Sci Bio/Technol. 2015;14(3):407–26. doi:10.1007/s11157-015-9372-8. [Google Scholar] [CrossRef]
57. Gill SS, Tuteja N. Reactive oxygen species and antioxidant machinery in abiotic stress tolerance in crop plants. Plant Physiol Biochem. 2010;48(12):909–30. doi:10.1016/j.plaphy.2010.08.016. [Google Scholar] [PubMed] [CrossRef]
58. Ghaffar Shahriari A, Mohkami A, Niazi A, Ghodoum Parizipour MH, Habibi-Pirkoohi M. Application of brown algae (Sargassum angustifolium) extract for improvement of drought tolerance in canola (Brassica napus L.). Iran J Biotechnol. 2021;19(1):e2775. doi:10.30498/IJB.2021.2775. [Google Scholar] [PubMed] [CrossRef]
59. Alsamadany H. Physiological, biochemical and molecular evaluation of mungbean genotypes for agronomical yield under drought and salinity stresses in the presence of humic acid. Saudi J Biol Sci. 2022;29(9):103385. doi:10.1016/j.sjbs.2022.103385. [Google Scholar] [PubMed] [CrossRef]
60. Cheng S, Zou Y, Kuča K, Hashem A, Abd_Allah EF, Wu QS. Elucidating the mechanisms underlying enhanced drought tolerance in plants mediated by arbuscular mycorrhizal fungi. Front Microbiol. 2021;12:809473. doi:10.3389/fmicb.2021.809473. [Google Scholar] [PubMed] [CrossRef]
61. Suwanchaikasem P, Nie S, Idnurm A, Selby‐Pham J, Walker R, Boughton BA. Effects of chitin and chitosan on root growth, biochemical defense response and exudate proteome of Cannabis sativa. Plant Environ Interact. 2023;4(3):115–33. doi:10.1002/pei3.10106. [Google Scholar] [PubMed] [CrossRef]
62. Vaseva II, Simova-Stoilova L, Kostadinova A, Yuperlieva-Mateeva B, Karakicheva T, Vassileva V. Heat-stress-mitigating effects of a protein-hydrolysate-based biostimulant are linked to changes in protease, DHN, and HSP gene expression in maize. Agron. 2022;12(5):1127. doi:10.3390/agronomy12051127. [Google Scholar] [CrossRef]
63. Wang W, Vinocur B, Shoseyov O, Altman A. Role of plant heat-shock proteins and molecular chaperones in the abiotic stress response. Trends Plant Sci. 2004;9(5):244–52. doi:10.1016/j.tplants.2004.03.006. [Google Scholar] [PubMed] [CrossRef]
64. Paparella S, Araújo SS, Rossi G, Wijayasinghe M, Carbonera D, Balestrazzi A. Seed priming: state of the art and new perspectives. Plant Cell Rep. 2015;34(8):1281–93. doi:10.1007/s00299-015-1784-y. [Google Scholar] [PubMed] [CrossRef]
65. Afzal I, Javed T, Amirkhani M, Taylor AG. Modern seed technology: seed coating delivery systems for enhancing seed and crop performance. Agric. 2020;10(11):526. doi:10.3390/agriculture10110526. [Google Scholar] [CrossRef]
66. Gupta S, Doležal K, Kulkarni MG, Balázs E, van Staden J. Role of non-microbial biostimulants in regulation of seed germination and seedling establishment. Plant Growth Regul. 2022;97(2):271–313. doi:10.1007/s10725-021-00794-6. [Google Scholar] [CrossRef]
67. Cardarelli M, Woo SL, Rouphael Y, Colla G. Seed treatments with microorganisms can have a biostimulant effect by influencing germination and seedling growth of crops. Plants. 2022;11(3):259. doi:10.3390/plants11030259. [Google Scholar] [PubMed] [CrossRef]
68. Farooq M, Aziz T, Hussain M, Rehman H, Jabran K, Khan MB. Glycinebetaine improves chilling tolerance in hybrid maize. J Agron Crop Sci. 2008;194(2):152–60. doi:10.1111/j.1439-037X.2008.00295.x. [Google Scholar] [CrossRef]
69. Liu Y, Xu H, Wen X, Liao Y. Effect of polyamine on seed germination of wheat under drought stress is related to changes in hormones and carbohydrates. J Integr Agric. 2016;15(12):2759–74. doi:10.1016/S2095-3119(16)61366-7. [Google Scholar] [CrossRef]
70. Li H, Yue H, Li L, Liu Y, Zhang H, Wang J, Jiang X. Seed biostimulant Bacillus sp. MGW9 improves the salt tolerance of maize during seed germination. AMB Express. 2021;11(1):74. doi:10.1186/s13568-021-01237-1. [Google Scholar] [PubMed] [CrossRef]
71. Campobenedetto C, Mannino G, Agliassa C, Acquadro A, Contartese V, Garabello C, et al. Transcriptome analyses and antioxidant activity profiling reveal the role of a lignin-derived biostimulant seed treatment in enhancing heat stress tolerance in soybean. Plants. 2020;9(10):1308. doi:10.3390/plants9101308. [Google Scholar] [PubMed] [CrossRef]
72. Rocha I, Ma Y, Carvalho MF, Magalhães C, Janoušková M, Vosátka M, et al. Seed coating with inocula of arbuscular mycorrhizal fungi and plant growth promoting rhizobacteria for nutritional enhancement of maize under different fertilisation regimes. Arch Agron Soil Sci. 2019;65(1):31–43. doi:10.1080/03650340.2018.1479061. [Google Scholar] [CrossRef]
73. Rocha I, Ma Y, Souza-Alonso P, Vosátka M, Freitas H, Oliveira RS. Seed coating: a tool for delivering beneficial microbes to agricultural crops. Front Plant Sci. 2019;10:1357. doi:10.3389/fpls.2019.01357. [Google Scholar] [PubMed] [CrossRef]
74. Adetumbi JA, Orimadegun IO, Akinyosoye ST, Akintayo OT, Agbeleye OA. Enhancing planting value of rice seed through priming with humic substance. J Exp Agric Int. 2019;29(6):1–8. doi:10.9734/JEAI/2019/46194. [Google Scholar] [CrossRef]
75. Ievinsh G, Vikmane M, Ķirse A, Karlsons A. Effect of vermicompost extract and vermicompost-derived humic acids on seed germination and seedling growth of hemp. Proc Latv Acad Sci. Section B. Nat Exact Appl Sci. 2017;71(4):286–92. doi:10.1515/prolas-2017-0048. [Google Scholar] [CrossRef]
76. Asadi Aghbolaghi M, Sedghi M, Sharifi RS, Dedicova B. Germination and the biochemical response of pumpkin seeds to different concentrations of humic acid under cadmium stress. Agric. 2022;12(3):374. doi:10.3390/agriculture12030374. [Google Scholar] [CrossRef]
77. Çavuşoğlu K, Ergin H, Çavuşoğlu D. Effects of humic acid pretreatment on some physiological and anatomical parameters in barley (Hordeum vulgare L.) exposed to salinity. Bangl J Bot. 2015;4(44):591–8. doi:10.3329/BJB.V44I4.38595. [Google Scholar] [CrossRef]
78. Nemahunguni NK, Gupta S, Kulkarni MG, Finnie JF, van Staden J. The effect of biostimulants and light wavelengths on the physiology of Cleome gynandra seeds. Plant Growth Regul. 2020;90(3):467–74. doi:10.1007/s10725-019-00546-7. [Google Scholar] [CrossRef]
79. Santos PLFD, Zabotto AR, Jordão HWC, Boas RLV, Broetto F, Tavares AR. Use of seaweed-based biostimulant (Ascophyllum nodosum) on ornamental sunflower seed germination and seedling growth. Ornam Hortic. 2019;25(3):231–7. doi:10.1590/2447-536x.v25i3.2044. [Google Scholar] [CrossRef]
80. Liu H, Chen X, Song L, Li K, Zhang X, Liu S, et al. Polysaccharides from Grateloupia filicina enhance tolerance of rice seeds (Oryza sativa L.) under salt stress. Int J Biol Macromol. 2018;124(C):1197–1204. doi:10.1016/j.ijbiomac.2018.11.270. [Google Scholar] [PubMed] [CrossRef]
81. Anjos Neto APD, Oliveira GRF, Mello SDC, Silva MSD, Gomes-Junior FG, Novembre ADDLC. Seed priming with seaweed extract mitigate heat stress in spinach: effect on germination, seedling growth and antioxidant capacity. Bragantia. 2020;79(4):502–11. doi:10.1590/1678-4499.20200127. [Google Scholar] [CrossRef]
82. Wilson HT, Xu K, Taylor AG. Transcriptome analysis of gelatin seed treatment as a biostimulant of cucumber plant growth. Sci World J. 2015;2015(2):1–14. doi:10.1155/2015/391234. [Google Scholar] [PubMed] [CrossRef]
83. Horii A, McCue P, Shetty K. Seed vigour studies in corn, soybean and tomato in response to fish protein hydrolysates and consequences on phenolic-linked responses. Bioresour Technol. 2007;98(11):2170–7. doi:10.1016/j.biortech.2006.08.030. [Google Scholar] [PubMed] [CrossRef]
84. Mo X, Qian J, Liu P, Zeng H, Chen G, Wang Y. Exogenous betaine enhances the protrusion vigor of rice seeds under heat stress by regulating plant hormone signal transduction and its interaction network. Antioxid. 2022;11(9):1792. doi:10.3390/antiox11091792. [Google Scholar] [PubMed] [CrossRef]
85. Guo X, Yu Z, Zhang M, Tang W, Sun Y, Li X. Enhancing the production of phenolic compounds during barley germination by using chitooligosaccharides to improve the antioxidant capacity of malt. Biotechnol Lett. 2018;40(9–10):1335–41. doi:10.1007/s10529-018-2582-8. [Google Scholar] [PubMed] [CrossRef]
86. Zeng D, Luo X, Tu R. Application of bioactive coatings based on chitosan for soybean seed protection. Int J Carbohydr Chem. 2012;2012(2):1–5. doi:10.1155/2012/104565. [Google Scholar] [CrossRef]
87. Samarah NH, AL-Quraan NA, Massad RS, Welbaum GE. Treatment of bell pepper (Capsicum annuum L.) seeds with chitosan increases chitinase and glucanase activities and enhances emergence in a standard cold test. Sci Hortic. 2020;269:109393. doi:10.1016/j.scienta.2020.109393. [Google Scholar] [CrossRef]
88. Siddiqui SA, Blinov AV, Serov AV, Gvozdenko AA, Kravtsov AA, Nagdalian AA, et al. Effect of selenium nanoparticles on germination of hordéum vulgáre barley seeds. Coatings. 2021;11(7):862. doi:10.3390/coatings11070862. [Google Scholar] [CrossRef]
89. Hussain S, Ahmed S, Akram W, Li G, Yasin NA. Selenium seed priming enhanced the growth of salt-stressed Brassica rapa L. through improving plant nutrition and the antioxidant system. Front Plant Sci. 2023;13:1050359. doi:10.3389/fpls.2022.1050359. [Google Scholar] [PubMed] [CrossRef]
90. Haghighi M, Teixeira DS, Mozafarian JA, Afifipour Z. Can Si and nano-Si alleviate the effect of drought stress induced by PEG in seed germination and seedling growth of tomato? Minerva Biotecnol. 2013;25(1):17–22. [Google Scholar]
91. Hong FS, Ling W, Chao L. Study of lanthanum on seed germination and growth of rice. Biol Trace Elem Res. 2003;94(3):273–86. doi:10.1385/BTER:94:3:273. [Google Scholar] [PubMed] [CrossRef]
92. Kthiri Z, Jabeur MB, Machraoui M, Gargouri S, Hiba K, Hamada W. Coating seeds with Trichoderma strains promotes plant growth and enhance the systemic resistance against Fusarium crown rot in durum wheat. Egypt J Biol Pest Control. 2020;30(1):1–10. doi:10.1186/s41938-020-00338-6. [Google Scholar] [CrossRef]
93. Yusnawan E, Inayati A, Baliadi Y, Romaidi, Wahyudi D, Daryono, et al. Effect of soybean seed treatment with Trichoderma virens on its growth and total phenolic content. Aip Conf Proc. 2019;2120(1):20003. doi:10.1063/1.5115604. [Google Scholar] [CrossRef]
94. Somagh HA, Mousavi SM, Omidi H, Elnaz M, Hemmati M. Canola seed germination and seedling growth in response to saline condition and bio-priming. Iran J Plant Physiol. 2017;7(4):2149–56. doi:10.22034/ijpp.2017.537979. [Google Scholar] [CrossRef]
95. Kocira S. Effect of amino acid biostimulant on the yield and nutraceutical potential of soybean. Chil J Agr Res. 2019;79(1):17–25. doi:10.4067/S0718-58392019000100017. [Google Scholar] [CrossRef]
96. di Stasio E, Cirillo V, Raimondi G, Giordano M, Esposito M, Maggio A. Osmo-priming with seaweed extracts enhances yield of salt-stressed tomato plants. Agron. 2020;10(10):1559. doi:10.3390/agronomy10101559. [Google Scholar] [CrossRef]
97. Zhang P, Zhang H, Wu G, Chen X, Gruda N, Li X, Dong J, Duan Z. Dose-dependent application of straw-derived fulvic acid on yield and quality of tomato plants grown in a greenhouse. Front Plant Sci. 2021;12:736613. doi:10.3389/fpls.2021.736613. [Google Scholar] [PubMed] [CrossRef]
98. Kahraman A. Effect of humic acid doses on yield and quality parameters of cowpea [Vigna unguiculata (L.) Walp] cultivars. Legume Res. 2017;40(1):155–9. doi:10.18805/lr.v0iOF.3763. [Google Scholar] [CrossRef]
99. Saidimoradi D, Ghaderi N, Javadi T. Salinity stress mitigation by humic acid application in strawberry (Fragaria x ananassa Duch.). Sci Hortic. 2019;256(3):108594. doi:10.1016/j.scienta.2019.108594. [Google Scholar] [CrossRef]
100. Rady MM, El-Mageed TAA, Abdurrahman HA, Mahdi AH. Humic acid application improves field performance of cotton (Gossypium barbadense L.) under saline conditions. J Anim Plant Sci. 2016;26(2):487. [Google Scholar]
101. Rathore SS, Chaudhary DR, Boricha GN, Ghosh A, Bhatt BP, Zodape ST, et al. Effect of seaweed extract on the growth, yield and nutrient uptake of soybean (Glycine max) under rainfed conditions. South Afr J Bot. 2009;75(2):351–5. doi:10.1016/j.sajb.2008.10.009. [Google Scholar] [CrossRef]
102. Mahmoud SH, Salama DM, El-Tanahy AMM, Abd El-Samad EH. Utilization of seaweed (Sargassum vulgare) extract to enhance growth, yield and nutritional quality of red radish plants. Ann Agric Sci. 2019;64(2):167–75. doi:10.1016/j.aoas.2019.11.002. [Google Scholar] [CrossRef]
103. Hidangmayum A, Sharma R. Effect of different concentrations of commercial seaweed liquid extract of Ascophyllum nodosum as a plant bio stimulant on growth, yield and biochemical constituents of onion (Allium cepa L.). J Pharmacog Phytochem. 2017;6(4):658–63. [Google Scholar]
104. Aziz NGA, Mahgoub MH, Siam SH. Growth, flowering and chemical constituents performence of Amaranthus tricolor plants as influenced by seaweed (Ascophyllum nodosum) extract application under salt stress conditions. J Appl Sci Res. 2011;7(11):1472–84. [Google Scholar]
105. El-Sanatawy AAM, Ash-Shormillesy SMAI, El-Yazied AA, El-Gawad HGA, Azab E, Gobouri AA, et al. Enhancing grain yield and nitrogen accumulation in wheat plants grown under a mediterranean arid environment by foliar spray with papain-released whey peptides. Agronomy. 2021;11(10):1913. doi:10.3390/agronomy11101913. [Google Scholar] [CrossRef]
106. Lucini L, Rouphael Y, Cardarelli M, Canaguier R, Kumar P, Colla G. The effect of a plant-derived biostimulant on metabolic profiling and crop performance of lettuce grown under saline conditions. Sci Hortic. 2015;182(448):124–33. doi:10.1016/j.scienta.2014.11.022. [Google Scholar] [CrossRef]
107. Berahim Z, Dorairaj D, Omar MH, Saud HM, Ismail MR. Spermine mediated improvements on stomatal features, growth, grain filling and yield of rice under differing water availability. Sci Rep. 2021;11(1):10669. doi:10.1038/s41598-021-89812-1. [Google Scholar] [PubMed] [CrossRef]
108. Chen F, Li Q, Su Y, Lei Y, Zhang C. Chitosan spraying enhances the growth, photosynthesis, and resistance of continuous Pinellia ternata and promotes its yield and quality. Molecules. 2023;28(5):2053. doi:10.3390/molecules28052053. [Google Scholar] [PubMed] [CrossRef]
109. Hernández V, Botella MÁ., Hellín P, Fenoll J, Flores P. Dose-dependent potential of chitosan to increase yield or bioactive compound content in tomatoes. Horticult. 2022;8(12):1152. doi:10.3390/horticulturae8121152. [Google Scholar] [CrossRef]
110. Ahmed HHA, Mohamed RAN, Hesham AA, Amira FE. Effect of pre-harvest chitosan foliar application on growth, yield and chemical composition of Washington navel orange trees grown in two different regions. Afr J Biochem Res. 2016;10(7):59–69. doi:10.5897/AJBR2016.0908. [Google Scholar] [CrossRef]
111. Efthimiadou A, Katsenios N, Chanioti S, Giannoglou M, Djordjevic N, Katsaros G. Effect of foliar application of chitosan on growth and yield in okra. Austr J Crop Sci. 2012;6(5):918–21. [Google Scholar]
112. Tao J, Leng J, Lei X, Wan C, Li D, Wu Y, et al. Effects of selenium (Se) uptake on plant growth and yield in common buckwheat (Fagopyrum esculentum Moench). Field Crop Res. 2023;302(363):109070. doi:10.1016/j.fcr.2023.109070. [Google Scholar] [CrossRef]
113. Luo H, Chen Y, He L, Tang X. Lanthanum (La) improves growth, yield formation and 2-acetyl-1-pyrroline biosynthesis in aromatic rice (Oryza sativa L.). BMC Plant Biol. 2021;21(1):1–13. doi:10.1186/s12870-021-03006-5. [Google Scholar] [PubMed] [CrossRef]
114. Djanaguiraman M, Nair R, Giraldo JP, Prasad PVV. Cerium oxide nanoparticles decrease drought-induced oxidative damage in sorghum leading to higher photosynthesis and grain yield. ACS Omega. 2018;3(10):14406–16. doi:10.1021/acsomega.8b01894. [Google Scholar] [PubMed] [CrossRef]
115. Akhter MS, Noreen S, Mahmood S, Aqeel M, Zafar ZU, Rashid M, et al. Silicon supplement improves growth and yield under salt stress by modulating ionic homeostasis and some physiological indices in Hordeum vulgare L. J Soil Sci Plant Nut. 2023;23(2):1694–712. doi:10.1007/s42729-023-01240-4. [Google Scholar] [CrossRef]
116. Asafor HC, Kladjim NH, Souleymanou A, Marie SM, Meka SS, Honore BD, et al. Growth and yield response of groundnut (Arachis hypogaea L.) to rhizobial and arbuscular mycorrhiza fungal inoculations in the western highlands of cameroon. Plant. 2022;10(3):633. doi:10.11648/j.plant.20221003.11. [Google Scholar] [CrossRef]
117. Agbodjato NA, Assogba SA, Babalola OO, Koda AD, Aguégué RM, Sina H, et al. Formulation of biostimulants based on arbuscular mycorrhizal fungi for maize growth and yield. Front Agron. 2022;4:899489. doi:10.3389/fagro.2022.894489. [Google Scholar] [CrossRef]
118. Jumrani K, Bhatia VS, Kataria S, Alamri SA, Siddiqui MH, Rastogi A. Inoculation with arbuscular mycorrhizal fungi alleviates the adverse effects of high temperature in soybean. Plants. 2022;11(17):2210. doi:10.3390/plants11172210. [Google Scholar] [PubMed] [CrossRef]
119. Gul S, Javed S, Azeem M, Aftab A, Anwaar N, Mehmood T, Zeshan B. Application of bacillus subtilis for the alleviation of salinity stress in different cultivars of wheat (Tritium aestivum L.). Agron. 2023;13(2):437. doi:10.3390/agronomy13020437. [Google Scholar] [CrossRef]
120. Rouphael Y, Colla G, Giordano M, El-Nakhel C, Kyriacou MC, de Pascale S. Foliar applications of a legume-derived protein hydrolysate elicit dose-dependent increases of growth, leaf mineral composition, yield and fruit quality in two greenhouse tomato cultivars. Sci Hortic. 2017;226(9):353–60. doi:10.1016/j.scienta.2017.09.007. [Google Scholar] [CrossRef]
121. Gao P, Zhu Z, Zhang P. Effects of chitosan-glucose complex coating on postharvest quality and shelf life of table grapes. Carbohyd Polym. 2013;95(1):371–8. doi:10.1016/j.carbpol.2013.03.029. [Google Scholar] [PubMed] [CrossRef]
Cite This Article
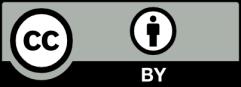
This work is licensed under a Creative Commons Attribution 4.0 International License , which permits unrestricted use, distribution, and reproduction in any medium, provided the original work is properly cited.