Open Access
ARTICLE
Effects of Potassium-Solubilizing Bacteria on Growth, Antioxidant Activity and Expression of Related Genes in Fritillaria taipaiensis P. Y. Li
1 Chongqing Engineering Laboratory of Green Planting and Deep Processing of Famous-Region Drug in the Three Gorges Reservoir Region, College of Biology and Food Engineering, Chongqing Three Gorges University, Chongqing, 404120, China
2 College of Modern Chinese Medicine Industry, Chengdu University of Traditional Chinese Medicine, Chengdu, 611130, China
3 College of Pharmacy, Dali University, Dali, 671000, China
* Corresponding Author: Nong Zhou. Email:
(This article belongs to the Special Issue: Microbial Biotechnology Applications on Plant Performance and Physiology for Sustainable Production )
Phyton-International Journal of Experimental Botany 2024, 93(4), 789-806. https://doi.org/10.32604/phyton.2024.049088
Received 27 December 2023; Accepted 19 March 2024; Issue published 29 April 2024
Abstract
This study aimed to examine the effects of inoculating Fritillaria taipaiensis P.Y.Li leaves with different strains of potassium-solubilizing bacteria (KSB), or combinations thereof, focusing on aspects of photosynthesis and physiological and biochemical characteristics. At present, some studies have only studied the rhizosphere microbial community characteristics of F. taipaiensis and have not discussed the effects of different microbial species on the growth promotion of F. taipaiensis. This paper will start from the perspective of potassium-solubilizing bacteria to conduct an in-depth study. Seed cultivation commenced at the base with three different KSBs in early October 2022. The growth of F. taipaiensis leaves was observed after different treatments. Both single-plant and compound inoculations were executed. A total of eight treatment groups were established, with aseptic fertilizer and sterilized soil functioning as the control group. The results reveal that intercellular CO concentration (Ci), stomatal conductance (Gs), and transpiration rate (Tr) were at their apex in the S7 group. Most treatment groups exhibited an increase in leaf area, photosynthetic pigment content, soluble sugar, soluble protein, Superoxide Dismutase (SOD), Peroxidase (POD), Catalase (CAT) activities, and proline content. The expression levels of POD, SOD, and CAT genes were evaluated, following inoculation with different KSB. The highest was the S7 group. The inoculation with various KSB, or combinations thereof, appears to bolster the growth and development of F. taipaiensis. The composite inoculation group S7, comprising Bacillus cereus, Burkholderia cepacia, and Bacillus subtilis, manifested the most favorable impact on the diverse indices of F. taipaiensis, thereby furnishing valuable insights for the selection of bacterial fertilizer in the artificial cultivation of F. taipaiensis.Keywords
F. taipaiensis is one of the foundational species of Fritillaria cirrhosa D. Don [1] and possesses therapeutic properties such as heat-clearing, lung-moistening, phlegm-resolving, and cough-relieving [2–5]. With growing insights into the medicinal value of Fritillaria cirrhosa D. Don and concurrent market demand, F. taipaiensis has emerged as a successful specimen in the artificial cultivation of Fritillaria cirrhosa D. Don [3]. This species is endowed with several natural advantages, including strong adaptability, mature production technology, high yield, good quality, and low altitude cultivation. However, the cultivation of F. taipaiensis remains limited within China.
Native to the towering mountain ranges of Southwest China [4–6], F. taipaiensis is cultivated on a modest scale in regions such as Sichuan, Shaanxi, and Chongqing. Advancements in artificial cultivation technology have fostered improvements in the quality and safety of F. taipaiensis [7]. However, the extensive use of chemical fertilizers has engendered a severe nutrient imbalance within the soil [8–11], thus gravely impairing the harvest of high-quality fritillaria. This presents a complex challenge in the artificial cultivation of F. taipaiensis, creating a pressing need to enhance the quality of this botanical species.
Previous research by Zhang et al. [12] found that cross-planting techniques could optimize the light conditions for the F. taipaiensis population and that bacterial fertilizer could enhance growth. Dong et al. [13] observed that the application of N-P fertilizer and microbial fertilizer increased soil microorganism counts in F. taipaiensis cultivation. From the perspective of mineral elements, Zhang et al. [14] have explored microbial impacts on plant growth. Effective application of plant-beneficial microorganisms can promote plant growth and increase crop yield. Microbial fertilizer can make up for the damage of NPK fertilizer to soil. For the development of a green agriculture strategy, plant-beneficial microorganisms will become an important member of the fertilizer field in the future. Furthermore, a past study [15] demonstrated a strong correlation between the quality of F. taipaiensis and rhizosphere soil factors. Inoculation with different AM fungi appeared to boost growth and significantly impact the yield and quality of F. taipaiensis.
Yet, studies on F. taipaiensis concerning leaf growth, physiological and biochemical indices, and related genes in conjunction with potassium-solubilizing bacteria (KSB) remain scarce. KSB can secrete gibberellin and auxin. Some bacteria can produce IAA, which is an important component of soil microorganisms. Some KSB can also fix nitrogen, solubilize phosphorus, and produce plant growth hormone and organic acid, it can promote the growth and development of plants through various direct or indirect actions. However, there is no study on the variation of potassium forms in soil inoculation of KSB into F. taipaiensis has not been studied. Since the leaf is the principal organ responsible for photosynthesis in plants [16–18], the distribution of effective components within F. taipaiensis can be ascertained through leaf growth and photosynthesis parameters. This, in turn, establishes a crucial theoretical foundation for scientific fertilization and rational cultivation techniques of F. taipaiensis.
In the present study, a systematic examination was conducted employing seven distinct treatments involving KSB and their combinations to inoculate 3- and 4-year-old bulblets of F. taipaiensis with exogenous KSB. Specifically, this investigation involved a rigorous assessment of growth phenotype indices (including plant height, and stem diameter), antioxidant enzyme activities, and osmotic adjustment substances. Utilizing qRT-PCR techniques, the expression levels of antioxidant enzyme genes in leaves were analyzed.
The objective of this research endeavor was to ascertain whether the presence of KSB in the soil rhizosphere could augment the growth of F. taipaiensis, enrich the content of photosynthetic pigment, and enhance its physiological and biochemical characteristics, alongside the content of associated regulatory genes, all to improve the quality of F. taipaiensis.
Further investigation was devoted to exploring the influence of KSB on F. taipaiensis growth, by determining the number of leaves, leaf length and width, stem diameter, plant height, leaf area and thickness, photosynthetic parameters, photosynthetic pigment content, soluble sugar and protein, activities of Superoxide dismutase (SOD), Peroxidase (POD), Catalase (CAT), malondialdehyde (MDA), and proline content, in addition to expression levels of SOD, POD and CAT genes in the leaves. The subsequent findings revealed that the activities of the aforementioned three antioxidant enzymes play an instrumental role in bolstering the plant’s resistance potential [19,20].
In summation, this study furnishes a substantial theoretical underpinning for the standardized cultivation and yield enhancement of F. taipaiensis and paves the way for future improvements in the quality and safety facilitated by the strategic deployment of KSB in rhizosphere soil.
The experiment was conducted from 2022 to 2023 at the F. taipaiensis with a four-year-old bulb planting base located in the scenic area of Hongchiba, Wuxi County, Chongqing City (108°94′22″E, 31°63′55″N), in the context of the project. The site experiences a sub-tropical monsoon climate characterized by four distinct seasons and a pronounced three-dimensional weather pattern. Situated at an altitude ranging from 1,800 to 2,630 m, the location records an annual average temperature below 17°C. The soil predominantly consists of yellow-brown soil [15], and it is categorized under the middle mountain ridge valley brown soil and yellow-brown soil mountainous region, exhibiting high soil fertility. The organic and inorganic acids produced by KSB can directly promote the weathering of potassium-bearing minerals by reducing the pH of the surrounding environment, resulting in the slow release of exchangeable potassium and increasing the content of environmentally available potassium. In this study, the loose soil was used after high-temperature sterilization, the soil was black-brown, had good adhesion, and the basic soil pH was about 7.1, the following Table 1 shows the content of a large number of elements and organic matter in the soil.
F. taipaiensis sourced from the Fritillaria base in Taibai, Wuxi County, was identified as F. taipaiensis by Professor Zhou Nong of Chongqing Three Gorges University. Seed cultivation commenced at the base in early October 2022. Seeds that exhibited favorable growth and similar size were earmarked for cultivation. Water was administered regularly before the inoculation treatment to preserve normal growth, pending plant germination and inoculation treatment. The pots used had a bottom diameter of 18 cm, a top diameter of 19.8 cm, and a height of 20 cm. Before use, each pot was thoroughly cleaned with 75% alcohol three times. Each pot contained five bulbs of F. taipaiensis. The concentration of the three inoculated bacteria was controlled at 2 * 108 CFU/mL.
The test soil comprised a blend of yellow loam, river sand, and organic fertilizer (2:1:1 ratio). The soil was obtained from Chongqing Three Gorges College, sterilized at 121°C for 2 h, and rested for 7 days before use.
The bacterial strain comprised Bacillus subtilis, Bacillus cereus, and Burkholderia cepacia, all of which were isolated from the rhizosphere soil of F. taipaiensis by the research group of the Biology and Food Engineering College of Chongqing Three Gorges University. After a comprehensive screening of the growth-promoting indicators of phosphate solubilization, potassium solubilization, iron production, and ability to produce IAA. The indexes of the three dominant growth-promoting bacteria are shown in Table 2. It was purified and identified on an LB bacterial culture medium (Manufacturer: Qingdao Hope Biotechnology Co., Ltd., China). The strains originated from several locations including Hongchiba National Forest Park, Wuxi County, Wushan County, Chongqing, Dangyang, Lihe Village, Xicao Village, Xinglong Town, Fengjie County, Chongqing.
The experiment was initiated in October 2022 at the experimental planting base within the Hongchiba scenic spot in Wuxi County, Chongqing. Utilizing plastic flower pots with diameters of 15 cm and heights of 20 cm, anhydrous alcohol was administered thrice as a preparatory measure before the test. Subsequent inoculations were performed twice, specifically in March and early April of 2023. About 100 ml suspension was inoculated in each basin in the single-strain inoculation group, 50 ml suspension in each basin in the two-strain inoculation group, and about 35 ml in each basin in the three-strain inoculation group. The control group received identical quantities of sterile water. Throughout the experiment and research, seven treatment groups were constituted, labeled S1 through S7. The control group, designated control group, was sterilized at high temperatures and remained uninoculated with bacteria. Three bacterial strains were deployed in this investigation, and they were inoculated individually, in two-group mixed inoculation, and three-group mixed inoculation respectively. The other daily management adhered to the conventional procedures for F.taipaiensis. Approximately five bulblets of F. taipaiensis were embedded in each pot, and ten replicates were designated for each treatment group. The specific inoculation conditions for the various treatment groups are elucidated in Table 3.
2.4 Determination of Indicators and Methods
In the early days of May of the same year, the growth indices of F. taipaiensis were evaluated, including parameters such as leaf quantity, stem diameter, plant stature, and leaf thickness using a caliper; leaf area was measured employing a leaf area meter, and photosynthetic aspects were quantified with a photosynthesis instrument. After the completion of these measurements at the base, fresh leaf samples were collected, transported to the laboratory in containers filled with liquid nitrogen, and then cleansed and desiccated with distilled water before being bifurcated. One segment was preserved at −80°C for subsequent gene expression analysis, while the other fresh specimen was utilized for determining light and pigment contents, the activities of SOD, POD, and CAT, and the concentration of soluble protein, MDA, and soluble sugar in the leaves of F. taipaiensis.
2.4.1 Determination of Growth Index of F. taipaiensis
After a period of 7 months following plantation, when the leaves of F. taipaiensis had reached their optimal growth phase, ten specimens were selected from various treatment regions after full leaf extension (between 10:00–11:00). The number of leaves, plant height, stem diameter, and leaf thickness of each F. taipaiensis were measured employing either a ruler or Vernier scale. The leaf area of F. taipaiensis was measured using a portable laser leaf area scanner (CI-203, CID, USA).
2.4.2 Leaf Area, Photosynthetic Parameters, and Photosynthetic Pigment Content of F. taipaiensis
The leaf area of F. taipaiensis was ascertained using a leaf area meter. The light and pigment contents were determined according to Shu et al. [21], while the Chlorophyll and Carotenoid contents were analyzed using the UV method. During the apex of the growth period, a portable LI-6400 photosynthesis analyzer was employed [12], and the NET photosynthetic rate (Pn), intercellular CO2 concentration (Ci), stomatal conductance (Gs), and transpiration rate (Tr) of the leaves were measured under illuminated conditions. The external CO2 concentration is [Ca, 285umol/mol]. Water use efficiency (WUE, umol/mol) was calculated by PN/TR according to Wang et al. [22], which calculated the stomata limitation (Ls) = 1 − Ci/Ca.
2.4.3 The Contents of Antioxidant Enzyme, Malondialdehyde (MDA), Soluble Sugar, and Soluble Protein Were Determined
At approximately 11:00 am during the peak growth phase, 1 g of fresh leaves was harvested for the measurement of SOD values through the nitrogen blue tetrazolium method [23]. POD values were ascertained via the guaiacol chromogenic method [24], with a 0.01 per minute increase in light absorption defined as a unit of enzyme activity (U). CAT values were measured with a UV photometer, and the concentrations of soluble proteins, soluble sugars, and MDA were determined by the Coomassie Brilliant Blue method [25] and barbituric acid method [26], respectively, using a UV photometer (UV-2450, Shimadzu, Japan).
2.4.4 Expression of Genes Related to Antioxidant Enzyme System
Total RNA was extracted from the leaves of F. taipaiensis using the TRIzol Plus RNA Purification Kit (Invitrogen, 12183-555). The purity, concentration, and quality of RNA were quantified by UV photometer and electrophoresis and subsequently stored at −80°C. The SuperScript™ III First-Strand Synthesis SuperMix for qRT-PCR reverse transcription kit (Invitrogen serial number: 11752-050) was employed, and high-quality RNA samples were reverse-transcribed into cDNA following the detailed instructions and reaction conditions: 25°C, 10 min; 50°C, 30 min; 85°C, 5 min, and kept at −20°C for future use. qRT-PCR was executed using the Power SYBR® Green PCR Master Mix reagent (Roche number: 4913914001), with triplicate samples for each condition.
The relative gene expression was quantified based on the CT value, and the real-time PCR of the amplification system and reaction conditions is depicted in Table 4. Reaction conditions: 95°C 1 min, 40 cycles; 95°C, 15 s, 63°C, 25 s, fluorescence collection, 55°C~95°C melting point curve.
Quantitative PCR Primer design was performed using Premier 6.0 and Beacon Designer 7.8 software, and then the primers were synthesized. The Primer sequences are as Table 5, and the relative expression of the target gene under different treatments was calculated according to the 2−∆∆Ct method [27,28].
The experimental data were processed utilizing Microsoft Excel 2019 (2019 version, Microsoft, America), and subsequent statistical analyses were performed employing SPSS 23.0 (23.0 version, IBM, USA) and Origin 2023 (2023 version, OriginLab, USA) software packages.
3.1 Effect of Inoculation with Different KSB on the Growth Indexes of F. taipaiensis
The growth indices of F. taipaiensis were ascertained employing the method delineated in above. When juxtaposed with the control group, the indices including leaf number, leaf length, leaf width, plant height, stem diameter, and leaf thickness of F. taipaiensis exhibited enhancement in the majority of the treatment groups inoculated with diverse KSB, as compared to the control groups (Table 6). Statistically, the growth indices of the treatment groups were markedly different from those of the control group (p < 0.05).
About the leaf count in each group, barring group S1, which was marginally inferior to control group, the leaf numbers in the remaining treatment groups exceeded that in control group. Among these, group S7 exhibited the maximum number of leaves, averaging 8 to 10 leaves per F. taipaiensis. Notably, the combined strains (S4, S5, S6, S7) manifested greater leaf numbers compared to the single strains (S1, S2, S3). The preeminent leaf length was observed in group S7, whereas the maximum leaf width was identified in group S4 (p < 0.05). In comparison to the control groups, the plant height in the KSB-inoculated groups was augmented, with the S4 and S7 groups attaining the zenith, 2.72 and 2.57 times respectively greater than the control group. Furthermore, the stem diameter of F. taipaiensis inoculated with KSB was superior to that of the control group, peaking in the S7 group at approximately 1.24 mm, while the leaf thickness was the most pronounced in group S1, succeeded by groups S2 and S7. The leaf area of F. taipaiensis exceeded that of control group across all treatments (p < 0.05); specifically, the leaf area of group S4 experienced an increase of 52.49% in comparison with the control group, and this difference was significant among most treatment groups (p < 0.05).
Empirical data has corroborated that the utilization of KSB for inoculation enables plants to more effectively capitalize on light. Moreover, bacterial fertilizers can foster a symbiotic alliance with the roots of F. taipaiensis, thereby facilitating plant growth [29]. Fig. 1 illustrates the leaf number, plant height, and leaf length of F. taipaiensis. inoculated with varying KSB. In comparison with the control group, the leaf number, plant height, and mean leaf length manifested significant augmentation, an indication that inoculation with KSB can efficaciously bolster the growth of leaves of F. taipaiensis, with pronounced disparities among the groups (p < 0.05).
Figure 1: Comparison of growth indexes of F. taipaiensis under different treatments (average ± SD CV, n = 6)
Note: Different lowercase letters indicate significant differences at 0.05 level.
Fig. 2 delineates the leaf width, stem diameter, and leaf thickness of F. taipaiensis inoculated with diverse KSB. When contrasted with the control group, both the leaf width and mean stem diameter of F. taipaiensis were significantly amplified. This suggests that inoculation with KSB can indeed spur the growth of F. taipaiensis leaves and plants, albeit without any conspicuous effect on leaf thickness. Significant differences were detected among the groups (p < 0.05).
Figure 2: Comparison of growth indexes of F. taipaiensis under different treatments (average ± SD CV, n = 6)
Note: Different lowercase letters indicate significant differences at 0.05 level.
3.2 Effect of Inoculation with Different KSB on Photosynthesis of F. taipaiensis
The comparative analysis of photosynthetic parameters and photosynthetic pigment content of F. taipaiensis across diverse treatments is tabulated in Table 7. The NET photosynthetic rate (Pn), intercellular CO2 concentration (Ci), stomatal conductance (Gs), and transpiration rate (Tr) were notably superior to those of the control group, with statistically significant differences among all treatment groups (p < 0.05).
As evidenced in Table 3, the photosynthetic behavior of F. taipaiensis leaves varied when inoculated with disparate KSB or complex bacterial strains within the same habitat. The net photosynthetic rate when inoculated with KSB was higher than that observed with single strains, and the net photosynthetic rate across all treatment groups was superior to the control group, registering a value 2.35 times that of the control group. This was followed by the S4 and S7 groups, which were 2.28 times as high as the control group. The enhancement in the intercellular CO2 concentration in F. taipaiensis when inoculated with KSB revealed that the intercellular CO2 concentration in composite bacterial groups was higher than that of single strains. The concentration was greater across all treatment groups in comparison with the control group, peaking in the S7 group at 2.35 times the control group, and exhibiting a significant difference among other groups (p < 0.05). Additionally, the stomatal conductance of F. taipaiensis leaves, when inoculated with different KSB or compound bacteria, was markedly higher than that of the control group. Specifically, the stomatal conductance in the S7 group was at its zenith, 204.17% higher than the control group. Furthermore, the leaf transpiration rate of F. taipaiensis was significantly higher than that of the control group, reaching a maximum in the S7 group at 4.37 times the control group, with all treatment groups demonstrating significant differences (p < 0.05). The leaf water use efficiency (WUE) of F. taipaiensis with KSB was similar to or lower than that of the control group, which was related to the very low transpiration rate of the control group The stomatal limit (LS) and intercellular CO2 concentration (Ci) showed an opposite trend. Compared with the control group, Ci showed an upward trend, while Ls showed a downward trend, the results showed that inoculation of KSB could improve the photosynthetic performance of F. taipaiensis, but there were some differences among different treatments.
3.3 Effect of Inoculation with Different KSB on Photosynthetic Pigment Content of F. taipaiensis
In comparison to the control group, the levels of chlorophyll a, chlorophyll b, total chlorophyll, and Carotenoid in the leaves of F. taipaiensis, when inoculated with various KSB, increased substantially. Most of these increments were statistically significant (p < 0.05), highlighting that the impact of mixed inoculation with different KSBs on the photosynthetic pigments of F. taipaiensis leaves varied within the same habitat. Detailed figures are depicted in Table 8.
From the chlorophyll a/b results, it can be observed that, excluding group S2, the remaining groups exhibited values greater than the control group. Based on the single inoculation groups (S1, S2, S3), the contents of chlorophyll a, chlorophyll b, and total chlorophyll followed the order S2 > S1 > S3 and the contents of Carotenoid were S1 > S2 > S3, respectively. This variance may be attributed to the diverse effects of the three types of KSB. As for chlorophyll a, total chlorophyll, and Carotenoid, the pattern was S4>S6>S5, respectively, whereas for chlorophyll b, it was S4 > S5 > S6, respectively. Collectively, the maximal total chlorophyll content among all treatment groups was observed in the S7 group.
3.4 Effect of Different KSB on Antioxidant Enzyme System and MDA Content of F. taipaiensis
SOD, POD, and CAT represent critical protective enzymes in the active oxygen scavenging system [30], functioning to deter the rapid accumulation of high-concentration oxygen, prevent the peroxidation of membrane lipids, and aid in decelerating plant senescence. As indicated in Table 9, POD activity in all treatment groups exceeded that in the control group, with the S7 group displaying the highest activity, being 1.84 times greater than that in the control group, without significant divergence among all treatment groups (p < 0.05). These findings demonstrate that inoculation with various KSB exerted minimal influence on POD activity in F. taipaiensis leaves, but distinctly augmented POD activity within the leaves.
The SOD activity of the treatment groups inoculated with either single or compound KSB surpassed that of the control group to varying extents, and a significant discrepancy was evident between the majority of the treatment groups and the control group (p < 0.05). The activity of SOD in S4, S5, S6, and S7 groups was superior to that in S1, S2, and S3 groups. The S6 group manifested the highest activity, a 54.71% increase compared to the control group, denoting a 48.82% enhancement.
Significant differences in CAT activity were present between different KSB and control groups (p < 0.05), signifying that KSB had a pronounced influence on CAT activity in F. taipaiensis leaves. The CAT activity in the S7 group was 2.7 times that of the control group, and the activity of SOD in the S4, S5, S6, and S7 groups was higher than in the S1, S2, S3 groups, manifesting an evident increase in CAT activity.
The investigation into the effects of diverse KSB on the MDA content of F. taipaiensis leaves revealed that the MDA content in all treatment groups was inferior to that in the control group, with a significant difference between the two categories (p < 0.05). This underscores that the inoculation of KSB substantially influenced MDA content within the leaves of F. taipaiensis. The MDA contents in the leaves of S4, S5, and S6, when inoculated with a mixture of two strains, were considerably reduced compared to those of S1, S2, and S3 inoculated with a single strain. The MDA content in the leaves of F. taipaiensis attained the lowest value in the S7 group, equating to approximately 1/3 of the control group.
3.5 Effect of Inoculation with Different KSB on the Contents of Soluble Sugar, Soluble Protein, and Proline in the Leaves of F. taipaiensis
Proline (Pro), soluble sugar, and soluble protein serve as principal osmotic regulators in plant systems [31]. In Table 10, it is evident that except for the S2 and S3 groups, the soluble protein content in the leaves of F. taipaiensis was elevated relative to the control group in varying magnitudes, with the S4 and S7 groups manifesting a statistically significant increase (p < 0.05). Specifically, the content of soluble protein in the S7 group was observed to be 1.54-fold higher than that in the control group.
Excluding the S1 and S3 groups, the soluble sugar content in the leaves of F. taipaiensis in the remaining treatment groups was greater to some extent than that in the control group. This finding denotes that the combined inoculation with KSB could facilitate the synthesis of soluble sugar in the leaves of F. taipaiensis. Furthermore, the soluble sugar content in group S7 was 1.85-fold higher than in the control group, and notably higher in groups S4, S5, S6, and S7 when compared to groups S1, S2, and S3. This result underscores that the production of soluble sugar in the leaves of F. taipaiensis was conspicuously augmented by the inoculation with the combinatory bacteria.
The content of proline in the leaves of F. taipaiensis was consistently higher than that of the control group across all treatment groups. Such an observation signifies that the combined inoculation with KSB could markedly stimulate the synthesis of proline within the leaves of F. taipaiensis. Moreover, the proline content of group S7 was the most pronounced, representing an increment of 137.79% relative to the control group. This, along with the heightened proline content in groups S4, S5, S6, and S7 as opposed to groups S1, S2, and S3, reinforces the indication that the combinatory inoculation plays a significant role in promoting the synthesis of soluble sugar in the leaves of F. taipaiensis.
3.6 Effects of Inoculation with KSB on the Expression of POD, SOD, and CAT Genes in F. taipaiensis Leaves
Superoxide dismutase (SOD) plays a catalytic role in converting the superoxide radical into H2O2 and O2 [32,33]. Concurrently, peroxidase (POD) and catalase (CAT) oxidize H2O2 into H2O and O2. As delineated in Fig. 3A through Fig. 3C, the antioxidant enzymes in groups inoculated with either distinct KSB or a complex of such bacteria were markedly elevated in comparison to those in the control group. Furthermore, the enzymatic activity within the combination strains (S4, S5, S6, S7) surpassed that of the single strains (S1, S2, S3). Although no substantial differences were noted in POD activity, significant disparities were observed in both SOD and CAT activity among the majority of treatment groups (p < 0.05). Specifically, POD activity reached its zenith in group S7, nearly doubling that of the control group, while the apex of SOD activity was found in group S6, closely followed by group S7. Both, however, were appreciably higher than other treatment groups and the control group. CAT activity was predominant in the S7 group, more than twofold that of most other treatment groups. Overall, the antioxidant enzyme activities in F. taipaiensis leaves followed the trend SOD > CAT > POD, congruent with the corresponding enzyme gene expression.
Figure 3: Effects of inoculation with different KSB on activities of POD (Peroxidase) (A), SOD (Superoxide dismutase) (B), and CAT (Catalase) (C) (average ± SD CV, n = 6)
Note: Different lowercase letters indicate significant differences at 0.05 level.
To substantiate that the inoculation with disparate KSB or complex bacterial amalgamations could augment the antioxidant activity in F. taipaiensis, an investigation was conducted into the differential expression of antioxidant enzyme genes within F. taipaiensis leaves from various treatment groups and the control group, namely POD, SOD, and CAT. Quantitative PCR (Q-PCR) analysis revealed that the relative expression levels of these genes were considerably elevated compared to the control group (p < 0.05). Significantly, these results were analogous to those observed for corresponding enzyme activity (Fig. 4). The relative expression of the target gene under different treatments was calculated according to the 2−∆∆Ct method.
Figure 4: Effects of inoculation with different KSB on the relative expression of Peroxidase (POD), Superoxide dismutase (SOD), and Catalase (CAT) genes
Note: Different lowercase letters indicate significant differences at 0.05 level.
In a comparative analysis, SOD and CAT were identified as the most prevalent antioxidant genes in F. taipaiensis leaves, succeeded by POD. The distinction in POD gene expression among different treatment groups was marked, with the zenith observed in the S7 group at 5.657, and an average expression level at 5.06-fold that of the control group. The S7 group’s expression level exceeded twice that of the S1, S2, S3 group. Variability in SOD gene expression across the treatment groups was also apparent; the apogee of SOD gene expression was 6.338 in the S6 group, a figure 6.73 times higher than the control group and marginally surpassing that of the S7 group, which was inoculated with a trio of mixed bacteria. Additionally, the CAT gene expression in the S3 group was significantly superior to that in the S4 group. The acme of CAT gene expression was observed in the S7 group at 7.509, an astounding 6.86 times greater than the control group. These findings underscore the profound impact that the inoculation of mixed KSB has on CAT activity within F. taipaiensis leaves.
3.7 Correlation Analysis of Some Indexes and Antioxidant Enzyme Genes in F. taipaiensis Leaves
Utilizing the Pearson product-moment correlation coefficient (r) to ascertain associations between various indices [34,35], the relationship becomes more potent as the value of r2 approaches unity. Graphical examination reveals that all the analyzed indices bear a negative correlation with MDA content, notably manifesting with POD activity and CAT gene expression (p < 0.01) in Fig. 5. There exists a positive correlation between the content of chlorophyll a and both Carotenoid and soluble sugar concentrations (p < 0.01). Chlorophyll b, conversely, exhibits a positive yet insignificant correlation with soluble sugar, and a negative association with other indices, while Carotenoid content is positively correlated with other indices; specifically, the contents of Carotenoids positively correlate with the contents of soluble sugar and soluble protein (p < 0.01). Further, POD activity demonstrates a positive correlation with the content of soluble protein (p < 0.05) and an appreciably positive correlation with other indices (p < 0.01). SOD activity exhibits positive correlations with SOD gene expression, POD gene expression, and CAT gene expression (p < 0.01). It also maintains a positive relationship with CAT content, soluble sugar, soluble protein, and proline content (p < 0.05), while CAT activity is positively correlated with soluble protein (p < 0.05), and other indicators reflect a significant positive correlation (p < 0.01). Moreover, the soluble protein content is positively associated with all the indices under study, and reveals a positive correlation with soluble sugar content (p < 0.01), all the indices, and the expression of the POD gene (p < 0.01). Additionally, proline content manifests positive correlations with the expression of the POD, SOD, and CAT genes (p < 0.01), a result that corroborates the positive correlation found among the expressions of these genes themselves (p < 0.01).
Figure 5: Correlation analysis of some indexes and antioxidant enzyme genes in F. taipaiensis leaves
Note: The bigger the value, the redder the color, the higher the correlation; the closer the circle, the lower the correlation; the negative correlation is blue.
During the growth phase of F. taipaiensis seedlings, the acquisition of various nutrients from the leaves, in conjunction with the synthesis of carbohydrates through photosynthesis, is essential for sustaining plant growth. Scientific fertilization, consequently, has evolved as a critical technological strategy in the cultivation of F. taipaiensis. Within the scope of this paper, a novel KSB was chosen to mitigate the risk of soil acidification engendered by prolonged chemical fertilizer application. This method aims to enhance soil microbial activity, reinforce the provision of soil nutrients, ameliorate the soil ecological environment, and thereby foster the growth of F. taipaiensis.
The physiological functions of KSB exert conspicuous influences on soil attributes, plant nutrient absorption, and overall plant maturation. Empirical evidence demonstrates that microorganisms can substantially augment above-ground biomass, plant height, stem diameter, and other agronomic characteristics of plants. In research conducted by Zhang et al. [12], it was discerned that AM fungi infection could discernibly impact the metabolism of total alkaloids, including compounds such as F. tinctoria and F. sibirica glycoside. Li et al. [36] discovered that the inoculation of organophosphorus bacteria could intensify the photosynthesis of Paris polyphylla, with the leaf functioning as the principal mechanism for nutrient accumulation and the chief organ for conducting photosynthesis. The present experiment was orchestrated to explore the ramifications of various KSB treatments on the growth and antioxidant capability of F. taipaiensis leaves. Through the analysis of parameters such as leaf number, leaf dimensions, plant height, leaf thickness, stem diameter, leaf photosynthetic parameters, chlorophyll content, and the concentration of soluble sugar, soluble protein, and proline in the leaves of F. taipaiensis, along with the activities of antioxidant enzymes and their correlated gene expression, this study aims to elucidate the effects of inoculating KSB on F. taipaiensis.
The correlation between leaf size and the growth and development of a plant has been established, with larger leaves generally indicative of superior plant vitality [37]. In the study, the leaf area across all treatment groups was observed to be considerably greater than that of the control group lacking inoculation. The S4 group exhibited the most extensive leaf area. Furthermore, the leaf differentiation, plant height, stem diameter, and leaf thickness of F. taipaiensis were all markedly superior to those observed in conventional cultivation. Particularly, the S7 treatment group demonstrated the most optimal growth index, thereby signifying that inoculation with KSB could expedite the growth of F. taipaiensis leaves. Notably, the effect of combined inoculation outperformed that of single-plant inoculation. Photosynthetic parameters are pivotal in determining the magnitude of plant photosynthetic capacity [38]. Without the integration of photosynthetic indices such as Gs, Tr, etc., photosynthesis is rendered unfeasible. In the analysis, the leaves of F. taipaiensis registered the highest NET photosynthetic rate in the S6 group and attained the maximal intercellular CO2 concentration, stomatal conductance, and transpiration rate in the S7 group. Additionally, the values of Pn, Gs, Tr, and Ci in each treatment group surpassed those in the control group. Such results inferred that inoculation with either KSB or compound bacteria could markedly elevate the photosynthetic rate of F. taipaiensis leaves. Photosynthetic pigments also play a vital role in photosynthesis [39]. In the study, the S7-treated group presented the highest content of chlorophyll a, total chlorophyll, and Carotenoid, while the S2-treated group demonstrated the highest content of chlorophyll b. The levels of chlorophyll a, chlorophyll b, Carotenoid, and total chlorophyll in the leaves of F. taipaiensis were augmented to varying extents across all treatment groups. Such observations indicate that inoculation with KSB could potentiate photosynthesis in the leaves of F. taipaiensis, thereby establishing a foundational platform for the growth and material accumulation of F. taipaiensis.
Malondialdehyde (MDA) serves as a product of cytoplasmic peroxidation [40,41]. A lower content of this compound typically indicates more favorable plant growth. The study revealed that the MDA content in the leaves of F. taipaiensis declined substantially following inoculation with KSB, with the most prominent effect being observed in group S7. Both soluble sugar and soluble protein function as osmotic regulators, influencing the structure and function of the plant cell membrane. Proline, as well, holds a crucial role in osmotic regulation within the plant cytoplasm [30]. Compared to the control group, the content of soluble sugar, soluble protein, and proline in the majority of treatment groups inoculated with KSB was elevated. The S7 group demonstrated the highest content of these three indices.
Superoxide dismutase (SOD), peroxidase (POD), and catalase (CAT) are the principal antioxidant enzymes in plants, and their activity serves as a significant index for assessing plant stress resistance [30–32]. This research discerned that the activities of SOD, POD, and CAT in most of the leaves of F. taipaiensis were markedly augmented post-inoculation with KSB, and this pattern was concomitant with the gene expression of these enzymes. Generally, the amalgamated inoculation of the three KSB (S7) exhibited the most favorable impact on the growth of F. taipaiensis.
The study indicated significant responses in the effects of KSB on growth, antioxidant activity, and expression of related genes in F. taipaiensis. The implementation of a combined application of Bacillus cereus, Burkholderia cepacia, and Bacillus subtilis could be considered for the large-scale artificial cultivation of F. taipaiensis. Such a strategy would not only augment the production benefits of F. taipaiensis but also protect the environment and the photosynthetic potential of F. taipaiensis. This study provided novel bacteria strains for the development of microbial fertilizer and the theoretical support for improving the yield of cultivated F. taipaiensis.
Acknowledgement: Not applicable.
Funding Statement: This work was funded by a Key Project of the Natural Science Foundation of Chongqing Education Committee (KJZD-K202101201).
Author Contributions: Conceptualization, J.Q.L.; methodology, J.Q.L. and M.Y.Y.; investigation, Z.F.S., X.T.K. and Y.H.W.; writing-original draft preparation, J.Q.L., Y.L. and X.L.; writing-review and editing, N.Z.; supervision, N.Z. All authors have read and agreed to the published version of the manuscript.
Availability of Data and Materials: All the data supporting the findings of this study are included in this article.
Ethics Approval: Not applicable.
Conflicts of Interest: The authors declare that they have no conflicts of interest to report regarding the present study.
References
1. China Pharmacopoeia Commission. Pharmacopoeia of the People’s Republic of China. Chi Pharm. 2020;1:36. [Google Scholar]
2. Kizil S, Arslan N, lmez-Bayhan S, Khawar KM. Effects of different planting dates on improving yield of Fritillaria imperialis L. and Fritillaria persica L. bulbs damaged by small narcissus fly (Eumerus strigatus Fallen). Afr J Biotechnol. 2008;7(24):4454–8. [Google Scholar]
3. Park I, Kim WJ, Yeo SM, Choi G, Kang YM, Piao R, et al. The complete chloroplast genome sequences of Fritillaria ussuriensis Maxim. and Fritillaria cirrhosa D. Don, and comparative analysis with other Fritillaria species. Mol. 2017;22(6):982. [Google Scholar]
4. Konchar K, Li XL, Yang YP, Emshwiller E. Phytochemical variation in Fritillaria cirrhosa D. Don (Chuan Bei Mu) in relation to plant reproductive stage and timing of harvest. Econ Bot. 2011;65:283–94. [Google Scholar]
5. Kumar P, Acharya V, Warghat AR. Comparative transcriptome analysis infers bulb derived in vitro cultures as a promising source for sipeimine biosynthesis in Fritillaria cirrhosa D. Don (Liliaceae, syn. Fritillaria roylei Hook.)-High value Himalayan medicinal herb. Phytochem. 2021;183:112631. [Google Scholar]
6. Bora PS, Agrawal P, Kaushik NK, Puri S, Sahal D, Sharma U. Antiplasmodial activity of the bulbs of Fritillaria cirrhosa D. Don (Syn: Fritillaria roylei Hook.UPLC-IM-Q-TOF-MS/MS-based biochemometric approach for the identification of marker compounds. J Ethnopharmacol. 2023;310:116389. [Google Scholar] [PubMed]
7. Putta S, Sastry Yarla N, Kumar Kilari E, Surekha C, Aliev G, Basavaraju Divakara M, et al. Therapeutic potentials of triterpenes in diabetes and its associated complications. Curr Top Med Chem. 2016;16(23):2532–42. [Google Scholar] [PubMed]
8. Chianu JN, Chianu JN, Mairura F. Mineral fertilizers in the farming systems of Sub-Saharan Africa. A review. Agron Sustain Dev. 2012;32:545–66. [Google Scholar]
9. Sanyal R, Samant A, Prashar V, Misra H, Saini A. Biochemical and functional characterization of OsCSD3, a novel CuZn superoxide dismutase from rice. Chem Bioeng. 2018;475:3105–21. [Google Scholar]
10. Fischer J, Stott J, Zerger A, Warren G, Sherren K, Forrester RI. Reversing a tree regeneration crisis in an endangered ecoregion. Proc National Acad Sci. 2009;106(25):10386–91. [Google Scholar]
11. Wang HJ, Huang B, Shi XZ, Darilek JL, Yu DS, Sun WX, et al. Major nutrient balances in small-scale vegetable farming systems in peri-urban areas in China. Nutr Cycl Agroecosys. 2008;81:203–18. [Google Scholar]
12. Zhang J, Feng B, Wu C. Effects of arbuscular mycorrhizal fungi on growth and Q-Markers of Fritillaria taipaiensis. Chin J Inf Tradit Chin Med. 2020;27:88–93. [Google Scholar]
13. Dong T. Effects of combined application of nitrogen and phosphorus fertilizer and microbial fertilizer on growth and quality of 2-year-old Fritillaria taipaiensis (Doctoral Thesis). Northwest A&F University; China; 2018. [Google Scholar]
14. Zhang Y, Zhao S, Zou Y, Wu Q. Effects of arbuscular mycorrhizal fungi on growth and root configuration of root-restricted culture of Poncirus trifoliate L. Hubei Agric Sci. 2014;53:2588–90. [Google Scholar]
15. Liu RC, Xiao ZY, Hashem A, Abd_Allah EF, Xu YJ, Wu QS. Unraveling the interaction between arbuscular mycorrhizal fungi and Camellia plants. Hortic. 2021;7(9):322. [Google Scholar]
16. Félix CR, Andrade DA, Almeida JH, Navarro HMC, Fell JW, Landell MF. Vishniacozyma alagoana sp. nov. a tremellomycetes yeast associated with plants from dry and rainfall tropical forests. Int J Syst Evol Microbiol. 2020;70(5):3449–54. [Google Scholar]
17. Wang X, Huang J, Li H. Principles and techniques of plant physiological and biochemical experiments. Plant Physiol J. 2013;49:510–4. [Google Scholar]
18. Li AH, Yuan FX, Groenewald M, Bensch K, Yurkov AM, Li K, et al. Diversity and phylogeny of basidiomycetous yeasts from plant leaves and soil: proposal of two new orders, three new families, eight new genera and one hundred and seven new species. Stud Mycol. 2020;96(1):17–140. [Google Scholar] [PubMed]
19. Singh H, Niharika, Lamichhane P, Gupta R, Kaushik N, Choi EH, Kaushik NK. Enhancing crop health and sustainability: exploring the potential of secondary metabolites and non-thermal plasma treatment as alternatives to pesticides. Plant Biotechnol Rep. 2023;17(6):803–20. doi:10.1007/s11816-023-00883-0. [Google Scholar] [CrossRef]
20. Khan V, Umar S, Iqbal N. Synergistic action of Pseudomonas fluorescens with melatonin attenuates salt toxicity in mustard by regulating antioxidant system and flavonoid profile. Physiol Plant. 2023;175(6):e14092. [Google Scholar] [PubMed]
21. Shu Z, Zhang X, Chen J, Chen G, Xu D. Simplification of chlorophyll content determination. Plant Physiol Commun. 2010;46:399–402. [Google Scholar]
22. Wang W, Han Q, Zong Y, Jia Z, Ding R. Difference of photosynthetic efficiency in different leaf type Medicago sativa and different rotation. Pratac Sci. 2010;27:50. [Google Scholar]
23. Sanchez-Moreno M, Leon P, Salas-Peregrin JM, Osuna A. Superoxide dismutase in trematodes. Arzneimittelforschung. 1988;37:903–5. [Google Scholar]
24. Drotar A, Phelps P, Fall R. Evidence for glutathione peroxidase activities in cultured plant cells. Plant Sci. 1985;42(1):35–40. [Google Scholar]
25. Xu Y, Fan H, Zhao D, Su W, Feng Y. Optimization of coomassie brilliant blue method for the determination of soybean water-soluble protein. Soybean Sci. 2022;41:196–202. [Google Scholar]
26. Raventós J. Method for the estimation of barbituric and thiobarbituric acids in biological materials. British J Pharm Chem. 1946;1(3):210–4. [Google Scholar]
27. Livak KJ, Schmittgen TD. Analysis of relative gene expression data using real-time quantitative PCR and the 2ΔΔCT method. Meth. 2001;25(4):402–8. [Google Scholar]
28. Pfaffl MW. A new mathematical model for relative quantification in real-time RT-PCR. Nucleic Acids Res. 2001;29(9):e45. [Google Scholar] [PubMed]
29. Kumar V, Sharma S, Kumar P. Insight into the genetics and genomics studies of the fritillaria species. In: Genetics and genomics of high-altitude crops. Singapore: Springer Nature Singapore; 2024. p. 89–113. [Google Scholar]
30. Alici EH, Arabaci G. Determination of SOD, POD, PPO and cat enzyme activities in Rumex obtusifolius L. Annual Research Review Bio. 2016;11(3):1–7. [Google Scholar]
31. Irigoyen JJ, Einerich DW, Sánchez-Díaz M. Water stress induced changes in concentrations of proline and total soluble sugars in nodulated alfalfa (Medicago sativa) plants. Physiol Plant. 1992;84(1):55–60. [Google Scholar]
32. Bafana A, Dutt S, Kumar A, Kumar S, Ahuja PS. The basic and applied aspects of superoxide dismutase. J Mol Catal B: Enzym. 2011;68(2):129–38. [Google Scholar]
33. Kaur H, Sirhindi G, Bhardwaj R, Alyemeni M, Siddique K, Ahmad P. 28-homobrassinolide regulates antioxidant enzyme activities and gene expression in response to salt- and temperature-induced oxidative stress in Brassica juncea. Sci Rep. 2018;8:8735. [Google Scholar] [PubMed]
34. Mohamad Sobri N, Midi PDH, Ibrahim NB, Ismail NA. Differences between pearson’s product moment correlation coefficient and an absolute value correlation coefficient in the presence of outliers. J Math Comput Sci (JMCS). 2016;1(1):1–11. [Google Scholar]
35. Favaretto V, Martinez C, Soriani H, Furriel R. Differential responses of antioxidant anzymes in pioneer and late successional tropical tree species grown under sun and shade conditions. Environ Exp Bot. 2011;70:20. [Google Scholar]
36. Li Z, Luo L, Lang J, Ye M, Yin F. Effects of inoculating organophosphorus bacteria on photosynthesis, physiology and biochemistry of Paris polyphylla var. yunnanensis. Chin J Exp Tradit Med Formulae. 2022;28:165–71. [Google Scholar]
37. Withington JM, Reich PB, Oleksyn J, Eissenstat DM. Comparisons of structure and life span in roots and leaves among temperate trees. Ecol Monogr. 2006;76(3):381–97. [Google Scholar]
38. Sakshaug E, Bricaud A, Dandonneau Y, Falkowski PG, Kiefer DA, Legendre L, et al. Parameters of photosynthesis: definitions, theory and interpretation of results. J Plankton Res. 1997;19(11):1637–70. [Google Scholar]
39. Sartory D, Grobbelaar J. Extraction of chlorophyll a from freshwater phytoplankton for spectrophotometric analysis. Hydrobiologia. 1984;114:177–87. [Google Scholar]
40. Draper HH, Dhanakoti SN, Hadley M, Piche LA. Malondialdehyde in biological systems. In: Cellular antioxidant defense mechanisms. CRC Press; 2019. p. 97–109. [Google Scholar]
41. Rocha S, Gomes D, Lima M, Bronze-da-Rocha E, Santos-Silva A. Peroxiredoxin 2, glutathione peroxidase, and catalase in the cytosol and membrane of erythrocytes under H2O2-induced oxidative stress. Free Radical Research. 2015;49(8):990–1003. [Google Scholar] [PubMed]
Cite This Article
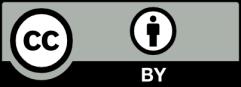
This work is licensed under a Creative Commons Attribution 4.0 International License , which permits unrestricted use, distribution, and reproduction in any medium, provided the original work is properly cited.