Open Access
ARTICLE
Changes in Leaf Stomatal Properties in Rice with the Growing Season
1 Rice and Product Ecophysiology, Key Laboratory of Ministry of Education for Crop Physiology and Molecular Biology, Hunan Agricultural University, Changsha, 410128, China
2 National Engineering Research Center of Rice, Hunan Agricultural University, Changsha, 410128, China
* Corresponding Author: Min Huang. Email:
Phyton-International Journal of Experimental Botany 2024, 93(4), 807-817. https://doi.org/10.32604/phyton.2024.048299
Received 04 December 2023; Accepted 20 March 2024; Issue published 29 April 2024
Abstract
Transplanting rice varieties grown in different seasons can lead to different yields due to different dry matter production. Early-season rice varieties transplanted in the late season can obtain high yields with short-growth duration and higher yields driven by higher dry matter production. To make clear the variations in dry matter production across seasons, four early-season rice varieties were chosen for late-season transplantation. The grain yield, dry matter accumulation, leaf photosynthetic, and leaf stomatal properties were studied. It was observed that the average yields of these four varieties in the late season were 33% greater, despite a reduced growth period of 13 days in comparison with the early season. Furthermore, there was a notable increase in both total and post-heading dry matter production during the late season. The leaf net photosynthetic rate, stomatal area, stomatal width, and stomatal length were higher in the late season. Despite no significant difference in stomatal density between seasons, strong positive linear relationships were observed between net photosynthetic rate and stomatal conductance, and between stomatal conductance and area. These relationships demonstrate that the increase of the stomatal width and length of the leaves in the late season leads to an increase in the stomatal area, thereby increasing the stomatal conductance and enhancing the photosynthesis of the leaves. Consequently, this leads to greater dry matter production and a higher yield compared to the early season. Therefore, when breeding new high-yielding and short-growing varieties, the large stomatal area can be used as a reference index.Keywords
The reduction of arable land requires increasing the yield per unit of rice crops, which has become an effective way to meet demand. Rice yield development is closely associated with dry matter production and the harvest index. At present, the rice harvest index is close to the theoretical upper limit. Enhancing yield to meet or potentially exceed this theoretical limit involves augmenting leaf light energy conversion efficiency to boost plant biomass accumulation. Leaf light energy conversion efficiency largely hinges on the photosynthetic capacity of leaves and more venation to transport the manufactured sugars from source to sink [1]. Enhancing leaf photosynthesis rate was one important aspect vital for future high-yield and efficient breeding and cultivation.
Numerous studies indicate a significant positive correlation between net photosynthetic rate and stomatal conductance [2–3]. The air-cell interface, where stomata facilitate CO2 uptake, is a major bottleneck for net photosynthesis [4–7]. Increases in guard cell turgor pressure lead to a greater stomatal dimension (such as size), which enhances the stomatal conductance [8]. Meanwhile, stomatal conductance is also affected by stomatal frequency (such as density) [9].
Transplanting early-season rice varieties in the late season has been shown to achieve high yields over a shorter growth duration. The development of short-growth duration rice has augmented the cropping intensity and food supply for the country, particularly in major rice-producing nations like China [10]. Enhancing cropping intensity is a key strategy for future food security [11]. Research suggests that short-growth duration rice yield was closely positively related to dry matter production [12–15]. Understanding the variations in dry matter production between early and late-season plantings of early-season varieties is crucial. Determining leaf stomatal properties in these short-growth duration rice populations is pivotal for selecting the new, high-yielding, short-growth duration varieties. Stomatal can work as a quick proxy to determine leaf performance and overall plant productivity.
2.1 Plant Materials and Experimental Design
In 2021, field trials were carried out in Yongan Town, Hunan Province, China (28°09′ N, 113°37′ E, 43 m asl), during both the early and late seasons of rice cultivation. Four early-season rice varieties, Jiyou 421 (JY421), Lingliangyou 179 (LLY179), Lingliangyou 268 (LLY268), and Zhuliangyou 4024 (ZLY4024), were used as materials in the early season and late season. The selection of these varieties was influenced by their extensive use among local rice farmers and their shorter growth period of under 115 days in the early season.
Soil properties of the experimental field were characterized as follows: pH of 5.88, 22.8 g kg−1 of organic matter, 112 mg kg−1 of available nitrogen, 23.2 mg kg−1 of available phosphorus, and 80 mg kg−1 of available potassium. These soil characteristics were determined from samples collected from the top 20 cm layer of soil before the field experiment started.
The arrangement of rice varieties followed a randomized block design, incorporating three replications, each occupying an area of 30 m2. Plots were consistent with varieties across both seasons.
On 25 March for the early season and 30 June for the late season, 80 grams of pre-germinated rice seeds were sown in each seedling tray. Seedlings were transplanted on 25 April during the early season and 20 July in the late season. The transplanting process was carried out with a spacing of 25 cm by 12 cm, placing 4 to 5 seedlings in each hill, utilizing a high-speed rice transplanter (PZ80-25; Dongfeng Iseki Agricultural Machinery Co., Ltd., Xiangyang, China). In both seasons, each plot was treated with 150 kg of nitrogen, 75 kg of phosphorus pentoxide, and 150 kg of potassium oxide per hectare. Nitrogen fertilizer application was divided into three stages: 50% as base fertilizer one day before transplanting, 30% during the early tillering stage seven days post-transplantation, and the remaining 20% at the start of panicle formation. Water management was consistent with local high-yield cultivation. Chemical controls were used to inhibit weed, insect, and pathogen populations.
2.2 Plant Sampling and Measurements
At the heading stage, three representative flag leaves per plot were chosen randomly between 09:00 and 11:00 AM to assess the photosynthetic rate, stomatal conductance, intercellular CO2 concentration, and transpiration rate, utilizing a portable, non-destructive photosynthesis analyzer (LI-6400XT; Li-Cor, Lincoln, NE, USA). Measurements were performed in regulated environmental settings, including a light intensity of 1200 μmol m–2 s–1, a reference CO2 concentration of 390 μmol mol–1, air temperature set at 32.5°C, and 65% relative humidity. After Pn measurement, the stomatal density and area were obtained by scanning electron microscope (HITACHI, SU8100). Scanning electron microscope sample preparation method:
(1) Take the middle part (1 mm × 1 mm) of fresh leaves and gently rinse the surface of the sample with phosphate buffer solution (PBS).
(2) Submerge directly into the fixative solution for electron microscopy and keep for 2 h at ambient temperature.
(3) Store the samples at 4°C following fixation.
(4) Wash the fixed samples three times with 0.1 M phosphate buffer (pH 7.4), 15 min for each rinse (0.1 M phosphate buffer PB (pH 7.4) prepared with 1% osmic acid was fixed at room temperature for 1–2 h in the dark).
(5) Progressively immerse the samples in a series of alcohol solutions with increasing concentrations from 30% up to 100%, each for a duration of 15 min, followed by a 15-min immersion in isoamyl acetate.
(6) Dry the sample in a critical point dryer.
(7) Affix the sample onto conductive carbon film double-sided tape and place it in the ion sputtering instrument for a 30-s gold coating.
(8) The images are subsequently observed under a scanning electron microscope to count the number of stomatal units in the same unit area, assessed with a 200 um ruler as the standard with Image-Pro Plus 6.0, and the five-point method was used for each graph.
(9) Measure the stomatal length, width, and area using Image-Pro Plus 6.0.
2.2.1 Leaf Area Index (LAI) and Pre-Heading Dry Matter Production
During the heading stage, ten representative rice plant hills were taken from each plot. Green leaves were then detached, and their area was quantified using a portable leaf area meter (Li-3100C). Following this, the plant’s aboveground biomass was dried at 105°C for 30 min and later desiccated at 70°C until a consistent weight was attained for dry matter evaluation.
2.2.2 Yield, Yield Components, and Total Dry Matter Production
At maturity, the grain yield and biomass were assessed from a central area of 0.36 m2 in each plot. Plant samples were hand-separated into straw (comprising rachises) and spikelets. To distinguish between filled and unfilled spikelets, they were submerged in tap water. Three 30 g samples of filled grains along with all unfilled spikelets were enumerated by hand. The straw and both filled and unfilled spikelets were weighed post-oven-drying at 70°C until reaching a stable weight. The harvest index was computed using the formula: weight of filled spikelets divided by the total weight, which includes straw, filled, and unfilled spikelets. Grain yield was then standardized to a moisture content of 13.5% of H2O. The daily rice grain yield was calculated by dividing the total grain yield by the growth period from the sowing date to maturity.
All data were analyzed using analysis of variance (Statistics 8.0, Analytical Software, Tallahassee, FL, USA). Means of seasons were compared by the least significant difference (LSD) test at the 0.05 probability level. An ANOVA model included the main effects of season, cultivar, and their two-way interaction effects.
The average temperatures of the early season were 25.58°C and late seasons 28.28°C, respectively (Fig. 1). The average temperatures of pre-heading during the early and late seasons were 23.79°C and 28.92°C, respectively (Fig. 1). The average temperatures of post-heading during the early and late seasons were 29.23°C and 27.26°C, respectively (Fig. 1).
Figure 1: Daily mean temperature during the early and late seasons. Thick and thin lines represent the average daily mean temperature across four varieties in the early- and late rice-growing seasons, respectively
The season had significant effects on nearly all tested parameters, except for the pre-heading dry matter production, stomatal density, and stomatal length (Table 1). Variety only had significant effects on Pn and Ci. The two-way interactive effects of season and variety were not significant or consistent when comparing all parameters. In light of these interactions, only means of the early season and late season were presented and compared in the following tables (Tables 2–5), in line with the objective of this study.
3.3 Yield, Daily Grain Yield, and Growth Duration
Early-season varieties planted during the late season exhibited an average yield that was 33% higher than those planted in the early season. Additionally, the mean daily grain yield of the late season was 50% higher compared to the early season (Table 2). The mean whole growth period in the late season was 13 days shorter than in the early season. The diversities in the whole growth period between the two seasons were primarily due to the pre-heading period.
3.4 Dry Matter Production, Harvest Index, and LAI
The mean total dry matter accumulation was significantly different between seasons, and the late season was 13% higher. Except for LLY268, the difference in dry matter accumulation between the two seasons mainly came from the post-heading (Table 3). The result showed that the mean harvest index in the late season was significantly higher than in the early season. Accordingly, the mean LAI was 19% lower in the early season.
Late-season-planted varieties outperformed early-season individuals. The mean Pn, gs, and Ci of the late season were 15%, 16%, and 5% higher than early season, respectively (Table 4). A robust positive linear correlation was observed between Pn and gs, as illustrated in Fig. 2.
Figure 2: Relationships between net photosynthetic rate (Pn) and stomatal conductance (gs) of four early-season rice cultivars in the early and late seasons. **means p < 0.01. R2 is a goodness-of-fit measure for the linear regression model
3.6 Stomatal Density, Length, Width, and Area
Changes in leaf stomatal characteristics were observed when early-season rice varieties were cultivated in different seasons, as indicated in Table 5 and Fig. 3. The average stomatal width of the four varieties exhibited an increase during the late season compared to the early seasons. As a result, there were significant differences in stomatal area between seasons, with the late season displaying a 23% increase in area compared to the early season. A robust positive linear correlation was observed between gs and stomatal area, as depicted in Fig. 4. In general, stomatal density in various varieties during the late season showed lower performance compared to the early season, except for LLY268, which exhibited an opposite trend.
Figure 3: Leaf stomatal scan picture of four early-season rice varieties grown in different seasons. The position pointed by the arrow is the stomata
Figure 4: Relationships of stomatal area and stomatal conductance (gs), stomatal area and yield of four early-season rice cultivars in the early and late seasons. **means p < 0.01. R2 is a goodness-of-fit measure for the linear regression model
Previous research has indicated that transplanting early-season rice varieties into the late season results in a shortened growth duration while maintaining stable yields [12,13,15]. Our study aligns with these earlier findings. In our study, the four early-season varieties transplanted late had an average of 33% higher yield than those transplanted early. This enhanced grain yield was primarily attributed to total dry matter production and harvest index. Discrepancies in dry matter production and harvest index between the early and late seasons of early rice varieties primarily originate from the post-heading stage, as indicated in Table 3. Previous studies have underscored the significance of post-heading dry matter production in influencing yield [16,17]. It is noteworthy that the post-heading mean temperature during the early season was 1.97°C lower than that in the late season, as illustrated in Fig. 1. This observation indicates that the elevated post-heading dry matter production in early-season rice varieties transplanted late was attributable not only to temperature alone but also to the inherent photosynthetic characteristics and/or LAI of the rice leaves.
Dry matter is a direct product of photosynthesis. In our study, the Pn of early-season rice varieties during the late season significantly exceeded that of the early season. This increased dry matter production in late-season individuals was attributed to this specific characteristic. Pn and gs exhibited a strong linear correlation (Fig. 2), while gs was mainly affected by stomatal area [18]. Stomatal properties were determined genetically [2,19,20] but also be affected by the external environment, such as CO2 concentration [21], temperature [22], and moisture [23,24]. Additionally, the external environment perceived by mature leaves can trigger systemic responses that influence stomatal development on the epidermis of new leaves, leading to alterations in stomatal properties [25–28]. Transplanting early-season rice varieties into the late season resulted in a notable increase of 5.13°C in the average temperature before heading. This temperature increase may elucidate the observed rise in the stomatal area of flag leaves during the late season, particularly driven by the enlargement of stomatal width and length. The overall stomatal area of the leaf is influenced by both the individual stomatal size and the stomatal density of the leaf. Despite the lower average stomatal density observed in the late season comparison with the early season in this study, the individual stomatal size during the late season was larger, resulting in a greater overall stomatal area in the late season than early season. The increase in the stomatal area improves stomatal conductance, which thereby increases photosynthesis produces more dry matter, and finally improves the yield (Fig. 4).
Early-season rice varieties demonstrated increased dry matter production and higher yields during the late season, primarily attributed to leaf net photosynthetic rates. Leaf net photosynthetic was significantly affected by stomatal conductance. The stomatal area determines the stomatal conductance. The increased temperature during pre-heading duration increased the length of the stomata, thereby increasing the stomatal area. The results of this paper can provide a reference for breeders to select new high-yield and short-growth varieties and high-yield cultivation of short-growth varieties.
Acknowledgement: Not applicable.
Funding Statement: This work was supported by the Science and Technology Innovation Program of Hunan Province (Grant No. 2021RC3088), the Hunan Provincial Natural Science Foundation of China (Grant No. 2023JJ40309), the National Natural Science Foundation of China (Grant No. 32001470).
Author Contributions: The authors confirm their contribution to the paper as follows: study conception and design: Min Huang; data collection: Jiana Chen, Fangbo Cao; analysis and interpretation of results: Jiana Chen; draft manuscript preparation: Jiana Chen, Min Huang. All authors reviewed the results and approved the final version of the manuscript.
Availability of Data and Materials: All data generated or analyzed during this study are included in this published article.
Ethics Approval: Not applicable.
Conflicts of Interest: The authors declare that they have no conflicts of interest to report regarding the present study.
References
1. Murchie EH, Pinto M, Horton P. Agriculture and the new challenges for photosynthesis research. New Phytol. 2009;181:532–52. [Google Scholar] [PubMed]
2. Giuliani R, Koteyeva N, Voznesenskaya E, Evans MA, Cousins AB, Edwards GE. Coordination of leaf photosynthesis, transpiration, and structural traits in rice and wild relatives (genus Oryza). Plant Physiol. 2013;162:1632–51. [Google Scholar] [PubMed]
3. Liu X, Li Y. Varietal difference in the correlation between leaf nitrogen content and photosynthesis in rice (Oryza sativa L.) plants is related to specific leaf weight. J Agric Sci. 2016;15:2002–11. [Google Scholar]
4. Adachi S, Nakae T, Uchida M, Soda K, Takai T, Oi T, et al. The mesophyll anatomy enhancing CO2 diffusion is a key trait for improving rice photosynthesis. J Exp Bot. 2013;64:1061–72. [Google Scholar] [PubMed]
5. Evans JR, Kaldenhoff R, Genty B, Terashima I. Resistances along the CO2 diffusion pathway inside leaves. J Exp Bot. 2009;60:2235–48. [Google Scholar] [PubMed]
6. Flexas J, Barbour MM, Brendel O, Cabrera HM, Carriquí M, Díaz-Espejo A, et al. Mesophyll diffusion conductance to CO2: an unappreciated central player in photosynthesis. Plant Sci. 2012;193:70–84. [Google Scholar] [PubMed]
7. Yamori W, Nagai T, Makino A. The rate-limiting step for CO2 assimilation at different temperatures is influenced by the leaf nitrogen content in several C3 crop species. Plant Cell Environ. 2011;34:764–77. [Google Scholar] [PubMed]
8. Bertolino LT, Caine RS, Gray JE. Impact of stomatal density and morphology on water-use efficiency in a changing world. Front Plant Sci. 2019;10:225. [Google Scholar] [PubMed]
9. Konrad W, Roth-Nebelsick A, Grein M. Modelling of stomatal density response to atmospheric CO2. J Theor Biol. 2008;25:638–58. [Google Scholar]
10. Khush GS. Green revolution: the way forward. Nat Rev Genet. 2001;2:815–22. [Google Scholar] [PubMed]
11. Ray DK, Foley JA. Increasing global crop harvest frequency: recent trends and future directions. Environ Res Lett. 2013;8:4041. doi:10.1088/1748-9326/8/4/044041. [Google Scholar] [CrossRef]
12. Chen J, Cao F, Yin X, Huang M, Zou Y. Yield performance of early-season rice cultivars grown in the late season of double-season crop production under machine-transplanted conditions. PLoS One. 2019;14:e0213075. doi:10.1371/journal.pone.0213075. [Google Scholar] [PubMed] [CrossRef]
13. Chen J, Huang M, Cao F, Yin X, Zou Y. Availability of existing early-season rice cultivars as resources for selecting high-yielding short-duration cultivars of machine-transplanted late-season rice. Exp Agric. 2020;56:218–26. doi:10.1017/S0014479719000310. [Google Scholar] [CrossRef]
14. Huang M, Lei T, Cao F, Chen J, Zou Y. Solar radiation utilization characteristics of double-season rice in China. Agron J.. 2021;113:270–5. doi:10.1002/agj2.20511. [Google Scholar] [CrossRef]
15. Xu L, Zhan X, Yu T, Nie L, Huang J, Cui K, et al. Yield performance of direct-seeded, double-season rice using varieties with short growth durations in central China. Field Crops Res. 2018;227:49–55. doi:10.1016/j.fcr.2018.08.002. [Google Scholar] [CrossRef]
16. Pal R, Mahajan Sardana V, Chauhan BS. Impact of sowing date on yield, dry matter and nitrogen accumulation, and nitrogen translocation in dry-seeded rice in North-West India. Field Crop Res. 2017;206:138–48. [Google Scholar]
17. Huang L, Yang D, Li X, Peng S, Wang F. Coordination of high grain yield and high nitrogen use efficiency through large sink size and high post-heading source capacity in rice. Field Crop Res. 2019;233:49–58. [Google Scholar]
18. Fanourakis D, Giday H, Milla R, Pieruschka R, Kjaer KH, Bolger M, et al. Pore size regulates operating stomatal conductance, while stomatal densities drive the partitioning of conductance between leaf sides. Ann Bot. 2015;115:555–65. [Google Scholar] [PubMed]
19. Doheny-Adams T, Hunt L, Franks PJ, Beerling DJ, Gray JE. Genetic manipulation of stomatal density influences stomatal size, plant growth and tolerance to restricted water supply across a growth carbon dioxide gradient. Philo Trans R Soc B: Biol Sci. 2012;367:547–55. [Google Scholar]
20. Ohsumi A, Kanemura T, Homma K, Horie T, Shiraiwa T. Genotypic variation of stomatal conductance in relation to stomatal density and length in rice (Oryza sativa L.). Plant Prod Sci. 2007;10:322–8. [Google Scholar]
21. Uprety DC, Dwivedi N, Jain V, Mohan R. Effect of elevated carbon dioxide concentration on the stomatal parameters of rice cultivars. Photosynthetica. 2002;40:315–9. doi:10.1023/A:1021322513770. [Google Scholar] [CrossRef]
22. Huang G, Yang Y, Zhu L, Peng S, Li Y. Temperature responses of photosynthesis and stomatal conductance in rice and wheat plants. Agric For Meteorol. 2021;300:108322. doi:10.1016/j.agrformet.2021.108322. [Google Scholar] [CrossRef]
23. Caine RS, Yin X, Sloan J, Harrison EL, Mohammed U, Fulton T, et al. Rice with reduced stomatal density conserves water and has improved drought tolerance under future climate conditions. New Phytol. 2019;221:371–84. doi:10.1111/nph.15344. [Google Scholar] [PubMed] [CrossRef]
24. Kumar S, Tripathi S, Singh SP, Prasad A, Akter F, Syed MA, et al. Rice breeding for yield under drought has selected for longer flag leaves and lower stomatal density. J Exp Bot. 2021;72:4981–92. doi:10.1093/jxb/erab160. [Google Scholar] [PubMed] [CrossRef]
25. Casson S, Gray JE. Influence of environmental factors on stomatal development. New Phy. 2008;178:9–23. doi:10.1111/j.1469-8137.2007.02351.x. [Google Scholar] [PubMed] [CrossRef]
26. Casson SA, Hetherington AM. Environmental regulation of stomatal development. Curr Opin Plant Biol. 2010;13:90–5. doi:10.1016/j.pbi.2009.08.005. [Google Scholar] [PubMed] [CrossRef]
27. Pillitteri LJ, Torii KU. Mechanisms of stomatal development. Annu Rev Plant Bio. 2012;63:591–614. doi:10.1146/annurev-arplant-042811-105451. [Google Scholar] [PubMed] [CrossRef]
28. Qi X, Torii KU. Hormonal and environmental signals guiding stomatal development. BMC Bio. 2018;16:1–11. doi:10.1186/s12915-018-0488-5. [Google Scholar] [PubMed] [CrossRef]
Cite This Article
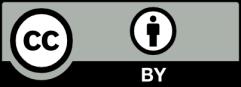
This work is licensed under a Creative Commons Attribution 4.0 International License , which permits unrestricted use, distribution, and reproduction in any medium, provided the original work is properly cited.