Open Access
ARTICLE
Chitosan Nanoparticles as Biostimulant in Lettuce (Lactuca sativa L.) Plants
1 Tecnológico Nacional de México, Instituto Tecnológico de Torreón, Ejido Ana, Torreón, Coahuila, 27170, México
2 Departamento de Horticultura, Universidad Autónoma Agraria Antonio Narro, Saltillo, 25315, México
3 Departamento de Materiales Avanzados, Centro de Investigación en Química Aplicada, Saltillo, Coahuila, 25294, México
* Corresponding Author: Hortensia Ortega-Ortiz. Email:
Phyton-International Journal of Experimental Botany 2024, 93(4), 777-787. https://doi.org/10.32604/phyton.2024.048096
Received 27 November 2023; Accepted 27 March 2024; Issue published 29 April 2024
Abstract
Biodegradable nanoparticles such as chitosan nanoparticles (CSNPs) are used in sustainable agriculture since they avoid damage to the environment; CSNPs have positive effects such as the accumulation of bioactive compounds and increased productivity in plants. This study aimed to investigate the impact of applying CSNPs on lettuce, specifically focusing on enzymatic activity, bioactive compounds, and yield. The trial was conducted using a completely randomized design, incorporating CSNPs: 0, 0.05, 0.1, 0.2, 0.4, and 0.8 mg mL. The doses of 0.4 mg mL improve yields up to 24.6% increases and 0.1 mg mL of CSNPs increases total phenols by 31.2% and antioxidant capacity by 34.6%. In addition, when low concentrations of CSNPs (0.05 and 0.1 mg L) were applied, an increase in catalase was determined. The CSNPs represent a good alternative to be used as a biostimulant in sustainable agriculture because they improve the yield and quality of lettuce by increasing the bioactive compounds.Keywords
Nomenclature
CSNPs | Chitosan nanoparticles |
Modern agriculture requires increased production, through the development of innovative technologies that improve agricultural yields, reduce inputs, and avoid environmental pollution [1]. In theagriculture sector, chitosan (CS) is a well-known bio-stimulant used to the promotion of growth and manage stresses including pest and diseases [2]. CS has multiple advantages such as non-toxicity, biocompatibility, and biodegradability [3–6]. In recent years, studies on agricultural applications of CS have focused on tolerance to abiotic stress and quality traits related to primary and secondary metabolisms [7].
The CS structured on a nanometer scale (Chitosan Nanoparticles, CSNPs) have the properties of both CS and nanoparticles (NPs). NPs have improved bioavailability, increased half-life, and greater surface area to volume ratio, effects that allow it various applications such as coatings, biostimulants in plants, plant protection, loading, and release of bioactive molecules [3,8].
Furthermore, the use of CSNP has demonstrated positive effects such as the accumulation of bioactive compounds, which now is an area of interest due to the human health benefits of these antioxidants [9], as demonstrated in wheat, Eleucine coracana and tomato [4,10,11]. The latest research on the application of CSNPs indicate its benefit on plant productivity.
On the other hand, lettuce (Laсtuca sativa L.) is one of the most economically important leafy vegetable crops, in the world. In Mexico, the production of this crop grew by 4.5% in 2020, compared to the previous year (SIAP, 2020). This crop is characterized by its nutritional properties, such as its high content of antioxidant compounds [12]. Based on the above, is possible that chitosan nanoparticles (CSNPs) act as biostimulants increasing the yield and synthesis of bioactive compounds in the lettuce crop. For this reason, this study was to evaluate the effect of CSNPs on the yield, enzymatic activity, and content of bioactive compounds in lettuce.
2.1 Synthesis and Characterization of Chitosan Nanoparticles (CSNPs)
The CSNPs were synthesized at the CIQA (Saltillo, Mexico). Synthesis of chitosan nanoparticles was carried out by ionic gelation following the methodology indicated by Kumaraswamy et al. (2018) [13], using chitosan (viscosimetric molecular weight 200,000 g/mol) (Marine, Hydrocolloids, Kerala, India) and sodium tripolyphosphate (Sigma-Aldrich, USA) as crosslinker. CS was prepared at 0.5% (w/v) in 0.5% (v/v) acetic acid with constant stirring (200 rpm) and TPP at 0.5% (w/v) in distilled water. Crosslinking was carried out at a 10:3 v/v ratio of CS: TPP, 0.5% TPP was added dropwise, under magnetic stirring (600 rpm), and then the solution was centrifuged at 10,000 rpm for 10 min to obtain the CSNPs. Finally, it was washed with deionized water three times, frozen, and dried by lyophilization for further characterization.
2.1.2 Characterization of CSNPs
The obtained CSNPs were characterized by different techniques such as UV-Vis spectroscopy, infrared spectroscopy (FTIR by ATR), light scattering (DLS), thermogravimetric analysis (TGA), and SEM scanning electron microscopy.
Five milligrams of sample were dispersed in 10 mL of deionized water, then sonicated for 10 min at a frequency of 70% in an ultrasound Branson brand; subsequently, the UV absorbance of the CSNPs was determined using a wavelength range from 190 to 400 nm in a UV-Vis spectrophotometer (Shimadzu, UV-240IPC) to identify the characteristic absorption length of the chitosan nanoparticles.
Infrared Spectroscopy Analysis by ATR (FTIR/ATR)
Infrared spectrophotometers of the CSNPs were determined in an FTIR spectrophotometer, Nicolet brand model iS50 FT-IR/ATR, in the range of, 400–4000 cm−1 by directly placing the powdered samples on the diamond crystal.
Particle Size Determination by Dynamic Light Scattering Technique (DLS)
The average size of the CSNPs was determined in a MICROTRAC brand dynamic light scattering (Nanotrac waveII Q); using the same dispersions prepared for UV-Vis employing as reference deionized water for the scattering medium. Five sweeps were performed at a temperature of 25°C in a time of 45 s for each sweep and the average of the measurements was plotted.
Thermogravimetric Analysis (TGA)
The thermal stability of CSNPs was assessed using thermogravimetric analysis (TA Instruments, TGA Q500), under a nitrogen atmosphere, employing a heating rate of 10°C/min within a temperature range of 25°C to 600°C.
Scanning Electron Microscopy (SEM)
A scanning electron microscope (JEOL-JMS-7401F) was used to analyze the samples. The nanoparticles were dispersed in water. Subsequently, a few drops of this dispersion were deposited onto a conductive sample holder, where they were left to dry at 25°C. Micrographs were obtained at magnifications of 9000x.
2.2 Growing Conditions and Plant Material
The study was conducted within a tunnel-style greenhouse under the auspices of the Horticulture Department at the Antonio Narro Autonomous Agrarian University, situated in Buenavista, Saltillo, Coahuila, Mexico. The greenhouse is positioned at 25° 21’ N and 101° 01’ W, with an elevation of 1790 meters. Seeds of lettuce (Lactuca sativa L.) cv. Isla Parris (Huertas®, La Huerta, Mexico) germinated on agricultural foam plates. 20 days after sowing, seedlings transplanted into 10 L black polyethylene bags with a 2:1 (v/v) Peat moss and perlite mixture. The planting density equated to six plants within every square meter. Steiner’s [14] nutrient solution was utilized for crop nutrition, administered via a drip irrigation system with three daily irrigations. During the initial week post-transplanting, irrigation was conducted with a 25% nutrient solution, followed by 50% solution in the second week, 75% in the third week, and 100% in the fourth week post-transplanting. The mean recorded was 22.4°C, with an average photosynthetically active radiation of 677 μmol m−2·s−1 and an average relative humidity of 62%.
The CSNPs were formulated in distilled water containing glycerin and Bionex (Arysta®) as dispersing and coadjutant agents, respectively. Subsequently, they underwent sonication for six minutes at an amplitude of 50% using an ultrasonic equipment (Branson, 1510R-DTH). Solutions were prepared at various concentrations: 0, 0.05, 0.1, 0.1, 0.2, 0.4, and 0.8 mg mL−1. These solutions were applied via foliar spray in the early morning hours (8:00 a.m.), with three applications every two weeks until harvest, commencing 15 days after transplanting (DAT).
At 60 days after transplanting (DAT), ten plants per treatment were analyzed, then measured and weighed to determine: head fresh and dry weight, yield, total phenols, antioxidant capacity, enzyme activity, catalase (CAT), and glutathione peroxidase (GPX).
Lettuces were harvested at 60 DAT obtaining the fresh weight by weighing on an analytical balance (Ohaus®, CS5000P), then dried for four days at room temperature and in an oven (ARSA, Model AR-290AD) at 70°C for 72 h to obtain the dry weight.
A stock solution of extract for analysis was prepared, mixing one gram of fresh pulp in 10 mL of ethanol in a plastic tube with a screw cap, which was placed on a rotatory shaker for 24 h at 5°C and 70 rpm. Afterward, the tubes were centrifuged at 3000 rpm for 5 min, and the supernatant was extracted for analytical tests [15]. The total phenolic content was measured using the Folin–Ciocalteu method [16]. The measurement of absorbance at 765 nm was conducted using a UV-visible spectrophotometer (VE-5100uv-VELAB). The phenolic content was calculated using a standard curve using gallic acid (Sigma, St. Louis, Missouri, USA) as a standard; results were reported in mg of equivalent gallic acid per 100 g of fresh weight (mg Equiv GA·100 g−1 FW), which is the main indicator for this variable.
The antioxidant capacity was evaluated using the in vitro DPPH+ method [17]. A mixture consisting of 50 µL of the sample and 1950 µL of DPPH+ solution was prepared to determine this capacity. After a 30 min incubation period, the absorbance was measured at 517 nm using a UV spectrophotometer (Genesys 10). Trolox (Aldrich, St. Louis, Missouri, USA) was used to establish the standard curve, and the results were expressed as antioxidant capacity (µM Equiv Trolox-100 g−1 fresh weight).
2.7.1 Determination of Enzyme Activity
To prepare the enzymatic extract, 0.2 g of freeze-dried plant tissue using a freezone lyophilizer (LABCONCO), along with 20 mg of polyvinylpyrrolidone, were combined with 1.5 mL of 0.1 M PB at pH 7–7.2 in a 2 mL Eppendorf tube. The mixture was then centrifuged at 12,000 rpm for 10 min at 4°C using a microcentrifuge (Labnet Int., Inc., Taiwan, PrismTm C2500-R). The resulting supernatant was collected and filtered through a 0.45-µm pore-size PVDF membrane [18].
The total protein concentration (TP) was assessed following Bradford’s colorimetric technique [19]. The results were expressed in g protein kg−1 DW.
2.7.3 Catalase (CAT) (EC 1.11.1.6)
CAT activity was determined as described by Cansev et al. [20]. This activity was quantified by measuring two reaction times, time 0 and time 1. The reaction was performed at 20°C and the hydrogen peroxide consumption was determined at 270 nm in a UV-Vis spectrophotometer (Genesys 10s UV-Vis, Thermo Scientific, Waltham, Massachusetts, USA). The calibration curve was prepared with 20 to 200 mM of hydrogen peroxide. The results were reported as specific activity (U mg−1 protein).
2.7.4 Glutation Peroxidase (GPX) (EC 1.11.1.9)
The activity of glutathione peroxidase (GPX) (EC 1.11.1.9) was quantified following the methodology of Flohé et al. [21] and modified by Xue et al. [22] using hydrogen peroxide as a substrate. The absorbance was read in a UV-VIS spectrophotometer (Genesys 10s UV-Vis, Thermo Scientific) at 412 nm, calculating the specific activity (U mg−1 protein) based on the calibration curve made with reduced glutathione (GSH) (0.02 to 1 mM).
The experiment was conducted in a completely randomized design with ten replicates per treatment, and to detect statistical differences between treatments analysis of variance and separation of means according to Fisher’s LSD test (p ≤ 0.05) was performed with the statistical program InfoStat version 2020 [23].
3.1 Synthesis and Characterization of CSNPs
The CSNPs were obtained by the ionic gelation method employing the electrostatic interaction of chitosan (CS) and sodium tripolyphosphate (TPP). By UV-vis spectroscopy, an absorption band was observed at 195 nm confirming the formation of CSNPs, while by FTIR-ATR the characteristic chemical groups of CSNPs were observed [24]. The synthesized CSNPs showed particle sizes of about 111 ± 21 nm with the 10:3 ratio of CS:TPP (Fig. 1a). Thermogravimetric analysis showed the thermal behavior of the CSNPs (Fig. 1b) and Fig. 1c showed the Scanning Electron Microscopy (SEM) images where the CSNPs were observed at the nanoscale size.
Figure 1: Characterization of CSNPs: (a) particle size distribution by DLS, (b) thermal behavior by TGA, and (c) scanning electron microscopy (SEM)
Previous studies have shown that the synthesis of CSNPs by ionic gelation is due to that the protonated amino groups (NH3+) of chitosan in the acidic medium are electrostatically attracted by the negative phosphate groups of TPP [13,25,26]. The above was demonstrated by characterizing CSNPs using the FTIR-ATR. The presence of the characteristic chemical groups of CSNPs such as the band at 3164 cm−1 was due to the vibration of -OH and -NH groups [27,28]. Furthermore, the peaks corresponding to the vibration of -CONH2 at 1628 and 1532 cm−1 due to the interaction of the protonated amino groups of CS with the negative phosphate groups of TPP were observed which confirms the formation of CSNPs [29].
On the other hand, Hoang et al. [5] mentioned that the size of NPs synthesized by the ionic gelation method is usually larger than 100 nm. Similar results were found with a 10:1 CS:TPP ratio where the CSNPs measured from 126.2 to 167.1 nm [30], Al-Nemrawi et al. [31], Kumaraswamy et al. [13] and Alishahi et al. [32] found that the particle size depends on the CS concentration, the CS: TPP ratio, the degree of deacetylation (DD) and the molecular weight of the CS used in the synthesis process.
The thermal behavior of CSNPs shows that the crosslinking of CS increases its thermal stability compared to chitosan from which CSNPs are formed due to the presence of phosphate groups (-P = O and -P-O) [33], four stages were observed: the first weight loss of 11.97%, which is as a result of the dehydration of the sample considering the removal of water, as well as the loss of short chains of the CS glycosidic bonds, the second weight loss from 200°C to 600°C of 39.40%, corresponds to the thermal degradation of the covalent bonds of the CS glycosidic groups with the TPP through dehydration and deamination [34]. In the third stage from 600°C to 625°C, a weight loss of only 7.57% and 32.38% from 625°C to 700°C is observed due to the degradation of polysaccharide backbones [35]; finally, a residue of 8.68% remains, which corresponds to metal residues.
3.2 Treatment of Lettuce with Chitosan Nanoparticles
Foliar spraying of CSNPs on lettuce positively affected biomass production, treatment with 0.4 mg mL−1 of CSNPs increased fresh weight by 24%, and with 0.5 mg mL−1 increased fresh weight by 31% (Fig. 2). These increments in biomass production are attributed to the capability of CSNPs to stimulate plant growth. These nanoparticles contain essential nutrients like C, O, N, and P, promoting cell division and elongation, activating enzymes, and enhancing protein synthesis, resulting in enhanced yield [36,37]. Foliar application of CSNPs is used to increase the growth and production in the plant. CSNPs get easily absorbed by leaves, penetrate the plant through stomata, travel down into the plant through the phloem, and provide nutrients.
Figure 2: Effect of foliar application of CSNPs on lettuce: (a) fresh weight and (b) dry weight. Note: Different letters above the bars indicate a significant difference (p < 0.05) according to the LSD test
To a different part [38,39], it has recently been shown the efficacy of CSNPs relies on the concentration, plant species, and growth stage, indicating that there is no single dose [5,40].
The biosynthesis of antioxidant compounds was enhanced through foliar spraying of CSNPs (Table 1). The treatment with 0.1 mg mL−1 of CSNPs increased the antioxidant capacity by 34.6%, and phenolic by 31.2% concerning the control treatment.
The increase in antioxidant capacity and phenolic compound content in lettuce due to the application of CSNP is related to the plant’s response to stimulants such as CS. When applied, these substances induce the generation of reactive oxygen species (ROS), prompting the synthesis of defensive compounds such as phenolic compounds, flavonoids, anthocyanins, proline, tocopherols, and carotenoids [4,41–43]. Analysis of the proteome or transcriptome of plant tissues treated with chitosan confirms this assumption [44]. Although there are few reports in this regard, the same may occur with the application of CSNPs [41] since CSNPs have the characteristics of CS and the properties of nanoparticles such as surface and interface effect as well as small size [45]. Similar outcomes regarding antioxidant activity were documented in Triticum aestivum L. [4] upon the use of CSNPs, and in Raphanus sativus L. [46] with the application of CS. Furthermore, for the accumulation of phenolic compounds increases have been reported due to the application of CSNPs in Oryza sativa L. [41] and Rosa damascena Mill [47].
The foliar application of CSNPs positively influenced the total protein content in lettuce (Table 2). The highest protein yield, notably at 0.4 mg mL−1 CSNPs concentration, was observed. Moreover, lower CSNPs concentrations (0.05 and 0.1 mg L−1) resulted in the highest CAT activity in lettuce, while the GPX enzyme activity remained relatively stable (Table 2). Similar results were reported in the protein content of Triticum aestivum L. with the application of CSNPs [4].
The increase could be attributed to CSNPs strengthening antioxidant systems such as H2O2 removal leading to protein protection [4], proving that CSNPs protect the plant from protein degradation.
Research has demonstrated that chitosan nanoparticles (CSNPs) can stimulate the production or synthesis of enzymatic antioxidants and facilitate the removal of H2O2. In their study, Hassan et al. [8] observed an increase in catalase (CAT) levels in basil leaves following the application of both CS and CSNPs. CAT is a key enzyme for biological defense mechanisms, as it facilitates the breakdown of accumulated H2O2 in plants into water (H2O) and oxygen (O2), thereby mitigating H2O2-induced damage to the cells of fruits and vegetables [48]. The elevation in CAT activity likely led to a reduction in accumulated H2O2 levels, consequently stabilizing the activity of glutathione peroxidase (GPX) at a basic level [4]. This could elucidate the absence of discernible disparities in GPX enzyme activity within lettuce treated with CSNPs.
The synthesis of CSNPs by the ionic gelation method represents a good option to obtain NPs with particle sizes close to 100 nm, good thermal stability, and biodegradability. The application of CSNPs (0.4 mg mL−1) in lettuce improves yields by up to 24.6% and 0.1 mg mL−1 of CSNPs increases total phenols by 31.2% and antioxidant capacity by 34.6%. In addition, when low concentrations of CSNPs (0.05 and 0.1 mg L−1) were applied, an increase in catalase was observed in lettuce. The foliar application of biodegradable chitosan nanoparticles represents an alternative for increasing yields and obtaining functional foods in sustainable agriculture.
Acknowledgement: To Germán Alvarado Tenorio and Jesús Alejandro Espinosa for their technical support in the laboratory analyses.
Funding Statement: CONAHCyT supported this work through Project A-1-S-20923 and Grant No. 725753 from S. C. Ramírez Rodríguez.
Author Contributions: The authors confirm their contribution to the paper as follows: study conception and design: Silvia C. Ramírez-Rodríguez; data collection, analysis, and interpretation of results: Pablo Preciado-Rangel and Marcelino Cabrera-De La Fuente; draft manuscript preparation: Susana Gonzalèz-Morales; resources, evaluation, and supervision: Hortensia Ortega-Ortiz. All authors reviewed the results and approved the final version of the manuscript.
Availability of Data and Materials: The data that support the findings of this study are available on request from the corresponding author upon reasonable request.
Ethics Approval: Not applicable.
Conflicts of Interest: The authors declare that they have no conflicts of interest to report regarding the present study.
References
1. Shahrajabian MH, Chaski C, Polyzos N, Tzortzakis N, Petropoulos SA. Sustainable agriculture systems in vegetable production using chitin and chitosan as plant biostimulants. Biomol. 2021;11(6):819. [Google Scholar]
2. Ingle PU, Shende SS, Shingote PR, Mishra SS, Sarda V, Wasule DL, et al. Chitosan nanoparticles (ChNPsa versatile growth promoter in modern agricultural production. Heliyon. 2022;8(11):e11893. [Google Scholar] [PubMed]
3. Divya K, Vijayan S, Nair SJ, Jisha MS. Optimization of chitosan nanoparticle synthesis and its potential application as germination elicitor of Oryza sativa L. Int J Biol Macromol. 2019;124:1053–9. [Google Scholar] [PubMed]
4. Hajihashemi S, Kazemi S. The potential of foliar application of nano-chitosan-encapsulated nano-silicon donor in amelioration the adverse effect of salinity in the wheat plant. BMC Plant Biol. 2022;22(1):1–15. [Google Scholar]
5. Hoang NH, Le Thanh T, Sangpueak R, Treekoon J, Saengchan C, Thepbandi W, et al. Chitosan nanoparticles-based ionic gelation method: a promising candidate for plant disease management. Polymers. 2022;14(4):662. [Google Scholar] [PubMed]
6. Hosseini SM, Mazinani S, Abdouss M, Kalhor H, Kalantari K, Amiri IS, et al. Designing chitosan nanoparticles embedded into graphene oxide as a drug delivery system. Polym Bull. 2022;79(1):541–54. [Google Scholar]
7. Malerba M, Cerana R. Recent advances of chitosan applications in plants. Polymers. 2018;10(2):118. [Google Scholar] [PubMed]
8. Hassan FAS, Ali EF, Mostafa NY, Mazrou R. Shelf-life extension of sweet basil leaves by edible coating with thyme volatile oil encapsulated chitosan nanoparticles. Int J Biol Macromol. 2021;177:517–25. [Google Scholar] [PubMed]
9. Maluin FN, Hussein MZ. Chitosan-based agronanochemicals as a sustainable alternative in crop protection. Molecules. 2020;25(7):1611. [Google Scholar] [PubMed]
10. Sathiyabama M, Manikandan A. Foliar application of chitosan nanoparticle improves yield, mineral content and boost innate immunity in finger millet plants. Carbohydr Polym. 2021;258:117691. [Google Scholar] [PubMed]
11. Faizan M, Rajput VD, Al-Khuraif AA, Arshad M, Minkina T, Sushkova S, et al. Effect of foliar fertigation of chitosan nanoparticles on cadmium accumulation and toxicity in Solanum lycopersicum. Biol. 2021;10(7):666. [Google Scholar]
12. Shatilov MV, Razin AF, Ivanova MI. Analysis of the world lettuce market. In: International Conference on Sustainable Development of Cross-Border Regions, 2019; Barnaul, Russian Federation. [Google Scholar]
13. Kumaraswamy R, Kumari S, Choudhary RC, Pal A, Raliya R, Biswas P, et al. Engineered chitosan based nanomaterials: bioactivities, mechanisms and perspectives in plant protection and growth. Int J Biol Macromol. 2018;113:494–506. [Google Scholar] [PubMed]
14. Steiner AA, Abraham A. The universal nutrient solution. In: Proceedings 6th International Congress on Soilless Culture, 1984; Luntern. p. 633–50. [Google Scholar]
15. Preciado-Rangel P, Troyo-Diéguez E, Valdez-Aguilar LA, García-Hernández JL, Luna-Ortega JG. Interactive effects of the potassium and nitrogen relationship on yield and quality of strawberry grown under soilless conditions. Plants. 2020;9(4):441. [Google Scholar] [PubMed]
16. Singleton VL, Orthofer R, Lamuela-Raventós RM. [14] Analysis of total phenols and other oxidation substrates and antioxidants by means of folin-ciocalteu reagent. Meth Enzymol. 1999;299:152–78. [Google Scholar]
17. Brand-Williams W, Cuvelier ME, Berset CLWT. Use of a free radical method to evaluate antioxidant activity. LWT-Food Sci. Technol. 1995;28(1):25–30. [Google Scholar]
18. Ramos SJ, Faquin V, Guilherme LRG, Castro EM, Ávila FW, Carvalho GS, et al. Selenium biofortification and antioxidant activity in lettuce plants fed with selenate and selenite. Plant Soil Environ. 2010;56(12):584–8. doi:10.17221/113/2010-PSE. [Google Scholar] [CrossRef]
19. Cheng Y, Wei H, Sun R, Tian Z, Zheng X. Rapid method for protein quantitation by Bradford assay after elimination of the interference of polysorbate 80. Anal Biochem. 2016;494:37–9. doi:10.1016/j.ab.2015.10.013. [Google Scholar] [PubMed] [CrossRef]
20. Cansev A, Gulen H, Eris A. The activities of catalase and ascorbate peroxidase in olive (Olea europaea L. cv. Gemlik) under low temperature stress. Horticulture Environ Biotechnol. 2011;52(2):113–20. doi:10.1007/s13580-011-0126-4. [Google Scholar] [CrossRef]
21. Flohé L, Günzler WA. Assays of glutathione peroxidase. In: Methods in enzymology. Academic Press; 1984. vol. 105, p. 114–20. [Google Scholar]
22. Xue T, Hartikainen H, Piironen V. Antioxidative and growth-promoting effect of selenium on senescing lettuce. Plant Soil. 2001;237(1):55–61. doi:10.1023/A:1013369804867. [Google Scholar] [CrossRef]
23. di Rienzo JA, Casanoves F, Balzarini MG, González LA, Tablada EM, Robledo CW. InfoStat versión 2020. Statistical software. Córdoba, Argentina: Grupo InfoStat (In Spanish). [Google Scholar]
24. Ramírez SC, Ortega H, Fortis M, Nava JM, Orozco JA, Preciado P. Chitosan nanoparticles improve the nutraceutical quality of triticale sprouts. Rev Mexicana Cienc Agrícolas. 2021;12:579–89 (In Spanish). [Google Scholar]
25. Divya K, Thampi M, Vijayan S, Varghese S, Jisha MS. Induction of defense response in Oryza sativa L. against Rhizoctonia solani (Kuhn) by chitosan nanoparticles. Microbial Pathog. 2020;149:104525. [Google Scholar]
26. Vázquez R, Caro-León FJ, Nakal A, Ruiz S, Doñoro C, García-Fernández L, et al. DEAE-chitosan nanoparticles as a pneumococcus-biomimetic material for the development of antipneumococcal therapeutics. Carbohydr Polym. 2021;273:118605. [Google Scholar]
27. Nasr F, Pateiro M, Rabiei V, Razavi F, Formaneck S, Gohari G, et al. Chitosan-phenylalanine nanoparticles (Cs-Phe Nps) extend the postharvest life of persimmon (Diospyros kaki) fruits under chilling stress. Coatings. 2021;11(7):819. [Google Scholar]
28. Cruz GJF, Aguirre D, Canepa C, Gómez MM, Solís JL, Santiago J, et al. Agricultural residues-based activated carbons impregnated with chitosan nanoparticles by hydrothermal treatment. J Phy Conf Ser. 2022;2180(1):012019. [Google Scholar]
29. Manikandan A, Sathiyabama M. Preparation of chitosan nanoparticles and its effect on detached rice leaves infected with Pyricularia grisea. Int. J. Biol. Macromol. 2016;84:58–61. [Google Scholar] [PubMed]
30. Suryadi Y, Priyatno TP, Samudra I, Susilowati DN, Sriharyani TS, Syaefudin S. Control of anthracnose disease (Colletotrichum gloeosporioides) using nano chitosan hydrolyzed by Chitinase derived from Burkholderia cepacia Isolate E76. J AgroBiogen. 2017;13(2):111–22. [Google Scholar]
31. Al-nemrawi NK, Alkhatib RQ, Ayyad H, Nid AA. Formulation and characterization of tobramycin-chitosan nanoparticles coated with zinc oxide nanoparticles. Saudi Pharm. J. 2022;30(4):454–61. [Google Scholar] [PubMed]
32. Alishahi A, Mirvaghefi A, Tehrani MR, Farahmand H, Shojaosadati SA, Dorkoosh FA, et al. Shelf life and delivery enhancement of vitamin C using chitosan nanoparticles. Food Chem. 2011;126(3):935–40. [Google Scholar]
33. Kahdestani SA, Shahriari MH, Abdouss M. Synthesis and characterization of chitosan nanoparticles containing teicoplanin using sol-gel. Polym. Bull. 2021;78(2):1133–48. [Google Scholar]
34. Moeini A, Cimmino A, Dal Poggetto G, di Biase M, Evidente A, Masi M, et al. Effect of pH and TPP concentration on chemico-physical properties, release kinetics and antifungal activity of Chitosan-TPP-Ungeremine microbeads. Carbohydr Polym. 2018;195:631–41. [Google Scholar] [PubMed]
35. Thandapani G, Prasad S, Sudha PN, Sukumaran A. Size optimization and in vitro biocompatibility studies of chitosan nanoparticles. Int J Biol Macromol. 2017;104:1794–806. [Google Scholar] [PubMed]
36. Chakraborty M, Hasanuzzaman M, Rahman M, Khan M, Rahman A, Bhowmik P. Mechanism of plant growth promotion and disease suppression by chitosan biopolymer. Agric. 2020;10(12):624. [Google Scholar]
37. Prajapati D, Pal A, Dimkpa C, Singh U, Devi KA, Choudhary JL, Saharan V. Chitosan nanomaterials: a prelim of next-generation fertilizers; existing and future prospects. Carbohydr Polym. 2022;288:119356. [Google Scholar] [PubMed]
38. Lin S, Reppert J, Hu Q, Hudson JS, Reid ML, Ratnikova TA, et al. Uptake, translocation, and transmission of carbon nanomaterials in rice plants. Small. 2009;5(10):1128–32. [Google Scholar] [PubMed]
39. Abdel-Aziz H, Hasaneen MN, Omar A. Effect of foliar application of nano chitosan NPK fertilizer on the chemical composition of wheat grains. Egypt. J. Bot. 2018;58(1):87–95. [Google Scholar]
40. Balusamy SR, Rahimi S, Sukweenadhi J, Sunderraj S, Shanmugam R, Thangavelu L, et al. Chitosan, chitosan nanoparticles and modified chitosan biomaterials, a potential tool to combat salinity stress in plants. Carbohydr Polym. 2022;284:119189. [Google Scholar] [PubMed]
41. Divya K, Thampi M, Vijayan S, Varghese S, Jisha MS. Induction of defense response in Oryza sativa L. against Rhizoctonia solani (Kuhn) by chitosan nanoparticles. Microb Pathog. 2020;149:104525. [Google Scholar] [PubMed]
42. Ishkeh SR, Shirzad H, Asghari MR, Alirezalu A, Pateiro M, Lorenzo JM. Effect of chitosan nanoemulsion on enhancing the phytochemical contents, health-promoting components, and shelf life of raspberry (Rubus sanctus Schreber). Appl Sci. 2021;11(5):2224. [Google Scholar]
43. Singh S. Enhancing phytochemical levels, enzymatic and antioxidant activity of spinach leaves by chitosan treatment and an insight into the metabolic pathway using DART-MS technique. Food Chem. 2016;199:176–84. [Google Scholar] [PubMed]
44. Du Jardin P. Plant biostimulants: definition, concept, main categories and regulation. Sci Hortic. 2015;196:3–14. [Google Scholar]
45. Boroumand H, Badie F, Mazaheri S, Seyedi ZS, Nahand JS, Nejati M, et al. Chitosan-based nanoparticles against viral infections. Front Cell Infect Microbiol. 2021;11:175. [Google Scholar]
46. Supapvanich S, Anan W, Chimsonthorn V. Efficiency of combinative salicylic acid and chitosan preharvest-treatment on antioxidant and phytochemicals of ready to eat daikon sprouts during storage. Food Chem. 2019;284:8–15. [Google Scholar] [PubMed]
47. Ali EF, Issa AA, Al-Yasi HM, Hessini K, Hassan FAJB. The efficacies of 1-methylcyclopropene and chitosan nanoparticles in preserving the postharvest quality of damask rose and their underlying biochemical and physiological mechanisms. Biol. 2022;11(2):242. [Google Scholar]
48. Zárate-Martínez W, Morales SG, Godina FR, Olivo AR, Maldonado AJ. Efecto de los ácidos fenólicos en el sistema antioxidante de plantas de tomate (Solanum lycopersicum Mill.). Agron Mesoamerican. 2021;32(3):854–68 (In Spanish). [Google Scholar]
Cite This Article
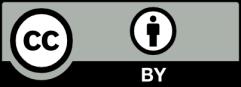
This work is licensed under a Creative Commons Attribution 4.0 International License , which permits unrestricted use, distribution, and reproduction in any medium, provided the original work is properly cited.