Open Access
ARTICLE
The Combination of Achnatherum inebrians Extracts and Soil Microorganisms Inhibited Seed Germination and Seedling Growth in Elymus nutans
1 State Key Laboratory of Herbage Improvement and Grassland Agro-Ecosystems, Key Laboratory of Animal Husbandry Innovation, Ministry of Agriculture and Rural Affairs, Engineering Research Center of Grassland Agriculture, Ministry of Education, West Gansu Province Prataculture Technology Innovation Center, College of Pastoral Agriculture Science and Technology, Lanzhou University, Lanzhou, 730020, China
2 Center for Grassland Research, National Forestry and Grassland Administration, CaF, Beijing, 100091, China
* Corresponding Author: Chunjie Li. Email:
(This article belongs to the Special Issue: The Impact of Invasive Alien Plant Species on Plant Communities and the Ecosystem Services They Provide)
Phyton-International Journal of Experimental Botany 2024, 93(3), 567-580. https://doi.org/10.32604/phyton.2024.047485
Received 07 November 2023; Accepted 06 February 2024; Issue published 28 March 2024
Abstract
In a greenhouse experiment, the effects of soil microorganisms and extracts of Achnatherum inebrians on the seed germination and seedling growth of Elymus nutans were studied. The results showed that both the extracts from aboveground and belowground parts of A. inebrians significantly inhibited the germination rate, germination potential, germination index, vigor index, seedling height, root length, and fresh weight of E. nutans, but increased malondialdehyde content, catalase, peroxidase and superoxide dismutase activity of E. nutans seedlings (p < 0.05). The allelopathy of aqueous extracts of the aboveground parts of A. inebrians was stronger than that of the precipitates. Aqueous extracts of the aboveground parts of A. inebrians decreased seed germination rate, germination potential, germination index, vigor index, seedling length, root length, and seedling fresh weight by 10.45%–74.63%, 24.18%–32.50%, 19.03%–73.36%, 37.83%–88.41%, 21.42%–53.14%, 2.65%–40.21%, and 20.45%–61.36%, respectively, and malondialdehyde content, peroxidase, catalase, and superoxide dismutase activity increased by 8.09%–62.24%, 27.83%–86.47%, 22.90%–93.17%, and 11.15%–75.91%, respectively. The above indexes were higher in live soil than in sterilized soil. Soil microorganisms increased the allelopathy of A. inebrians. The seed germination rate, germination potential, germination index, vigor index, seedling length, and seedling fresh weight of E. nutans planted in live soil decreased by 8.22%–48.48%, 10.00%–51.85%, 8.19%–53.26%, 16.43%–60.03%, 12.91%–28.81%, and 9.09%–22.86% compared with sterilized soil, respectively. Malondialdehyde content, peroxidase, catalase, and superoxide dismutase activity of E. nutans planted in live soil increased by 53.91%–81.06%, 15.71%–57.34%, 33.33%–86.31%, and 9.78%–52.51% compared with sterilized soil, respectively. The existence of soil microorganisms enhanced the allelopathy of the secondary metabolites of A. inebrians. A combination of microorganisms and aqueous extracts from the aboveground parts of A. inebrians had the strongest allelopathic effect on E. nutans.Keywords
Grass that causes poisoning and even the death of livestock is referred to as poisonous grass. Such grasses grow widely in natural grasslands around the world. The average number of dead livestock caused by poisonous grasses in China is approximately 8.4 × 105 head per year, with a direct economic loss of approximately $410 million [1]. More than 4.5 × 107 hm2 of the Chinese natural grasslands have been invaded by poisonous grasses. Among them, 1.12 × 107 hm2 of this area has been seriously infested with these invasive plants. These dangerous grasses primarily include species of the Poaceae, Ranunculaceae, Euphorbiaceae, Thymelaeaceae, and Leguminosae [2,3].
Drunken horse grass (Achnatherum inebrians) is a common poisonous grass in the Poaceae, and it is widely distributed in the natural grasslands of Northern China [4–6]. Studies have shown that this species has invaded more than 85% of the land in some severely degraded areas [7–9]. A. inebrians grows rapidly on grasslands, and it competes with other plants for space to grow, nutrients, water, and other resources, which leads to degradation of the grassland and a decrease in high-quality forage [10,11]. The strong allelopathic effects of A. inebrians is the most important reason that enables it to compete with other plants. Previous study has reported that the rhizosphere soil of A. inebrians decreased the germination rate and index of the sedge grass Carex alatauensis seeds by 80% and 82.5%, respectively [12]. A water extract of A. inebrians significantly inhibited the seeds germination and seedling growth of the perennial grass (Stipa capillata) [13]. The volatile compounds of A. inebrians increased the malondialdehyde (MDA) content and the activities of superoxide dismutase (SOD) and peroxidase (POD) in perennial ryegrass (Lolium perenne) seedlings by 15.64%, 27.72% and 23.38%, respectively [14].
A variety of compounds are involved in the process of allelopathy, which is often affected by numerous factors [15,16]. Many studies have shown that soil microorganisms alter the activity of allelochemicals in plants, and they can also alter the competitiveness of invasive plants in populations and communities [17–20]. Existing studies have focused on the degradation of toxic compounds into toxic allelochemicals by free-living microbial communities in the soil [19–21], the enhancement of plant tolerance to allelochemicals [22,23], and the expansion of the impact of allelochemicals by soil microorganisms through transfer networks in the soil [24].
The primary distribution of allelochemicals in A. inebrians, their form of existence, and the effects of soil microorganisms on allelopathy have not been examined to date. In this study, the allelopathy of aqueous extracts and aqueous extract sediments of different parts of A. inebrians on Elymus nutans in sterilized and live soil were examined. Pot germination tests were conducted in a greenhouse using both the aboveground and belowground parts of drunken horse grass as test materials to assess morphological, physiological, and biochemical indicators. This study proposed the following hypotheses: (1) There is variable distribution of the allelochemicals in the different parts of A. inebrians; (2) Most of the allelochemicals in A. inebrians are soluble; and (3) Soil microorganisms affect the degree of stress imposed on the plants by allelochemicals.
2.1 Soil Samples and Plant Materials
In October 2021, the A. inebrians were collected from the experimental field of Jinniushan Park, Yuzhong County, Lanzhou City, Gansu Province, China (103°49′15″E, 35°34′20″N), and transported to the laboratory. The seeds of E. nutans were wild resources, collected in September 2020 in Maqu County, Gannan Tibetan Autonomous Prefecture, Gansu Province, China (100°45′45″–102°29′00″E, 33°06′30″–34°30′15″N), and were stored in a dry and ventilated place in the laboratory after cleaning. Soil samples were taken from a bare field of Lanzhou University (Yuzhong campus) that had not been cultivated for several years. Sterilized soil was obtained by heating live soil in an oven at 150°C for 12 h. Each treatment was repeated three times.
2.2 Preparation of Allelochemicals from A. inebrians
The aboveground and belowground parts of A. inebrians plants were separated after washing; 75.0 g of biomass was weighed and ground with a grinder and put it in a 1000 ml conical bottle. Then, extract is obtained by adding 1000 ml of distilled water to the conical bottle and shaking it on a shaker for 48 h. The aqueous extract of A. inebrians was filtered with double filter paper to obtain 75 g·L−1 aqueous extract of the stems, leaves, and roots of A. inebrians. The precipitate on the filter paper was recovered. The aqueous extract and the precipitate were irradiated under an ultraviolet lamp for 1 h and then stored at 4°C.
2.3 Determination of Allelopathy
The germination experiment was conducted in the greenhouse of the Yuzhong Campus of Lanzhou University. The average daytime greenhouse temperature was 25°C, the average nighttime greenhouse temperature was 18°C, the average daytime relative humidity was 72%, and the average nighttime relative humidity was 81%. Ten uniform and plump E. nutans seeds of the same size were selected, soaked in 2% NaClO for 10 min, and then soaked in distilled water for 10 min, dried, and scattered in flowerpots containing sterilized soil and fresh live soil. A total of 150 ml of A. inebrians stem, leaf, and root aqueous extract was added to 9.4 g of aqueous extract of sediment of A. inebrians [23], using distilled water as control (CK). The appropriate quantity of distilled water was supplemented every day, and the germinated seeds were counted every day (seedlings exposed to the soil surface formed the germination standard). The germination experiment was completed after 15 days. Other indicators were measured after the plants had been taken back to the laboratory on day 16. Each treatment was repeated three times.
2.4 Seed Germination Index, Enzymatic Activity, and MDA Content
The germination rate (GR), germination index (GI), and vigor index (VI) were calculated on the 15th day. The germination potential (GP) was calculated on the 5th day of germination. On the 15th day of germination, 10 seedlings were randomly selected from each flowerpot, and the seedling length (SL), root length (RL), and seeding fresh weight were measured after excess water had been absorbed by filter paper. The average value was taken to calculate the allelopathic effect index (RI). If there were less than 10 plants, all available plants were measured and the average value was taken.
The calculation formula of each index is as follows [25–27]:
In this formula, Gt is the daily germination number, and Dt is the corresponding germination day.
In this formula, GI is the germination index, and S is the root length.
In this formula, C represents the data of the control group, T represents the data of the treatment group, RI < 0 represents an inhibitory effect, and RI > 0 represents a promoting effect.
SOD, POD, and CAT activities as well as MDA content were calculated according to the method of Sun et al. [28].
In this formula, A532 is the absorbance at 532 nm, A600 is the absorbance at 600 nm, and A450 is the absorbance at 450 nm. VT is the total volume of extracted enzyme liquid (ml), and Vs is the volume of enzyme liquid taken during determination (mL).
In this formula, ΔA470 is the change of absorbance value during the reaction time, and W is the plant fresh weight (g). VT is the total volume of extracted enzyme liquid (ml), and Vs is the volume of enzyme liquid taken during determination (mL). t is the reaction time (min).
In this formula, AS0 is the absorption value of the added deactivated enzyme solution, and AS1 and AS2 are the absorbance values of the sample tube. VT is the total volume of crude enzyme extract (ml), V1 is the volume of liquid extracted by the crude enzyme (ml), and FW is the sample fresh weight (g). The factor of 0.1 indicates that every 0.1 decrease in A240 represents 1 enzyme activity unit (μ). t is the time from the first reading to the last reading (min).
In this formula, SOD activity is expressed in fresh-weight enzyme units per gram, ACK is the absorbance value of CK, and AE is the absorbance value of the sample tube. V is the total volume of the sample liquid (mL), Vt is the sample dosage at the time of determination (mL), and W is the sample fresh weight (g).
Data analysis were performed with SPSS 25.0 (SPSS, Inc., Chicago, IL, USA). One-way ANOVA was used to determine the effects of allelochemical treatment and soil treatment on germination parameters. ANOVA was also used to analyze differences in the length of seedling, root, biomass, MDA content, as well as POD, SOD, and CAT activities. Significant differences between all parameters in plants grown on sterilized and live soil under the same allelochemical treatment conditions were determined using an independent t-test. Statistical significance was defined at the 95% confidence level. Data in figures are shown as means with standard errors. MDA content as well as POD, SOD, and CAT activities are depicted using GraphPad Prism 8.3.0 (GraphPad Software, San Diego, California, USA).
3.1 Seed Germination Characteristics of E. nutans
The GR of E. nutans seeds in both sterilized and live soil were decreased under allelochemical treatment (Table 1), but a significant difference in GR was not found between sterilized and live soil. In both sterilized and live soil, the order of GR under different allelochemicals was D > C > B > A. There were no significant differences in the GR of A, B, C, and D treatments in sterilized soil. Compared with CK, the GR in A, B, C, and D treatments decreased significantly by 54.79%, 49.32%, 41.10%, and 27.40% (p < 0.05). In live soil, compared with CK, the GR under A and B treatments decreased significantly by 74.63% and 40.30%, respectively (p < 0.05).
The GP of E. nutans seeds in sterilized and live soil decreased under all allelochemical treatments (Table 1). The GP of CK, A, B, and D treatments was higher than that of live soil. Compared with sterilized soil, C treatment in live soil decreased significantly by 50%. The existence of soil microorganisms under allelochemical treatment inhibited the GP. In sterilized soil, compared with CK, the GP of treatments A, B, C, and D decreased significantly by 57.14%, 52.38%, 63.49%, and 25.40%, respectively. In live soil, compared with CK, the GP in A treatment decreased significantly by 67.50% (p < 0.05).
In the same column, different lowercase letters indicate significant differences between treatments under one-way ANOVA at the p < 0.05. In the same row, different capital letters indicate significant differences between treatments under the t-test at the p < 0.05.
Compared with seeds without allelochemical treatment, the GI of E. nutans seeds in sterilized and live soil were decreased under all allelochemical treatments (Table 2). The GI of CK, A, C, and D treatments in sterilized soil were higher than those in live soil. Compared with sterilized soil, in live soil, the GI of A and D treatments significantly decreased by 53.26% and 33.54%, respectively (p < 0.05). Soil microorganisms had an inhibitory effect on the GI treated with allelochemicals. In both sterilized and live soil, the GI treated with allelochemicals can be ordered according to D > C > B > A. In sterilized soil, compared with CK, the GI of A, B, and C treatments significantly decreased by 49.79%, 60.10%, and 40.57%, respectively (p < 0.05). In live soil, the GI of A and B treatments were significantly lower than that of the CK treatment by 73.36% and 54.71%, respectively (p < 0.05).
The seed VI of E. nutans in sterilized and live soil decreased under all allelochemical treatments. The VI in sterilized soil was higher than that in live soil of CK, A, B, C, and D treatments. Compared with sterilized soil, CK and A treatments in live soil significantly decreased by 16.43% and 60.03%, respectively (p < 0.05). Soil microorganisms had an inhibitory effect on the VI treated with allelochemicals. In both sterilized and live soil, the order of the VI under different allelochemicals was D > C > B > A. In sterilized soil, compared with CK, the seed VI of A, B, C, and D treatments significantly decreased by 75.77%, 59.88%, 54.48%, and 37.83%, respectively (p < 0.05). Compared with CK planted in live soil, the VI planted in live soil of A, B, C, and D treatments significantly decreased by 88.41%, 74.07%, 54.82%, and 48.25%, respectively (p < 0.05).
In the same column, different lowercase letters indicate significant differences between treatments under one-way ANOVA at the p < 0.05. In the same row, different capital letters indicate significant differences between treatments under the t-test at the p < 0.05.
3.2 Growth Characteristics of E. nutans Seedlings
The SL of E. nutans under any allelochemical treatment planted in sterilized and live soil decreased compared with E. nutans without treatment (Table 3). The SL of E. nutans planted in sterilized soil exceeded that of seeds planted in live soil under CK, A, B, C, and D treatments. Compared with sterilized soil, B treatment in live soil decreased significantly by 28.82% (p < 0.05). Soil microorganisms had an inhibitory effect on the SL o treated with allelochemicals. The SL planted in sterilized and live soil and treated with allelochemicals could be ordered according to C > D > B > A. In sterilized soil, significant differences were found between A, B, C, D, and CK treatments. Compared with E. nutans in the CK treatment planted in sterilized soil, the SL of A, B, C, and D treatments significantly decreased by 48.30%, 24.18%, 21.42%, and 23.19%, respectively (p < 0.05). Compared with E. nutans planted in live soil of the CK treatment, the SL of A, B, C, and D treatments decreased significantly by 53.14%, 43.12%, 27.88%, and 34.83%, respectively (p < 0.05).
After any form of allelochemical treatment, the RL of E. nutans seedlings planted in both sterilized and live soil was significantly decreased (p < 0.05). The RL seedlings planted in sterilized soil of CK, A, B, C, and D treatments were similar to the lengths of seedlings planted in live soil. In both sterilized and live soil, the RL under D treatment was slightly shorter than that under C treatment except for the sterilized soil treatment. The RL of seedlings under different allelochemical treatments can be ordered according to D > C > B > A. Compared with CK, the RL seedlings of A, B, C, and D treatments significantly decreased by 36.28%, 30.44%, 18.23%, and 20.18%, respectively (p < 0.05). There was no difference between treatments in live soil.
The fresh weight of E. nutans seedlings planted in both sterilized and live soil decreased under any allelochemical treatment (Table 3). The fresh weight of seedlings planted in sterilized soil of CK, A, B, C, and D treatments was similar to weights obtained in live soil. In response to treatment with different allelochemicals, the fresh weight of seedlings planted in sterilized and live soil decreased in the order of D > C > B > A. In sterilized soil, significant differences were found between treatments A, B, and CK. Compared with CK, the fresh weight under A and B treatments significantly decreased by 50.00% and 61.36%, respectively (p < 0.05). Compared with CK, the fresh weight under A, B, and C treatments significantly decreased by 55.56%, 48.89%, and 48.89%, respectively (p < 0.05).
In the same column, different lowercase letters indicate significant differences between treatments under one-way ANOVA at the p < 0.05. In the same row, different capital letters indicate significant differences between treatments under the t-test at the p < 0.05.
3.3 MDA Content as Well as POD, CAT, and SOD Activity of E. nutans
The MDA content of E. nutans under any allelochemical treatment was higher than that of CK. The MDA content of E. nutans in sterilized soil under any allelochemical treatment was lower than that in live soil (Fig. 1). Compared with sterilized soil, the MDA contents of CK, A, B, C, and D treatments in live soil significantly increased by 116.94%, 428.03%, 167.67%, 157.08%, and 157.01%, respectively (p < 0.05). Soil microorganisms had an inhibitory effect on the MDA activity of E. nutans treated with allelochemicals. In sterilized soil, there were no significant differences between A, B, C, D, and CK. The MDA contents of E. nutans in live soil of A, B, C, and D treatments were significantly higher than that of CK by 164.82%, 138.23%, 69.93%, and 67.65%, respectively (p < 0.05).
Figure 1: MDA content of E. nutans under allelochemical treatments. CK: Without allelochemical treatment; A: An aqueous extract of an above-ground part of A. inebrians; B: A part of aqueous extract sediments on that ground of the A. inebrians; C: Aqueous extract of that belowground part of A. inebrians, D: A part of aqueous extract sediments on that belowground part of A. inebrians. Different lowercase letters on top of bars indicate significant differences (p < 0.05) of plants between the different allelochemical treatments
The POD activity of E. nutans under allelochemical treatment was higher than that of CK. The POD activity of E. nutans in sterilized soil under any allelochemical treatment was lower than that in live soil (Fig. 2). Compared with sterilized soil, A and C treatments in live soil increased significantly by 73.46% and 119.00%, respectively (p < 0.05). Soil microorganisms inhibited the POD activity of E. nutans treated with allelochemicals. In sterilized soil, the POD activity was higher under A than under CK by 392.61% and 230.95%, respectively. Compared with CK, the POD activity of E. nutans planted in live soil under A, B, C, and D treatments increased by 640.00%, 240.00%, 206.60%, and 80.00%, respectively.
Figure 2: POD activity of E. nutans under allelochemical treatments. CK: Without allelochemical treatment; A: An aqueous extract of an above-ground part of A. inebrians; B: A part of aqueous extract sediments on that ground of the A. inebrians; C: Aqueous extract of that belowground part of A. inebrians, D: A part of aqueous extract sediments on that belowground part of A. inebrians. Different lowercase letters on top of bars indicate significant differences (p < 0.05) of plants between the different allelochemical treatments
The CAT activity of E. nutans with allelochemical treatment was higher than that of CK. The CAT activity in sterilized soil under any allelochemical treatment was lower than that in live soil (Fig. 3). Compared with sterilized soil, the CAT activities of A, B, and D treatments in live soil significantly increased by 87.44%, 81.24%, and 229.00%, respectively (p < 0.05). Soil microorganisms had an inhibitory effect on the CAT activity of E. nutans treated with allelochemicals. In sterilized soil, compared with CK, the CAT activity under treatment A significantly increased by 188.73%. In live soil, compared with CK, the CAT activities under A, B, and D treatments significantly increased by 441.18%, 242.86%, and 191.19%, respectively.
Figure 3: CAT activity of E. nutans under allelochemical treatments. CK: Without allelochemical treatment; A: An aqueous extract of an above-ground part of A. inebrians; B: A part of aqueous extract sediments on that ground of the A. inebrians; C: Aqueous extract of that belowground part of A. inebrians, D: A part of aqueous extract sediments on that belowground part of A. inebrians. Different lowercase letters on top of bars indicate significant differences (p < 0.05) of plants between the different allelochemical treatments
The SOD activity of E. nutans under any allelochemical treatment was higher than that of CK. Except for the SOD activity of E. nutans treated with allelochemicals in sterilized soil under CK treatment, which was higher than that in the live soil. The SOD activities in sterilized soil under all other treatments were significantly lower than in live soil (p < 0.05) (Fig. 4). Compared with sterilized soil, the SOD activities in A and B treatments in live soil significantly increased by 110.55% and 99.03%, respectively (p < 0.05). Soil microorganisms had an inhibitory effect on the SOD activity of E. nutans treated with allelochemicals. In sterilized soil, compared with CK, the SOD activity of A treatment increased by 125.91%. In live soil, compared with CK, the SOD activities under A and B treatments were significantly higher than that of CK by 315.30% and 126.85%, respectively (p < 0.05).
Figure 4: SOD activity of E. nutans under allelochemical treatments. CK: Without allelochemical treatment; A: An aqueous extract of an above-ground part of A. inebrians; B: A part of aqueous extract sediments on that ground of the A. inebrians; C: Aqueous extract of that belowground part of A. inebrians, D: A part of aqueous extract sediments on that belowground part of A. inebrians. Different lowercasee letters on top of bars indicate significant differences (p < 0.05) of plants between the different allelochemical treatments
3.4 Allelopathic Effect Indices of E. nutans
The RI was used to evaluate the allelopathic effect of A. inebrians on E. nutans using the GR, GP, GI, VI, SL, RL, and seedling fresh weight (Table 4). As shown by the RI means, GP, GR, GI, VI, SL, RL, and seedling fresh weight had negative values (from −0.02 to −0.79). The synthetical allelopathic indexes of A. inebrians of aboveground and belowground extracts were negative (from −0.17 to −0.79 and from −0.02 to −0.69). The synthetical allelopathic indexes of sterilized soil and live soil were negative (from −0.17 to −0.79 and from −0.02 to −0.74) (Table 4).
In the same column, different lowercase letters indicate significant differences between treatments under one-way ANOVA at the p < 0.05. In the same row, different capital letters indicate significant differences between treatments under the t-test at the p < 0.05.
4.1 Seed Germination, Seedling Growth, and Physiological Characteristics of E. nutans Seeds
The bases of plant growth, development, and adaptation to adversity are seed germination and seedling growth, which are sensitive and vulnerable to allelopathic stress. After treatment with allelochemicals, the seed germination rate, vigor, as well as growth of seedlings and radicles decreased [29–31]. The results of the present study showed that the GR, GP, GI, and VI of E. nutans decreased by any A. inebrians extract. Compared with aqueous extract and aqueous extract precipitate of belowground parts of A. inebrians, the inhibitory effect of aboveground parts on seed germination was particularly strong; the inhibitory effect of the aqueous extracts of aboveground parts on seed germination was the strongest [32].
The decline of seedling and radicle length is the most intuitive response to stress. Research has shown that allelopathic stress can cause the growth of seedlings and radicles to slow down or even stagnate, resulting in a decrease in biomass [33–35]. The results of this study showed that the growth of E. nutans seedlings was inhibited by allelopathic stress at the seed germination. The inhibitory effect was reflected by decreases in seedling length, root length, and fresh weight. Compared with belowground parts, the allelopathic effects of the aqueous extracts of A. inebrians on seedling growth were the strongest. Zhu et al. found that the aqueous extracts from the aboveground parts of A. inebrians have a stronger inhibitory effect on the germination of four other plants than the extracts of the belowground parts [34].
SOD, POD, and CAT constitute the active oxygen scavenging system in cells and play a key role in the metabolism of active oxygen [16,36,37]. MDA is the product when the plant is subjected to stress, and its content reflects the degree of injury to cells [38,39]. Plant responses to allelochemicals include decreasing ROS breakdown products and enhancing the activities of antioxidant defense enzymes [40,41]. The results showed that the activities of SOD, POD, and CAT as well as the MDA content in seedlings increased significantly under A. inebrians plant aqueous extract and aqueous extract treatments. This result indicates that the seedlings were stressed by allelochemicals, which led to increases in the activities of antioxidant defense enzymes and cell damage [42,43]. The MDA content, as well as POD, SOD, and CAT activities of E. nutans seedlings treated with aboveground parts of water immersion and water immersion sediment, were generally higher than that of the belowground part of water immersion and water immersion sediment. Moreover, the water extract treatment with the aboveground part of A. inebrians had the most significant effects on antioxidant defense enzyme activity and ROS decomposition products. The above results indicate that seed germination, seedling growth, and physiological characteristics support hypotheses (1) and (2): Allelochemicals in shoots and roots of A. inebrians inhibit seed germination and seedling growth of E. nutans; however, the allelopathic potentials of aboveground and belowground parts of A. inebrians differ. Allelochemicals of A. inebrians are mainly distributed in the aboveground part and are mainly water-soluble substances.
The positive or negative of RI value indicates whether allelopathy has a positive effect on plants [44–46]. In terms of the average values of seed germination and seedling growth, the RI values were negative, indicating that all allelochemicals exerted inhibitory effects on the growth of E. nutans. The magnitude of inhibition could be ordered according to A > B > C > D, where A had the strongest inhibitory effect on E. nutans.
4.2 Influence of Soil Microorganisms on Allelopathy
Soil microbial species are diverse and their composition varies greatly in both space and time. The complex composition of soil microorganisms causes allelochemicals to change when they enter the soil [47–49]. Existing research showed that in response to the leaves of Rhododendron formosanum entering the soil, Pseudomonas transformed the main allelochemical (−)-catechin into the more phytotoxic protocatechuic acid. Moreover, protocatechuic acid could enhance the inhibitory effect of (−)-catechin on seed germination at low concentrations [50]. Combined with the results of this study, soil microorganisms enhanced the allelopathy of A. inebrians to seedings of E. nutans, as reflected by seed germination, seedling growth, MDA content, as well as POD, SOD, and CAT activities of E. nutans seedings. The results are also consistent with hypothesis (3). Soil microorganisms significantly enhance the allelopathy of A. inebrians, which may be associated with the decomposition of allelochemicals by soil microorganisms into other chemical substances. The allelopathic effect of the transformed chemical substances on plants may impose stronger inhibition than allelochemicals before their decomposition and transformation [51,52].
The response of E. nutans to A. inebrians extracts and soil microorganisms through the determination of seed germination and seedling physiological indexes were studied. The changes in seed germination under allelochemical treatment directly reflect the damage caused by allelopathic stress on plants. Physiological indicators also reflected the strong allelopathic effect of A. inebrians on E. nutans. The germination and seedling growth of E. nutans were examined in both sterilized soil and live soil treated with the aqueous extract of the aboveground parts, the sediment of the aqueous extract of the aboveground parts, the aqueous extracts of the belowground parts, and the sediment of the belowground parts of A. inebrians. The results showed that A. inebrians had strong allelopathy. The inhibitory effect of the aboveground parts of A. inebrians on seed germination and seedling growth of E. nutans was stronger than that of aerial parts. The allelochemicals of A. inebrians were mainly distributed in the aboveground parts. The growth of E. nutans was inhibited by increasing activities of antioxidant defense enzymes and membrane peroxidation. In addition, the allelopathy of A. inebrians was stronger in live soil than in sterilized soil. Soil microorganisms effectively improve the allelopathy of A. inebrians.
Acknowledgement: The authors sincerely thank the anonymous reviewers who made valuable comments on this paper.
Funding Statement: This work was supported by the Budgetary Project the Chinese Academy of Sciences Leads the Sub-Project of Class A Project (XDA26020202), the National “973” Program Project Topics (2014CB138702), the Basic Scientific Research Business Expenses of Central Universities (Lzujbky-2022-kb10), the 111 Wisdom Base (B12002), and the Fundamental Research Funds for the Central Public Welfare Research Institutes (Chinese Academy of Forestry) (CAFYBB2021ZD001).
Author Contributions: All authors contributed to the conception and design of this study. Material preparation, data collection, and analyses were performed by Rui Zhang, Taixiang Chen, Zhenjiang Chen, Hao Chen, and Xuekai Wei. The first draft of the manuscript was written by Rui Zhang and all authors commented on previous versions of the manuscript. The manuscript was polished by Malik Kamran. All authors read and approved the final manuscript.
Availability of Data and Materials: The datasets generated and analyzed in the current study are available from the corresponding author upon reasonable request.
Ethics Approval: Not applicable.
Conflicts of Interest: The authors declare no conflict of interest. The funders had no role in the design of the study; in the collection, analyses, or interpretation of data; in the writing of the manuscript; or in the decision to publish the results.
References
1. Zhao B, Liu Z, Wan X. Damage and control of poisonous-weeds in Chinese western grassland. Sci Agric Sin. 2008;10:3094–103 (In Chinese). [Google Scholar]
2. Xu Z, Li X, Zhang L. A bibliometric analysis of research trends and hotspots in alpine grassland degradation on the Qinghai-Tibet Plateau. PeerJ. 2023;11:e16210. [Google Scholar] [PubMed]
3. Zhao ML, Gao XL, Wang J, He XL, Han B. A review of the most economically important poisonous plants to the livestock industry on temperate grasslands of China. J Appl Toxicol. 2013;33(1):9–17. [Google Scholar] [PubMed]
4. Liu Y, Hou W, Jin J, Christensen MJ, Gu L, Cheng C, et al. Epichloe gansuensis increases the tolerance of Achnatherum inebrians to low-P stress by modulating amino acids metabolism and phosphorus utilization efficiency. J Fungi. 2021;7(5):390. [Google Scholar]
5. Liang JJ, Gao GY, Zhong R, Liu BW, Christensen MJ, Ju YW, et al. Effect of Epichloe gansuensis endophyte on seed-borne microbes and seed metabolites in Achnatherum inebrians. Microbiol Spectr. 2023;11(2):e01350–22. [Google Scholar] [PubMed]
6. Yao X, Chen ZJ, Wei XK, Chen SH, White J, Huang X, et al. A toxic grass Achnatherum inebrians serves as a diversity refuge for the soil fungal community in rangelands of Northern China. Plant Soil. 2020;448(1–2):425–38. [Google Scholar]
7. Guo Y, Zhang R, Sun T. Hazards, prevention, control and comprehensive utilization of poisonous weeds in natural grassland in Gansu Province. Acta Agrestia Sin. 2017;25(2):243–56 (In Chinese). [Google Scholar]
8. Sun Z, An S, Li P. Primarily studies on seed germination characteristics of Achnatherum inebriants (Hance) Keng. Seed. 2014;33(1):10–3+8. [Google Scholar]
9. Chen YZ, Xu CL, Ma KK, Hou QQ, Yu XJ. Responses of community traits and soil characteristics of Achnatherum inebrians-type degraded grassland to grazing systems in alpine meadows on the Qinghai-Tibet Plateau. Front Plant Sci. 2023;14:1270304. [Google Scholar] [PubMed]
10. Yao X, Christensen MJ, Bao GS, Zhang CP, Li XZ, Li CJ, et al. A toxic endophyte-infected grass helps reverse degradation and loss of biodiversity of over-grazed grasslands in Northwest China. Sci Rep. 2015;5:18527. [Google Scholar] [PubMed]
11. Saimilakezi T, An SZ, Jin GL, Dong YQ, Yue YH, Han WQ. Biomass characteristics and growth analysis of various components of Achnatherum inebrians (drunken horse grass) population in different habitats. Appl Ecol Environ Res. 2020;18(5):6485–97. [Google Scholar]
12. Xiao H, Wang F, Xu C. Effects of rhizosphere soil of Achnatherum inebrians on seed germination and seedling growth of 6 plant species in alpine meadow. Grassland Turf. 2017;37(1):38–43 (In Chinese). [Google Scholar]
13. Jin G, Zhu X, Wei X. Seed germination and seedling growth of accompanying species. Xinjiang Agric Sci. 2016;53(8):1494–9 (In Chinese). [Google Scholar]
14. Xia C, Zhong R, Zhang X. Allelopathic effects of volatile compounds from endophyte-free and infected Achnatherum inebrians on Lolium perenne. Pratacultural Sci. 2015;32(5):658–66 (In Chinese). [Google Scholar]
15. Lin CC, Liu YT, Chang PH, Hsieh YC, Tzou YM. Inhibition of continuous cropping obstacle of celery by chemically modified biochar: an efficient approach to decrease bioavailability of phenolic allelochemicals. J Environ Manage. 2023;348:119316. [Google Scholar] [PubMed]
16. Bao L, Liu Y, Ding Y, Shang J, Wei Y, Tan Y, et al. Interactions between phenolic acids and microorganisms in rhizospheric soil from continuous cropping of Panax notoginseng. Front Microbiol. 2022;13:791603. [Google Scholar] [PubMed]
17. Hamad AA, Kashaigili JJ, Eckert S, Eschen R, Schaffner U, Mbwambo JR. Impact of invasive Lantana camara on maize and cassava growth in East Usambara. Tanzania Plant-Environ Interact. 2022;3(5):193–202. [Google Scholar] [PubMed]
18. Schandry N, Becker C. Allelopathic plants: models for studying plant-interkingdom interactions. Trends Plant Sci. 2020;25(2):176–85. [Google Scholar] [PubMed]
19. Zhu X, Li W, Shao H, Tang S. Selected aspects of invasive Solidago canadensis with an emphasis on its allelopathic abilities: a review. Chem Biodivers. 2022;19(10):e2022007. [Google Scholar]
20. Xia Z, He Y, Korpelainen H, Niinemets U, Li C. Allelochemicals and soil microorganisms jointly mediate sex-specific belowground interactions in dioecious Populus cathayana. New Phytol. 2023;240(4):1519–33. [Google Scholar] [PubMed]
21. Tabaglio V, Fiorini A, Sterling TMM, Schulz M. Abutilon theophrasti’s resilience against allelochemical-based weed management in sustainable agriculture-due to collection of highly advantageous microorganisms? 2023;12(4):700. [Google Scholar]
22. Xiang W, Wei X, Tang H, Li L, Huang R. Complete genome sequence and biodegradation characteristics of benzoic acid-degrading Bacterium Pseudomonas sp. SCB32. Biomed Res Int. 2020;2020:6146104. doi:10.1155/2020/6146104. [Google Scholar] [PubMed] [CrossRef]
23. Xiao Z, Zou T, Lu S, Xu Z. Soil microorganisms interacting with residue-derived allelochemicals effects on seed germination. Saudi J Biol Sci. 2020;27(4):1057–65. [Google Scholar] [PubMed]
24. Barto EK, Hilker M, Mueller F, Mohney BK, Weidenhamer JD, Rillig MC. The fungal fast lane: common mycorrhizal networks extend bioactive zones of allelochemicals in soils. PLoS One. 2011;6(11):e27195. [Google Scholar] [PubMed]
25. Wang X, Zhang R, Wang J, Di L, Wang H, Sikdar A. The effects of leaf extracts of four tree species on Amygdalus pedunculata seedlings growth. Front Plant Sci. 2021;11:587579. [Google Scholar] [PubMed]
26. Zhang KM, Shen Y, Yang J, Miu X, Bhowmik PC, Zhou X, et al. The defense system for Bidens pilosa root exudate treatments in Pteris multifida gametophyte. Ecotox Environ Safe. 2019;173:203–13. [Google Scholar]
27. Wu B, Shi S, Zhang H, Du Y, Jing F. Study on the key autotoxic substances of alfalfa and their effects. 2023;12(18):3263. [Google Scholar]
28. Sun Q, Hu J. Research technology of plant physiology. Yangling, China: Northwest A&F University Press; 2006. [Google Scholar]
29. Ghani MU, Yuan H, Tian M, Kamran M, Hou F. Aqueous litter extracts of native grass species suppress exotic plant species under allelopathic conditions. J Plant Growth Regul. 2023;42(3):1665–80. [Google Scholar]
30. Otify AM, Mohamed OG, El-Amier YA, Saber FR, Tripathi A, Younis IY. Bioherbicidal Activity and metabolic profiling of allelopathic metabolites of three Cassia species using UPLC-qTOF-MS/MS and molecular networking. Metabolomics. 2023;19(3):16. [Google Scholar] [PubMed]
31. Vashishth DS, Bachheti A, Bachheti RK, Husen A. Allelopathic effect of Callistemon viminalis’s leaves extract on weeds, soil features, and growth performance of wheat and chickpea plants. J Plant Interact. 2023;18(1):2248172. [Google Scholar]
32. Huang X, li CJ, Nan ZB. Effects of Achnatherum inebrians/Neotyphodium endophyte symbiont on seed germination of Stipa capillata and Poa sphondylodes. Pratacultural Sci. 2010;27(7):84–7 (In Chinese). [Google Scholar]
33. Gallego JCA, Caro JG, Campos VH, Lobon NC. Effect of leaf litter from Cistus ladanifer L. on the germination and growth of accompanying shrubland species. Plants. 2020;9(5):593. [Google Scholar] [PubMed]
34. Mishra AK, Sudalaimuthuasari N, Hazzouri KM, Saeed EE, Shah I, Amiri KMA, et al. Tapping into plant-microbiome interactions through the lens of Multi-Omics techniques. Cells. 2022;11(20):3254. [Google Scholar] [PubMed]
35. Deng H, Zhang Y, Liu K, Mao Q, Agathokleous E. Allelopathic effects of eucalyptus extract and wood vinegar on germination and sprouting of rapeseed (Brassica rapa L.). Environ Sci Pollut Res. 2023;12:2838–2016. [Google Scholar]
36. Zhang J, Li J, Gong J, Liu J, Wang Y, Zhao F, et al. A novel highly thermostable and stress resistant ROS scavenging metalloprotein from Paenibacillus. Arch Biochem Biophys. 2024;751:109837. [Google Scholar] [PubMed]
37. Song XW, Yao Y, Yu PC, Zhang W, Liu WF, Wang LY, et al. Sodium nitroprusside improved the quality of Radix Saposhnikoviae through constructed physiological response under ecological stress. Sci Rep. 2023;13(1):15823. [Google Scholar] [PubMed]
38. Lei L, Zhao Y, Shi K, Liu Y, Hu Y, Shao H. Phytotoxic activity of alkaloids in the desert plant Sophora alopecuroides. Toxins. 2021;13(10):706. [Google Scholar] [PubMed]
39. Liu HH, Chong PF, Liu ZH, Bao XG, Tan BB. Exogenous hydrogen sulfide improves salt stress tolerance of Reaumuria soongorica seedlings by regulating active oxygen metabolism. PeerJ. 2023;11:e15881. [Google Scholar] [PubMed]
40. Bashar HMK, Juraimi AS, Ahmad-Hamdani MS, Uddin MK, Asib N, Anwar MP, et al. Determination and quantification of phytochemicals from the leaf extract of Parthenium hysterophorus L. and their physio-biochemical responses to several crop and weed species. Plants. 2022;11(23):3209. [Google Scholar] [PubMed]
41. Wang Y, Zhao Y, Dong B, Wang D, Hao J, Jia X, et al. The aqueous extract of Brassica oleracea L. exerts phytotoxicity by modulating H2O2 and O2- Levels, antioxidant enzyme activity and phytohormone levels. Plants. 2023;12(17):3086. [Google Scholar] [PubMed]
42. Zhang XL, Zhu QQ, Chen CY, Xie B, Tang BG, Fan MH, et al. The growth inhibitory effects and non-targeted metabolomic profiling of Microcystis aeruginosa treated by Scenedesmus sp. Chemosphere. 2023;338:139446. [Google Scholar] [PubMed]
43. Lara-Nuñez A, Romero-Romero T, Ventura JL, Blancas V, Anaya AL, Cruz-Ortega R. Allelochemical stress causes inhibition of growth and oxidative damage in Lycopersicon esculentum Mill. Plant Cell Environ. 2006;29(11):2009–16. [Google Scholar]
44. Li J, Chen L, Chen Q, Miao Y, Peng Z, Huang B, et al. Allelopathic effect of Artemisia argyi on the germination and growth of various weeds. Sci Rep. 2021;11(1):4303. [Google Scholar] [PubMed]
45. Hua Z, Xie Q, Li Y, He M, Wang Y, Wu H, et al. Effects of 13C isotope-labeled allelochemicals on the growth of the invasive plant Alternanthera philoxeroides. Sci Rep. 2023;13(1):13756. [Google Scholar] [PubMed]
46. Wang KL, Wang T, Ren C, Dou PP, Miao ZZ, Liu XQ, et al. Aqueous extracts of three herbs allelopathically inhibit Lettuce Germination but promote seedling growth at low concentrations. Plants. 2022;11(4):486. [Google Scholar] [PubMed]
47. Liu Y, Wang H, Qian X, Gu J, Chen W, Shen X, et al. Metagenomics insights into responses of rhizobacteria and their alleviation role in licorice allelopathy. Microbiome. 2023;11(1):109. [Google Scholar] [PubMed]
48. Bhattacharyya PN, Islam NF, Sarma B, Nath BC, Al-Ani LKT, Lesueur D. Frankia-actinorhizal symbiosis: a non-chemical biological assemblage for enhanced plant growth, nodulation and reclamation of degraded soils. Symbiosis. 2023. doi:10.1007/s13199-023-00956-2. [Google Scholar] [CrossRef]
49. Facenda G, Real M, Galan-Perez JA, Gamiz B, Celis R. Soil effects on the bioactivity of hydroxycoumarins as plant allelochemicals. Plants. 2023;2(6):1728. [Google Scholar]
50. Wang CM, Li TC, Jhan YL, Weng JH, Chou CH. The impact of microbial biotransformation of catechin in enhancing the allelopathic effects of Rhododendron formosanum. PLoS One. 2013;8(12):e85162. [Google Scholar] [PubMed]
51. Bonanomi G, Zotti M, Idbella M, Mazzoleni S, Abd-ElGawad AM. Microbiota modulation of allelopathy depends on litter chemistry: mitigation or exacerbation? Sci Total Environ. 2021;776:145942. [Google Scholar] [PubMed]
52. David AS, Thapa-Magar KB, Afkhami ME. Microbial mitigation-exacerbation continuum: a novel framework for microbiome effects on hosts in the face of stress. Ecol. 2018;99(3):517–23. doi:10.1002/ecy.2153. [Google Scholar] [PubMed] [CrossRef]
Cite This Article
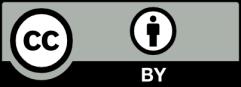
This work is licensed under a Creative Commons Attribution 4.0 International License , which permits unrestricted use, distribution, and reproduction in any medium, provided the original work is properly cited.