Open Access
REVIEW
Plant Nitrogen Metabolism: Balancing Resilience to Nutritional Stress and Abiotic Challenges
1 Department of Plant Pathology, Faculty of Agricultural Sciences & Technology, Bahauddin Zakariya University, Multan, 60800, Pakistan
2 Centro de Investigación de Estudios Avanzados del Maule (CIEAM), Vicerrectoría de Investigación y Postgrado, Universidad Católica del Maule, Talca, 3460000, Chile
3 College of Food Science and Nutritional Engineering, China Agricultural University, Beijing, China
4 Department of Biology, College of Science, Taif University, P.O. Box 11099, Taif, 21944, Saudi Arabia
5 Department of Biotechnology, Faculty of Biological Sciences, Lahore University of Biological and Applied Sciences, Lahore, Pakistan
6 Department of Plant Pathology, College of Agriculture, University of Sargodha, Sargodha, 40100, Pakistan
7 Department of Agriculture and Food Technology, Karakorum International University, Gilgit, Pakistan
8 Department of Biology, Faculty of Science, King Khalid University, Abha, Saudi Arabia
* Corresponding Authors: Syed Atif Hasan Naqvi. Email: ; Yasir Iftikhar. Email:
# These authors have contributed equally to this work
(This article belongs to the Special Issue: Abiotic and Biotic Stress Tolerance in Crop)
Phyton-International Journal of Experimental Botany 2024, 93(3), 581-609. https://doi.org/10.32604/phyton.2024.046857
Received 17 October 2023; Accepted 13 February 2024; Issue published 28 March 2024
Abstract
Plant growth and resilience to abiotic stresses, such as soil salinity and drought, depend intricately on nitrogen metabolism. This review explores nitrogen’s regulatory role in plant responses to these challenges, unveiling a dynamic interplay between nitrogen availability and abiotic stress. In the context of soil salinity, a nuanced relationship emerges, featuring both antagonistic and synergistic interactions between salinity and nitrogen levels. Salinity-induced chlorophyll depletion in plants can be alleviated by optimal nitrogen supplementation; however, excessive nitrogen can exacerbate salinity stress. We delve into the complexities of this interaction and its agricultural implications. Nitrogen, a vital element within essential plant structures like chloroplasts, elicits diverse responses based on its availability. This review comprehensively examines manifestations of nitrogen deficiency and toxicity across various crop types, including cereals, vegetables, legumes, and fruits. Furthermore, we explore the broader consequences of nitrogen products, such as N2O, NO2, and ammonia, on human health. Understanding the intricate relationship between nitrogen and salinity, especially chloride accumulation in nitrate-fed plants and sodium buildup in ammonium-fed plants, is pivotal for optimizing crop nitrogen management. However, prudent nitrogen use is essential, as overapplication can exacerbate nitrogen-related issues. Nitrogen Use Efficiency (NUE) is of paramount importance in addressing salinity challenges and enhancing sustainable crop productivity. Achieving this goal requires advancements in crop varieties with efficient nitrogen utilization, precise timing and placement of nitrogen fertilizer application, and thoughtful nitrogen source selection to mitigate losses, particularly urea-based fertilizer volatilization. This review article delves into the multifaceted world of plant nitrogen metabolism and its pivotal role in enabling plant resilience to nutritional stress and abiotic challenges. It offers insights into future directions for sustainable agriculture.
Keywords
Nitrogen, represented by the chemical symbol N, plays a vital role in sustaining life on Earth. It is indispensable for plant health as it enables the production of amino acids, the building blocks of proteins. Proteins, in turn, are pivotal for promoting robust plant growth. Additionally, nitrogen is a fundamental component of DNA, RNA, and nucleic acids, essential for all living organisms. When nitrogen is deficient in the soil, plants suffer from stunted growth due to their inability to synthesize essential proteins and maintain critical nucleic acid structures [1,2]. Nitrogen plays a crucial role in plant growth and is a cornerstone of global food production. Research has indicated that nitrogen is the primary factor influencing genome size, with phosphorus being of lesser significance in this context [3]. The efficient use of nitrogen is responsible for an increase in root length, diameter, branching, and biomass content in the roots. As the root network spreads to a larger area, water absorption is also improved [4]. The concentration of nitrogen in the atmosphere is about 78%, but unfortunately, plants cannot use this nitrogen directly. First, this nitrogen is fixed through fertilizer manufacturing or by microorganisms. Nitrogen is also present in the soil, but 98% of it is organic nitrogen that plants cannot uptake [5]. The cycle of interconversion between inert Nitrogen (N2) and reactive nitrogen (that plants can uptake and is responsible for cellular life) is known as the Nitrogen cycle. Before 1909, nitrogen was naturally fixed; however, after that, the Haber-Bosch process was invented for artificial nitrogen fixation on an industrial scale [6]. Nitrogen for plants is available in various forms, such as nitrate and ammonium (in inorganic form), urea, free amino acids, and short peptides (in organic forms); however, urea must undergo hydrolysis to transform into ammonium nitrogen before becoming accessible for crop utilization [7]. Nitrogen is responsible for chlorophyll production, which increases the rate of photosynthesis, gives plants greening, and increases crop quality and yield. Additionally, it enhances plant resistance to abiotic stresses by promoting protein synthesis, enzyme activation for stress response, and osmotic regulation [8]. Salinity is one of the major abiotic stresses that can be mitigated by applying appropriate amounts of nitrogen, which helps regulate ion balance, alleviate osmotic stress, and reduce competition for water. For example, 12 g/kg of soil proved to be effective in enhancing salinity tolerance in sorghum crops [9]. China, India, the USA, Pakistan, Iran, Egypt, Thailand, Australia, Argentina, and South Africa are among the countries where salinity poses a significant problem for crop productivity. Additionally, salinity is a concern in other countries such as Chile, Turkey, Syria, Algeria, Tunisia, Sudan, and the Gulf states. In the case of Iran, the decline in wheat yield occurred first, followed by a decline in barley yield due to salinization [10]. The influence of salinity on nitrogen availability in soils significantly affects the crop, leading to symptoms of chlorosis, stunted growth, discoloration, and necrosis of older leaves in severe cases. Many crops also exhibit curled small leaves and grains with low protein content [11,12]. Two of the processes including leaching (loss of Nitrogen with the flowing water) and denitrification (reduction of nitrogen from nitrite to gaseous nitrogen due to microbial activity) are losing nitrogen from soil [5]. Over the past three decades, the use of Nitrogen has increased as it is an essential nutrient for plant growth, development, and yield [13].
The use of Nitrogenous fertilizers has increased by 48% since 2008 increasing food production [2]. More use of nitrogenous fertilizers has increased the crop yield but is also polluting the environment [14]. Excessive application of Nitrogen also causes yield losses due to soil acidification. It also decreases the available content of potassium and phosphorus [13]. Agricultural practices (mainly fertilizer applications) have enhanced plant growth by increasing reactive nitrogen compounds (ammonia, nitrate, and nitrite), but this enhancement is harmful to the ecosystem. Additionally, climate changes alter the transport, availability, and timing of nitrogen-related processes, including nitrogen fixation, nitrification, and denitrification [15]. Excessive nitrate due to synthetic fertilizers is the second chemical threat to the surface and groundwater [16,17]. In water, nitrogen exists in four forms nitrate, ammonia, ammonium, and nitrite, but nitrate is the most toxic of all. Increasing levels of nitrate in water is becoming fatal for aquatic life and human beings because it causes cancer and blue-baby syndrome in adults and infants respectively due to the reaction with protein [17,18]. The excessive use of nitrogen is the primary cause of salinity and the aforementioned problems, making it a major issue for discussion. The harmonization of agronomic, genetic, and biotechnological tools will enhance nitrogen assimilation efficiency in crops, aligning agricultural systems with global needs to preserve environmental functions and resources [19]. A small amount of copper is also required for good utilization of Nitrogen by the plants from the soil [20]. The preservation of a high GSH/GSSG ratio, which is essential for DHAR (dehydroascorbate reductase) and other GSH-dependent enzymes engaged in antioxidant defense mechanisms, was also aided by increases in NO-mediated GR (glutathione reductase) activity during salinity stress [21]. Applications of nanoparticles (Nano-fertilizers) have demonstrated significant potential in enhancing plants’ ability to withstand environmental challenges, such as drought, high and low temperatures, and salinity, by modulating the physiological, biochemical, and molecular processes of plants [22]. Therefore, this review summarizes the literature on problems created by the excessive use of N, its relation with salinity, and different approaches for improving NUE.
Water evaporates from desert playas, which are flat, arid regions located at the base of undrained desert basins. These areas are covered with water in spring, leading to an increase in salt concentrations from 7000 to 14000 mM NaCl [23]. This salinity is an important abiotic stress factor in the arid and semi-arid areas of the world due to its effect on the productivity of different crops by suppressing photosynthesis. In this case, the concentration of sodium ions is increased while that of potassium ions is decreased [24]. Currently, 20% of the global land area is affected by salinity. It is believed that 30% of agricultural land could be lost within the next 25 years due to salt stress, with a total loss that could reach 50% by 2050. According to the geography and environmental conditions, different types of soluble salts are found in different fields including sodium sulfate and sodium chloride [9]. Depending on the nitrogen source, salinity has a variable impact on the intake of several nutrients like plants becoming more sensitive when ammonia is used as a source of fertilizer. Main cereal crops including rice, wheat, maize, and sorghum, and similarly important cash crops like sugarcane and cotton are greatly affected by salinity. Molecular studies showed that there are three ways by which salts suppress the growth of plants. First is the osmotic stress (water is drawn out from the cell due to higher salts conc.) that increases the concentrations of plant toxins (ion toxicity due to accumulation of Na+ and Cl− ions), second includes ionic stresses and the third one is the oxidative stress carried out by Reactive Oxygen Species (ROS). Photosynthetic activity is disturbed by the imbalance of ions, imbalance of nutrients, and hormonal imbalance while the most important is the disturbance in water uptake caused by salinity [25]. Salinity stress results in severe oxidative damage, MG toxicity, cell death, and nuclear damage, which significantly decreases the growth of the seedlings [26]. Strongly salinized soils have a lower capacity to absorb CH4 than moderately salinized soils do. According to one of the studies, CH4 uptake was limited in severely salinized soil. This was probably due to the poor activity of Methylocella spp.; the most prevalent methanotrophs, and the fact that few of them were even able to oxidase methane in these soils. The inorganic nitrogen content of soil decreases with increasing salinity [27]. Nitrogen fertilization promotes N2O emission from saline soils because of large quantities of nitrogen present as a substrate for soil nitrification and denitrification, thereby facilitating N2O emission [28]. The relation between salinity and different doses of Nitrogen was checked in an experiment on Crocus sativus L. According to that the negative impact of salinity was mitigated by 50 kg N/ha while 100 kg of N/ha enhanced the negative impact of the crop. The negative impact of salinity can be assessed by the number of leaves, length of leaves, weight of dry shoots, number of corms, and weight of corms [29].
The Sun is the sole source of energy for life on Earth. Sunlight, specifically blue and red light, is captured by chlorophyll in plants, algae, and Cyanobacteria. Through photosynthesis, these organisms convert sunlight, carbon dioxide, and water into chemical energy, producing glucose as the final product. Chlorophyll is found in the green parts of plants within the thylakoid membrane of the chloroplast. In the structure of chlorophyll, four nitrogen atoms surround the magnesium ion, forming a complex structure. This is often referred to as a porphyrin ring or tetrapyrrole ring [30]. The chlorophyll content is dependent on the chloroplast structure, which is influenced by the availability of nitrogen for the plants. When the effect of nitrogen was examined on maize crops using a low level of nitrogen fertilizer, it resulted in a reduction in the photosynthetic area and the chlorophyll content [31]. The main element to control the dynamics of various ecosystems on Earth is Nitrogen [32]. Wheat uses Nitrogen in greater quantity as compared to the other nutrients and due to its deficiency, the surface area of the leaf and the photosynthetic area is decreased, and the plant’s green color is reduced [33]. When a proper amount of fertilizer was applied (138 kg/ha) it increased the pigment PS II and photosynthetic area of the leaf [2]. In the case of sugar beet, the highest content of chlorophyll and highest productivity was obtained when the application of Nitrogen was 120 kg/ha [34]. High nitrogen concentration increases potassium, phosphorus, iron, calcium, magnesium, and copper uptake in plants by promoting root growth, altering PH, stimulating microbial activity, and increasing the solubility of these fertilizers. Since 1980, due to the use of Nitrogenous fertilizers in China 70% increase in grain has been recorded [35]. Nitrogen is also responsible for the structure and composition of cell walls [36]. In the case of Oats Nitrogen is also responsible for reproduction and vegetative growth [37]. In saline soils, the application of nitrogen increased the concentration of chlorophyll, thereby enhancing photosynthesis and yield in oat crops. The augmentation in grain concentration (amount or density of grains in crops like cereals) was attributed to the alleviating effect on salinity through the use of nitrogen [38]. According to Jiang et al. [39] soil salinity decreases by increasing the Nitrogen concentration up to a limit while excessive (more the recommended dose, which is different for different crops) application of Nitrogen promotes Salinity [39]. The use of nitrogen fertilizers appeared to have a beneficial effect on reducing alfalfa’s salt stress and preserving the production of shoot biomass, but N fertilization may restrict the plant’s capacity to fix atmospheric N [40]. N fertilizer was successful in alleviating the adverse impacts of NaCl and Na2SO4 in Sorghum when the applied concentration of Nitrogen was 12 g N kg−1 of soil [9].
3.2 Nitrogen’s Dual Nature: Essential Nutrient and Industrial Marvel
Humans are incapable of directly utilizing elemental nitrogen and must absorb alternative forms such as NO2− and NO3−, with NO3− being obtainable from green plants as well as inadvertent ingestion of contaminated water [41]. The protein structure encompasses 16% nitrogen, while certain neurotransmitters and peptide hormones are composed of amino acids (building blocks of protein) [42]. Carbon, Phosphorus, and Nitrogen are the building blocks of DNA and RNA. Five nitrogen’s are present in pyrimidine and three nitrogen’s in purine are the main nucleotides [43]. Children need higher protein (35%) for growth, while adults require 15%. Understanding metabolism, intake, and health effects is crucial because of increasing Nitrogen demand [41]. In adults, the body maintains a nitrogen balance, with protein neither stored nor accumulated. However, during growth, reproduction, illness, or injury, the body’s nitrogen demand increases, resulting in a positive balance. Conversely, if protein utilization exceeds intake or catabolism outpaces consumption, a Negative Nitrogen Balance occurs. Nitric oxide acts as a crucial messenger molecule, facilitating physiological functions like blood vessel dilation, platelet activation, and immune modulation. Proteins provide 2%–5% of the body’s energy, equivalent to 4 calories per gram, akin to carbohydrates [41]. During the first month, human milk contains 1.3 grams of proteins per 100 ml and this quantity goes to 1.1 grams per 100 ml after the first month [42]. Sometimes NO3− is converted into NO2− that can cross the placental barrier and can cause the death of the fetus [41]. In Earth’s troposphere, N2O serves as the primary source of O3 while nitrogen and argon were initially used in lamps to prevent blackening, however, nitrogen lamps offer color to light, enhance filament brilliance, and maintain consistent characteristics [44,45]. There are many other uses of Nitrogen as it is used in the pharmaceutical industries for the preservation of Blood, eggs, and sperm. Similarly, Nitrous oxide can be used as an anesthetic. In the process of metal production, Nitrogen is an important component that prevents oxidation. It can also be used as a blanketing agent. It also has applications in the food industry as it is used for freezing and cooling food due to its property of low temperature. It also reduces the damage to food and helps the food to transfer over long distances. Nitrogen is also used to remove unstable organic chemicals from the reaction [32] (Fig. 1).
Figure 1: Some common uses of nitrogen in different fields
4 Nitrogen Cycle and Fixation (Biological, Industrial, Abiotic and Lightening)
The number of major pools of Nitrogen on this earth are two which first one being the atmosphere where Nitrogen exists in molecular form (N2) while the second one is the reactive nitrogen pool that exists in biological molecules. The interconversion of Nitrogen between these two pools is known as the Nitrogen cycle [46]. Five major steps are involved in the Nitrogen Cycle that includes Nitrogen Fixation, Mineralization, Immobilization, Nitrification, and Denitrification [47]. The process of conversion of molecular Nitrogen into Biological Nitrogen is known as Nitrogen Fixation [48,49]. Nitrogen fixation is carried out by symbiotic and free-living bacteria; it can also be fixed artificially through lightning [50]. Biological nitrogen fixation is carried out by prokaryotes that convert atmospheric nitrogen molecules into ammonia with the help of the nitrogenase enzyme. Beijerinck discovered this process in 1901. Among these prokaryotes, some include free-living bacteria like Azotobacter, which inhabits soil, Cyanobacteria found in water, as well as Azospirillum and Rhizobium, which form symbiotic associations with legumes [51]. Nitrogenase enzyme contains molybdenum, iron, and vanadium [52]. These bacteria that are involved in nitrogen fixation are named diazotrophs [53]. The nitrogen molecule is composed of two nitrogen atoms joined by a triple covalent bond, Nitrogenase catalyzes the breaking of this bond and the addition of three hydrogen atoms to each nitrogen atom. Since the Nitrogenase enzyme can only function in an anaerobic environment, Leg Hb is a pigment found in leguminous plants. It serves as a scavenger of oxygen. For Nitrogenase to function, it exhausts all the oxygen and generates an anaerobic environment. Nitrogen is fixed using hydrogen donors. Numerous substances, including pyruvic acid, glucose, sucrose, etc.; are hydrogen donors. Energy is obtained by the utilization of ATP. The molecules of generated ammonia must be bound by another carbon-containing chemical [54]. In the center of each mature nodule, there are billions of bacteria present that fix nitrogen [55]. The white or grey color of nodules represents that nodules are young and do not fix the nitrogen. If the color of nodules is pink or reddish it means they are fixing the Nitrogen. Old nodules that are not fixing the nitrogen their color become green [56]. Cyanobacteria may form a relation to the roots of plants intracellularly or intercellularly [57]. Heterotrophic and Cyanobacterial nitrogen fixation depends upon the quality of carbon and the availability of micronutrients respectively and the rate of nitrogen fixation on land is decreased due to a low supply of phosphorus [58]. In non-symbiotic associations, bacteria release nitrogen through the breakdown of bacterial cells, utilizing carbon and energy from the environment for nitrogen fixation. Energy from plant roots, in the form of carbohydrates, fuels this process. Factors influencing biological nitrogen fixation include tillage, acidic soils, intercropping, fungicides, pesticides, insecticides, nitrogen fertilization, temperature, and moisture. Among free-living heterotrophs, aerobic organisms like Methanosarcina and Clostridium exist, while heterotrophic microaerophiles include Frankia, Azospirillum, and Bradyrhizobium, and aerobic heterotrophic free-living organisms include Azobacter and Derxia. Root-associated species comprise microaerophilic Herbaspirillium and endophytic Acetobacter, with Frankia and Bradyrhizobium in symbiotic association. Free-living autotrophs include microaerophilic organisms like Rhodospirillum and Bradyrhizobium, aerobic free-living autotrophs like Cyanobacteria, and symbiotic autotrophs such as Anabaena azalea and cyanobacteria [59,60]. Atmospheric nitrogen can be fixed through lightning, where nitrogen and oxygen combine to form NO, which then reacts with rainwater to produce nitrous acid or nitric acid, subsequently converted into nitrates for plant uptake [61,62] (Fig. 2). Many chemical reactions were conducted to combine Nitrogen and Hydrogen after the detection of ammonia in the chemical lab of the Margulies brothers. Haber decided to synthesize ammonia by using heat and iron as a catalyst [63]. In 1909 he forcefully combined the nitrogen with hydrogen at a high temperature of 500°C and high pressure of about 150–200 atm [64]. Haber thought that this reaction would be reversible and then he detected the movement of ammonia, H, and N to opposite sides when he experimented with normal atmospheric pressure [63]. For the production of ammonia, 2% of the world’s natural gas is being used as a source of hydrogen and it uses 1%–2% of the world’s energy sources. Annually 140 million tons of Nitrogen are being fixed with the help of Haber Bosch process [63]. The Chemical equation for synthetic Nitrogen fixation is given below:
Figure 2: Comparison between BNF, artificial nitrogen fixation and nitrogen fixation through lightening
In the case of abiotic nitrogen fixation in soil, 10 T g of Nitrogen can be fixed per year in the desert through photochemical fixation. In photochemical Nitrogen Fixation, organic nitrogen is used to generate ammonium and sometimes it supplies more than half of the bioavailable nitrogen in the ecosystem. Photochemical Nitrogen fixation is important because sometimes microorganisms are unable to decompose organic Nitrogen into ammonium but it can be fixed by sunlight [65]. The second step of the Nitrogen cycle in which ammonium is oxidized into nitrite is known as Nitrification. Nitrite ions are further oxidized to nitrate ions by other nitrifying bacteria like Nitrobacter. Both nitrite and nitrate are forms of nitrogen that plants can readily absorb [66,67]. The last step of the Nitrogen cycle includes the conversion of nitrate into molecular Nitrogen and the activity is carried out by microorganisms [68]. Among different communities of plants, the measurements of denitrification differ [69] (Fig. 2).
5 What is Nitrogen Use Efficiency, Importance and Improvements
As nitrogen is a crucial factor in crop production, its excessive application not only boosts plant growth and yield but also escalates production costs and contributes to environmental degradation, underscoring the necessity for users to grasp nitrogen use efficiency and cultivate varieties with improved nitrogen use efficiency. Nitrogen use efficiency is commonly employed to estimate the conversion of nitrogen inputs into agricultural products and to gauge the risk of nitrogen losses to the broader environment, determined by factors such as nitrogen uptake, mobilization, and assimilation, with only 30%–50% of applied nitrogen being utilized by plants, while the remainder is wasted [5]. Agronomists and genetic researchers propose two different definitions of NUE. According to agronomists, NUE is the relation between available Nitrogen for the plant or Nitrogen uptake by plants and the generation of grains per Unit of Nitrogen. According to genetic researchers, NUE relates to the number of genes like glutamine synthetize, to are important for better Nitrogen Use Efficiency [70]. When the roots of many plants take up nitrate, some of it is assimilated into the roots, but the majority is transported to the shoot, where it is first reduced to nitrite by nitrate reductase in the cytoplasm. Then further converted to ammonium by nitrite reductase in the plastids and glutamine synthetase (GS) in the plastids and cytoplasm. This thing makes glutamine important for enhancing NUE [71]. By altering the expression of genes for nitrate transport, the Indica subspecies of rice was genetically improved for better nitrogen use efficiency (NUE) through increased absorption of nitrates. In China, the NUE of the rice cultivar Wuyunjing 7 was enhanced through the same genetic changes [72,73]. It can also be defined as determining the amount of Nitrogen stored by plants or released into the atmosphere in the form of nitrous oxide. NUE of leguminous crops is better because they store maximum Nitrogen inside them, while it is not completely known whether they do not release Nitrogen into the atmosphere or release it in very small amounts. Overall, Nitrogen use efficiency is the amount of Nitrogen available for plants how much of that is used by plants, and how much Nitrogen they retain at the time of harvest [74]. Nitrogen use efficiency is the product of utilizing the efficiency of Nitrogen and the uptake efficiency of Nitrogen also including remobilization and assimilation efficiencies of Nitrogen. Nitrogen uptake efficiency depends upon the amount of ammonium and nitrate present in soil and the ability of plant cells to store them [75].
In the case of cereal crops, the worldwide nitrogen use efficiency remained at 30%. Improvements in NUE can control the use of high amounts of Nitrogen. According to an estimate about England fields, through better crop management NUE can extend but will remain below 75%. The was a difference in Nitrogen use efficiency when both ammonium and nitrates were present as compared to when only nitrate was present. In the case of High ammonium NUE was found to be lower [70]. The Nitrogen Use efficiency in China is lower as compared to the developed countries that is 10%–20% [76]. It has been found that microbes show high NUE even when there is less Nitrogen [77]. During the last years, 47 Mt of Nitrogen was applied to cereal crops out of a total of 83 Mt, Southeast Asia, and North East Asia used 71% and 32% of that Nitrogen, respectively [78]. Recovery of Nitrogen depends upon the crop type, fertilizer type, cultural practices, and environmental factors [79]. NUE is lower when farmers use conventional methods to grow their crops. It is also lowered when there is poor coordination among plant requirements and the time of application (mostly applied earlier that is lost due to the temperature or any other abiotic stress) [74]. NUE is also decreased due to the balance in the nutrient’s applications [80].
NUE can be improved using fertilizers with a slow-release process [81]. NUE has been reported to improve in Pakistan using potassium fertilizer [80]. Improvements in cultural practices can improve NUE [82]. Nitrogen Use Efficiency depends upon translocation of Nitrogen in sensing leaves, and it can be improved by Mycorrhizal fungi [83]. NUE can also be improved by using genetically improved verities [78] Urea is the most abundantly used fertilizer worldwide and for its better use efficiency, it is coated with Sulphur. Nitrogen use efficiency can also be improved by using a Urea-Triazone solution as an alternative to granular urea as it dissolves or releases nitrogen slowly [84]. Application of water and nutrients directly to the rhizosphere of plants improves the NUE [81]. Broad and healthy rhizosphere also have higher NUE as experimented in rice. We can prevent the environment by using varieties with high NUE by studying their genetic makeup [76]. Different tillage techniques have varying degrees of soil disturbance, which impacts soil N dynamics and plant N availability. The conservation tillage (less or no tillage) approach frequently results in higher NUE overall, however, the link between the two varies throughout the studies [85]. Cotton yields were higher in conservation tillage plots than in conventional tillage plots with an optimal application of nitrogen, according to a lengthy (10-year) study done in the southern United States of America [86]. Slow-release nitrogen fertilizers may reduce nitrogen leaching and denitrification losses and enhance the timing of nitrogen release and uptake in response to crop needs. Similarly, slow-release synthetic urea-based fertilizers like isobutylidene diurea (IBDU) and crotobylidene diurea (CDU), which are coated N sources, have also enhanced the NUE. When compared to broadcasting, N fertilizer injection, band application, and placement under the seeds during planting all resulted in higher NUE and lower NH3 volatilization in winter wheat. Above-discussed factors to enhance NUE play a vital role in sustainable agriculture by reducing pollution and enhancing crop productivity. For NUE, the plant’s intrinsic capability must be enhanced and chosen to promote effective uptake, utilization of N, and production of higher yield under conditions of moderate or minimal N availability [19]. In an experiment conducted in India nanotechnology was used for the efficient application of Nitrogen and Zinc along with the practice of organic forming [87]. The loading of Urease enzymes upon the fertilizers limits the release of Nitrogen from urea via hydrolysis. These enzyme-loaded fertilizers (nano fertilizers) are termed nanoparticles and the technology used to make these particles is called nanotechnology [88]. In nanotechnology, fertilizers are used in small amounts, and during the experiments, a great increase in yield was recorded (Table 1).
6 Symptoms of Nitrogen Deficiency and Excess
The content of chlorophyll is reduced, and degradation of enzymes occurs due to the reduced level of nitrogen in plants. As degradation of protein leads to a change in the enzyme activity, which causes the leaf senescence. The level of Rubisco activase, endase, ATP synthesis, glutamine synthesis, etc. also reduced due to the reduced level of nitrogen. At the initial stage of senescence, the level of mRNA increases, and it becomes very low when leaves become completely senescent [92]. The degradation of chlorophyll, leading to leaf yellowing, contributes to the decline in crop plant growth, primarily due to low nitrogen levels, affecting overall crop yield [93]. This nitrogen deficiency reduces cell division, initially manifesting as lower leaf yellowing, potentially leading to leaf drop in severe cases, and decreases seed protein content. Furthermore, nitrogen deficiency induces early maturity, further reducing yield [93,94]. During vegetative growth, the surface area of the leaf is reduced [95]. Low fertilization rates may result in seed yields that fall short of expectations [96]. Reduction in yield of seed yield was measured up to 30%. In the case of photosynthetic machinery both chlorophyll inflorescence and chlorophyll concentration were found to be reduced due to a deficiency of Nitrogen [97] (Fig. 1). The primary metabolism of plants is reduced due to the deficiency of Nitrogen in the soil. Due to less availability of Nitrogen, the rate of transpiration and photosynthesis is reduced [98]. Due to this plant growth is affected [82]. When there was a low level of Nitrogen in Arabidopsis the flowering was promoted in it [98]. At the heading and seedling stage, the deficiency of Nitrogen does not have much effect on yield while yield losses are maximum when the plant is deficient with Nitrogen at the tillering stage [99].
A higher concentration of nitrogen than required causes problems in plants and disturbs our environment. It affects the growth, development, and composition of plants, and seed quality is affected. Using higher amounts of nitrogen limits the content of phenolic and flavonoids, reducing antioxidant power [100]. The excessive use of nitrate increases plant biomass, but the excessive application of ammonium shows a negative impact on plant growth [101]. Nitrogen toxicity causes leaves to turn dark green, extending the period of vegetative growth. The height of the plant increases while the stem remains thin, eventually leading to lodging. The water use efficiency of the plant decreases due to nitrogen toxicity, resulting in the burning of leaves and stunted growth. Lesions form on leaves, stems, and roots, and the leaves also exhibit downward rolling [12]. The breakdown of particles and formation of harmful chemicals named carcinogens and allergens are due to Nitrogen toxicity. Disease attacks become more by the use of higher amounts of Nitrogen (Fig. 3) [101].
Figure 3: Response of plants at different concentrations of applied Nitrogen in the ecosystem
7.1 Nitrogen Use Efficiency of Cereals
The maximum cultivable land in the world is under the cultivation of cereal crops. Additionally, approximately 90% of cereal production comes from wheat, rice, and maize. The yearly application of nitrogen to cereal crops is 94 million tons, of which 60% is wasted, posing a threat to the environment. Nitrogen Use Efficiency (NUE) in cereal crops depends on two things: how the plant uptakes nitrogen and how much of it can be used. NUE can also be calculated at the time of harvest. Moreover, the higher application of nitrogen decreases NUE. It can be enhanced by the availability of other micro and macronutrients and by crop rotation. The use of nano-fertilizers can also increase NUE [79]. Agronomic practices like time of application, place, and proper amount of fertilizer enhance the NUE [102].
7.2 Wheat, Symptoms of Nitrogen Deficiency and Excess
Wheat provides humans with a lot of carbohydrates and proteins (10%–15%) that are useful for human health, so it is one of the vital staple crops. Due to the plantation of new industries and the movement of people toward the cities, the demand for this staple food is being increased. Dietary fibers are also present in wheat along with Vitamin B [103]. Older leaves first show the stress of nitrogen as their color changes from green to light green and finally, it turns yellow due to the shortage of chlorophyll. The whole field gives a yellowish appearance due to nitrogen stress [104]. Due to the deficiency of nitrogen in wheat the synthesis of chloroplast, in which the photosynthesizing pigment chlorophyll is present, is reduced which directly affects the process of photosynthesis. The physiology of the plant is disturbed as plant growth is stunted, the size of the leaf is reduced which decreases the area of photosynthesis, and the yield of a crop is reduced. Grain quality is also disturbed as the content of protein and amino acids is reduced [105]. When nitrogen supply increases the demand for nitrogen for wheat crops, the number of spikes and spikelet’s is reduced. weight of grain is also reduced. NUE of the crop is reduced and reproductive growth becomes poor which results in yield loss [106]. The toxicity of nitrogen breaks the resistance of the wheat plants and the plant show excessive vegetative growth. Weed problems are more common in such types of fields [107].
7.3 Rice, Symptoms of Nitrogen Deficiency and Excess
Rice is one of the three important food crops. In terms of food, about half of the world’s population depends on rice. Regarding cultivation area, rice ranks second. Ninety percent of the world’s rice is produced in Asia. If we include the cultivation in Latin America and Africa along with Asia, these three regions together account for 98% of the world’s rice production [108]. The deficiency of nitrogen in rice occurs when demands for nitrogen increase like at the tillering stage. In addition, older leaves start changing their color from the tip resulting in yellowing of the whole leaf. Moreover, under severe conditions of N deficiency leaves may be died. The size of leaves is also affected as they become smaller with short length and width disturbing the area of photosynthesis and color becoming yellowish [109]. Under nitrogen toxicity, the greenery of rice plants is increased which attracts the insects and increases the disease attack. At the stage of maturity lodging of the plant occurs, and leaves become folded along with patches on them [110]. Plants show stunted growth due to the lack of photosynthesis which is dependent on the content of chlorophyll. Chlorophyll is present in the chloroplast, whose structure is destroyed in rice plants due to the toxicity of nitrogen [111].
8 Maize, Symptoms of Nitrogen Deficiency and Excess
Worldwide cultivation of maize comes at number three after Rice and Wheat [112]. The Queen of cereals needs a short span for its growth. It can be used as food for humans and to feed the animals as a feeder crop [113]. It can be used in different ways by making its flour that is then used to make bakery products, it can be eaten after roasting, and its fructose level is high and provides a lot of amino acids [112]. Maize provides us with many nutrients as described below. Symptoms appear by the yellowing of older leaves showing necrosis at the tip and the color of other nitrogen-deficient leaves becomes pale green [114]. Nitrogen is the component of cell protoplast, which plays an important role in plant growth, so nitrogen stress retarded the growth of plants resulting in yield losses. Number of barren plants also increased [115]. Due to the nitrogen deficiency, the shoot biomass of the plant is also affected [116]. In an experiment of spectral and true color response, the maize leaves were tested in which nitrogen-deficient leaves showed high spectral reflection and it was found that it was due to the lack of chlorophyll [117]. Nutritional deficiency limits the growth of scarlet eggplant by altering the absorption and use efficiency of macronutrients, leading to reduced plant height, stem diameter, leaf number, and leaf area, as well as decreased dry matter production in both above and below ground biomass. Nitrogen deficiency hinders cell division, affects cell elongation in later leaf development, and reduces the production of photosynthetic pigments. This, in turn, impacts the absorption of light and the metabolism of magnesium, essential for chlorophyll molecules. Additionally, nitrogen deficiency decreases the rate of transpiration and the efficiency of absorbing and using other nutrients. Moreover, excessive nitrogen use lowers the soil pH, which can impede crop growth and reduce yield [118–120].
9 Barley, Symptoms of Nitrogen Deficiency and Toxicity in Barley
As nitrogen is important in the production of barley, the plant is disturbed due to the deficiency of nitrogen. Symptoms of nitrogen stress first appear on older leaves, which start changing their color from the tip. The color of the lower leaves becomes light green. Under continuous stress, the color of other leaves starts diverting from green, while the pale green color of older leaves changes to white. Finally, it results in a reduction in protein levels and also in yield [121]. Due to nitrogen stress, a 50% reduction in photosynthesis has been observed in barley. It also reduces the shoot growth. Although the growth rate of plants becomes slow the level of starch proteins and amino acids is increased due to nitrogen stress. The size of leaves has been observed smaller as compared to the nitrogen-sufficient plants [122]. The toxicity of nitrogen in plants shows effects on the growth of plants and the pathogenic and non-pathogenic stresses on plants are increased [123]. Due to excessive use of ammonia yellowing of leaves occurs and in highly affected verities yield may decrease up to 60%. Sometimes it can be fatal for the plants. Roots and shoots may also lower [124].
10 Sorghum, Symptoms of Nitrogen Deficiency and Toxicity in Barley
Sorghum, the fifth most important crop after the previously discussed four, provides sustenance for approximately 750 million people. It thrives in hot regions, requiring a temperature of 25°C for optimal yield. During water scarcity, it conserves water by rolling its leaves. With a protein content of 17%, sweet sorghum is cultivated in about 100 countries across 44 million hectares worldwide. Its potential for ethanol production, coupled with low production costs, makes it an economically viable option [125,126]. Sorghum plants facing the nitrogen stress show chlorosis of leaves, reduction in growth and sometimes it can be fatal. It decreases the number of leaves and the length of roots. The appearance of the purple color is also a symptom of nitrogen deficiency in sorghum [127]. Chlorosis occurs due to a decrease in leaf chlorophyll contents while leaf reflectance is increased [128]. The amount of nucleic acid, proteins, chlorophyll, and lipids in plants is decreased due to the production of relative oxygen species that are the result of nitrogen toxicity. Its toxicity produces some poisonous compounds that affect the germination of seeds and reduce plant growth. It also causes toxic problems in men [129].
11 Importance of Legumes (Nutritional and Nitrogen Fixation)
Among the flowering plants, the Leguminosae family ranks as the third largest family, comprising 800 genera. Within this family, some members are classified as grain crops, known as pulses, while others are considered weeds. Legumes are of great economic importance due to their high nutritional value, containing two to three times more protein than cereal crops. In addition to protein, they are rich in vitamins, folic acid, minerals, fatty acids, dietary fibers, and carbohydrates. They play a vital role in providing sustenance for millions of people. Soybean and pulses are cultivated over 121.5 million hectares and 82.4 million hectares of the world’s cultivable area [130,131]. The popularity of grain legumes is because of their higher content of proteins or oils [132]. The third most important crop is chickpea with 15% of total production among the pulses [133]. It acts as a vital source of protein for small farmers and is also used to feed animals [134]. Cowpeas have high nutritive value and improve soil fertility [135]. The protein content of soybean is 40%–42% while oil contents are 20%–22%. Among vegetable proteins, soybean contributes about 60% [136]. Lentil is cultivated worldwide as it provides maximum levels of essential amino acids, minerals, and Vitamin B1. Its major producers are Canada, Turkey, and India [137]. Legumes fix atmospheric nitrogen into ammonia by making symbiotic associations with bacteria known as rhizobia [138]. The association between the roots of legumes and soil bacteria results in the formation of nodules that release Nitrogenase enzymes and fix the atmospheric molecular nitrogen. This is a symbiotic type of association because both partners are providing benefits to each other. Bacteria get carbohydrates from plants and use them as a source of energy and to facilitate the reaction of Nitrogen fixation and nodule formation that provide reduced nitrogen to the plants. Biological Nitrogen fixation is important as it reduces the cost of production and prevents the environment from pollution that is caused by excessive use of industrial nitrogen. About 50–200 Mt of nitrogen is being fixed biologically per year [131]. Although legumes fix atmospheric nitrogen the amount of nitrogen fixed biologically is not too much to get a high yield. Therefore, we have to provide them with nitrogen by artificial means [132]. For the growth and germination of chickpeas there should be a small amount of nitrogen up to the establishment of symbiotic association and in nitrogen-deficient soils it is provided artificially and is known as a starter dose. Chickpea requirements of nitrogen are 15–20 kg per hectare [134], while in an experiment 40 kg of nitrogen proved to be optimum for cowpea yield and sometimes there is no effect of N on its yield [135]. Similarly, in peanuts, the same amount of nitrogen proved to be helpful as in cowpeas [139]. While optimum yield of soybean was obtained when nitrogen application rates were from 30–80 kg per hectare [136].
11.1 Symptoms of Nitrogen Deficiency and Excess in Legumes: Chickpeas, Cowpeas, Lentils, Beans
Symptoms of nitrogen deficiency first appear on older leaves showing yellowing and pigments of pink color on the upper surface of older leaves. These pigments also appear on the stem [140]. Pink pigmentation on leaves is caused by severe deficiency of nitrogen and first leaves become red then pink. The whole plant gives a yellowish appearance. Older leaves finally become white and drop [134]. Due to nitrogen deficiency, plant growth is retarded, leading to a change in the color of mature leaves, which become light yellow-green. Since nitrogen is a key component of chlorophyll, photosynthesis is also reduced in the presence of nitrogen deficiency. The overall growth of the plant is stunted, exhibiting a pale yellow color due to the lack of nitrogen. Cupping of upper-middle leaves occurs, and old leaves display a yellow color, sometimes leading to their death. Nitrogen deficiency in the late season results in reduced biomass and ultimately decreases the yield of lentil crops [135–137]. The maximum Nitrogen Use Efficiency of lentils is determined to be 110.1%. Nitrogen deficiency in the early season is observed during nodule development, and in the late season, it may be attributed to various biotic or abiotic factors. Initial symptoms manifest on older leaves, while younger leaves remain unaffected. Under mild conditions, a light green color predominates in the plant. Yellowing is evident in the upper three leaves, while the lower three leaves may become necrotic [138–140].
In case of excessive use of nitrogen in fava beans, the number of nodules and the dry weight is decreased. Moreover, in underground parts, the secretion of flavonoids are reduced due to the toxicity of nitrogen fertilizer. In an experiment, the reduction in flavonoid was observed at 13.2% in the case of a nitrogen-rich crop compared to the nitrogen-deficit crop [141]. In the case of soybean, excessive nitrogen affects the growth of nodules and the Nitrogenase activity. The food moving towards the nodules is decreased due to N toxicity. Finally, the Nitrogen Use Efficiency and the Nitrogen fixation is reduced [142].
Nitrogen is essential for the primary process of photosynthesis, and any disruption in its concentration can disturb this vital process. Despite its importance for plant growth, the application of Nitrogen fertilizer can lead to emissions of nitrogen oxide and dinitrogen oxide, subsequently decreasing the Nitrogen Use Efficiency of crops. The Nitrogen Use Efficiency varies among vegetables, ranging from 5% to 67.3% across different crops, with an increase observed when industrial nitrogen is combined with manure [143]. Leafy vegetables like spinach and lettuce necessitate nitrogen during their vegetative growth phase, as they are harvested before reaching the reproductive stage, requiring rapid leaf size development. Failure to apply nitrogen during the initial growth stage of spinach may result in smaller leaves. Conversely, tuber vegetables require nitrogen equally during both their vegetative and tuber phases [144]. Cucurbits require nitrogen levels ranging from over 110 kg to under 220 kg per hectare for optimal growth and development. Onions achieve optimal growth and yield with 100 kg of nitrogen per hectare, while potatoes exhibit maximum production with nitrogen application rates between 100 and 150 kg per hectare [145–147]. Optimal growth for radishes is achieved with 2.15 kg of nitrogen per 1000 kg of fleshy leaves, while tomatoes benefit from 240 kg of nitrogen for better growth, development, and yield. Vegetables are essential for human body growth as they supply dietary fibers, vitamins, plant chemicals, and minerals, enhancing vision, reducing heart diseases, certain cancers, and diabetes. Crucifers contain 40% non-starch parts and 50% dietary fibers in dry weight, while Alliaceae members contain 5% sulfur in dry weight, with onions being the highest fiber provider in the family. Tomatoes serve as a potassium source in First World countries and aid in skin protection, while potatoes offer ample carbohydrates, containing 7% starch when cooked and 13% when cooled, as well as essential amino acids [148–150].
12.1 Symptoms of Nitrogen Deficiency and Toxicity in Vegetable Crops: Cucurbits, Onion, Potato, Radish, Tomato
Optimal growth for radishes is achieved with 2.15 kg of nitrogen per 1000 kg of fleshy leaves, while tomatoes benefit from 240 kg of nitrogen for better growth, development, and yield. Vegetables are essential for human body growth as they supply dietary fibers, vitamins, plant chemicals, and minerals, enhancing vision, reducing heart diseases, certain cancers, and diabetes. Crucifers contain 40% non-starch parts and 50% dietary fibers in dry weight, while Alliaceae members contain 5% sulfur in dry weight, with onions being the highest fiber provider in the family. Tomatoes serve as a potassium source in First World countries and aid in skin protection, while potatoes offer ample carbohydrates, containing 7% starch when cooked and 13% when cooled, as well as essential amino acids [151]. The symptoms of nitrogen deficiency include chlorosis in the leaves. The size of the bulb is also reduced due to the deficiency of nitrogen [152]. Other symptoms include yellowing of younger leaves due to decreased chloroplast production, with symptoms evenly distributed across the entire leaf [153]. This yellowing signifies a reduction in chlorophyll content and impacts overall photosynthetic efficiency. Concurrently, plant growth is stunted as cell division decreases, while protein levels also decline, affecting the plant’s metabolic processes. Additionally, reduced nitrogen levels result in smaller leaves, altering the mass-to-volume ratio and diminishing light absorption, ultimately hindering photosynthesis. Notably, nitrogen deficiency can prove fatal for cotyledons and radishes demonstrate heightened anthocyanin levels under nitrogen stress, indicative of physiological responses to nutrient deficiencies. Similarly, in tomatoes, nitrogen stress leads to a decline in leaf nitrogen content, thereby reducing nitrogen levels in tissues and resulting in a subsequent decrease in plant biomass production [153–157]. The color of leaves deviates from green to less green [158]. Nitrogen stress disturbs the metabolic activities of tomato plants [159]. The toxicity of ammonium causes the change in PH between the cells, disturbs the balance of osmosis, and causes the unavailability of minerals to the plant. It decreases the calcium and magnesium in the plant. The toxicity of ammonium decreases the synthesis of chlorophyll which is the main factor in the process of photosynthesis. So, the process of photosynthesis is decreased, and less food is produced which causes the stunted growth of plants. The symptoms of yellowing and necrosis also appear on the leaves and the underground parts of the plant [160]. The middle and lower leaves are curved. The size of fruits is also reduced [151]. Nitrogen toxicity reduces the immunity of onion and it becomes affected by biotic or abiotic stress. The maturity of onion is delayed with two centers at the bulb due to the excessive use of nitrogen [161]. The toxicity of nitrogen causes a decrease in yield [162]. The yield of potatoes is decreased due to the toxicity of nitrogen because amino acids are not converted into protein. The plant focuses on the growth of stems and leaves [163]. Initially, by the excessive use of nitrogen, the growth of plants is increased and then goes on decreasing. And Nitrogen Use Efficiency is also decreased [164]. The toxicity of ammonium in radish causes a decrease in the processes of photosynthesis. It also decreases the rate of transpiration. In the case of below-ground parts, the accumulation of dry matter is reduced. While ammonium level is increased in the chloroplasts that block the passage of materials and affect the enzymatic activity of plants [165]. The toxicity of nitrogen causes to decrease in the immunity of the plant against biotic stress [166]. It affects the quality of fruits, has an effect on secondary metabolites of plants and disturbs the level of vitamins in fruits. The fruits with an excessive supply of nitrogen become soft and are destroyed earlier. The yield of tomatoes is decreased because the plant focuses on vegetative growth when there is excessive application of nitrogen. And it results in a decrease in yield [167]. It also causes a delay in the ripening of fruits and their size becomes smaller [168].
Nitrogen is important in the growth and development of fruits as it is part of the cell wall and is also vital for the metabolism of plants [169]. In biology fruit means the part of the plant that can be eaten [170]. Fruits are important for human beings as they contain dietary fibers, which help lower the level of cholesterol. They provide a high amount of minerals, plant chemicals, and vitamins, therefore they are considered protective [171]. Different fruits contain 61%–89.1% water, 0.5%–1.1% protein, Fats up to 4.4%, 4.4%–34.8% sugars, 2%–14.8% dietary fibers, and starch from trace amounts to 3% [172]. Citrus contains 0.17%v protein, Banana contains 0.18% and Apples contain 0.15%. In the case of carbohydrates, Apples contain 1.29% carbohydrates, Banana contains 1.27% and citrus contains 0.72% carbohydrates [170].
13.1 Symptoms of Nitrogen Deficiency and Toxicity in Fruits: Apple, Banana, Citrus, Grapes, Mango
The color of the leaves changes uniformly without patches or streaking due to nitrogen deficiency in apples, resulting in smaller-sized leaves. Additionally, symptoms initially manifest on older leaves before progressing toward the upper leaves, potentially leading to necrosis in severe conditions. Terminal shoots emerge but remain shorter. In terms of fruit development, apples under nitrogen deficiency bear fewer fruits, which ripen early and are smaller in size. Furthermore, plants experiencing nitrogen deficiency produce fruits of lower quality and nutritional value [173]. Due to nitrogen deficiency, the level of chlorophyll is decreased in leaves due to which it loses its green color. Initially, symptoms appear on lower leaves then in severe conditions upper leaves show a pink color [174]. The stem becomes thin which is pseudostem. The size of petioles becomes smaller and the life of leaves decreases. The growth of plants is also decreased [175]. First lower leaves are affected and then deficiency symptoms progress toward upper leaves with a light green to yellow color. Fresh leaves are smaller and slenderer. In addition, the older leaves before shedding change their color to yellow, and green streaks appear on the leaves. It also affects the color of fruits that become pale. The plants facing nitrogen deficiency may remain smaller due to a reduction in growth [176]. Sometimes the color of the veins becomes yellow but another part of the leaf remains green [177]. The color of leaves changes from green to light green and finally yellow while other parts of the plant show red or pink color. Reduction in growth of shoots appears. In fruits the available Nitrogen for yeast become decreased [178]. This occurs in leaves due to the low protein and break up of plastids, so the level of chlorophyll is decreased which is responsible for chlorosis. The vegetative growth of plants is decreased [179]. As in other fruits deficiency symptoms start appearing from older leaves and then move toward younger leaves, similar is the case here. Moreover, in severe conditions the whole plant becomes yellow. Chlorophyll content is reduced which reduces vegetative growth and finally, plant growth is reduced. Finally, the production of mango is decreased [180].
Fruits are produced with low calcium levels due to excessive nitrogen usage, and bananas exhibit the development of slender pseudostems under nitrogen toxicity, causing the slender stems to bend when bearing fruits. This delay in reproductive growth onset later in the season is accompanied by decreased Nitrogen Use Efficiency and elevated soil pH. In citrus, nitrogen toxicity impairs root conduction of nitrates towards the leaves, resulting in reduced enzyme activity, lower fruit quality, and compromised cell membranes. Moreover, heightened vegetative growth from nitrogen toxicity shortens the reproductive growth period, ultimately leading to decreased yield [181]. In grapes, poor stem growth results in delayed and low-quality fruit maturation, accompanied by darkening and cupping of the leaves, indicative of a vegetative growth dominance over reproductive growth. Excessive nitrogen application in mangoes renders the fruits more vulnerable to insect and pathogenic attacks, impeding proper color development upon ripening and leading to lower calcium levels. This disturbance in the vegetative growth phase ultimately diminishes yield [182].
14 Nitrogen and Climate: Role in Climate Change
Humans play a crucial role in altering the nitrogen cycle, and various nitrogen forms contribute to environmental degradation by influencing the climate. Certain nitrogen forms yield cooling effects, whereas others result in warming. Di nitrogen, a significant nitrogen form, plays a key role in cooling the environment. Similar to ozone, which captures sunlight rays from the sun, N2 gas enhances the ozone layer, trapping more sunlight and generating cooling effects. In contrast, nitrous oxide is a gas that depletes the ozone layer, leading to a warming effect as more rays reach the Earth. Both dinitrogen and nitrous oxide are classified as greenhouse gases [183]. Excessive nitrogen can cause air pollution, and water pollution by producing algal blooms, disturbing aquatic life, and destroying our soil. Therefore, the gasses emitted due to the toxicity of nitrogen and are responsible for pollution; include ammonia, Nitrous oxide, Nitrogen dioxide, and Nitric oxide.
14.1 Atmospheric Effects: Emission of Ammonia and Nitrous Oxide
Ammonia is a gas with a bad smell and it is harmful to both humans and the environment as it causes a disturbance for the eyes and skin and if it is present in high amounts then it disturbs our air passageways and lungs. Moreover, it leads to death, while in the case of eyes, it causes permanent damage to the eyes. Ammonia also causes diseases of the heart and sometimes heart attacks may occur due to this gas [184]. Oxides of Nitrogen (NO) inhibit the synthesis of different enzymes including aconitase, NADPH-oxidase, Ribonucleotide reductase, and other oxidoreductases [185]. High NO2 levels can prolong and exacerbate common viral infections and gravely damage the lungs [186]. According to recent estimates, NO2 pollution is responsible for 4.0 (1.8–5.2) million new cases of pediatric asthma each year, or 13% (range, 6%–16%) of the world’s asthma incidence [187]. In the case of the environment, it is deposited in the lakes, in the air, and soil. It increases the acidity of lake water which can be fatal for the aquatic life. Then ammonia can be deposited into the land and create problems. As the ratio of carbon to nitrogen in the soil is disturbed it causes the emission of nitrogen from the soil in any form. Ammonia can be deposited into the soil in two ways including dry deposition and wet deposition. In the case of dry deposition, ammonia combines with the dust particles present in the atmosphere and the force of gravity brings it to land. Ammonia combines with precipitation in wet deposition, where the agriculture sector contributes up to 70% of total anthropogenic emissions, primarily from livestock waste or excreta and the excessive use of fertilizers, mainly nitrogen [184].
Out of total anthropogenic gasses agricultural sector contributes two-thirds of it and the main contributor among the agricultural sector is the application of Fertilizers. Moreover, it contributes in the form of BNF, ammonia, and other nitrogen oxides. Two processes, denitrification and nitrification emit these gases. In addition, out of these gases, the emission of nitrous oxide is exceeding the predicted limits. As total emission of this gas in 1860 was 5.3 ± 0.4 Tg N2O-N a−1 exceeded up to 13.9 ± 1.4 Tg N2O-N a−1 up to the year 2020. It has been estimated that in previous years 15%–70% of the applied nitrogen is used by the plants while 5%–25% is being wasted in the soil, water, and environment [188]. The important factors responsible for the change in the climate are ozone depletion and global warming. Moreover, the main factor for ozone depletion and warming is the emission of greenhouse gases. China’s temperature rose by 0.91°C in the last century, exceeding the global increase of 0.85°C. Northern China experienced a greater temperature rise than the south. Nitrous oxide, a potent greenhouse gas from nitrogenous fertilizers, has a high warming potential, 25 times that of methane and 298 times that of carbon dioxide. Decreasing nitrogen fertilizer use can help control these harmful gas emissions [189]. Initially, inorganic fertilizers were promoted, but now Organic Nitrogen is recommended due to its favorable yield results and fewer harmful effects [190]. Similarly, Nitrogen dioxide is also responsible for respiratory diseases and for the diseases of Heart [191] (Fig. 4).
Figure 4: Effects of excessive nitrogen on different factors of the environment including aquatic life, soils, atmosphere, humans, and plants. Please increase the size of the very small letters. As they are, they are unreadable
14.2 Effect of Nitrogen on the Aquatic Environment
Ammonium, nitrate, nitrite, and ammonia are water-soluble; they can leach into groundwater, lakes, and rivers. After humans consume this water, nitrate can have various harmful effects on human health, while ammonium is generally considered non-toxic. Drinking water contaminated with nitrate can cause chest cancer, birth defects, and many problems in a child of above three months inside the mother. While the rest of the contaminated water is added to the lakes and becomes a threat to aquatic life. As that water contains more nitrogen in the form of nitrate the production and growth of aquatic plants and algae is increased. In addition, the competition for food and other requirements is increased in the aquatic ecosystem. When the growth of algae is increased so much that it covers the surface of the water this condition is known as algal bloom. Moreover, due to this algal bloom light cannot enter the water as it acts as a translucent object and also consumes much of the minerals and available food in the aquatic ecosystem. Besides these common blooms, some toxic blooms produce toxic compounds. These toxins are not only harmful to aquatic life but they have toxic effects on the terrestrial ecosystem. As humans consume fish these toxic compounds with the help of fish enter the human body and cause different problems in humans and sometimes, they can be fatal. They also affect other animals like dogs and birds etc. by drinking contaminated water or consuming the fishes affected with that algal bloom. By the death of algal bloom, the concentration of carbon dioxide in the water is increased and the available oxygen is reduced which proved to be fatal for the aquatic animals. Not only do algal blooms affect aquatic life but the direct addition of different nitrogen forms also affects aquatic animals. That has many impacts on the lifestyle of the organisms, decreasing available food for them, and calcium carbonate becomes more acidic which is used for making the skeleton of some organisms. Therefore, the skeleton made with acidic calcium carbonate is weak. Water also becomes more acidic that have negative impacts. It converts ammonia into ammonium which affects the central nervous system and can be fatal [184].
14.3 Effect on Carbon Cycle and Biodiversity
The excessive use of nitrogen is a key factor disrupting the carbon cycle. Nitrogen plays a crucial role in aiding microbes during the decomposition process, releasing carbon dioxide. Nitrogen-loving plants thrive, while those intolerant to high nitrogen levels perish. The imbalance also negatively impacts various species, rendering some more susceptible to drought and exhibiting poor growth under less drought stress [192]. When excessive nitrogen is applied to the plants it is not taken up by plants and cannot leach down with the water. So, it is added up into the soil and reacts with soil in different ways that cause soil acidification by decreasing soil PH. Except for nitric acid other forms of Nitrogenous fertilizers do not contribute directly to changing the PH but they aid in different ways to change it. In addition, one of the major problems caused by acidic soil is that it disturbs the growth of roots. Roots become weaker and cannot absorb nutrients properly which will result in stunted growth of the plants. Another problem caused by the low PH of soil is the addition of a toxic element. Aluminum is present in the clay and cannot dissolve in high PH, but due to higher concentrations of Nitrogenous fertilizers, the soil PH is decreased supporting the aluminum to dissolve and become available to the plants. When plants uptake that aluminum they face many problems as it is toxic. It affects the leaves, roots, and shoots of plants, and internally causes disturbance in cell division, metabolism, respiration, and many other processes of the plant body. It also disturbs the uptake of nutrients like magnesium, phosphorous, etc. Nitrogen toxicity causes soil salinity, which affects plant growth by changing the morphology, physiology, and molecular structures of plants. It also changes the morphology of soil.
Excessive fertilizers that are added to the soil decrease the length and strength of roots. Similarly, they are toxic to soil microorganisms and even can be fatal. Both of these things support soil erosion. Soil is compact and is held together by soil microorganisms and roots of plants and through many different processes. So, water, wind, snow, and animals can remove the topsoil that is rich in nutrients, that flows with the water into rivers and oceans. This is known as soil erosion. So the soil behind is not fertile for the growth of plants and the plants produced show weak health status and the symptoms of different nutrient deficiencies. Similarly, when there are short roots and no microorganisms to hold the compact layers of land together. Then topsoil will easily flow and the remaining weak soil will not be favorable for plant growth, as it does not contain minerals. Another problem related to excessive nitrogen is the leaching of other minerals. Nitrate combines with other minerals like calcium and when nitrate leaches down it brings those minerals with itself. It also helps in the addition of toxic metals to plants. As PH is lowered, it changes the forms of toxic metals like lead, cadmium, etc. Moreover, these forms become available for the plants and can be fatal for them. Moreover, it reduces the organic carbon in the soil [184]. Due to the emission of nitrogen into the atmosphere, rainwater becomes acidic. In addition, when it rains the acidity of plants increases causing many problems like loss of minerals by the plant body and it can lead to the death of the plant. The effect of acid rain is not limited to the plants but the microbial communities are also affected due to the acid rain. The balance of the ecosystems is [184]. Denitrification is a process in which bacteria convert Nitrogen into Nitrous oxide and Nitrogen gas.
As we are using excessive Nitrogenous fertilizers the nitrates increase the process of denitrification [192] (Fig. 4).
14.4 Impact of Climate Change on the Nitrogen Cycle
The nitrogen cycle is being disturbed due to human activities like excessive use of Nitrogenous fertilizers, deforestation, construction sites, burning of fossil fuels, pharmaceutical industries, and growing leguminous crops [193]. It has been found that burning fossil fuels produces nitrous oxide gas and contributes to the addition of nitrogen to the environment. Therefore, this Nitrous oxide gas causes acid rain which is a problem for plant health. The nitrogen Cycle has been disturbed in the last 300 years. Burning of fossil fuels is one of the major factors in disturbing this nitrogen cycle by adding reactive Nitrogen into the Atmosphere [194]. Excessive nitrogen poses numerous harmful effects on plant health, contributing to the loss of nitrogen into lakes, rivers, and through leaching down. Consequently, the natural system of Biological Nitrogen Fixation is disrupted. Additionally, deforestation leads to soil erosion, carrying away topsoil, minerals, and nitrogen [193]. Chemicals that are released from pharmaceutical industries are considered pollutants that disturb the nitrogen cycle in soil and water environments. Drugs that are being used by humans and other animals are also being added to the environment and becoming one of the factors for pollution. The waste of these drugs is being added to the water bodies and nitrifying bacteria are present there. These added chemicals disturb the nitrifying bacteria that inhabit the process of nitrification. It has been reported that harmful chemical compounds are removed during the process of nitrification. Nitrification involves chemicals that are more sensitive to harm than ammonification because a wide variety of microbes are involved in the process of ammonification. Different types of pharmaceutical chemicals disturb ammonification while some of them do not affect the formation of ammonia. When the complex of nystatin and pharmacies are present in the water in amounts of hundred mili grams per kilogram then they affect the formation of NH3− Similarly, when the quantity of erythromycin is 20 mg per kilogram of water then it reduces the synthesis of NH3− However, the presence of benzylpenicillin does not affect too much. Denitrification is less affected by the chemicals from the pharmaceutical industry, but certain types of chemicals can completely halt the denitrification process. For example, when Acetaminophen is present in the water in amounts of 250 mg per litter of water then it completely stops the process of denitrification. Anammox helps in nitrogen fixation. These bacteria have five different types but they are sensitive to abiotic stress and are also affected by the pollutants like that of pharmaceuticals [195]. Nitrogen assimilation is also disturbed by Cd toxicity so the foliar application of Alpha lipoic acid (Tomato plant) limits the cadmium uptake and enhances the nitrogen assimilation [196].
Nitrogen is necessary not only for plants but for all forms of life on the planet Earth. Similarly, its deficiency leads to a lack of chlorophyll giving the plants yellow color and a decrease in photosynthesis so the food produced is not enough for the consumers. It also pollutes the atmosphere and causes the problem of global warming by depleting the ozone layer. It also disturbs human health as it affects the respiratory system, Reproductive system, and heart. The rhizosphere is also being disturbed due to its excessive applications. Because we are utilizing excessive amounts to increase output, all of it is doing is making things worse for us rather than helping our crops produce more. The nitrogen use efficiency of different plants is different so we should apply nitrogen according to the requirements of the plant and in split form. Therefore, to overcome the problems of excess, deficiency, and pollution caused by Nitrogen we should analyze the soil before applying the Nitrogen which will decrease the chances of these problems. In addition, we should decrease the application of Nitrogenous fertilizer by improving the Nitrogen Use efficiency of plants which will help us to control pollution caused by Nitrogen. To lower production costs and boost productivity, we need to transition to nano-fertilizers.
Acknowledgement: Not applicable.
Funding Statement: The authors extend their appreciation to the Deanship of Scientific Research at King Khalid University for funding this work through large group research project under Grant Number RGP2/304/44.
Author Contributions: Muhammad Farhan, Manda Sathish, Syed Atif Hasan Naqvi and Mustansar Mubeen: Conceptualization; Rafia Kiran, Aroosa Mushtaq, Alaa Baazeem, Ammarah Hasnain, Fahad Hakim, Yasir Iftikhar, Aqleem Abbas, Muhammad Zeeshan Hassan and Mahmoud Moustafa: Writing original draft, editing, and revision; Syed Atif Hasan Naqvi and Mustansar Mubeen: Project administration and visualization; Aroosa Mushtaq, Alaa Baazeem, Ammarah Hasnain: Literature collection; Fahad Hakim and Syed Atif Hasan Naqvi: Figure preparations; Yasir Iftikhar: Validation and finalize the review; Syed Atif Hasan Naqvi, Manda Sathish and Alaa Bazeem: Supervision, resources and funding acquisition. All authors have read and agreed to the published version of the manuscript. All authors listed have made a substantial, direct, and intellectual contribution to the work and approved it for publication.
Availability of Data and Materials: Data sharing does not apply to this article.
Ethics Approval: Not applicable.
Conflicts of Interest: The authors declare that the review was written without any commercial or financial relationships that could be construed as a potential conflict of interest.
References
1. Aczel MR. What is the nitrogen cycle and why is it key to life? Front Young Minds. 2019;7:1–9. [Google Scholar]
2. Zhang H, Zhao Q, Wang Z, Wang L, Li X, Fan Z. Effects of nitrogen fertilizer on photosynthetic characteristics, biomass, and yield of wheat under different shading conditions. Agron. 2021;11(10):1–20. [Google Scholar]
3. Kang M, Wang J, Huang H. Nitrogen limitation as a driver of genome size evolution in a group of karst plants. Sci Rep. 2015;5(1):1–8. [Google Scholar]
4. Razaq M, Zhang P, Shen HL, Salahuddin. Influence of nitrogen and phosphorous on the growth and root morphology of Acer mono. PLoS One. 2017;12(2):1–13. [Google Scholar]
5. Matsuoka M, Kumar A, Muddassar M, Matsuyama A, Yoshida M, Zhang KYJ. Discovery of fungal denitrification inhibitors by targeting copper nitrite reductase from Fusarium oxysporum. J Chem Inf Model. 2017;57(2):203–13. [Google Scholar] [PubMed]
6. Stein LY, Klotz MG. The nitrogen cycle. Curr Biol. 2016;26:94–8. [Google Scholar]
7. Muratore C, Espen L, Prinsi B. Nitrogen uptake in plants: the plasma membrane root transport systems from a physiological and proteomic perspective. Plants. 2021;10(4):681. [Google Scholar] [PubMed]
8. Anas M, Liao F, Verma KK, Sarwar MA, Mahmood A, Chen ZL. Fate of nitrogen in agriculture and environment: agronomic, eco-physiological and molecular approaches to improve nitrogen use efficiency. Biol Res. 2020;53(1):1–20. [Google Scholar]
9. Elsiddig AMI, Zhou G, Zhu G, Nimir NEA, Suliman MSE, Ibrahim MEH, et al. Nitrogen fertilizer promoting salt tolerance of two sorghum varieties under different salt compositions. Chil J Agr Res. 2023;83(1):3–13. [Google Scholar]
10. Shahid SA, Zaman M, Heng L. Soil salinity: historical perspectives and a world overview of the problem. In: Guideline for salinity assessment, mitigation and adaptation using nuclear and related techniques. Springer; 2018. p. 43–53. [Google Scholar]
11. Akhtar M, Hussain F, Ashraf MY, Qureshi TM, Akhter J, Awan AR. Influence of salinity on nitrogen transformations in soil. Commun Soil Sci Plant Anal. 2012;43(12):1674–83. [Google Scholar]
12. McCauley A, Jones C, Jecobson J. Plant nutrient functions and deficiency and toxicity symptoms. Nutr Manag. 2009;9:1–16. [Google Scholar]
13. Sun J, Li W, Li C, Chang W, Zhang S, Zeng Y, et al. Effect of different rates of nitrogen fertilization on crop yield, soil properties and leaf physiological attributes in banana under subtropical regions of China. Front Plant Sci. 2020;11:613760. [Google Scholar] [PubMed]
14. Mălinaş A, Vidican R, Rotar I, Mălinaş C, Moldovan CM, Proorocu M. Current status and future prospective for nitrogen use efficiency in wheat (Triticum aestivum L.). Plants. 2022;11(2):217. [Google Scholar] [PubMed]
15. Greaver TL, Clark CM, Compton JE, Vallano D, Talhelm AF, Weaver CP, et al. Key ecological responses to nitrogen are altered by climate change. Nat Publ Gr. 2016;6(9):836–43. [Google Scholar]
16. Yuan SJ, Chen JJ, Lin ZQ, Li WW, Sheng GP, Yu HQ. Nitrate formation from atmospheric nitrogen and oxygen photocatalysed by nano-sized titanium dioxide. Nat Com. 2013;4(1):2249. [Google Scholar]
17. Ritter WF, Bergstrom L. Nitrogen and water. Agricultural nonpoint source pollution: watershed management and hydrology. 1st edLewis; 2000. p. 59. [Google Scholar]
18. Khanfar AR. Dissolved nitrogen in drinking water resources in Al-Mahareth village of Assir–Saudi Arabia. Saudi J Biol Sci. 2010;17(3):265–8. [Google Scholar] [PubMed]
19. Gojon A. Nitrogen nutrition in plants: rapid progress and new challenges. J Exp Bot. 2017;68(10):2457–62. [Google Scholar] [PubMed]
20. Kuziemska B, Trębicka J, Wysokinski A. Uptake and utilization of nitrogen from organic fertilizers influenced by different doses of copper. Agron. 2021;11(6):1219. [Google Scholar]
21. Prajapati P, Gupta P, Kharwar RN. Nitric oxide mediated regulation of ascorbate- glutathione pathway alleviates mitotic aberrations and DNA damage in Allium cepa L. under salinity stress. Int J Phytoremediation. 2023;25(4):403–14. [Google Scholar] [PubMed]
22. Kumar D, Parkash O, Deo R, Shekhar C. Titanium dioxide nanoparticles potentially regulate the mechanism(s) for photosynthetic attributes, genotoxicity, antioxidants defense machinery, and phytochelatins synthesis in relation to hexavalent chromium toxicity in Helianthus annuus L. J Hazard Mater. 2023;454:131418. [Google Scholar] [PubMed]
23. Pessarakli M. Handbook of plant and crop physiology. 3rd edBoca Raton: CRC Press; 2014. [Google Scholar]
24. Soliman M, Elkelish A, Souad T, Alhaithloul H, Farooq M. Brassinosteroid seed priming with nitrogen supplementation improves salt tolerance in soybean. Physiol Mol Biol Plants. 2020;26:501–11. [Google Scholar] [PubMed]
25. Hussain S, Shaukat M, Ashraf M, Zhu C, Jin Q, Zhang J. Salinity stress in arid and semi-arid climates: effects and management in field crops. Clim Change Agri. 2019;13:201–26. [Google Scholar]
26. Gupta P, Seth CS. 24-epibrassinolide regulates functional components of nitric oxide signalling and antioxidant defense pathways to alleviate salinity stress in Brassica juncea L. cv. Varuna. J Plant Growth Regul. 2022;42(7):4207–22. [Google Scholar]
27. Yang W, Hu Y, Yang M, Wen H, Jiao Y. Methane uptake and nitrous oxide emission in saline soil showed high sensitivity to nitrogen fertilization addition. Agron. 2023;13(2):473. [Google Scholar]
28. Zhao C, Hu C, Sun X, Huang W. Influence of temperature and moisture on nitrogen mineralization in vegetable fields of central China. Chin J Eco-Agric. 2012;20(7):861–6. [Google Scholar]
29. Hashemi SE, Madahhosseini S, Pirasteh-Anosheh H, Sedaghati E, Race M. The role of nitrogen in inducing salt stress tolerance in Crocus sativus L.: assessment based on plant growth and ions distribution in leaves. Sustain. 2023;15(1):567. [Google Scholar]
30. Pareek S, Sagar NA, Sharma S, Kumar V, Agarwal T, González-Aguilar GA, et al. Chlorophylls: chemistry and biological functions. In: Fruit veg phytochem: Chem hum health. 2nd edJohn Wiley & Sons, Ltd.; 2017. p. 269–84. [Google Scholar]
31. Wu Y, Qiang L, Rong J, Wei C, Liu XL, Kong FL, Yuan JC. Effect of low-nitrogen stress on photosynthesis and chlorophyll fluorescence characteristics of maize cultivars with different low-nitrogen tolerances. J Integr Agric. 2019;18(6):1246–56. [Google Scholar]
32. Hu J. Human alteration of the nitrogen cycle and its impact on the environment. IOP Conf Ser Earth Environ Sci. 2018a;178(1):6–11. [Google Scholar]
33. Bojović B, Marković A. Correlation between nitrogen and chlorophyll content in wheat (Triticum aestivum L.). Kragujev J Sci. 2009;31:69–74. [Google Scholar]
34. Bianco GV, Sacchetti A, Grande M, D’Orazio A, Milella A, Bruno G. Effective hole conductivity in nitrogen–doped CVD–graphene by singlet oxygen treatment under photoactivation conditions. Sci Rep. 2022;12(1):8703. [Google Scholar] [PubMed]
35. Shah JM, Bukhari SAH, Zeng JB, Quan XY, Ali E, Muhammad N, et al. Nitrogen (N) metabolism related enzyme activities, cell ultrastructure and nutrient contents as affected by N level and barley genotype. J Integr Agri. 2017;16(1):190–8. [Google Scholar]
36. Rivai RR, Miyamoto T, Awano T, Takada R, Tobimatsu Y, Umezawa T, et al. Nitrogen deficiency results in changes to cell wall composition of sorghum seedlings. Sci Rep. 2021;11(1):23309. [Google Scholar] [PubMed]
37. Lin YC, Hu YG, Ren CZ, Guo LC, Wang CL, Jiang Y, Zeng ZH. Effects of nitrogen application on chlorophyll fluorescence parameters and leaf gas exchange in naked oat. J Integr Agric. 2013;12(12):2164–71. [Google Scholar]
38. Song X, Zhou G, Ma BL, Wu W, Ahmad I, Zhu G, et al. Nitrogen application improved photosynthetic productivity, chlorophyll fluorescence, yield and yield components of two oat genotypes under saline conditions. Agron. 2019;9(3):115. [Google Scholar]
39. Jiang J, Liu W, Yang Z, Ma J, Zhang C, Zhang X. Effects of irrigation and nitrogen on growth and water productivity of maize grown in saline soil. Arch Agron Soil Sci. 2023;69(8):1223–35. [Google Scholar]
40. Elgharably A, Benes S. Alfalfa biomass yield and nitrogen fixation in response to applied mineral nitrogen under saline soil conditions. J Soil Sci Plant Nutr. 2021;21:744–55. [Google Scholar]
41. Follett JR, Follett RF. Utilization and metabolism of nitrogen by humans. In: Follett R, Hatfield JL, editors. Nitrogen in the environment: Sources, problems and management. Amsterdam, Netherlands: Elsevier Science; 2001. p. 65–92. [Google Scholar]
42. Palmer S. Recommended dietary allowances. Eur J Clin Nutr. 1990;44(Suppl. 2):13–21. [Google Scholar] [PubMed]
43. Melino VJ, Casartelli A, George J, Rupasinghe T, Roessner U, Okamoto M, et al. RNA catabolites contribute to the nitrogen pool and support growth recovery of wheat. Front Plant Sci. 2018;9:1539. [Google Scholar] [PubMed]
44. Lavrentieva NN, Dudaryonok AS. Nitrogen dioxide line shift coefficients induced by air pressure. Mol Phys. 2022;120(9):e2052370. [Google Scholar]
45. Langmuir I, Orange JA. Tungsten lamps of high efficiency—I and II. Proc Am Inst Electr Eng. 1913;32(10):1893–914. [Google Scholar]
46. Takai K. The nitrogen cycle: aa large, fast, and mystifying cycle. Microbes Environ. 2019;34(3):223–5. [Google Scholar] [PubMed]
47. Rosswall T. Microbiological regulation of the biogeochemical nitrogen cycle. Plants Soil. 1982;67:15–34. [Google Scholar]
48. Inomura K, Bragg J, Riemann L, Follows MJ. A quantitative model of nitrogen fixation in the presence of ammonium. PLoS One. 2018;13(11):1–16. [Google Scholar]
49. Bernhard A. The nitrogen cycle: processes, players, and human impact. Nat Educ Knowl. 2010;3(10):25. [Google Scholar]
50. Fay P, Stewart WDP, Walsby AE, Fogg GE. Is the heterocyst the site of nitrogen fixation in blue-green algae? Nature. 1968;220:810–2. [Google Scholar] [PubMed]
51. Wagner SC. Biological nitrogen fixation. Nat Edu Know. 2011;3(10):15. [Google Scholar]
52. Giller KE, Mapfumo P. Biological nitrogen fixation, forms and regulating factors. Encyclopedia of Soil Science. New York: Marcel Dekker; 2002. p. 107–9. [Google Scholar]
53. Bueno Batista M, Dixon R. Manipulating nitrogen regulation in diazotrophic bacteria for agronomic benefit. Biochem Soc Trans. 2019;47(2):603–14. [Google Scholar] [PubMed]
54. Dance I. Elucidating the coordination chemistry and mechanism of biological nitrogen fixation. Chem Asian J. 2007;2(8):936–46. [Google Scholar] [PubMed]
55. Danso SKA, Eskew DL. Enhancing biological nitrogen fixation. IAEA Bull. 1984;26(2):29–34. [Google Scholar]
56. Warembourg FR, Montange D, Bardin R. The simultaneous use of CO2 and N2 labelling techniques to study the carbon and nitrogen economy of legumes grown under natural conditions. Physio Plant. 2007;56:46–55. [Google Scholar]
57. Santi C, Bogusz D, Franche C. Biological nitrogen fixation in non-legume plants. Ann Bot. 2013;111(5):743–67. [Google Scholar] [PubMed]
58. Vitousek PM, Cassman K, Cleveland C, Crews T, Field CB, Rastettner EB, et al. Towards an ecological understanding of biological nitrogen fixation. Biogeochem. 2002;57:1–45. [Google Scholar]
59. Mahmud K, Makaju S, Ibrahim R, Missaoui A. Current progress in nitrogen fixing plants and microbiome research. Plants. 2020;9(1):1–17. [Google Scholar]
60. Montañez A. Overview and case studies on biological nitrogen fixation: perspectives and limitations. Sci Agric. 2000;1–11. [Google Scholar]
61. Drapcho DL, Sisterson D, Kumar R. Nitrogen fixation by lightning activity in a thunderstorm. Atmos Environ. 1983;17(4):729–34. [Google Scholar]
62. Dechorgnat J, Nguyen CT, Armengaud P, Jossier M, Diatloff E, Filleur S, et al. From the soil to the seeds: the long journey of nitrate in plants. J Exp Bot. 2011;62(4):1349–59. [Google Scholar] [PubMed]
63. Glamorgan V. Robert le rossignol, 1884–1976: engineer of the ‘haber’ process. R Soc. 2017;71:263–96. [Google Scholar]
64. Humphreys J, Lan R, Tao S. Development and recent progress on ammonia synthesis catalysts for Haber-Bosch process. Adv Energy Sustain Res. 2021;2(1):2000043. [Google Scholar]
65. Doane TA. The abiotic nitrogen cycle. ACS Earth Sp Chem. 2017;1(7):411–21. [Google Scholar]
66. Shiozaki T, Ijichi M, Isobe K, Hashihama F, Nakamura KI, Ehama M, et al. Nitrification and its influence on biogeochemical cycles from the equatorial Pacific to the Arctic Ocean. ISME J. 2016;10(9):2184–97. [Google Scholar] [PubMed]
67. Fan H, Bolhuis H, Stal LJ. Nitrification and nitrifying bacteria in a coastal microbial mat. Front Microbiol. 2015;1(6):1367. [Google Scholar]
68. Suri N, Zhang Y, Gieg LM, Ryan MC. Denitrification biokinetics: towards optimization for industrial applications. Front Microbiol. 2021;5(12):610389. [Google Scholar]
69. Alldred M, Binas SB. Effects of wetland plants on denitrification rates: a meta-analysis. Ecol Appl. 2016;26:676–85. [Google Scholar] [PubMed]
70. Menz J, Range T, Trini J, Ludewig U, Neuhäuser B. Molecular basis of differential nitrogen use efficiencies and nitrogen source preferences in contrasting Arabidopsis accessions. Sci Rep. 2018;8(1):3373. [Google Scholar] [PubMed]
71. Xu G, Fan X, Miller AJ. Plant nitrogen assimilation and use efficiency. Annu Rev Plant Biol. 2012;63:153–82. [Google Scholar] [PubMed]
72. Hu B, Wang W, Ou S, Tang J, Li H, Che R, et al. Variation in NRT1. 1B contributes to nitrate-use divergence between rice subspecies. Nat Genet. 2015;47(7):834–8. [Google Scholar] [PubMed]
73. Chen J, Zhang Y, Tan Y, Zhang M, Zhu L, Xu G, et al. Agronomic nitrogen-use efficiency of rice can be increased by driving Os NRT 2.1 expression with the Os NAR 2.1 promoter. Plant Biotechnol J. 2016;14(8):1705–15. [Google Scholar] [PubMed]
74. Sharma LK, Bali SK. A review of methods to improve nitrogen use efficiency in agriculture. Sustain. 2017;10(1):51. [Google Scholar]
75. Chardon F, Barthélémy J, Daniel-Vedele F, Masclaux-Daubresse C. Natural variation of nitrate uptake and nitrogen use efficiency in Arabidopsis thaliana cultivated with limiting and ample nitrogen supply. J Exp Bot. 2010;61(9):2293–302. [Google Scholar] [PubMed]
76. Cui X, Yan QW, Sun JL, Xiao S, Xie FC, Chen YJ. Research progress on nitrogen use and plant growth. J Northeast Agric Univ (English Edition). 2014;21(2):68–74. [Google Scholar]
77. Mooshammer M, Wanek W, Hämmerle I, Fuchslueger L, Hofhansl F, Knoltsch A, et al. Adjustment of microbial nitrogen use efficiency to carbon: nitrogen imbalances regulates soil nitrogen cycling. Nat commun. 2014;5(1):3694. [Google Scholar] [PubMed]
78. Dobermann AR. Nitrogen use efficiency-state of the art. Agron Faculty Publ. 2005;1:316. [Google Scholar]
79. Sandhu N, Sethi M, Kumar A, Dang D, Singh J, Chhuneja P. Biochemical and genetic approaches improving nitrogen use efficiency in cereal crops: a review. Front Plant Sci. 2021;12:657629. [Google Scholar] [PubMed]
80. Shahzad AN, Qureshi MK, Wakeel A, Misselbrook T. Crop production in Pakistan and low nitrogen use efficiencies. Nat Sustain. 2019;2(12):1106–14. [Google Scholar]
81. Shaviv A. Environmental friendly nitrogen fertilization. Sci China Ser C Life Sci. 2005;48:937–47. [Google Scholar]
82. Haroon M, Idrees F, Naushahi HA, Afzal R, Usman M, Qadir T, et al. Nitrogen use efficiency: farming practices and sustainability. J Exp Agric Int. 2019;36(3):1. [Google Scholar]
83. Xing Y, Jiang W, He X, Fiaz S, Ahmad S, Lei X, et al. A review of nitrogen translocation and nitrogen-use efficiency. J Plant Nut. 2019;42(19):2624–41. [Google Scholar]
84. Hojjatie MM. Urea triazone fertilizers-A slow-release nitrogen fertilizer. Int J Agric Sci Food Technol. 2021;7(3):272–6. [Google Scholar]
85. Giacomini SJ, Machet JM, Boizard H, Recous S. Dynamics and recovery of fertilizer 15N in soil and winter wheat crop under minimum versus conventional tillage. Soil Tillage Res. 2010;108(1–2):51–8. [Google Scholar]
86. Boquet DJ, Hutchinson RL, Breitenbeck GA. Long-term tillage, cover crop, and nitrogen rate effects on cotton: yield and fiber properties. Agron J. 2004;96(5):1436–42. [Google Scholar]
87. Kumar A, Singh K, Verma P, Singh O, Panwar A, Singh T, et al. Effect of nitrogen and zinc nanofertilizer with the organic farming practices on cereal and oil seed crops. Sci rep. 2022;12(1):6938. [Google Scholar] [PubMed]
88. Wang P, Lombi E, Zhao FJ, Kopittke PM. Nanotechnology: a new opportunity in plant sciences. Trends Plant Sci. 2016;21(8):699–712. [Google Scholar] [PubMed]
89. El-Saadony MT, ALmoshadak AS, Shafi ME, Albaqami NM, Saad AM, El-Tahan AM, et al. Vital roles of sustainable nano-fertilizers in improving plant quality and quantity–an updated review. Saudi J Biol Sci. 2021;28(12):7349–59. [Google Scholar] [PubMed]
90. Tarafdar JC, Raliya R, Mahawar H, Rathore I. Development of zinc nanofertilizer to enhance crop production in pearl millet (Pennisetum americanum). Agric Res. 2014;3:257–62. [Google Scholar]
91. Jaberzadeh A, Moaveni P, Moghadam HR, Zahedi H. Influence of bulk and nanoparticles titanium foliar application on some agronomic traits, seed gluten and starch contents of wheat subjected to water deficit stress. Not Bot Horti Agrobot Cluj-napoca. 2013;41(1):201–7. [Google Scholar]
92. Chenping X. Nitrogen deficiency-induced protein changes in immature and mature leaves of creeping bentgrass. J Amer Soc Hort Sci. 2011;136(6):399–407. [Google Scholar]
93. Huang CH, Singh GP, Park SH, Chua NH, Ram RJ, Park BS. Early diagnosis and management of nitrogen deficiency in plants utilizing Raman spectroscopy. Front Plant Sci. 2020;11:663. [Google Scholar] [PubMed]
94. Uchida R. Essential nutrients for plant growth: nutrient functions and deficiency symptoms. In: Silva JA, Uchida R, editors. Plant nutrient management in Hawaii’s soils, approaches for tropical and subtropical agriculture. Honolulu: University of Hawaii at Manoa; 2000. p. 31–55. [Google Scholar]
95. Trujillo Marín EE, Wang C, Singha A, Bloem E, Zandi P, Barabasz-Krasny B, et al. Reduced nitrogen proportion during the vegetative growth stage improved fruit yield and nitrogen uptake of cherry tomato plants under sufficient soil water regime. Acta Agric Scand B Soil Plant Sci. 2022;72(1):700–8. [Google Scholar]
96. Yuan S, Ling Y, Xiong Y, Zhang C, Sha L, You M, et al. Effect of nitrogen fertilizer on seed yield and quality of Kengyilia melanthera (Triticeae, Poaceae). PeerJ. 2022;10:e14101. [Google Scholar] [PubMed]
97. Tang J, Sun B, Cheng R, Shi Z, Luo D, Liu S, et al. Effects of soil nitrogen (N) deficiency on photosynthetic N-use efficiency in N-fixing and non-N-fixing tree seedlings in subtropical China. Sci Rep. 2019;9(1):4604. [Google Scholar] [PubMed]
98. Sanagi M, Aoyama S, Kubo A, Lu Y, Sato Y, Ito S, et al. Low nitrogen conditions accelerate flowering by modulating the phosphorylation state of FLOWERING BHLH 4 in Arabidopsis. PNAS. 2021;118(19):e2022942118. [Google Scholar] [PubMed]
99. Xiong Q, Tang G, Zhong L, He H, Chen X. Response to nitrogen deficiency and compensation on physiological characteristics, yield formation, and nitrogen utilization of rice. Front Plant Sci. 2018;9:1075. [Google Scholar] [PubMed]
100. Elhanafi L, Houhou M, Rais C, Mansouri I, Elghadraoui L, Greche H. Impact of excessive nitrogen fertilization on the biochemical quality, phenolic compounds, and antioxidant power of Sesamum indicum L seeds. J Food Qual. 2019;4:2019. [Google Scholar]
101. Verdenal T, Dienes-Nagy Á., Spangenberg JE, Zufferey V, Spring JL, Viret O, et al. Understanding and managing nitrogen nutrition in grapevine: a review. Oeno One. 2021;55(1):1–43. [Google Scholar]
102. Salim N, Raza A. Nutrient use efficiency (NUE) for sustainable wheat production: a review. J Plant Nutr. 2020;43(2):297–315. [Google Scholar]
103. Shewry PR, Hey SJ. The contribution of wheat to human diet and health. Food Energy Secur. 2015;4(3):178–202. [Google Scholar] [PubMed]
104. Snowball K, Robson AD. Nutrient deficiencies and toxicities in wheat: a guide for field identification. Mexico: CIMMYT; 1991. [Google Scholar]
105. Wang Y, Wang D, Tao Z, Yang Y, Gao Z, Zhao G, et al. Impacts of nitrogen deficiency on wheat (Triticum aestivum L.) grain during the medium filling stage: transcriptomic and metabolomic comparisons. Front Plant Sci. 2021;12:674433. [Google Scholar] [PubMed]
106. Mojid MA, Wyseure GC, Biswas SK. Requirement of nitrogen, phosphorus and potassium fertilizers for wheat cultivation under irrigation by municipal wastewater. J Soil Sci Plant Nutr. 2012;12(4):655–65. [Google Scholar]
107. Diacono M, Rubino P, Montemurro F. Precision nitrogen management of wheat. A review. Agron Sustain Dev. 2013;33(1):219–41. [Google Scholar]
108. Yu F, Feng S, Du W, Wang D, Guo Z, Xing S, et al. A study of nitrogen deficiency inversion in rice leaves based on the hyperspectral reflectance differential. Front Plant Sci. 2020;11:573272. [Google Scholar] [PubMed]
109. Witt C, Buresh RJ, Balasubramanian V, Dawe D, Dobermann A. Improving nutrient management strategies for delivery in irrigated rice in Asia. Better Crops Int. 2002;16(2):24–31. [Google Scholar]
110. Shrestha J, Kandel M, Subedi S, Shah KK. Role of nutrients in rice (Oryza sativa L.a review. Agrica. 2020;9(1):53–62. [Google Scholar]
111. Khan Z, Nauman Khan M, Luo T, Zhang K, Zhu K, Rana MS, et al. Compensation of high nitrogen toxicity and nitrogen deficiency with biochar amendment through enhancement of soil fertility and nitrogen use efficiency promoted rice growth and yield. Gcb Bioenergy. 2021;13(11):1765–84. [Google Scholar]
112. Adiaha MS. The impact of Maize (Zea mays L.) and it uses for human development: a review. Int J Sci World. 2017;5:93. [Google Scholar]
113. Adhikari K, Bhandari S, Aryal K, Mahato M, Shrestha J. Effect of different levels of nitrogen on growth and yield of hybrid maize (Zea mays L.) varieties. J Agric Nat Resour. 2021;4(2):48–62. [Google Scholar]
114. Singh AK, Singh JP, Singh JP. Boron in crop production from soil to plant system: a review. Arch Agric Environ Sci. 2020;5(2):218–22. [Google Scholar]
115. Shrestha J, Chaudhary A, Pokhrel D. Application of nitrogen fertilizer in maize in Southern Asia a review. Peruv J agron. 2018;2(2):22–6. [Google Scholar]
116. Schlüter U, Mascher M, Colmsee C, Scholz U, Bräutigam A, Fahnenstich H, et al. Maize source leaf adaptation to nitrogen deficiency affects not only nitrogen and carbon metabolism but also control of phosphate homeostasis. Plant Physiol. 2012;160(3):1384–406. [Google Scholar]
117. Sridevy S, Vijendran AS, Jagadeeswaran R, Djanaguiraman M. Nitrogen and potassium deficiency identification in maize by image mining, spectral and true colour response. Indian J Plant Physiol. 2018;23:91–9. [Google Scholar]
118. Rhezali A, Lahlali R. Nitrogen (N) mineral nutrition and imaging sensors for determining N status and requirements of maize. J Imag. 2017;3(4):51. [Google Scholar]
119. Tian G, Qi D, Zhu J, Xu Y. Effects of nitrogen fertilizer rates and waterlogging on leaf physiological characteristics and grain yield of maize. Arch Agron Soil Sci. 2021;67(7):863–75. [Google Scholar]
120. Ngosong C, Bongkisheri V, Tanyi CB, Nanganoa LT, Tening AS. Optimizing nitrogen fertilization regimes for sustainable maize (Zea mays L.) production on the volcanic soils of Buea Cameroon. Hindawi: Adv Agric. 2019;2019(1–8):4681825. doi:10.1155/2019/4681825. [Google Scholar] [CrossRef]
121. Tanaka R, Nakano H. Barley yield response to nitrogen application under different weather conditions. Sci Rep. 2019;9(1):8477. [Google Scholar] [PubMed]
122. Comadira G, Rasool B, Karpinska B, Morris J, Verrall SR, Hedley PE, et al. Nitrogen deficiency in barley (Hordeum vulgare) seedlings induces molecular and metabolic adjustments that trigger aphid resistance. J Exp Bot. 2015;66(12):3639–55. [Google Scholar] [PubMed]
123. Fiaz M, Ahmed I, Hassan SM, Niazi AK, Khokhar MF, Farooq MA, et al. Antibiotics induced changes in nitrogen metabolism and antioxidative enzymes in mung bean (Vigna radiata). Sci Total Environ. 2023;873:162449. [Google Scholar] [PubMed]
124. Britto DT, Kronzucker HJ. NH4+ toxicity in higher plants: a critical review. J Plant Physiol. 2002;159(6):567–84. [Google Scholar]
125. Ramatoulaye F, Mady C, Fallou S. Production and use sorghum: a literature review. JNHFS. 2016;4(1):1–4. [Google Scholar]
126. Olugbemi O. Sweet sorghum and nitrogen fertilizer application â€″a review. MAYFEB J Agric Sci. 2017;2:28–35. [Google Scholar]
127. Christin H, Petty P, Ouertani K, Burgado S, Lawrence C, Kassem MA. Influence of iron, potassium, magnesium, and nitrogen deficiencies on the growth and development of sorghum (Sorghum bicolor L.) and sunflower (Helianthus annuus L.) seedlings. J Biotech Res. 2009;1(3):64–71. [Google Scholar]
128. Zhao D, Reddy KR, Kakani VG, Reddy VR. Nitrogen deficiency effects on plant growth, leaf photosynthesis, and hyperspectral reflectance properties of sorghum. Eur J Agron. 2005;22(4):391–403. [Google Scholar]
129. Ahmad I, Zhu G, Zhou G, Song X, Hussein Ibrahim ME, Ibrahim Salih EG. Effect of N on growth, antioxidant capacity, and chlorophyll content of sorghum. Agron. 2022;12(2):501. [Google Scholar]
130. Stagnari F, Maggio A, Galieni A, Pisante M. Multiple benefits of legumes for agriculture sustainability: an overview. Chem Biol Technol Agric. 2017;4(1):1–3. [Google Scholar]
131. Schwember AR, Schulze J, Del Pozo A, Cabeza RA. Regulation of symbiotic nitrogen fixation in legume root nodules. Plants. 2019;8(9):333. [Google Scholar] [PubMed]
132. Cheema ZA, Ahmad A. Effects of urea on the nitrogen fixing capacity and growth of grain legumes. Int J Agric Biol. 2000;2(4):388–94. [Google Scholar]
133. Aliloo AA, Khorsandy H, Mustafavi SH. Response of chickpea (Cicer arietinum L.) cultivars to nitrogen applications at vegetative and reproductive stages. Cercet Agron Mold. 2013;45(4):49–55. [Google Scholar]
134. W/Lemma, Senbet Haile W, Beyene S. Response of chickpea (Cicer arietinum L.) to nitrogen and phosphorus fertilizer s in halaba and taba, Southern Ethiopia. Ethiop J Nat Resour. 2013;13(2):115–28. [Google Scholar]
135. Daramy MA, Sarkodie-Addo J, Dumbuya G. Effect of nitrogen and phosphorus fertilizer application on growth and yield performance of cowpea in Ghana. JEBAS. 2017;5(1):38–44. [Google Scholar]
136. Yadav SS, McNeil D, Stevenson PC. Lentil: an ancient crop for modern times. New York City: Springer Science & Business Media; 2007. [Google Scholar]
137. Choudhry M. Growth, yield and nitrogen content of lentil (Lens culinaris Medic) as affected by nitrogen and diquat application (Doctoral Dissertation). University of Saskatchewan: Canada; 2012. [Google Scholar]
138. Kabir MH, Das P, Islam MM, Hossain MB, Mamun ANK, VRR. Effect of different doses of nitrogen on nitrogen fixation and yield of lentil using tracer technique. GSC Biol Pharm Sci. 2019;6(3):69–75. doi:10.30574/gscbps.2019.6.3.0027. [Google Scholar] [CrossRef]
139. Hussain N, Bahar FA, Mehdi SS, Bhat MA, Hussain A, Kanth RH, et al. A brief insight into nutritional deficiencies in pulses and their possible management strategies a review. Curr Appl Sci Technol. 2021;40(5):101–13. [Google Scholar]
140. de Souza Osório CR, Marques Teixeira GC, Barreto RF, Silva Campos CN, Freitas Leal AJ, Teodoro PE, et al. Macronutrient deficiency in snap bean considering physiological, nutritional, and growth aspects. PLoS One. 2020;15(6):e0234512. [Google Scholar]
141. Liu Y, Yin X, Xiao J, Tang L, Zheng Y. Interactive influences of intercropping by nitrogen on flavonoid exudation and nodulation in faba bean. Sci Rep. 2019;9(1):4818. [Google Scholar] [PubMed]
142. Xia X, Ma C, Dong S, Xu Y, Gong Z. Effects of nitrogen concentrations on nodulation and nitrogenase activity in dual root systems of soybean plants. J Soil Sci Plant Nutr. 2017;63(5):470–82. [Google Scholar]
143. Pan SY, He KH, Lin KT, Fan C, Chang CT. Addressing nitrogenous gases from croplands toward low-emission agriculture. npj Clim Atmos Sci. 2022;5(1):43. [Google Scholar]
144. Lombardo S, Pandino G, Mauromicale G. Optimizing nitrogen fertilization to improve qualitative performances and physiological and yield responses of potato (Solanum tuberosum L.). Agron. 2020;10(3):352. [Google Scholar]
145. van Eerd LL, O’Reilly KA. Yield, nitrogen dynamics, and fertilizer use efficiency in machine-harvested cucumber. Hort Sci. 2009;44(6):1712–8. [Google Scholar]
146. Gebretsadik K, Dechassa N. Response of onion (Allium cepa L.) to nitrogen fertilizer rates and spacing under rain fed condition at Tahtay Koraro. Ethiopia Sci Rep. 2018;8(1):1–8. [Google Scholar]
147. Liu K, Du J, Zhong Y, Shen Z, Yu X. The response of potato tuber yield, nitrogen uptake, soil nitrate nitrogen to different nitrogen rates in red soil. Sci Rep. 2021;11(1):22506. [Google Scholar] [PubMed]
148. Zhang J, He P, Ding W, Xu X, Ullah S, Abbas T, et al. Estimating nutrient uptake requirements for radish in China based on QUEFTS model. Sci Rep. 2019;9(1):11663. [Google Scholar] [PubMed]
149. Wang X, Xing Y. Evaluation of the effects of irrigation and fertilization on tomato fruit yield and quality: a principal component analysis. Sci Rep. 2017;7(1):350. [Google Scholar] [PubMed]
150. Dias JS. Nutritional quality and health benefits of vegetables: a review. Food Nut Sci. 2012;3(10):1354–74. [Google Scholar]
151. Eysinga JPNLRV, Smilde KW. Nutritional disorders in cucumbers and gherkins. Wageningen: Centre for Agricultural Publishing and Documentation; 1969. p. 46. [Google Scholar]
152. Jones MG, Hughes J, Tregova A, Milne J, Tomsett AB, Collin HA. Biosynthesis of the flavour precursors of onion and garlic. J Exp Bot. 2004;55(404):1903–18. [Google Scholar] [PubMed]
153. Naumann M, Koch M, Thiel H, Gransee A, Pawelzik E. The importance of nutrient management for potato production part II: plant nutrition and tuber quality. Pot Res. 2020;63:121–37. [Google Scholar]
154. Bewket GB. Review on nitrogen: forms, functions and effects on potato growth performance. World Sci News. 2018;101:44–54. [Google Scholar]
155. Kovacik P. Deficiency symptoms of nitrogen, phosphorus, potassium and sulphur in radish plants. Acta Fytotech Zootech. 2001;4(2):38–42. [Google Scholar]
156. Su N, Wu Q, Cui J. Increased sucrose in the hypocotyls of radish sprouts contributes to nitrogen deficiency-induced anthocyanin accumulation. Front Plant Sci. 2016;7:1976. [Google Scholar] [PubMed]
157. Bénard C, Bourgaud F, Gautier H. Impact of temporary nitrogen deprivation on tomato leaf phenolics. Int J Mol Sci. 2011;12(11):7971–81. [Google Scholar]
158. Passam HC, Karapanos IC, Bebeli PJ, Savvas D. A review of recent research on tomato nutrition, breeding and post-harvest technology with reference to fruit quality. Eur J Plant Sci Biotechnol. 2007;1(1):1–21. [Google Scholar]
159. Reimer JJ, Thiele B, Biermann RT, Junker-Frohn LV, Wiese-Klinkenberg A, Usadel B, et al. Tomato leaves under stress: a comparison of stress response to mild abiotic stress between a cultivated and a wild tomato species. Plant Mol Biol. 2021;107(3):177–206. [Google Scholar] [PubMed]
160. Campos CN, de Mello Prado R, Caione G. Silicon and excess ammonium and nitrate in cucumber plants. Afr J Agric Res. 2016;11(4):276–83. [Google Scholar]
161. Moyne AL, Waite-Cusic J, Harris LJ. Water application method influences survival or growth of escherichia coli on bulb onions during field curing. J Food Protect. 2022;85(6):961–72. [Google Scholar]
162. Zhao C, Ni H, Zhao L, Zhou L, Borrás-Hidalgo O, Cui R. High nitrogen concentration alter microbial community in Allium fistulosum rhizosphere. PLos One. 2020;15(11):e0241371. [Google Scholar] [PubMed]
163. Messele B. Effects of nitrogen and phosphorus rates on growth, yield, and quality of onion (Allium cepa L.) at Menschen Für Menschen Demonstration Site, Harar, Ethiopia. Agri Res Tech Open Access J. 2016;1(3):001–8. [Google Scholar]
164. He X, Qiu H, Xie K, Wang Y, Hu J, Li F, et al. Effects of water saving and nitrogen reduction on the yield, quality, water and nitrogen use efficiency of Isatis indigotica in Hexi Oasis. Sci Rep. 2022;12(1):550. [Google Scholar] [PubMed]
165. Olivera Viciedo D, de Mello Prado R, Lizcano Toledo R, Nascimento dos Santos LC, Peña Calzada K. Respuesta de las plántulas del rábano (Raphanus sativus L.) a diferentes concentraciones de nitrógeno amoniacal en ausencia y presencia de silicio. Agron Colom. 2017;1:198–204. [Google Scholar]
166. Islam MN, Hasanuzzaman AT, Zhang ZF, Zhang Y, Liu TX. High level of nitrogen makes tomato plants releasing less volatiles and attracting more Bemisia tabaci (Hemiptera: aleyrodidae). Front Plant Sci. 2017;8:466. [Google Scholar] [PubMed]
167. Frías-Moreno MN, Espino-Díaz M, Dávila-Aviña J, Gonzalez-Aguilar GA, Ayala-Zavala JF, Molina-Corral FJ, et al. Preharvest nitrogen application affects quality and antioxidant status of two tomato cultivars. Bragantia. 2020;79:134–44. [Google Scholar]
168. Bodale I, Mihalache G, Achiţei V, Teliban GC, Cazacu A, Stoleru V. Evaluation of the nutrients uptake by tomato plants in different phenological stages using an electrical conductivity technique. Agric. 2021;11(4):292. [Google Scholar]
169. Pande KK, Dimri DC. Response of nitrogen application in fruit trees: a review. Int J Curr Microbiol Appl Sci. 2020;9(5):545–59. [Google Scholar]
170. Rejman K, Górska-Warsewicz H, Kaczorowska J, Laskowski W. Nutritional significance of fruit and fruit products in the average polish diet. Nutri. 2021;13(6):2079. [Google Scholar]
171. Singh AK, Pal R, Abrol G, Punetha S, Sharma P, Pandey AK. Nutritional and medicinal value of underutilized fruits. Acta Sci Agric. 2019;3(1):16–22. [Google Scholar]
172. Slavin JL, Lloyd B. Health benefits of fruits and vegetables. Adv Nutr. 2012;3(4):506–16. [Google Scholar] [PubMed]
173. Miñarro M. Weed communities in apple orchards under organic and conventional fertilization and tree-row management. Crop Protection. 2012;39:89–96. [Google Scholar]
174. Weinert MP, Simpson M. Subtropical banana nutrition-matching nutrition requirements to growth demands. Wollongbar: NSW Department of Primary Industries; 2016. Available from: http://www.musalit.org. [Accessed 2023]. [Google Scholar]
175. Samuels G, Cibes-Viade H. Mineral-deficiency symptoms of bananas. In: 2nd Annual Meeting, 1964; Bridgetown; Barbados from Caribbean Food Crops Society. doi:10.22004/ag.econ.263727. [Google Scholar] [CrossRef]
176. Zekri M, Obreza TA. Macronutrient deficiencies in citrus: nitrogen, phosphorus, and potassium. University of Florida Cooperative Extension Service, Institute of Food and Agricultural Sciences: UF, USA; 2003. p. 1–3. [Google Scholar]
177. Futch SH, Tucker DPH. A guide to citrus nutritional deficiency and toxicity identification. Univeristy of Florida IFAS Extension. 2008;1–6. Available from: https://edis.ifas.ufl.edu/publication/CH142. [Accessed 2023]. [Google Scholar]
178. Leslie J. Farmer Nutrient Management Decisions: A Study of Farms in the Gully Creek Watershed in Southern Ontario. Canada: Doctoral dissertation, University of Guelph; 2016. Available from: http://atrium.lib.uoguelph.ca. [Accessed 2023]. [Google Scholar]
179. Ozdemir G, Sabir A, Tangolar S. The effects of different nitrogen doses on yield, quality and leaf nitrogen content of some early grape cultivars (V. vinifera L.) grown in greenhouse. Afric J Biotechnol. 2010;9(32):5108–12. [Google Scholar]
180. Prado RM, Caione G, Silva DJ. Macronutrients and micronutrients deficiency symptoms in mango. Jodhpur, India: Studium Press LLC; 2012. p. 470–8. [Google Scholar]
181. Becker FG, Cleary M, Team RM, Holtermann H, Agenda D, et al. Nutrition and fertilization in mango. Literature review. Syria Stud. 2015;7:37–72. [Google Scholar]
182. Weinert M. Understanding crop nutrition a guide for Australian mango growers, Mango industry communications program 2016-7 (MG15006); 2010. Available from: https://www.horticulture.com.au/growers/help-your-business-grow/. [Accessed 2023]. [Google Scholar]
183. Fremantle K, de Vito L, Williams B, Longhurst J. A framework for assessing the contribution of UK local authorities toward compliance with the EU ambient air quality directive. J Environ Plan Manage. 2022;65(2):356–73. [Google Scholar]
184. Wang X, Fan J, Xing Y, Xu G, Wang H, Deng J, et al. The effects of mulch and nitrogen fertilizer on the soil environment of crop plants. Adv Agron. 2019;153:121–73. [Google Scholar]
185. Nussler K. Inflammation, synthase and inducible nitric oxide. J Leukoc Biol. 1993;54:171–8. [Google Scholar] [PubMed]
186. Spannhake EW, Reddy SP, Jacoby DB, Yu XY, Saatian B, Tian J. Synergism between rhinovirus infection and oxidant pollutant exposure enhances airway epithelial cell cytokine production. EHP. 2002;110(7):665–70. [Google Scholar] [PubMed]
187. Achakulwisut P, Brauer M, Hystad P, Anenberg SC. Global, national, and urban burdens of paediatric asthma incidence attributable to ambient NO2 pollution: estimates from global datasets. Lancet Planet Health. 2019;3(4):e166–78. [Google Scholar] [PubMed]
188. Harris E, Yu L, Wang YP, Mohn J, Henne S, Bai E, et al. Warming and redistribution of nitrogen inputs drive an increase in terrestrial nitrous oxide emission factor. Nat Commun. 2022;13(1):4310. [Google Scholar] [PubMed]
189. Shu X, Wang Y, Wang Y, Ma Y, Men M, Zheng Y, et al. Response of soil N2O emission and nitrogen utilization to organic matter in the wheat and maize rotation system. Sci Rep. 2021;11(1):4396. [Google Scholar] [PubMed]
190. Shurpali NJ, Rannik Ü, Jokinen S, Lind S, Biasi C, Mammarella I, et al. Neglecting diurnal variations leads to uncertainties in terrestrial nitrous oxide emissions. Sci Rep. 2016;6(1):25739. [Google Scholar] [PubMed]
191. Samoli E, Aga E, Touloumi G, Nisiotis K, Forsberg B, Lefranc A, et al. Short-term effects of nitrogen dioxide on mortality: an analysis within the APHEA project. Eur Respir J. 2006;27(6):1129–38. [Google Scholar] [PubMed]
192. Soule ME, Simberloff D. What do genetics and ecology tell us about the design of nature reserves? Bio Conservat. 1986;35(1):19–40. [Google Scholar]
193. Hu Y, Zhang K, Li Y, Sun Y, Li H, Yang G. Human activities increase the nitrogen in surface water on the eastern loess plateau. Geofluids. 2021;2021:1–9. [Google Scholar]
194. Hu J. Human alteration of the nitrogen cycle and its impact on the environment. IOP Conf Ser Earth Environ Sci. 2018b;178(1):8–13. [Google Scholar]
195. Pashaei R, Zahedipour-Sheshglani P, Dzingelevičienė R, Abbasi S, Rees RM. Effects of pharmaceuticals on the nitrogen cycle in water and soil: a review. Environ Monit Assess. 2022;194(2):105. [Google Scholar] [PubMed]
196. Yadav M, Gupta P, Seth CS. Foliar application of α-lipoic acid attenuates cadmium toxicity on photosynthetic pigments and nitrogen metabolism in Solanum lycopersicum L. Acta Physiol Plant. 2022;44(11):112. [Google Scholar]
Cite This Article
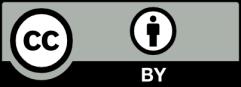
This work is licensed under a Creative Commons Attribution 4.0 International License , which permits unrestricted use, distribution, and reproduction in any medium, provided the original work is properly cited.