Open Access
ARTICLE
Phenolic Profile and Volatiles of in vitro Propagated Lavandula angustifolia Mill. Seedlings
1 Biotechnology Department, Konya Food and Agriculture University, Konya, 42080, Türkiye
2 Plant Production and Technologies Department, Konya Food and Agriculture University, Konya, 42080, Türkiye
* Corresponding Author: Dilek Killi. Email:
Phyton-International Journal of Experimental Botany 2024, 93(3), 427-444. https://doi.org/10.32604/phyton.2024.046271
Received 25 September 2023; Accepted 24 January 2024; Issue published 28 March 2024
Abstract
An effective in vitro propagation protocol was designed for Lavandula angustifolia Miller, a medicinal aromatic plant that is a prominent source of volatile organic compounds (VOCs). Murashige and Skoog media were supplemented with various concentrations of Plant Growth Regulators (PGRs), and the growth parameters of the nodal segments were examined. Nodal explants formed callus when they were supplemented with 2 mg/L of 6-Benzylaminopurine (BAP). The superior hormonal concentration of Murashige and Skoog (MS) media for the proliferation of shoots from callus cultures (39.33%) was 5 mg/L of 2-Isopentenyl adenine (2iP), and the favorable media for the growth of L. angustifolia callus cultures was 1 and 2 mg/L of BAP, with a 98% formation rate in each case. The callus cultures and in vitro propagated L. angustifolia seedlings obtained from various PGR concentrations of MS media were exposed to qualitative and quantitative analysis in terms of phenolic profiles, flavonoids, High-performance liquid chromatography (HPLC) analysis of phenolic acids, and headspace-SPME analysis for volatiles. Such analysis revealed that micropropagated seedlings grown in media containing 1 mg/L of 6-Furfurylaminopurine (KIN) accumulated the highest yield (11.95 ± 0.01 mg GAE/g) of phenolic acids. In contrast, the lowest concentration (2.17 ± 0.04 mg GAE/g) was detected in 0.5 mg/L of BAP + 0.5 mg/L of Naphthaleneacetic acid (NAA) media. The plantlets grown in 0.5 mg/L of BAP + 0.5 mg/L of NAA media showed the highest flavonoid yield (31.67 ± 0.06 μg/g QE/g). In contrast, callus samples exhibited the lowest yield (11.59 ± 0.02 μg/g QE/g) of flavonoids in MS media supplemented with a concentration of 0.5 mg/L of BAP. HPLC analysis revealed the variability of phenolic acid contents within the callus cultures as well as plantlets, with gallic acid, 4-OH benzoic acid, chlorogenic acid, vanillic acid, caffeic acid, cinnamic acid, and rosmarinic acid being the prominent constituents. The presence of twenty-two chemicals was revealed by headspace-SPME analysis. Eucalyptol, nonanal, borneol, carvone, and β-caryophyllene were the most abundant. This study demonstrated that micropropagation of L. angustifolia may be an effective method to produce large numbers of genetically identical plantlets for the production of high-value bio compounds.Keywords
The genus Lavandula is a member of the Lamiaceae Lindl. family (mint) and has been used for therapy and other purposes for about 2500 years. It comprises 39 different species, multiple hybrids, and around 400 different known varieties [1]. Most of them are highly appreciated in the fragrance, pharmaceutical, food, and flavor sectors due to their scent, and therapeutic characteristics [2] The Lamiaceae family contains over 240 genera and approximately 7200 species [3]. Among them, Lavandula angustifolia is considered one of the abundant sources of organic compounds and volatile acids, which includes over 100 ingredients that may be used fresh or dried, including 1%–6% volatile oils (monoterpenic compounds, alcohols, and esters), triterpenic acids, coumarins, flavones, resins, and polyphenols [4]. Lavender is primarily indigenous to the Mediterranean region, the Arabian Peninsula, Russia, and Africa [5]. Due to its extensive geographical distribution, and decorative, medicinal, and aromatic characteristics, this species may contribute significantly to the economies of a large number of countries. Bulgaria, France, and China are the world’s major lavender oil suppliers. However, numerous other nations have recently increased output significantly [6]. Pharmacological uses of Lavandula, such as the essential oils and extracts, are widely documented due to their antiseptic, antifungal, diuretic, antispasmodic, analgesic, sedative, carminative, hypotensive, antimicrobial, and general tonic effects [7].
Like most other fragrant plants, lavender plants can be reproduced both generatively and vegetatively. Certain varieties of lavender can only be multiplied vegetatively by shoot cuttings, while others may be multiplied more quickly and easily by employing both techniques. Therefore, micropropagation is considered one of the best ways to reproduce these kinds of plants as well as produce pathogen-free seedlings [8]. The in vitro availability of PGRs such as auxin and cytokinin is critical for callus formation. Light, darkness, and temperature, on the other hand, are all elements that influence callus formation. Calluses can range in color from green to light green, cream, white, and brown and can be categorized into subgroups based on their macroscopic properties [9].
In recent times, there has been an increasing trend toward employing pharmaceutical plants in the fields of perfumery and cosmetics. Hence, the employment of micropropagation techniques that facilitate the swift generation of plants presents itself as a feasible substitute to the traditional in vivo propagation methods. Furthermore, the significance of these techniques is deemed critical in the preservation of uncommon, threatened, and valuable botanical specimens that exhibit medicinal and economic significance. Micropropagation uses aseptic in vitro culture methods to create new plants from plant components such as roots, stems, anthers, shoots, pollen, seeds, organs, embryos, explants, calluses, single cells, or protoplasts. The study conducted by [10] explored the potential of micropropagation for various lavender species and cultivars under in vitro conditions. The findings indicated that both vegetative and generative propagations can be utilized for the propagation of lavender plants [11]. Plant growth regulators (PGRs) are known to perform diverse and significant roles in the growth and development of plants, including but not limited to germination, stem elongation, leaf growth and development, flowering, fruit set and growth, and ripening [12]. The significance of their involvement in micropropagation procedures cannot be overstated, as they play a crucial role in the initiation and growth of plants from explants. According to [13], the occurrence of secondary metabolites in plants is typically limited to specific organs and is also associated with a particular developmental stage of the plant. Moreover, the functionality of secondary metabolites is of utmost importance for the adaptability and conformity of plants to their surroundings [14]. The pharmaceutical, food, and cosmetic industries have increasingly utilized secondary metabolites due to the rise in population and subsequent surge in demand. The conventional techniques employed for the synthesis and procurement of these crucial metabolites appeared inadequate to cater to the elevated requisites of the populace. In recent years, the biotechnological approach has emerged as a viable alternative for the rapid production of secondary metabolites as a solution to the aforementioned issue.
Linalol, linalool acetate, and lavandulyl acetate are the main chemicals identified in L. angustifolia essential oils [15]. The amount of linalyl acetate was reported at around 47.56%, linalool at 28.06%, lavandulyl acetate at 4.34%, and α-terpineol 3.75%, representing 97.81% of the total identified oils [16]. The first isolation of four new compounds (4-hydroxybenzoic acid, methyl-3-(2-hydroxy-4-methoxyphenyl) propanoate, methyl caffeate, and 7-Methoxycoumarin) from L. angustifolia is reported in the literature by [17]. Since then, in vitro essential oil production of L. angustifolia from other sources employing different methods has been reported [1,7,16,18–24].
Plant tissue culture methodologies provide an innovative technique for micropropagation that avoids collecting and transporting plants from their natural settings. Since L. angustifolia is highly prominent in nutraceutical, therapeutic, and pharmaceutical characteristics, its VOCs are currently proficiently used in cosmetics, perfume, and pharmaceuticals as well as in other industries. Our study investigated the micropropagation possibilities of nodal explants of L. angustifolia Mill. Also, we looked at the phenolic profile, volatiles, and phenolic acids of the in vitro-propagated seedlings. We used high-performance liquid chromatography (HPLC) to separate compound elements and headspace-SPME to determine the volatile contents of both dried callus and seedling samples.
The aims of the study were:
i) To develop an indirect organogenesis micropropagation protocol for the L. angustifolia Mill. using nodal explant types.
ii) To determine the phenolic profiles and volatiles of in vitro propagated seedlings and callus cultures of L. angustifolia.
Nodal segments of healthy, 4- to 5-year-old L. angustifolia “Sevtopolis” seedlings were used as explants obtained during their flowering season from the fields of Konya Food and Agriculture University (37°52′ 34.5″N, 32°28′27.1″E). The study was carried out in the laboratory of the Department of Biotechnology at Konya Food and Agriculture University between 2021 and 2022.
2.2 Surface Sterilization and Preparation of Culture Conditions
The nodes were taken as explants and cleaned under running tap water for a few minutes. The nodes were then immersed in 70% ethanol (EtOH) for 1 min, and then submerged in a solution containing 20% sodium hypochlorite (NaOCl) + 80% sterile water for 15 min while being agitated occasionally. The sterilizing operation was completed according to the protocol followed by [25] and subsequently by cleaning the nodes three times in the sterile cabinet with sterile water. Murashige and Skoog (MS) media solidified with 5 g/L agar and supplemented with several concentrations and combinations of PGRs such as 6-Benzylaminopurine (BAP), 6-Furfurylaminopurine (KIN), Isopentenyl adenine (2iP), KIN + 2,4-Dichlorophenoxyacetic acid (2.4 D) + active carbon, and Thidiazuron (TDZ) were used to determine the most suitable media for callus initiation, development, and shoot proliferation. The pH of the media was adjusted to 5.8 using 1 molar NaOH and 1 normal KOH before autoclaving at 121°C for 20 min. All cultures were incubated at 24°C ± 1°C under white fluorescent lights for a 16 h light photoperiod with a photosynthetic photon flux density of 50 μmol/m2 s.
Nodal explants were aseptically cultured on MS media fortified with six different PGRs; BAP (2 mg/L), BAP (5 mg/L), KIN + 2.4D (2 mg/L), KIN (5 mg/L), 2iP (5 mg/L) and 2iP (10 mg/L), including 5 g/L agar. The experiment was carried out in ten triplets, with each combination including 15 culture containers. After four weeks of incubation, a fraction of responding explants were observed. After three subcultures, fresh calli (100 g) from each callus line were harvested and lyophilized. The resulting powdered material (ca. 5 g) was pooled and divided into three portions for phenolics analysis.
In vitro-initiated callus cultures of L. angustifolia from the callus induction stage were used as explants to find out how different concentrations of MS media with different PGRs affect the growth and proliferation parameters. The excised, soft, creamy, and friable types of callus with a length of approximately 5 mm were aseptically cultured on MS media, including control media and twelve further different combinations and concentrations of PGRs as follows: 1, 2.5, and 5 mg/L of KIN, 0.5, 1, 5, and 10 mg/L of BAP, 0.25 and 2 mg/L of TDZ, 5, 10, and 20 mg/L of 2iP. The experiment was carried out in ten sets of three replicates (ten explants per magenta), and the observations were made after four weeks of incubation.
To evaluate the effects of different combinations of auxin and cytokinin, in vitro multiplied seedlings (approx. 1 cm shoots) from the shoot proliferation stage were sub-cultured into three dissimilar MS media as follows: 0.5 mg/L of BAP + 0.5 mg/L of IAA, 0.5 mg/L of BAP + 0.5 mg/L of IBA, and 0.5 mg/L of BAP + 0.5 mg/L of NAA. In the majority of micro-propagation studies, auxins are preferred at higher concentrations and cytokinins at lower levels. Each combination was sub-cultured three times over the course of four weeks. After each subculture, growth metrics were observed.
2.6 Total Phenolic Content (TPC, F-C Assay)
Analyses of the phenolic contents of 17 samples (Table 1), including callus and plantlets from the callus stage, were conducted according to the Folin-Ciocalteu method performed by [26]. Samples were dried and then ground to a fine powder using a grinder. Following that, 4 mg/mL of dried callus and plantlet samples were dissolved in an HPLC-grade methanol solution by vortexing now and then. 1.25 mL of liquid reagent was mixed with 1.25 mL of DI water (DW) to make 50% Folin-Ciocalteu. After that, samples were filtered using a PVDF/L syringe filter with a 25-mm diameter and 0.45-m pore size. 2.5 g of Na2CO3 was weighed and then dissolved in 50 mL of DW to make 5% Na2CO3. For calibration, a 0.1% gallic acid stock solution was made with 2 mg of gallic acid powder and 2 mL of HPLC-grade methanol. Six gallic acid concentrations 0, 20, 40, 60, 80, and 100 µg/mL were prepared. For each concentration, 30 µL of gallic acid, 30 µL of methanol, 150 µL of DW, and 15 µL of Folin reagent were added to a well in a 96-well microplate. After waiting for 5 min, the reaction was initiated by adding 30 µL of 5% Na2CO3. Each concentration tube included 30 µL of gallic acid, which was mixed with 30 µL of methanol, 150 µL of DW, and 15 µL of Folin reagent. After 5 min, the reaction was started by adding 30 µL of 5% Na2CO3. The absorbance of each dilution reaction was measured at 725 nm (in triplicate) after a 60-min incubation in the dark at room temperature using multimode microplate reader equipment. The gallic acid standard curve was formed after this method was repeated for each concentration (Fig. 1).
Figure 1: Gallic acid equivalent (μg/mL) dry weight of both callus and plantlets samples. Each histogram is the mean ± S.E. of n = 3. Different letters above histograms indicate significant differences at p ≤ 0.05
2.7 Total Flavonoid Content (TFC)
The aluminum chloride colorimetric method, with minor modifications, was adapted from [27]. Initially, a 10% solution of AlCl3.6.H2O was prepared. Teng of AlCl3.6.H2O was dissolved in 100 mL of methanol for this purpose. The standard curve was then created by generating several concentrations of quercetin (12.5, 25, 37.5, 50, 62.5, 75, 87.5, and 100 g/mL) using methanol. Material preparation was done by weighing 10 mg from each of the 17 treatments and dissolving it in 2 mL of MeOH with occasional vortexing. The plant extract concentration was fixed at 5 mg/mL. The materials were then filtered via a PVDF/L syringe filter with a 25-mm diameter and 0.45 m pore size. The flavonoid levels in the 17 samples were determined using the Quercetin Calibration Chart data [28]. Following that, 500 mL of each plant extract was collected in test tubes, and 1500 mL of MeOH, 100 mL of 10% AlCl3.6.H2O, and 2800 mL DW were individually added to each tube. Following 30 min of incubation at room temperature, the absorbance of the reaction solution was measured at 415 nm using a HITACHI U-5100 UV/V spectrophotometer-Tokyo, Japan (each measured in triplicate).
High-performance liquid chromatography (HPLC) analyses of L. angustifolia callus cultures and in vitro propagated plantlets (Tables 2 and 3) were performed using a Waters e2695 Alliance system, which included an LC-20AT pump, a DGU-20A5 online degasser, a SIL-20A autosampler, an ACE 5 C18 2504,6 mm id 5 m column oven, and a 2489 UV/V is detector. The separation was carried out on a Zorbax Eclipse XDB-C8 analytical column (250, 4.6, 5 m) at 40°C. A (2% acetic acid), B (50% ACN: 50% acetic acid (0.5% acetic acid), and C made up the mobile phase (acetonitrile). As solvents, methanol and H2O (50:50) were utilized. The flow rate was 1.2 mL per min. Phenolic detection and quantification were carried out at 280 nm of UV light (Table 4).
2.9 Volatile Component Analysis
The headspace-SPME method was used to analyze volatile components. Originally, 10 g of plant material from five groups (BAP + IAA, 2iP, KIN, BAP + NAA, and BAP + IBA) were combined in a Waring mixer for three minutes (without the addition of water). 1 g of the plant material was sealed with septa in 10-mL headspace vials. SPME headspace volatiles were collected for 30 min on a Supelco 57348 2 cm, 50/30 m DVB/Carboxen/PDMS Stable-Flex fiber. The fiber was preconditioned at 250°C for 10 min. The period of 30 min was chosen since it produced the greatest number of compounds without affecting composition quality. After sampling, the SPME device was put into the GC injector for the 62-min GC analysis using an RTX-5M column. Headspace analysis was conducted with the assistance of Karadeniz Technical University.
In our study, the determination of appropriate concentrations of PGRs for callus induction and shoot formation in tissue culture is guided by a thorough examination of relevant scholarly publications and empirical observations. The chemicals (Agar, PGRs, etc.) utilized in this study were obtained from DUCHEFA-The Netherlands. For chemical analysis, all solvents were purchased from Sigma.
The utilization of nodal segments with various concentrations of PGRs; TDZ and BAP has proven to be an effective method for in vitro propagation of Lavandula. Consequently, this approach demonstrates a consistent and uniform development while also exerting an influence on the proliferation of shoots and the rooting of plantlets [10].
Previous studies have indicated that lower concentrations of BAP are effective in shoot multiplication rates, proliferation, and initiating callus cultures of L. angustifolia [29,30], while higher concentrations of cytokinin and auxins may lead to the highest leaf length growth [31]. TDZ concentrations of 0.25 and 2 mg/L were selected based on their reported efficacy in shoot induction [25] and [32].
Data was collected for examination of TPC and TFC contents (Table 5), and experiments were repeated three times. Table data are reported as mean ± standard errors (SE). Using the acquired data, a one-way analysis of variance (ANOVA) was performed. To identify homogeneous groups, the Duncan post-hoc test was utilized. The statistically significant difference was determined using a p value of p ≤ 0.05. The statistical analyses were carried out using IBM SPSS Statistics 22 (IBM SPSS, Chicago, IL, USA).
After four weeks of incubation, observations on growth parameters indicated that friable type callus development (Fig. 2) had occurred in two vessels (3%) of MS media containing 5 g/L agar supplemented with 2 mg/L of BAP (Table 6). Under either full darkness of the incubation room, the control and other treatments showed no improvement (0%).
Figure 2: Friable callus development from the L. angustifolia nodal segment
The first shoot was initiated in MS media enriched with 5 mg/L of BAP (Fig. 3). Table 7 contains findings on contamination, browning, shoot induction, and callus formation percentages, following three successful subcultures in MS media. The number of shoots per explant varied between five to twelve.
Figure 3: Shoot callus formation in 5 mg/L of BAP before (A) and after (B) four weeks of incubation
Table 7 shows the micropropagation, callus formation, and browning rates from 150 cultured explants. Fifty-nine of the 150 cultured callus grew shoots, which means that 5 mg/L of 2iP is the best medium for the growth of shoots from L. angustifolia callus cultures. Callus explants in 1 and 2 mg/L of BAP were reported in 147 out of 150 explants (98%), with this treatment being the optimal medium for L. angustifolia callus development and survival. The 5 mg/L of 2iP (39.33%) followed by 1 mg/L of KIN (30.67%) proved to be the ideal media for micropropagation of L. angustifolia from callus cultures. Treatments of 0.5, 1, and 10 mg/L of BAP had no influence (0%) on the micropropagation rate of explants. It was revealed that 1 and 2 mg/L of BAP treatments (98%) favorably influenced callus formation, but 20 mg/L of 2iP (20%) was shown to be an unfavorable medium for callus formation. Browning occurred at a significant incidence (63.33%) in 2 mg/L of TDZ, while no browning was observed in 2 mg/L of BAP media (0% in all trials).
The medium supplemented with 0.5 mg/L of BAP + 0.5 mg/L of IAA, 0.5 mg/L of BAP + 0.5 mg/L of IBA, and 0.5 mg/L of BAP + 0.5 mg/L of NAA concentrations of auxin and cytokinin did not affect plantlet root development (Fig. 4) after three subcultures (four weeks of incubation each). During the rooting stage, the plantlets exhibited continued growth, with the tallest plantlet measuring 9 cm in a growth media containing 0.5 mg/L of BAP and 0.5 mg/L of IBA.
Figure 4: In vitro propagated seedlings obtained from several media. (A) No root development was seen in media containing 0.5 mg/L BAP + 0.5 mg/L NAA. The tallest plantlet was 3 cm tall. (B) No root development was seen in media containing 0.5 mg/L BAP + 0.5 mg/L IAA. The tallest plantlet stood 1.5 cm tall. (C) No root development was seen in media containing 1 mg/L KIN. (D) No root development was seen in media supplemented with 10 mg/L 2iP. The tallest plantlet was 1 cm tall. (E) No root development was seen in 0.5 mg/L BAP + 0.5 mg/L IBA. The higher mean height of the plantlets in this media (9 cm) indicated the most effective media for the shoot growth of L. angustifolia
3.4 Total Phenolic Content (TPC, F-C Assay) and Total Flavonoid Content (TFC)
Total Phenolic Content (TPC) and Total Flavonoid Content (TFC) analysis of callus samples indicates that plants propagated on the control media exhibited the greatest phenolic content (6.32 ± 0.08 mg/g). In contrast, the cultures grown in 0.5 mg/L of BAP + 0.5 mg/L of NAA provided the lowest amount of phenolic content (2.17 ± 0.04 mg/g).
Plantlets propagated on the 1 mg/L of KIN and control growth media medium showed the greatest flavonoid content yield (21.38 ± 0.06 µg/g). The lowest quantity of flavonoid content was obtained from plantlets cultivated on the growth media that included 0.5 mg/L of BAP (11.59 ± 0.02 µg/g). TPC and TFC analysis of plantlet samples (Table 5) revealed that 1 mg/L of KIN media had the greatest phenolic content (11.95 ± 0.01 mg/g). On the other hand, growth media supplemented with 20 mg/L of 2iP produced plants containing the lowest phenolic content (2.24 ± 0.002 mg/g). Plantlets propagated in media containing 0.5 mg/L of BAP + 0.5 mg/L of NAA exhibited the maximum flavonoid content (31.67 ± 0.06 µg/g). In contrast, plantlets propagated on the growth media containing 10 mg/L of BAP had the lowest levels of flavonoid content (14.93 ± 0.04 µg/g).
High-performance liquid chromatography (HPLC) findings for callus cultures were determined quantitatively using 11 standards. The maximum concentration of gallic acid was obtained in extracts of dried callus cultures of L. angustifolia grown on MS control media (310 μg/g), followed by 1 mg/L of KIN (303 μg/g), and the lowest concentration was observed from extracts of 0.5 mg/L of BAP + 0.5 mg/L of NAA media (64 μg/g). On the other hand, compared to the other treatments the highest yields (324 μg/g) of 4-OH Benzoic acid were extracted from callus cultures grown on 0.5 mg/L of TDZ media, followed by 0.5 mg/L of BAP (290 μg/g) media, while the lowest quantity was obtained from cultures cultivated on 5 mg/L of 2iP (8 μg/g) media. The maximum concentration of chlorogenic acid was obtained from callus cultures developed in the control media (78 μg/g), while the lowest was observed in those from 0.5 mg/L of TDZ (1 μg/g) media. There was no chlorogenic acid discovered in calluses from the medium containing 0.5 mg/L of BAP + 0.5 mg/L of IBA and 0.5 mg/L of BAP + 0.5 mg/L of IAA. Vanillic acid was another important extracted component, with the maximum amount supplied by the callus cultures propagated in media containing 0.5 mg/L of 2iP (224 μg/g), while the lowest amount was provided by the cultures obtained from 0.5 mg/L of BAP and 0.5 mg/L of BAP + 0.5 mg/L of NAA medium, giving 4 μg/g. The maximum quantity of caffeic acid (92 μg/g) was exhibited by the callus cultures grown in 0.5 mg/L of BAP + 0.5 mg/L of NAA media, whereas the lowest amount was produced by callus cultures grown in 0.5 mg/L of BAP + 0.5 mg/L of IBA (3 μg/g) media. There was no caffeic acid found in the medium 0.5 mg/L of TDZ, 0.5 mg/L of BAP + 0.5 mg/L of IAA, 5 mg/L of 2iP, and 1 mg/L of KIN of the seedlings. Cinnamic acid levels were greatest in the calluses propagated in 1 mg/L of KIN (5 μg/g), followed by 5 mg/L of 2iP and 0.5 mg/L of BAP + 0.5 mg/L of IBA (2 μg/g) media. The cultures in control, 0.5 mg/L of BAP, 2 mg/L of BAP, 0.5 mg/L of BAP + 0.5 mg/L of NAA, 0.5 mg/L of TDZ, and 10 mg/L of KIN medium supplied no cinnamic acid. Rosmarinic acid was found at its maximum concentration in callus cultures cultivated on 0.5 mg/L of BAP + 0.5 mg/L of IBA (22 μg/g) media. In comparison, the smallest level was identified in calluses propagated 10 mg/L of KIN (4 μg/g) media. There was no rosmarinic acid found in the cultures propagated in 0.5 mg/L of BAP + 0.5 mg/L of NAA, 0.5 mg/L of TDZ, 5 mg/L of 2iP, and 1 mg/L of KIN medium. In our study, the optimal media for producing benzoic acid from L. angustifolia callus cultures was determined to be 0.5 mg/L of BAP + 0.5 mg/L of NAA (265 μg/g) (Table 2).
Table 3 displays findings on HPLC results for in vitro-propagated plantlets. The study used an HPLC instrument to analyze 11 standards, with the maximum output of gallic acid observed in extracts of dried in vitro propagated plantlets of L. angustifolia grown on MS media with 20 mg/L of 2iP (118 μg/g), followed by 10 mg/L of BAP (60 μg/g). In contrast, the plantlets grown in 0.5 mg/L of BAP + 0.5 mg/L of IBA yielded the least quantity (3 μg/g). The findings, on the other hand, demonstrate that 41 µg/g of 4-OH Benzoic acid was obtained from plantlets grown in 10 mg/L of BAP medium, followed by 1 mg/L of KIN (33 μg/g), which displays the greatest yields compared to others. The lowest quantity was observed from plantlets grown in 0.5 mg/L of BAP + 0.5 mg/L of IAA (7 μg/g). The quantity of chlorogenic acid in the list is significant due to the production of the greatest rate from the seedlings grown in the 0.5 mg/L of BAP + 0.5 mg/L of IBA medium, which yielded 1603 μg/g. In contrast, plantlets grown in 20 mg/L of 2iP media only generated 5 μg/g. Chlorogenic acid was not detected in the seedlings grown in the 10 mg/L of BAP media. The maximum production of vanillic acid (513 μg/g) was obtained from plantlets grown in 0.5 mg/L of BAP + 0.5 mg/L of IBA, while the lowest was found in plantlets grown in 10 mg/L of BAP (160 μg/g). Meanwhile, plantlets grown in 1 mg/L of KIN media accounted for the most caffeic acid (59 μg/g), whereas the plantlets grown in 0.5 mg/L of BAP + 0.5 mg/L of IAA (19 μg/g) accounted for the least. This means that no caffeic acid was detected in the seedlings propagated in 10 mg/L of BAP.
3.6 Volatile Component Analysis
A total of 21 components were isolated from L. angustifolia plantlets grown in vitro (Table 8). The highest concentration of eucalyptol was found in plantlets cultivated on 5 mg/L of 2iP (5.32%). The lowest yield was generated by the plantlets propagated in 0.5 mg/L of BAP + 0.5 mg/L of NAA media, which produced 2.8%. Results indicate that the MS medium containing 0.5 mg/L of BAP, and 0.5 mg/L of IAA (0%) have no effect on eucalyptol production in L. angustifolia calluses/plantlets. In contrast, the highest nonanal concentration was found in plantlets cultivated on 5 mg/L of BAP (4.11%), while the lowest concentration was found in plantlets grown on 1 mg/L of KIN (2.18%). The results indicate that plantlets grown in 0.5 mg/L of BAP + 0.5 mg/L of IAA and 0.5 mg/L of BAP + 0.5 mg/L of NAA did not accumulate nonanal. The highest concentration of Borneol was found in plantlets cultivated on 0.5 mg/L of BAP + 0.5 mg/L of NAA media (11.90%), while the lowest concentration was found in plantlets grown in 1 mg/L of KIN media (3.65%). Carvone yield was highest at 22.78% seedlings grown in 0.5 mg/L of BAP + 0.5 mg/L of IAA. In contrast, the lowest concentration was found in seedlings obtained from the 5 mg/L of 2iP media, which accounted for 6.92% of the total. Among the various trials, β-Caryophyllene emerged as the most prevalent component. Notably, the highest yield of this compound was obtained from plantlets in the presence of 1 mg/L of KIN media, constituting 30.74% of the total output. Conversely, the lowest production of β-Caryophyllene was recorded in the trial involving plantlets grown in 0.5 mg/L of BAP + 0.5 mg/L of IBA, amounting to 14.90%. In contrast to the aforementioned findings, the presence of β-Caryophyllene was not detected in plantlets cultivated on 0.5 mg/L of BAP and 0.5 mg/L of IAA. The study found that certain volatile components were present in the in vitro propagated plantlets of L. angustifolia. The identified components accounted for varying percentages in different concentrations of PGRs, such as BAP+IAA, 2iP, KIN, BAP+NAA, and BAP+IBA. The percentages ranged from 0.59% to 20.63%, as shown in Table 8.
The present investigation focused on the in vitro propagation of L. angustifolia Mill., a significant aromatic and medicinal plant that serves as the primary origin of various volatile organic compounds (VOCs). The micropropagation was conducted under aseptic conditions, and the impact of various plant growth regulators on callus induction, shoot formation, and root formation was documented. This study investigated the total phenolic content (TPC), total flavonoid content (TFC), and high-performance liquid chromatography (HPLC) analyses of callus and in vitro propagated plantlets, as well as the volatile profile of plantlets.
Reference [33] conducted a comprehensive study into the long-term micropropagation of five distinct cultivars of L. angustifolia. The researchers examined morphogenesis, and physiological, and biochemical parameters across a span of nine passages. The study elucidates the disparities in shoot multiplication indices across different cultivars, variations in water content, levels of photosynthetic activity, and enzyme activity. Both our study and the study conducted by [33] highlight the efficacy of in vitro propagation. However, they diverge in terms of their respective emphases. Our study primarily focuses on the potential for bio-compound production, whereas reference [33] provided a comprehensive examination of physiological and biochemical factors, such as water content, proline, ascorbic acid, and enzyme activities. Both studies offer significant contributions to the field of L. angustifolia micropropagation, albeit from distinct perspectives, thereby enhancing the comprehensive understanding of the process.
According to [34], the utilization of BAP as a PGR exhibits promising outcomes for the propagation of L. angustifolia. Reference [35] found that the most effective combination for the proliferation of callus cultures was MS media supplemented with 8.88 µM (1 mg/L) BAP + 5.36 µM (1 mg/L) NAA (92%). The research conducted by [36] demonstrated the favorable impact of 2,4-D on the generation of callus in L. angustifolia. Moreover, it was proposed that subjecting leaf explants to a concentration of 2 mg/L of 2,4-D and 2 mg/L of BAP under conditions of darkness may serve as a viable method for inducing callus and promoting its growth. Our study‘s findings indicate that BAP media was identified as the optimal media for the growth and development of L. angustifolia callus cultures, whereas 2iP media demonstrated the highest efficacy for the propagation of shoots from callus cultures of L. angustifolia. The findings about shoot proliferation in our study are consistent with those reported by [37].
The issue of oxidative browning in the callus culture of L. angustifolia hindered the formation and survival of callus cultures. According to [38], this issue is widely recognized as one of the most significant and pressing obstacles encountered in the realm of plant tissue cultures. The findings of our study indicate that the MS media, which was supplemented with 2 mg/L of TDZ and 20 mg/L of 2iP, was the most effective medium for reducing oxidative browning in the callus cultures of L. angustifolia. Reference [38] proposed the use of antioxidants, such as citric acid at a concentration of 10–50 mg/L or ascorbic acid at a concentration of 20–100 mg/L, as a means of mitigating the reduction in browning intensity observed in callus cultures. Reference [35] suggested that the leaf explants of L. angustifolia are a suitable option for indirect organogenesis regeneration due to their ability to cause less phenolic oxidation and contamination.
Our investigations have delineated that varying concentrations of auxin and cytokinin media did not exert any influence on the development of roots in plantlets, even following three successive subcultures, each of which lasted for a duration of four weeks. In contrast, the plantlets in the rooting phase exhibited growth, whereas no instances of root development were detected across all experimental groups subjected to the rooting media treatments. In terms of the initiation of L. angustifolia roots, the study by [39] highlighted a favorable outcome, achieved through the use of half-strength modified MS basal media, which was supplemented with 30 g/L sucrose and varying concentrations of IAA, IBA, and NAA. The utilization of half-strength MS and extended plantlet maintenance were not feasible in our investigation. Consequently, it is plausible to assert that these circumstances could hurt the rooting phases of our research.
Reference [22] conducted a study that underscored that the callus culture of L. officinalis water extracts in 0.5 mg/L of BAP + 0.5 mg/L of NAA media had the highest phenolic content (35.74 ± 0.48 mg GAE/g). In their study, the growth media that was enriched with 0.5 mg/L of KIN + 2 mg/L of 2,4-D yielded the lowest quantity of 24.22 ± 1.03 mg GAE/g. In contrast, the media containing 0.5 mg/L of BAP + 1 mg/L of 2,4-D exhibited the highest level of flavonoid contents, measuring at 32.42 ± 0.46 mg QE/g, while the media enriched with 0.5 mg/L of BAP + 2 mg/L of NAA yielded the minimum flavonoid content (11.64 ± 0.80 mg QE/g). In contrast to our investigation, it was observed that the control media exhibited the highest level of phenolic accumulation among the callus samples, while the lowest level was recorded in the media containing 0.5 mg/L of BAP and 0.5 mg/L of NAA. The growth media containing a combination of 0.5 mg/L of BAP resulted in the lowest flavonoid contents in the plantlets, while plants developed on the control medium and growth media with 1 mg/L of KIN recorded the highest flavonoid contents.
Our findings regarding HPLC analysis of callus cultures and in vitro propagated plantlet samples indicate that the maximum concentration of rosmarinic acid was observed solely in 1 mg/L of KIN media, with a value of 68 µ/g, as determined from the dried samples. The minimum value was derived from desiccated callus culture specimens that comprised a blend of 10 mg/L of KIN media. The findings demonstrate that the incorporation of various concentrations of BAP and NAA, TDZ, 2iP, and KIN into the media did not yield any significant impact on the synthesis of rosmarinic acid in the callus cultures of L. angustifolia. Additionally, it was observed that the in vitro propagated plantlets of L. angustifolia, treated with 0.5 mg/L of BAP + 0.5 mg/L of IAA, 10 mg/L of BAP, and 5 mg/L of 2iP (0%), did not exhibit any presence of rosmarinic acid. Upon examination of [40] research, it is apparent that the reported rosmarinic acid levels in Lavandula spp. are inconsistent, with the highest amount being documented as 3.0–3.3 mg/g (3000–3300 µ/g). Our study revealed that the dried plantlet samples exhibited the highest concentration of rosmarinic acid at 0.068 mg/g (68 µ/g) when grown in 1 mg/L of KIN media.
Our study investigated the volatile components of L. angustifolia plantlets cultivated on five different types of media. The results revealed that the major components of the L. angustifolia plantlets were eucalyptol (5.32%) in 5 mg/L of 2iP, nonanal (4.11%) in 5 mg/L of 2iP, borneol (11.90%) in 0.5 mg/L of BAP + 0.5 mg/L of NAA, carvone (22.78%) in 0.5 mg/L of BAP + 0.5 mg/L of IAA, and β-Caryophyllene (30.74%) in 1 mg/L of KIN media, respectively. The aforementioned quantities exhibit a significant disparity when compared to the findings of [41]’s research, where the eucalyptol content in the fresh lavender mixture was found to be 12.08% as per the tabulated data.
Reference [42] discovered that Lavandula x intermedia essential oil exhibits variations in the concentrations of linalool, linalyl acetate, and camphor, with values of 34.8 (43.3%), 4.35 (42.5%), and 7.27 (12.5%), respectively. Furthermore, the research conducted by [43] revealed that the primary components present in L. viridis samples grown in vivo were 1,8-cineole (74.0%, 51.9%, and 57.8%) and camphor (2.9%, 15.3%, and 8.7%), respectively. The abovementioned pointed out the in vitro production of valuable plant metabolites such as linalool and linalyl acetate. However, our results show that such metabolites cannot be produced in vitro, at least in the early stage of plant growth. This reality is also valid for phenolic production at lower quantities.
Our findings suggest that somaclonal variation in plant tissue culture operations can have significant implications. It has been observed that in vitro settings impacted the biochemical profile of essential oils in callus cultures and in vitro propagated plantlets of L. angustifolia, as the HPLC analysis revealed a lower frequency of chemicals in propagated plantlets and callus cultures as well as a lack of production of major phenolic compounds.
This study has shown a successful indirect micropropagation protocol for L. angustifolia Mill. This is evident by examining the several concentrations of PGRs in MS media. Furthermore, by conducting HPLC and headspace-SPME analysis of both dried callus cultures and micro-propagated plantlets, the effect of somaclonal variations in vitro settings was observed. These effects are evident in the chemical composition of the plantlets, which will determine their value as a source of nutraceuticals and the viability of the plants when grown under field or greenhouse conditions where biotic stress may occur.
Acknowledgement: None.
Funding Statement: The authors received no specific funding for this study.
Author Contributions: The authors confirm contribution to the paper as follows: study conception and design: Sökmen, A., data collection: Yahya, M. A., Tunali, F., analysis and interpretation of results: Killi, D., Sökmen, A., Yahya, M. A., Tunali, F., draft manuscript preparation: Killi, D., Yahya, M. A., Tunali, F., Sökmen, A. All authors reviewed the results and approved the final version of the manuscript.
Availability of Data and Materials: All data is available in the figures and tables presented in the manuscript.
Ethics Approval: None.
Conflicts of Interest: The authors declare that they have no conflicts of interest to report regarding the present study.
References
1. Upson T, Andrews S. The genus Lavandula. 1st edUSA: Timber Press, Inc.; 2004. [Google Scholar]
2. Martins RDP, Gomes RADS, Malpass ACG, Okura MH. Chemical characterization of Lavandula dentata L. essential oils grown in uberaba-mg. Ciência Rural. 2019;49:e20180964. [Google Scholar]
3. Wu S, Sun Y, Niu G, Pantoja GLG, Rocha AC. Responses of six Lamiaceae landscape species to saline water irrigation. J Environ Hortic. 2016;34(1):30–35. [Google Scholar]
4. Chrysargyris A, Panayiotou C, Tzortzakis N. Nitrogen and phosphorus levels affected plant growth, essential oil composition and antioxidant status of lavender plant (Lavandula angustifolia Mill.). Ind Crops Prod. 2016;83:577–86. [Google Scholar]
5. Basch E, Foppa I, Liebowitz R, Nelson J, Smith M, Sollars D, et al. Lavender (Lavandula angustifolia Miller). J Herb Pharmacother. 2004;4(2):63–78. [Google Scholar]
6. Giray FH. An analysis of world lavender oil markets and lessons for Turkey. J Essent Oil Bear Pl. 2018;21:1612–23. [Google Scholar]
7. Uritu CM, Mihai CT, Stanciu GD, Dodi G, Alexa-Stratulat T, Luca A, et al. Medicinal plants of the family Lamiaceae in pain therapy: a review. Pain Res Manag. 2018;7801543. [Google Scholar]
8. Hopkins William G. Plant biotechnology. Philadelphia, PA: Infobase Publishing; 2007. [Google Scholar]
9. Ikeuchi M, Sugimoto K, Iwase A. Plant callus: mechanisms of induction and repression. Plant Cell. 2013;25:3159–73. [Google Scholar] [PubMed]
10. Andrade LB, Echeverrigaray S, Fracaro F, Pauletti GF, Rota L. The effect of growth regulators on shoot propagation and rooting of common lavender (Lavandula vera DC). Plant Cell, Tissue Organ Cult. 1999;56:79–83. [Google Scholar]
11. George EF, Hall MA, de Klerk GJ. Micropropagation: uses and methods. In: Plant propagation by tissue culture. Dordrecht: Springer; 2008. p. 29–64. [Google Scholar]
12. Gaba VP. Plant growth regulators in plant tissue culture and development. In: Plant development and biotechnology. Boca Raton, FL: CRC Press; 2005. p. 87–99. [Google Scholar]
13. Güven A, Gürsul İ. Bitki Doku Kültürlerinde Sekonder Metabolit Sentezi. GIDA. 2014;39(5):299–306 (In Turkish). [Google Scholar]
14. Erb M, Kliebenstein DJ. Plant secondary metabolites as defenses, regulators, and primary metabolites: the blurred functional trichotomy. Plant Physiol. 2020;184(1):39–52. [Google Scholar] [PubMed]
15. D’Auria FD, Tecca M, Strippoli V, Salvatore G, Battinelli L, Mazzanti G. Antifungal activity of Lavandula angustifolia essential oil against Candida albicans yeast and mycelial form. Med Mycol. 2005;43(5):391–6. [Google Scholar] [PubMed]
16. Verma RS, Rahman LU, Chanotiya CS, Verma RK, Chauhan A, Yadav A, et al. Essential oil composition of Lavandula angustifolia Mill. cultivated in the mid hills of Uttarakhand, India. J Serb Chem Soc. 2010;75(3):343–8. [Google Scholar]
17. Yadikar N, Bobakulov KM, Eshbakova KA, Aisa HA. Phenolic compounds from Lavandula angustifolia. Chem Nat Compd. 2017;53:562–4. [Google Scholar]
18. Smigielski K, Raj A, Krosowiak K, Gruska R. Chemical composition of the essential oil of Lavandula angustifolia cultivated in Poland. J Essent Oil Bear Pl. 2009;12:338–47. [Google Scholar]
19. Blažeković B, Yang W, Wang Y, Li C, Kindl M, Pepeljnjak S, et al. Chemical composition, antimicrobial and antioxidant activities of essential oils of Lavandula × intermedia ‘Budrovka’ and L. angustifolia cultivated in Croatia. Ind Crops Prod. 2018;123:173–82. [Google Scholar]
20. Küçük S, Çetintaş E, Kürkçüoğlu M. Volatile compounds of the Lavandula angustifolia Mill. (Lamiaceae) species cultured in Turkey. J Turk Chem Soc A: Chem. 2018;5:1303–8. [Google Scholar]
21. Yadikar N, Bobakulov K, Li G, Aisa HA. Seven new phenolic compounds from Lavandula angustifolia. Phytochem Lett. 2018;23:149–54. [Google Scholar]
22. Buran A, Topdemir A. Phenolic and flavonoid amounts and antioxidant capacity of Lavandula officinalis (lavender) callus grown in different growth regulator combinations. Anatol J botan. 2022;6:115–21. [Google Scholar]
23. Lema-Rumińska J, Kulus D, Tymoszuk A, Varejão JM, Bahcevandziev K. Profile of secondary metabolites and genetic stability analysis in new lines of Echinacea purpurea (L.) Moench micropropagated via somatic embryogenesis. Ind Crops Prod. 2019;142:111851. [Google Scholar]
24. Lema-Rumińska J, Sadowska K, Tymoszuk A, Andrzejewska J. Scutellarin and other metabolites as well as morphological and molecular characterization of the Scutellaria barbata lines from in vitro and in vivo cultivation. Ind Crops Prod. 2023;195:116464. [Google Scholar]
25. Hamza AM, El-Kafie A, Omaima M, Kasem MM. Direct micropropagation of English lavender (Lavandula angustifolia Munstead) plant. J Plant Prod. 2011;2(1):81–96. [Google Scholar]
26. Zambrano C, Kotogán A, Bencsik O, Papp T, Vágvölgyi C, Mondal KC, et al. Mobilization of phenolic antioxidants from grape, apple and pitahaya residues via solid state fungal fermentation and carbohydrase treatment. LWT. 2018;89:457–65. [Google Scholar]
27. Woisky R, Salatino A. Analysis of propolis: some parameters and procedures for chemical quality control. J Apic Res. 1998;37:99–105. [Google Scholar]
28. Lamaison J, Carnart A. Teneurs en principaux falvonoïdes des fleurs et des feuilles de Crataegus monogyna Jacq. et de Crataegus laevigata (Poiret) DC. en fonction de la période de végétation. 1991;25(1):12–6 (In French). [Google Scholar]
29. Yegorova N, Kruglova N, Galin I, Stavtzeva I. Induction of morphogenesis in the callus culture of Lavandula angustifolia Mill. BIO Web Conf. 2020;24:98. [Google Scholar]
30. Al-Bakhit A, Sawwan J, Al-Mahmoud M. In vitro propagation of two Lavandula species: Lavandula angustifolia and Lavandula latifolia L. Medica. Jordan J Agric Sci. 2007;3(1). [Google Scholar]
31. Parkash V, Singh H. Lavandula angustifolia L. (Lavenderan important aromatic medicinal shrub and its in vitro micro-propagation for conservation. J Agric Sci Technol. 2013;9:691–702. [Google Scholar]
32. Dewir YH, Nurmansyah, Naidoo Y, Teixeira da Silva JA. Thidiazuron-induced abnormalities in plant tissue cultures. Plant Cell Rep. 2018;37:1451–70. [Google Scholar] [PubMed]
33. Yegorova NA, Mitrofanova IV, Brailko VA, Grebennikova OA, Paliy AE, Stavtseva IV. Morphogenetic, physiological, and biochemical features of lavandula angustifolia at long-term micropropagation in vitro. Russ J Plant Physiol. 2019;66:326–34. [Google Scholar]
34. Pistelli L, Noccioli C, D’Angiolillo F, Pistelli L. Composition of volatile in micropropagated and field grown aromatic plants from Tuscany Islands. Acta Biochim Pol. 2013;60(1):43–50. [Google Scholar] [PubMed]
35. Devasigamani L, Devarajan R, Loganathan R, Rafath H, Padman M, MV GR, et al. Regeneración de plantas de Lavandula angustifolia L. a partir de callos formados de explantes de hojas in vitro como estrategia de conservación. Biotecnología Vegetal. 2020;20(2):75–82 (In Spanish). [Google Scholar]
36. Keykha F, Khadem A, Bagheri A, Sharifi A, Ameri M. Optimization of Lavender (Lavandula angustifolia) callus culture. Plant Tissue Cult Biotechnol. 2014;24:279. [Google Scholar]
37. Prasad A, Shukla SP, Mathur A, Chanotiya CS, Mathur AK. Genetic fidelity of long-term micropropagated Lavandula officinalis Chaix.: an important aromatic medicinal plant. Plant Cell Tissue Organ Cult. 2015;120:803–11. [Google Scholar]
38. Miri SM. Re: is browning of the callus a problem? 2018. Available from: https://www.researchgate.net/post/Is-browning-of-the-callus-a-problem [Accessed 2023]. [Google Scholar]
39. Sabzevar TS, Ghavidel AR, Foroghian S. The effect of phytohormones on Lavender (Lavandula angustifolia Mill.) organogenesis. J Pharm Pharmacol. 2015;3:338–44. [Google Scholar]
40. Sik B, Kapcsándi V, Székelyhidi R, Hanczné EL, Ajtony Z. Recent advances in the analysis of rosmarinic acid from herbs in the lamiaceae family. Nat Prod Commun. 2019;14(7):1934578X19864216. [Google Scholar]
41. Yilmaz MA. Essential oil composition of Lavandin (Lavandula x intermedia) cultivated in Bismil-Turkey. Acad Perspect Proc. 2018;1:1120–5. [Google Scholar]
42. Nimet K, Baydar H. Türkiye’de Lavanta Üretim Merkezi Olan Isparta İli Kuyucak Yöresi Lavantalarının (Lavandula x intermedia Emeric ex Loisel.) Uçucu Yağ Özellikleri. Selcuk J Agric Food Sci. 2011;25(4):42–6 (In Turkish). [Google Scholar]
43. Gonçalves S, Serra H, Nogueira JMF, Almeida R, Custódio L, Romano A. Headspace-SPME of in vitro shoot-cultures and micropropagated plants of Lavandula viridis. Biol Plant. 2008;52:133–6. [Google Scholar]
Cite This Article
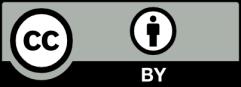
This work is licensed under a Creative Commons Attribution 4.0 International License , which permits unrestricted use, distribution, and reproduction in any medium, provided the original work is properly cited.