Open Access
ARTICLE
Effects of Inoculation with Phosphate Solubilizing Bacteria on the Physiology, Biochemistry, and Expression of Genes Related to the Protective Enzyme System of Fritillaria taipaiensis P. Y. Li
1 College of Pharmacy, Chengdu University of Traditional Chinese Medicine, Chengdu, 611130, China
2 College of Pharmacy, Dali University, Dali, 671000, China
3 College of Food and Biological Engineering, Chongqing Three Gorges University, Chongqing, 404120, China
4 Diqing Tibetan Autonomous Prefecture Institute of Plateau Biology, Diqing, 674400, China
5 Chongqing Institute of Medicinal Plant Cultivation, Chongqing, 408435, China
* Corresponding Authors: Guangzhi Wang. Email: ; Liang Han. Email:
; Nong Zhou. Email:
Phyton-International Journal of Experimental Botany 2024, 93(2), 247-260. https://doi.org/10.32604/phyton.2024.046452
Received 01 October 2023; Accepted 04 January 2024; Issue published 27 February 2024
Abstract
Fritillaria taipaiensis P. Y. Li is a widely used medicinal herb in treating pulmonary diseases. In recent years, its wild resources have become scarce, and the demand for efficient artificial cultivation has significantly increased. This article is the first to apply phosphate solubilizing bacteria isolated from the rhizosphere soil of F. taipaiensis P. Y. Li to the cultivation process of F. taipaiensis P. Y. Li. The aim is to identify suitable reference strains for the artificial cultivation and industrial development of F. taipaiensis P. Y. Li by examining the effects of various phosphate solubilizing bacteria and their combinations on photosynthesis, physiological and biochemical properties, and gene expression related to the protective enzyme system in F. taipaiensis P. Y. Li. The experiment, conducted in pots at room temperature, included a control group (CK) and groups inoculated with inorganic phosphorus-solubilizing bacteria: W1 (Bacillus cereus), W2 (Serratia plymuthica), W12 (Bacillus cereus and Serratia plymuthica), and groups inoculated with organophosphorus-solubilizing bacteria: Y1 (Bacillus cereus), Y2 (Bacillus cereus), Y12 (Bacillus cereus and Bacillus cereus), totaling seven groups. Compared to CK, most growth indices in the bacterial addition groups showed significant differences, with W12 achieving the highest values in all indices except the leaf area index. The content of photosynthetic pigments, photosynthetic parameters, and osmoregulatory substances increased variably in each bacterial treatment group. W12 exhibited the highest content of chlorophyll a and soluble protein, while W1 had the highest free proline content. The activities of peroxidase (POD), superoxide dismutase (SOD), and catalase (CAT) in all inoculated groups were higher than in CK, with significant changes in SOD and CAT activities. The malondialdehyde (MDA) content in all inoculated groups was lower than in CK, with Y12 being the lowest, at approximately 30% of CK. Gene expression corresponding to these three enzymes also increased variably, with POD expression in Y2 being the highest at 2.73 times that of CK. SOD and CAT expression in Y12 were the highest, at 1.84 and 4.39 times that of CK, respectively. These results indicate that inoculating phosphate solubilizing bacteria can enhance the growth of F. taipaiensis P. Y. Li, with the mixed inoculation groups W12 and Y12 demonstrating superior effects. This lays a theoretical foundation for selecting bacterial fertilizers in the cultivation process of F. taipaiensis P. Y. Li.Keywords
Fritillaria taipaiensis P. Y. Li, a dried bulb of the Liliaceae Fritillaria plant, is listed as a source for the medicinal Fritillaria cirrhosa in the 2020 edition of the Chinese Pharmacopoeia [1]. This plant is characterized by its unique combination of bitter and sweet flavors and a slightly cold nature. It is known for its medicinal properties, such as alleviating coughs, facilitating expectoration, reducing heat, and moistening the lungs, making it widely used in treating pulmonary diseases like lung-heat cough and cough-phlegm imbalance [2]. Since its successful cultivation in 1983, the associated cultivation techniques have become mature [3]. However, despite ongoing research into its medicinal, nutritional, and economic benefits, a persistent imbalance remains between the growing market demand and the limited supply. While efficient cultivation methods can help alleviate this scarcity to some extent, the scientific application of fertilizers and the improvement of soil quality in cultivation areas are crucial for optimizing the yield and quality of these medicinal herbs.
Phosphorus, a crucial nutrient for plant development, is involved in the structural composition of cellular components and various metabolic pathways. The majority of China’s arable land is high in total phosphorus content, yet 95% of this phosphorus exists in forms not readily absorbed by plants. This leads to a deficiency of soil-available phosphorus on about 74% of China’s cultivated land [4]. Moreover, prolonged overuse of chemical fertilizers can cause soil compaction, leading to reduced fertility, soil quality, and health, and escalating concerns about the quality and safety of agricultural products [5]. Phosphate solubilizing microorganisms (PSMs), essential components of the soil ecosystem, can convert insoluble phosphorus into forms absorbable by plants, thereby increasing the effective phosphorus content of the soil [6]. These PSMs include phosphate solubilizing bacteria (PSB), fungi (PSF), and actinomycetes (PSA), with bacteria representing a significant portion of total PSMs [7]. Phosphate solubilizing bacteria exhibit diverse species and solubilization mechanisms. Some species dissolve insoluble soil phosphate through the secretion of organic acids, aiding in the dissolution of mineral phosphorus like calcium phosphate and apatite [8]. Others secrete metabolites like phosphatase, nuclease, and phytase, activating organic phosphorus [9]. Additionally, certain bacterial strains secrete iron carriers that chelate metal ions and form complexes, facilitating phosphate availability for plant uptake and promoting plant growth [10].
Research on phosphate solubilizing bacteria, including species such as Bacillus, Pseudomonas, Burkholderia, Serratia, and Flavobacterium, has gained prominence in recent years [11]. Based on the substrate they decompose, these bacteria are classified into inorganic and organophosphorus solubilizing categories. The use of phosphate solubilizing bacteria to modulate plant growth has become a focal point of research; however, most studies are limited to crops, with relatively few exploring Chinese herbal medicine [12–16]. Mu [17] investigated the impact of exogenous arbuscular mycorrhiza (AM) fungi, potassium-solubilizing bacteria, and phosphate solubilizing bacteria on the mycorrhizal colonization rate of F. taipaiensis P. Y. Li roots and the medicinal quality, providing a basis for using beneficial microorganisms in F. taipaiensis P. Y. Li’s inter-root zone and enhancing the bulbs’ medicinal quality. Zhang et al. [18] studied the effects of staggered planting and increased application of mycorrhizal fertilizers on the photosynthetic characteristics, yield, and quality of F. taipaiensis P. Y. Li, contributing to the experimental foundation for its high-quality and high-yield cultivation. However, these studies mainly focused on exogenous strains, leaving the interactions between beneficial microorganisms isolated from the inter-root soil of F. taipaiensis P. Y. Li and the plant itself largely unexplored.
In this experiment, two strains of inorganic phosphorus-solubilizing bacteria and two strains of organophosphorus-solubilizing bacteria were selectively isolated from the rhizosphere soil of F. taipaiensis P. Y. Li. These strains were inoculated into pots containing four-year-old bulbs of F. taipaiensis P. Y. Li to investigate the effects of phosphorus-dissolving bacterial strains on the plant’s growth and development. Key observational parameters included the photosynthetic characteristics of F. taipaiensis P. Y. Li leaves, essential physiological and biochemical indices, and differential gene expression in the protective enzyme system. This study aimed to identify the most effective phosphate solubilizing bacterial strains or synergistic bacterial combinations for the cultivation of F. taipaiensis P. Y. Li, thereby providing a scientific basis for future development and production strategies.
This study utilized four-year-old bulbs of F. taipaiensis P. Y. Li, scientifically identified by Professor Zhou Nong from Chongqing Three Gorges College, belonging to the Liliaceae family. These bulbs were cultivated in Wuxi County, Chongqing Municipality (N31°36′26.07″, E108°49′18.75″), with a rigorous selection process ensuring uniformity in size and quality.
The test strains included dominant phosphate solubilizing bacteria, isolated from the rhizosphere soil of F. taipaiensis P. Y. Li from ten different locations across China. The dilution coating plate method was employed for separation. For inorganic phosphorus bacteria, the culture medium used was (g/L: glucose 10, (NH4)2SO4 0.5, yeast extract 0.5, NaCl 0.3, KCl 0.3, MgSO4 0.3, MnSO4 0.03, FeSO4 0.03, Ca3 (PO4)2 5.0, agar 15.0) (Product number: HB8670, Manufacturer: Qingdao Hope Biotechnology Co., Ltd., China) and organic phosphorus bacteria culture medium (g/L: glucose 10, (NH4)2SO4 0.5, Yeast Extract Powder 0.5, NaCl 0.3, KCl 0.3, MgSO4 0.3, MnSO4 0.03, FeSO4 0.03, CaCO3 1.0, lecithin 0.2, Agar 15.0) (Product number: HB8673, Manufacturer: Qingdao Hope Biotechnology Co., Ltd.) The selection was based on the formation of clear phosphorus solubilizing circles. A second screening used an inorganic phosphorus bacteria culture medium without agar (Product number: HB8670-1, Manufacturer: Qingdao Hope Biotechnology Co., Ltd.) (substituting calcium phosphate with equal amounts of apatite, aluminum phosphate, and zinc phosphate hydrate) to evaluate solubility in different inorganic phosphorus sources. For organic phosphorus bacteria, an agar-free medium (Product number: HB8673-1, Manufacturer: Qingdao Hope Biotechnology Co., Ltd.) with sucrose and starch replacing glucose was used to assess lecithin solubility under different carbon sources. The strains identified for preliminary research included two inorganic phosphorus-solubilizing strains, Bacillus cereus and Serratia plymuthica, and two organic phosphorus-solubilizing strains, both Bacillus cereus. The test soil, comprising a blend of yellow loam, river sand, and organic fertilizer (2:1:1 ratio), was obtained from Chongqing Three Gorges College. It was sieved through a 2 mm mesh, sterilized at 121°C for 2 h, and rested for 7 days before use.
The pot-based experiment commenced in October 2022 at Xiliuxi, Group III, Hongqiba Community, Wuxi County, Chongqing Municipality. This habitat cultivation experiment involved positioning pots on the rooftops of local farmers’ houses. The pots used had a bottom diameter of 18 cm, a top diameter (caliber) of 19.8 cm, and a height of 20 cm. Before use, each pot was thoroughly cleaned with 75% alcohol three times. Each pot contained five bulbs of F. taipaiensis P. Y. Li, spaced uniformly. The experiment included 10 parallel replicates for each treatment group, as detailed in Table 1. Groups W1, W2, and W12 were designated for inoculation with inorganic phosphorus bacteria, while groups Y1, Y2, and Y12 were set for inoculation with organophosphorus bacteria (noting that Y1 and Y2, both Bacillus cereus, differ in their ability to dissolve lecithin when the carbon source changes, with their overall ability being higher than other strains. Hence, each strain was studied both as a single bacterial agent and in combination for their inoculation effects). Pre-cultivated stable phosphorus-dissolving bacterial strains were prepared into inoculants by adjusting the bacterial suspension to 2 × 108 CFU/mL using sterile water, with sterile water alone used as a control. The inoculation occurred in two stages: the first in early March 2023, following the germination of the F. taipaiensis P. Y. Li bulbs, and the second 30 days after the first inoculation. Each inoculation consisted of 20 mL of bacterial suspension per live seedling (10 mL/plant for each bacterial treatment in the mixed inoculation groups, applied after mixing). Routine management practices specific to F. taipaiensis and P. Y. Li cultivation were followed throughout the experiment.
2.3 Measurement Indicators and Methods
In May 2023, pest and disease-free samples of F. taipaiensis P. Y. Li were selected for evaluating various indices.
To enhance the robustness of the experimental results, measurements were conducted on the rhizosphere soil of each group. The average soil pH for the CK was 7.59, while for the other groups, it ranged between 7.473 and 7.683. The soil pH in the W12 and Y12 groups was slightly higher than in CK, whereas it was lower in the other groups. Regarding total phosphorus content, the CK group’s rhizosphere soil had 1019.681 mg/kg, while the other groups ranged from 985.78 to 1121.406 mg/kg. Only Y1 had a lower total phosphorus content than CK, while the others were higher. In terms of available phosphorus content, CK’s soil contained 57.131 mg/kg, whereas the other groups ranged from 67.984 to 85.803 mg/kg, significantly higher than CK’s. The plate counting method was utilized to count microorganisms in the rhizosphere soil of each group. CK had a bacterial count of 0.309 × 107 CFU/g, while the other groups ranged from 2.188 × 107 CFU/g to 3.477 × 107 CFU/g, all significantly higher than CK. These results indicate the effects of applying phosphorus-degrading bacteria, providing a foundation for subsequent experiments.
2.3.1 Determination of Growth Indicators
For this part, 6 pots were randomly selected from each treatment group (comprising 10 pots), with one plant per pot chosen for growth indicator assessment. The leaf count of each plant was recorded, and dimensions like height, stem thickness, and leaf thickness were measured using vernier calipers or rulers. A leaf was randomly selected from each plant, and after reaching full expansion, the leaf area of F. taipaiensis P. Y. Li was measured using a portable laser leaf area scanner (CI-203; CID, USA) without the need for leaf detachment.
2.3.2 Measurement of Photosynthesis Parameters
Photosynthetic parameters were measured using a photosynthesis meter (GFS-3000; WALZ, Germany) the under optimal conditions: a bright, cloud-free day during peak stomatal opening hours (11:00–13:00). From each of the six pots in a group, one leaf of F. taipaiensis P. Y. Li was selected to assess the net photosynthetic rate (Pn), stomatal conductance (Gs), intercellular carbon dioxide concentration (Ci), and transpiration rate (Tr), following the method outlined by Zhao et al. [19]. Additionally, stomatal limiting values (Ls) were calculated using the formula Ls = 1−Ci/Ca, where Ci is the intercellular CO2 concentration, and Ca is the atmospheric CO2 concentration [20]. After these measurements, the sampled leaves were collected and taken to the laboratory for further analysis of physiological and biochemical indices.
2.3.3 Determination of Physiological and Biochemical Indexes
The content of photosynthetic pigments was measured using UV spectrophotometry (UV-2450, Shimadzu, Japan), adhering to light-avoidance protocols throughout the process [21]. Enzymatic activities within the leaf’s protective enzyme system were determined following the methodology described by Zhang et al. [22]. Peroxidase (POD) activity was gauged using the guaiacol method, where an increase of 0.01 in absorbance at 470 nm per minute was considered as one unit of enzyme activity. Catalase (CAT) activity was measured via UV spectrophotometry, defining a unit of enzyme activity as a 0.1 decrease in absorbance at 240 nm (A240nm). Superoxide dismutase (SOD) activity was determined by the nitro blue tetrazolium photochemical reduction method, with one unit of enzyme activity being 50% inhibition of NBT photochemical reduction. Additionally, Malondialdehyde (MDA) and soluble sugar contents were assessed using the thiobarbituric acid colorimetric method. The soluble protein content was determined using the Thomas Brilliant Blue method, and free proline was quantified by UV spectrophotometry (UV-2450; Shimadzu, Japan).
2.3.4 Analysis of Differential Gene Expression of Leaf Protective Enzyme System
RNA extraction and purification from the leaves of F. taipaiensis P. Y. Li were performed using the TRIzol® Plus RNA Purification Kit (product number: 12183-555, Invitrogen, USA) and the RNase-Free DNase Set (product number: 79254, Qiagen, Germany). The quality and quantity of RNA were then evaluated by UV spectrophotometry, focusing on the absorbance ratio (OD260/280 and OD260/230), and confirmed by 1.2% agarose gel electrophoresis. Subsequently, cDNA synthesis was carried out according to the protocol provided by the SuperScript™ III First-Strand Synthesis SuperMix for qRT-PCR (product number: 11752-050, Invitrogen, USA). Target genes were identified from the genomic data of F. taipaiensis P. Y. Li available on NCBI, with primers designed using Primer Premier 6.0 (6.0 version, Premier, Canada) and Beacon Designer 7.8 software (7.8 version, Premier Biosoft, USA). ISPH was selected as the internal reference gene. The primers used are listed in Table 2. qRT-PCR amplification was performed using the Power SYBR® Green PCR Master Mix kit (product number: 4913914001, Roche, Switzerland), with the reaction conditions set as 95°C for 1 minute, followed by 40 cycles of 95°C for 15 s and 63°C for 25 s, with fluorescence collection, and concluding with a melting point curve analysis from 55°C to 95°C.
Each sample underwent triplicate analysis, and the relative expression level of each gene was statistically analyzed by 2 (Ct internal reference gene−Ct target gene) (2−ΔΔCt) [23,24].
The experimental data were processed utilizing Microsoft Excel 2019 (2019 version, Microsoft, USA), and subsequent statistical analyses were performed employing SPSS 23.0 (23.0 version, IBM, USA) and Origin 2023 (2023 version, OriginLab, USA) software packages.
3.1 Effect of Inoculation with Phosphate Solubilizing Bacteria on the Growth Indexes of F. taipaiensis P. Y. Li
The application of individual phosphate solubilizing bacteria and their combined inoculation had a significant impact on the growth of F. taipaiensis P. Y. Li. Different treatments resulted in varied growth indices (Table 3). Compared to the control group (CK), the treatment groups, where bacteria were added, showed considerable improvement in growth indices. In terms of plant height, stem thickness, and leaf thickness, all bacteria-supplemented groups exceeded the performance of CK. The W12 group, which received a combined application of two types of inorganic phosphorus-solubilizing bacteria, displayed the highest values in all indices except leaf area, indicating that such combined inoculation effectively enhances the growth of F. taipaiensis P. Y. Li. Notably, the effect of mixed application was more pronounced than that of single inoculation.
3.2 Effect of Inoculation with Phosphate Solubilizing Bacteria on Photosynthetic Parameters of F. taipaiensis P. Y. Li
The photosynthetic indices of F. taipaiensis P. Y. Li leaves, inoculated with various phosphate solubilizing bacteria and their combinations, showed significant variation (Table 4). Except for the Ci in groups W2 and Y1, which was lower than in CK, the Pn, Gs, Ci, and Tr values of the other treatment groups were superior to CK, with most exhibiting significant variability. Specifically, the Pn and Tr were highest in the W1 group, being 2.3 and 4.6 times higher than CK, respectively. G and Ls reached their peak in the Y1 group, with values 16 and 1.07 times greater than CK, respectively. The Ci was highest in the W12 group, being 1.13 times higher than in CK.
3.3 Effect of Inoculation with Phosphate Solubilizing Bacteria on Photosynthetic Pigment Content of F. taipaiensis P. Y. Li
According to Table 5, the phosphate solubilizing bacteria had varying effects on the photosynthetic pigment content of F. taipaiensis P. Y. Li. Group W12 showed the highest chlorophyll content, while W1 led in chlorophyll b content. Both W12 and Y12 were dominant in carotenoid content. The W2 group exhibited the highest chlorophyll a/b ratio, and W1 had the highest total chlorophyll content. Comparing single and mixed inoculation groups, W12 had higher chlorophyll a and carotenoid contents than W1 and W2. Among the organophosphorus solubilizing bacteria treatments, Y12 showed significantly higher chlorophyll a, carotenoids, and total chlorophyll content compared to Y1 and Y2. Most indices in the bacteria-supplemented groups surpassed those of the CK, confirming the beneficial impact of phosphate solubilizing bacteria on photosynthetic pigments.
3.4 Effect of Inoculation with Phosphate Solubilizing Bacteria on Protective Enzyme System and MDA Content of F. taipaiensis P. Y. Li
As shown in Table 6, peroxidase (POD) activity in all treatment groups was higher than in the control group (CK), with the highest activity observed in Y2, indicating a 1.35-fold increase compared to CK (p < 0.05). The POD activity in the W12 group was significantly higher than in W1 and W2 (p < 0.05). In the organophosphorus solubilizing bacteria treatments, Y12 had higher POD activity than Y1 but lower than Y2. Superoxide dismutase (SOD) activity showed substantial improvement (p < 0.05) in all treatment groups compared to CK, with the highest activity in W12. Both inorganic and organophosphorus solubilizing bacteria treatments, as well as mixed treatments (W12 and Y12), exhibited elevated SOD activity compared to single-species treatments. Catalase (CAT) activity significantly increased in all treatment groups compared to CK (p < 0.05), with Y12 displaying the highest activity, 1.83 times greater than CK. The malondialdehyde (MDA) content in leaves of F. taipaiensis P. Y. Li was lower in all bacterial treatment groups compared to CK. The mixed inoculation groups showed lower MDA content than single inoculation groups, with Y12 reaching the lowest level, approximately 30% of CK.
3.5 Effect of Inoculation with Phosphate Solubilizing Bacteria on Soluble Sugars and Proteins and Free Proline Content of F. taipaiensis P. Y. Li
According to Table 7, with a few exceptions, the inoculation with phosphate solubilizing bacteria generally led to an increase in the contents of soluble sugars, proteins, and free proline in F. taipaiensis P. Y. Li compared to CK. Specifically, the W2 group showed the highest soluble sugar content, while W12 exhibited the most significant increase in soluble protein content, and W1 led in free proline content. These increases were 1.24, 1.23, and 1.46 times higher than CK, respectively.
Specifically, the W2 group showed the highest soluble sugar content, while W12 exhibited the most significant increase in soluble protein content, and W1 led in free proline content. These increases were 1.24, 1.23, and 1.46 times higher than CK, respectively.
3.6 qRT-PCR Analysis of Genes Related to Leaf Protective Enzyme System under Different Treatments
The quantitative reverse transcription polymerase chain reaction (qRT-PCR) analyses, as shown in Fig. 1, focused on three genes essential to the leaf-protective enzyme system of F. taipaiensis P. Y. Li. The results indicated enhanced expression of peroxidase (POD), superoxide dismutase (SOD), and catalase (CAT) genes in all treated groups compared to CK. The highest expression of POD was observed in Y2, being 2.73-fold greater than CK. For SOD and CAT, the highest expressions were found in Y12, being 1.84 and 4.39 times that of CK, respectively. Comparing mixed bacterial inoculations with single bacterial additions, apart from the POD expression in Y12 being lower than in Y2, the mixed groups generally showed higher enzyme gene expressions than their corresponding single addition counterparts. Among the mixed treatment groups, Y12 surpassed W12 in the expression of all three enzyme genes. Overall, the use of phosphorus-dissolving bacteria enhanced the expression of POD, SOD, and CAT genes related to the leaf protective enzyme system in F. taipaiensis P. Y. Li, with the groups treated with organophosphorus solubilizing bacteria exhibiting particularly notable improvements.
Figure 1: Differential expression of genes related to the protective enzyme system in leaves of F. taipaiensis P. Y. Li under different treatments
Note: Comparisons were made for the same variable under different treatment groups,different lowercase letters upon column indicate significant differences at 0.05 level.
The correlation analysis between physiological and biochemical indices and the relative expression of POD, SOD, and CAT genes in F. taipaiensis P. Y. Li leaves were presented in Fig. 2. The analysis revealed several significant correlations: The expression of SOD was highly and positively correlated with CAT (r = 0.984, p < 0.01). POD expression showed a significant positive correlation with both CAT (r = 0.814, p < 0.05) and SOD (r = 0.848, p < 0.05). Carotenoid content was positively correlated with POD expressions (r = 0.705, p < 0.05), and also showed positive correlations with the relative expression of SOD (r = 0.823, p < 0.05). SOD activity correlated positively with carotenoid content (r = 0.802, p < 0.05). A substantial positive correlation was observed between POD activity and soluble protein content (r = 0.766, p < 0.05).
Figure 2: Correlation analysis between different indicators
Note: Red in the figure indicates positive correlation, blue indicates negative correlation, and numbers indicate correlation coefficients.
The impact of phosphate solubilizing microorganisms on plant growth is a significant area of study, primarily due to their ability to solubilize insoluble soil phosphorus, enhance the efficiency of phosphorus fertilizers, and increase phosphorus uptake in crops. This process not only improves the utilization of phosphorus fertilizers but also potentially leads to an increase in crop yields [25]. Among these microorganisms, phosphorus-solubilizing bacteria form a notable subgroup, making them a focus of scientific research.
Photosynthesis is crucial for plant growth and biomass accumulation, as it provides the necessary energy for these processes [26]. Studies have indicated that microbial fertilizers can improve plant photosynthetic characteristics, enhance photosynthetic efficiency, and promote the accumulation of photosynthesis products [27,28]. These enhancements are often reflected in improved agronomic traits like biomass, plant height, and stem thickness [29,30]. Leaves, being the primary sites of photosynthesis and crucial organs for plant growth, directly influence photosynthetic intensity. The size of the leaf area is particularly important as it determines the light-exposed area and consequently affects plant growth and development in its environment [31]. In this study, except for the Y12 group, all treated groups had a larger leaf area than CK, and other growth indices such as plant height, stem thickness, number of leaves, and leaf thickness were also higher.
The efficiency of plant photosynthesis and its intensity are closely linked to the interaction of various factors, including Pn, Gs, Ci, and others. Stomatal limitation on photosynthesis can vary; in some cases, an increase in Gs and Ci leads to a higher photosynthetic rate, reducing stomatal limitation. In other cases, a decrease in both Gs and Ci results in a reduced photosynthetic rate and increased stomatal limitation [32]. In this study, it was observed that only the Ci values of W2 and Y1 were lower than CK, with their stomatal limitation values slightly higher than CK. Conversely, all other groups surpassed CK in these indices. This suggests that the use of phosphorus-dissolving bacteria may reduce stomatal limitations, thereby enhancing the plant’s photosynthetic rate.
Chlorophyll, crucial for light absorption in photosynthesis, and carotenoids, essential in transferring light energy to chlorophyll, play significant roles in determining the photosynthetic intensity of plants [33,34]. This study observed that most treatment groups exhibited higher chlorophyll content compared to CK, indicating that the application of phosphate solubilizing bacteria can increase the content of photosynthetic pigments, enhance light capture, and consequently improve the plant’s photosynthetic capacity.
Soluble sugars, soluble proteins, and free proline are important osmoregulatory substances in plants. They contribute to osmoregulation, protect enzymatic activities like CAT and POD, stabilize proteins, and maintain essential physiological processes including photosynthesis [35]. In this study, increases in the content of soluble sugars were observed in three groups, soluble proteins in five groups, and free proline in all treated groups compared to CK. These findings highlight the biochemical effects of phosphorus-dissolving bacteria on plant physiology.
SOD, POD, and CAT form a crucial enzyme defense system in plants, protecting against damage from reactive oxygen species and other peroxide radicals [36]. The activities of these enzymes are linked to a plant’s ability to withstand stress. In this research, the activities of POD, SOD, and CAT in all treated groups exceeded those in CK, with mixed-addition groups showing higher enzyme activities than their single-addition counterparts. This observation is supported by qRT-PCR results, which indicated higher relative expression of these enzymes in the treatment groups compared to CK, and also demonstrated interactions among these enzymes. MDA is a byproduct of cellular membrane lipid peroxidation and serves as an indicator of membrane damage and a plant’s ability to repair itself [37]. The study revealed that MDA content in all treated groups was lower than in CK, with the most significant reduction observed in the mixed bacterial addition group. This suggests that the use of phosphorus-dissolving bacteria can reduce the damage caused by lipid peroxidation in plant membranes, thereby supporting normal plant growth and health.
The burgeoning field of rhizosphere microbiome research has unveiled its profound potential to influence plant health and development, as evidenced by several studies on Fritillaria species. Mu et al. [38] explored the relationship between rhizosphere microorganisms and alkaloid content in F. taipaiensis P. Y. Li. They found that as the plant aged, microbial diversity in the rhizosphere soil decreased, leading to continuous cropping challenges and reduced medicinal quality. This study laid a theoretical foundation for micro-ecological control strategies to counteract continuous cropping problems in F. taipaiensis P. Y. Li. Tang et al. [39] investigated the rhizosphere microorganisms of Fritillaria thunbergii. Their findings suggested a reciprocal interaction between the growth of F. thunbergii, soil factors, and rhizosphere microorganisms. The study highlighted the intrinsic link between bacterial species, diversity, and the accumulation of alkaloids in F. thunbergii, an aspect crucial for medicinal plant cultivation. Zhao et al. [40] isolated 23 strains of endophytic bacteria from Fritillaria ussuriensis Maxim, some of which showed potent antagonistic effects against Staphylococcus aureus. This discovery points to the rich endophytic bacterial resources in F. ussuriensis Maxim and their potential in medical applications. Yang et al. [41] focused on isolating bioprotective bacteria from Fritillaria przewalskii Maxim for rot disease control. Their research offers valuable insights for preventing and managing rot disease in F. przewalskii Maxim and related crops. With the advancement in high-throughput sequencing technology and macrogenomics, the rhizosphere microbiome, often regarded as the “second genome” of plants, has attracted significant attention. He et al. [42] proposed a concept linking microecology and the geoherbalism of traditional Chinese medicine (TCM), shedding light on the formation of geoherbalism from a TCM microecological perspective. Current research in TCM geoherbalism focuses on the influence of rhizosphere microorganisms on the formation of Dao-di herbs, particularly their secondary metabolites. However, the mechanisms underlying these interactions are still not fully understood. A comprehensive analysis of the composition of rhizosphere microorganisms and their interactions with host plants offers valuable insights. This understanding can enhance the quality of medicinal plants and promote efficient use of biological resources [43].
5.1 Application of Phosphate Solubilizing Bacteria during the Growth Process of Fritillaria taipaiensis P. Y. Li
This study concludes that applying phosphate solubilizing bacteria during the growth process of F. taipaiensis P. Y. Li significantly enhances chlorophyll content and boosts photosynthesis, a benefit that stands irrespective of stress conditions. The benefits of bacterial inoculation extend beyond these aspects to include improvements in cellular osmotic substances, the activity of protective enzymes in plants, and their genetic expressions, all showing varying degrees of enhancement. The application of phosphate solubilizing bacteria enhances nutrient accumulation in the rhizosphere, which is beneficial for the growth and biomass accumulation of F. taipaiensis P. Y. Li. Among the studied groups, W12 (containing B. cereus and S. plymuthica) and Y12 (containing two strains of B. cereus) demonstrated superior effects compared to their respective single strain additions, showing a more significant impact on plant growth. For large-scale artificial cultivation of F. taipaiensis P. Y. Li, it is suggested to consider applying these combinations of either inorganic or organic phosphorus-solubilizing bacteria. This approach not only promises to enhance production efficiency but also to preserve the ecological environment. Thus, it provides a valuable reference for advancing the industrialization of artificial cultivation practices for F. taipaiensis P. Y. Li.
Acknowledgement: Not applicable.
Funding Statement: This work was funded by Chongqing Municipal Technology Innovation and Application Development Program (cstc2020jscx-gksb0001); Yunnan Academician (Expert) Workstation Project (202105AF150073).
Author Contributions: Conceptualization, Z. Shi; methodology, F. Pan, J. Lang and N. Zhou; investigation, Z. Shi, X. Kong, G. Wang and M. Ye; writing—original draft preparation, Z. Shi and Q. Wu; writing—review and editing, L. Han and N. Zhou; supervision, N. Zhou. All authors have read and agreed to the published version of the manuscript.
Availability of Data and Materials: All the data supporting the findings of this study are included in this article.
Ethics Approval: Not applicable.
Conflicts of Interest: The authors declared that they have no conflicts of interest to report regarding the present study.
References
1. National Pharmacopoeia Commission. Pharmacopoeia of the people’s republic of China: part I. Beijing, China: Chin Med Sci and Tech Press; 2020. p. 36. [Google Scholar]
2. Rui P, Peng M, Rang M, Nian S. Analysis of the bioactive components from different growth stages of Fritillaria taipaiensis P. Y. Li. Acta Pharm Sin B. 2023;3(3):167–73. [Google Scholar]
3. Luo M, Deng C, Li P, Tan Q, Luo S, Xu G, et al. Research progress in medicinal plant Fritillaria taipaiensis P. Y. Li. Chin Wild Plant Resour. 2021;40(2):42–56. [Google Scholar]
4. Yin Z, Shi F, Jiang H, Roberts D, Chen S, Fan B. Phosphate solubilization and promotion of maize growth by Penicillium oxalicum P4 and Aspergillus niger P85 in a calcareous soil. Can J Microbiol. 2015;61:913–23. [Google Scholar] [PubMed]
5. Qin X, Guo S, Zhai L. How long-term excessive manure application affects soil phosphorous species and risk of phosphorous loss in fluvo-aquic soil. Environ Pollut. 2020;266:115304. [Google Scholar] [PubMed]
6. Silva L, Pereira M, Carvalho A, M X, Buttrós V, Pasqual M, et al. Phosphorus-solubilizing microorganisms: a key to sustainable agriculture. Agric. 2023;13:462. [Google Scholar]
7. Tian J, Ge F, Zhang D. Roles of phosphate solubilizing microorganisms from managing soil phosphorus deficiency to mediating biogeochemical P cycle. Biol. 2021;10(2):158. [Google Scholar]
8. Andersson K, Tighe M, Guppy C, Milham P, McLaren T. Incremental acidification reveals phosphorus release dynamics in alkaline vertic soils. Geoderma. 2015;259(5):35–44. [Google Scholar]
9. Castagno L, Sannazzaro A, Gonzalez M, Pieckenstain F, Estrella M. Phosphobacteria as key actors to overcome phosphorus deficiency in plants. Ann Appl Biol. 2021;178:256–67. [Google Scholar]
10. Rawat P, Das S, Shankhdhar D. Phosphate-solubilizing microorganisms: mechanism and their role in phosphate solubilization and uptake. J Soil Sci Plant Nutr. 2021;21:49–68. [Google Scholar]
11. Hayat R, Ali S, Amara U, Khalid R, Ahmed I. Soil beneficial bacteria and their role in plant growth promotion: a review. Ann Microbiol. 2010;60(4):579–98. [Google Scholar]
12. Bakhshandeh E, Pirdashti H, Lendeh K. Phosphate and potassium-solubilizing bacteria effect on the growth of rice. Ecol Eng. 2017;103:164–9. [Google Scholar]
13. Vyas P, Gulati A. Organic acid production in vitro and plant growth promotion in maize under controlled environment by phosphate-solubilizing fluorescent Pseudomonas. BMC Microbiol. 2009;9(1):174–88. [Google Scholar] [PubMed]
14. Singh H, Reddy M. Effect of inoculation with phosphate solubilizing fungus on growth and nutrient uptake of wheat and maize plants fertilized with rock phosphate in alkaline soils. Eur J Soil Biol. 2011;47(1):30–4. [Google Scholar]
15. He D, Wan W. Phosphate-solubilizing bacterium Acinetobacter pittii gp-1 affects rhizosphere bacterial community to alleviate soil phosphorus limitation for growth of soybean (Glycine max). Front Microbiol. 2021;12:737116. [Google Scholar] [PubMed]
16. Ullah M, Daur I, Khan B. Rock phosphate and phosphorus solubilizing bacteria effect on yield and yield components of chickpea. Gesunde Pflanz. 2023;75:2889–98. [Google Scholar]
17. Mu M. Study on effect of continuous cropping obstruction of Fritillaria taipaiensis P. Y. Li and its meitigation measures. Dali University: Dali, China; 2019. [Google Scholar]
18. Zhang J, Wang X, Feng B, Zhou X, Chen H, Pan S, et al. Effects of wrong planting and fertilization on photosynthetic characteristics, yield and quality of Fritillaria taipaiensis P. Y. Li. Acta Agric Boreali-Occident Sin. 2022;31(5):628–39. [Google Scholar]
19. Zhao X, Song R, Yan X. Effects of arbuscular mycorrhizal fungal inoculation on growth and photosynthesis of Camptotheca acuminata seed lings. Chin J Plant Ecol. 2009;33(4):783–90. [Google Scholar]
20. Wei Z, Guo D, Li H, Ding B, Zhang J, Zhou N, et al. Photosynthetic parameters and physiological indexes of Paris polyphylla var. yunnanensis influenced by arbuscular mycorrhizal fungi. China J Chin Mater Med. 2015;40(20):3945–52. [Google Scholar]
21. Fernández-Marín B, García-Plazaola J, Hernández A. Plant photosynthetic pigments: methods and tricks for correct quantification and identification. In: Sánchez-Moreiras A, Reigosa M, editors. Advances in plant ecophysiology techniques. 2018. p. 29–50. [Google Scholar]
22. Favaretto F, Martinez A, Soriani H. Differential responses of antioxidant enzymes in pioneer and late-successional tropical tree species grown under sun and shade conditions. Environ Exp Bot. 2011;70(1):20–8. [Google Scholar]
23. Livak K, Schmittgen T. Analysis of relative gene expression data using real-time quantitative PCR and the 2−ΔΔCT Method. Methods. 2001;25(4):402–8. [Google Scholar] [PubMed]
24. Adnan M, Morton G, Hadi S. Analysis of rpoS and bolA gene expression under various stress-induced environments in planktonic and biofilm phase using 2−ΔΔCT method. Mol Cell Biochem. 2011;357(1–2):275–82. [Google Scholar] [PubMed]
25. Zhang Y, Chen F, Wu X, Luan F, Zhang L, Fang X. Isolation and characterization of two phosphate-solubilizing fungi from rhizosphere soil of moso bamboo and their functional capacities when exposed to different phosphorus sources and pH environments. PLoS One. 2018;13(7):e0199625. [Google Scholar] [PubMed]
26. Wang X, Liu Q, Yan B, Zhao G, Liu G. Responses of photosynthetic characteristics of Dodonaea viscosa to nitrogen and phosphorus addition in a dry–hot valley. Acta Ecol Sin. 2019;39(22):8615–29. [Google Scholar]
27. Sheteiwy S, Ahmed M, Kobae Y. The effects of microbial fertilizers application on growth, yield and some biochemical changes in the leaves and seeds of Guar (Cyamopsis tetragonoloba L.). Food Res Int. 2023;172:113122. [Google Scholar] [PubMed]
28. Wu F, Li J, Chen Y. Effects of phosphate solubilizing bacteria on the growth, photosynthesis, and nutrient uptake of Camellia oleifera abel. For. 2019;10(4):348. [Google Scholar]
29. Zeng Q, Wu X, Wen X. Identification and characterization of the rhizosphere phosphate-solubilizing bacterium Pseudomonas frederiksbergensis JW-SD2 and its plant growth-promoting effects on poplar seedlings. Ann Microbiol. 2017;67:219–30. [Google Scholar]
30. Sun Y, Zhang Q, Miao X, Liu J, Yu L, Ma C. Effects of phosphorus-solubilizing bacteria and arbuscular mycorrhizal fungi on production performance and root biomass of alfalfa. Sci Agric Sin. 2019;52(13):2230–42. [Google Scholar]
31. Liu F, Yang W, Wang Z. Plant size effects on the relationships among specific leaf area, leaf nutrient content, and photosynthetic capacity in tropical woody species. Acta Oecol. 2010;36(2):149–59. [Google Scholar]
32. Xu D. Some problems in stomatal limitation analysis of photosynthesis. Plant Physiol J. 1997;4:241–4. [Google Scholar]
33. Li G, Zhang C, Wang Q, Wang K, Liu S, Yang X, et al. Plant chlorophyll metabolism pathways and their molecular regulation. Sichuan Agric Sci Technol. 2021;4:41–5. [Google Scholar]
34. Swapnil P, Meena M, Singh K. Vital roles of carotenoids in plants and humans to deteriorate stress with its structure, biosynthesis, metabolic engineering and functional aspects. Curr Plant Biol. 2021;26:100203. [Google Scholar]
35. Dawood M. Influence of osmoregulators on plant tolerance to water stress. Sci Agric. 2016;13(1):42–58. [Google Scholar]
36. Li D, Li C, Sun H. Effects of drought on soluble protein content and protective enzyme system in cotton leaves. Front Agric China. 2010;4:56–62. [Google Scholar]
37. Li Y, Zhang S, Jiang W. Cadmium accumulation, activities of antioxidant enzymes, and malondialdehyde (MDA) content in Pistia stratiotes L. Environ Sci Pollut R. 2013;20:1117–23. [Google Scholar]
38. Mu M, Zhang D, Zhang H, Yang M, Guo D, Zhou N. Correlation between rhizospheric microorganisms distribution and alkaloid content of Fritillaria taipaiensis P. Y. Li. China J Chin Mater Med. 2019;44(11):2231–5. [Google Scholar]
39. Tang Y, Huang X, Yan X, Yuan X. Accumulation dynamics of alkaloids during the growth of Fritillaria Thunbergii Miq. and its correlation with rhizosphere microenvironment. J Zhejiang Univ Tradit Chin Med. 2021;45(8):816–23. [Google Scholar]
40. Zhao S, Wang Y, Tian Y, Sheng J, Tian X. Screening and characterization of bacteriostatic active strains of endophytic bacteria from Fritillaria ussuriensis maxim. Lishizhen Med Mater Med Res. 2016;27(7):1736–8. [Google Scholar]
41. Yang T, Zhao J, Fang Y, Wang Z, Li X, Yang H. Isolation of biocontrol microbes from Fritillaria przewalskii and its prevention and treatment of rot disease. J Chin Med Mater. 2021;44(1):1–6. [Google Scholar]
42. He D, Wang H, Chen J, Lai C, Yan Z, Huang L. Microecology and geoherbalism of traditional Chinese medicine. China J Chin Mater Med. 2020;45(2):290–302. [Google Scholar]
43. Zhu L, Yan H, Liu P, Zhang Z, Zhang S, Guo S, et al. Research progress on effects of rhizosphere microorganisms on quality formation of medicinal plants and their interaction mechanisms. Chin Tradit Herb Drugs. 2021;52(13):4064–73. [Google Scholar]
Cite This Article
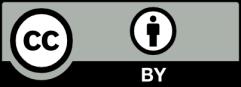
This work is licensed under a Creative Commons Attribution 4.0 International License , which permits unrestricted use, distribution, and reproduction in any medium, provided the original work is properly cited.