Open Access
ARTICLE
Bacterial Inoculation and Co-Inoculation Improves Durum Wheat Productivity in Alkaline Calcareous Soils
1 Laboratoire de Biologie et Physiologie des Organismes, Faculté des Sciences Biologiques, Université des Sciences et de la Technologie Houari Boumediene, Bab Ezzouar, Alger, 16111, Algérie
2 Department of Nature and Life Sciences, Faculty of Sciences, University of Medea, Medea, 26000, Algeria
3 Laboratoire de Recherche sur les Zones Arides, USTHB/ENSA, Alger, 16111, Algeria
4 Biophysical Environment Station, Center for Scientific and Technical Research on Arid Regions, Touggourt, 30240, Algeria
5 Department of Silviculture, Forestry and Game Management Research Institute, Na Olivě 550, Opočno, 51773, Czech Republic
6 Department of Environmental Sciences, COMSATS University Islamabad, Vehari Campus, Vehari, 61100, Pakistan
7 Department of Soil and Environmental Sciences, Faculty of Crop Production Sciences, The University of Agriculture, Peshawar, 25130, Pakistan
8 Department of Math and Computer Science, Faculty of Sciences, Pole Urban Ouzera, University of Medea, Medea, 26000, Algeria
9 Department of Plant Production, College of Food and Agriculture Sciences, King Saud University, Riyadh, 11451, Saudi Arabia
10 Department of Veterinary Sciences, University of Messina, Polo Universitario dell’Annunziata, Messina, 98168, Italy
* Corresponding Authors: Jakub Černý. Email: ; Aftab Jamal. Email:
Phyton-International Journal of Experimental Botany 2024, 93(12), 3313-3329. https://doi.org/10.32604/phyton.2024.059865
Received 18 October 2024; Accepted 02 December 2024; Issue published 31 December 2024
Abstract
Intensive agricultural practices have undeniably reduced soil fertility and crop productivity. Furthermore, alkaline calcareous soils represent a significant challenge for agricultural production, particularly durum wheat, which is vital for ensuring food security. It is therefore essential to explore new cereal management strategies to maintain food production and promote crop sustainability. The application of soil microorganisms, particularly plant growth–promoting rhizobacteria (PGPR), as inoculants to enhance crop production is a growing area of interest. This study investigates the effects of the rhizobacteria Paenibacillus polymyxa SGH1 and SGK2, applied both individually and in combination, on the growth and productivity of durum wheat in alkaline calcareous soil. We conducted field experiments over two growing seasons using a randomized complete block design with three blocks, considering four treatments: non-inoculated wheat grains (T0), inoculation with the P. polymyxa SGH1 strain (T1), inoculation with the P. polymyxa SGK2 strain (T2), and co-inoculation with both strains (T3). The results clearly showed that SGH1 and SGK2 inoculation improved the morphometric characteristics of wheat plants, with co-inoculation of both strains that induced more pronounced improvements compared to T0 in terms of collar diameter (+16.9%), tillers plant−1 (+89.8%), and SA/RA ratio (+35.5%). Co-inoculation was also the most effective treatment for improving the wheat grain yield (+41.1% in season I and + 16.6% in season II). In addition, T3 significantly increased the grain starch content (+220%). T1 determined the highest grain protein content in both seasons (9.5% in season I and 9.66% DW in season II). This study demonstrated that bacterial inoculation and co-inoculation strategies can significantly enhance wheat productivity and grain quality in alkaline calcareous soils while reducing at the same time the ecological footprint of agriculture.Keywords
Durum wheat (Triticum turgidum subsp. durum (Desf.) Husn.) is one of the main cereal crops worldwide. It plays a vital role in providing sustenance to a substantial portion of the world’s population [1,2]. Italy is the leading producer of durum wheat in the European Union, harvesting 4.2 × 106 Mg from over 1.2 × 106 ha [3]. However, the persistent global demand for durum wheat, coupled with the challenges posed by limited arable land and adverse soil conditions, highlights the pressing need for sustainable strategies to enhance durum wheat yields [4].
The presence of alkaline calcareous soils is one of the most significant challenges for durum wheat cultivation, particularly in arid and semi-arid areas [5,6]. Actually, Algeria has approximately 9 million ha of agricultural land, the majority of which consists of calcareous soils that lack essential nutrients such as phosphorus [7]. Calcareous soils, owing to their high pH levels and abundance of calcium carbonate (CaCO3), impose significant constraints on crop growth due to the impairment of soil nutrient availability, with particular reference to P-fixation and Fe-precipitation [8,9]. Consequently, alkaline calcareous soils often decrease durum wheat yields, affect grain quality, and enhance the vulnerability to various environmental stress factors [6]. These challenges may be addressed by innovative agronomic practices, such as the use of arbuscular mycorrhizal fungi (AMF), which offer a promising solution [10]. AMF, a relatively common plant symbiotic fungi, demonstrated to affect the stability of community structures and ecosystems [11]. AMF is currently regarded not only as plant symbionts but also as essential components for plants and soil [12]. Despite a substantial body of research indicating that AMF inoculation fosters agroecosystems, the underlying mechanisms by which AMF inoculation, water stress, and density influence crop growth, biomass allocation, yield formation, and soil quality remain unclear, especially for rainfed wheat [12].
In recent years, bacterial inoculation and co-inoculation strategies have attracted more interest than the traditional AMF approaches [13]. This shift reflects a growing recognition of the diverse benefits that can be gained by using these strategies as an alternative to AMF. Bacterial inoculation is the targeted application of specific bacterial strains to seeds or soil. This initiates plant-microbe interactions that offer numerous advantages to the host plant [14]. It stimulates plant growth directly through the promotion of nutrient acquisition and hormone production, or indirectly by controlling phytopathogens and mitigating plant stress [15]. Inoculation with phosphate-solubilizing bacterial strains, for example, promotes plant growth by enhancing P-availability [13]. Co-inoculation, on the other hand, consists of the application of multiple bacterial strains at the same time to potentially create a powerful combination that will significantly enhance plant performance [16,17]. Several studies demonstrated substantial improvements in durum wheat growth, yield, and nutrient utilization when subjected to bacterial inoculation in alkaline calcareous soils [13,18]. These outcomes prove that it is possible to harness the rhizospheric microbiome as a powerful tool for sustainable durum wheat cultivation in challenging soil environments.
Paenibacillus polymyxa is the standout species among the plant growth-promoting bacteria (PGPR). It is a Gram-positive bacterium, renowned for its capacity to form endospores. The nitrogen-fixing potential of this species was first demonstrated by Von Bredemann [19], but it gained widespread recognition after the work of Hino et al. [20]. Negi et al. [21] reported that P. polymyxa exhibits the highest nitrogenase activity in wheat rhizospheres. Furthermore, this species possesses a range of other beneficial traits for host plants. P. polymyxa can produce compounds that solubilize phosphate [22], antibiotics against plant pathogens [23], and growth-promoting substances [24].
P. polymyxa is an excellent candidate for the study of the PGPR/wheat association thanks to its adaptability to the rhizosphere, nitrogen-fixing capabilities, production of growth-promoting substances, exopolysaccharides, antibiotics, and phosphate solubilization. Research on the topic demonstrated that inoculating wheat with P. polymyxa strains significantly influences plant growth, productivity, and nitrogen utilization [21,25]. For instance, the inoculation of spring wheat (T. aestivum) with P. polymyxa promoted seedling growth and induced positive effects on wheat nutritional status, growth, and development [26] due to the ability of P. polymyxa to invade plant roots and form biofilms in the wheat rhizosphere [27]. The efficacy of seed inoculation with single or multiple PGPR strains on spring wheat yield was assessed in several studies [28,29]. It was also demonstrated that the inoculation with P. polymyxa induced biochemical alterations in wheat plants and developed resistance against water stress [30]. The isolate Paenibacillus sp. S7, identified as P. polymyxa, was found to significantly enhance spring wheat growth [31].
Achieving high yields hinges on aligning the phenological phases of yield components with the availability of water, nitrogen, phosphorus, and potassium. Nitrogen is of particular significance in wheat cultivation, considering that wheat grains contain approximately 12% to 13.5% of proteins [32]. Thus, ensuring a continuous supply of nitrogen to wheat plants through the biological nitrogen fixation operated by P. polymyxa may represent a potential tool for enhancing wheat productivity.
In this context, the inoculation and co-inoculation of efficient P. polymyxa strains on wheat grains immediately after sowing represents a cost-effective alternative to the extensive use of mineral fertilizers. This study builds upon existing research to elucidate the unique contributions of P. polymyxa strains SGH1 and SGK2, applied both individually and in combination, to enhance the growth and productivity of durum wheat in alkaline calcareous soils. It was hypothesized that the co-application of these strains may ensure a complementarity activity and consequently result in significant improvements in wheat yield and nutrient utilization. In contrast to previous studies, our research was performed over two growing seasons, thus providing a more comprehensive and detailed analysis of the effects of these PGPR strains on durum wheat. Furthermore, our research is distinguished by the comprehensive analysis of the specific mechanisms underlying the interaction between these PGPR strains and wheat. The final objective of this study is to reduce the reliance on chemical fertilizers, thereby preserving agroecosystems and ensuring the long-term sustainability of durum wheat in alkaline calcareous soils.
2.1 Location, Climate and Soil
Field experiments were conducted over two growing seasons (2019/2020 and 2020/2021), which will henceforth be referred to as season I and season II, at the Institute of Agronomy in Medea, located in the north-western region. The western zone of the Titteri region is situated to the north of the Tellian Atlas in Algeria. It has an elevation of 1187 m above sea level and it is located at 35°54′31″ N, 2°23′16″ E. The region is predominantly cultivated with cereal crops, with durum wheat being the most prevalent species. The climate of the zone is semi-arid, with an average annual rainfall of approximately 300 mm and average air temperatures of 20°C. The mean air temperatures recorded during season I showed the typical seasonal pattern of the region, characterized by mild winters (minimum temperatures never fell below 0°C) and high temperatures during the summer months, with the highest temperature recorded in July (34.3°C) (Fig. 1a and b). Season II showed a significant increase of temperature in April. May recorded the highest maximum temperature (23.9°C), followed by below-average temperatures in June and July (20.1°C and 17.3°C, respectively). The monthly rainfall patterns were similar across the two growing seasons (Fig. 1), with the highest rainfall levels falling in January (550.5 mm in season I and 655.5 mm in season II). The total rainfall during the crop cycle was 1503.5 mm in season I and 1593.5 mm in season II.
Figure 1: Climatic data (monthly air temperatures and monthly rainfall) from the experimental station located in the field experiments over the two growing seasons: (a) Season I, 2019/2020; (b) Season II, 2020/2021
The soil in this region is typic Mollisols, as defined by the USDA (United States Department of Agriculture) Soil Taxonomy classification. Soil samples were collected at a depth of 15 cm following a zigzag model within a homogeneous 30 m2 area, in order to perform physio-chemical analyses in accordance with the standard laboratory procedures set out by Jones [33]. The soil in the experimental site was classified as silty-clay-sandy, calcareous, and alkaline with 7.9 pH (Table 1).
2.2 Experimental Design and Cultural Practices
Soil seedbed was prepared with a manual weeding followed by the addition of dry organic manure (1 kg m−2). Then, the soil was ploughed 12 days before sowing and leveled. In season I, soil seedbed preparation was performed on 22 November 2019 and sowing occurred on 4 December 2019. In season II, the seedbed was prepared on 15 November 2020 and sowing took place on 2 January 2021. In both seasons, wheat seeds were sourced from a certified, untreated seed lot of the durum wheat cultivar Waha (2014 harvest, Tiaret, Algeria). The main characteristics of this cultivar are plant height at maturity, 80–90 cm; ear color, light amber to red; size, medium to large; cold tolerance; resistance to rust diseases; sensitivity to spring frosts; sowing dose, 100–120 kg ha−1; optimal grain yield, 4.5 t ha−1; thousand-grain weight (TGW), medium; semolina quality, quite good. The quality of this cultivar makes it a highly sought-after product on the Algerian market.
The field experiments were carried out using a randomized complete block (RCB) design with three blocks (Fig. 2a–c). Four treatments were considered: non-inoculated wheat grains (T0), inoculation with the P. polymyxa SGH1 strain (T1), inoculation with the P. polymyxa SGK2 strain (T2), and co-inoculation with the two strains (T3). The elementary plots measured 1 m2, resulting in a total net experimental area of 12 m2 (12 plots). The distance between two adjacent elementary plots was 0.5 m, while the blocks were separated by an alley of 1 m. The seeds were planted by hand in six rows, with a distance of 16 cm between the rows and 0.5 cm between the grains on the line. Forty-three grains were planted per line, resulting in an expected maximum density of 260 seeds m−2 per plot. The control plots were planted first to prevent contamination by bacterial inoculum.
Figure 2: Pictures of the experimental area at three different durum wheat growth stages: (a) plant emergence; (b) tillering; and (c) maturity stage
Irrigation was performed by the field capacity: considering the soil texture and a depth of 30 cm, a bulk density of 1.5 g cm−3, and a retention capacity of 35 g of water 100 g−1 of fine dry soil, soil retention was 157.5 mm, calculated as follows: 300 × 1.5 × 0.35 = 157.5 mm. Irrigation water had a pH range of 6.5–8, a turbidity range of 0–5 NTU, and a chlorine concentration of 0 mg L−1. One week after plant emergence, each plot was irrigated with 20 L of water.
Non-stained seeds of a normal physiognomy were weighed and categorized into weight classes ranging from 30 to 77 mg. A grain weight frequency histogram revealed that the modal class was between 45 and 47 mg. Consequently, this weight class was retained for the purposes of our study, representing 14.2% of the total grains used in all experiments. The use of seeds of the same weight class ensured uniform seedling growth and exudation.
In a study carried out by Athmani-Guemouri et al. [34], it was demonstrated that the diversity of P. polymyxa strains isolated from the rhizosphere of durum wheat cultivated in the highlands of Algeria for less than 70 years showed notable phenotypic and genotypic variability. Therefore, over time, durum wheat plants were able to select only the most suited P. polymyxa strains from a pool of different strains. In order to ascertain their potential impact on the plant, we inoculated durum wheat with a strain belonging to the “group of most adapted strains” (SGK2), which is capable of hindering the development of nine phytopathogenic fungi, and a strain belonging to the “group of the least adapted strains” (SGH1), which has a high nitrogen fixation capacity. The P. polymyxa strains (SGH1 and SGK2) employed in this study were previously isolated from durum wheat rhizosphere in the Tiaret area, a region with a history of cereal farming dating back to the Roman era. The strains were isolated using an immuno-enzyme immuno-trapping method, as described by Guemouri [35]. The assessment of strain identification and diversity was carried out through the API (Analytical Profile Index) method, the RFLP (Restriction Fragment Length Polymorphism) method, and by sequencing the 16S rRNA gene [34,36].
Based on the Enterobacterial Repetitive Intergenic Consensus Polymerase Chain Reaction (ERIC-PCR) method, these bacterial strains are classified within the ERIC-PCR group 1. SGK2 showed the ability to degrade xyloglucan and to impede the in vitro development of several phytopathogenic fungi, including F. graminearum, F. culmorum, F. verticillioides, and M. nivale, agents of fusarium wilt of durum wheat. Both SGH1 and SGK2 strains have the capacity to produce high levels of indole-3-acetic acid (IAA), with yields of 161 and 134.96 µg mL−1, respectively. Furthermore, they are capable of secreting exopolysaccharides (EPS) and solubilizing inorganic phosphates. The SGK2 strain displays a greater capacity for phosphate solubilization (tricalcium and monocalcium) than SGH1, with the degree of monocalcium phosphate solubilization by the two bacterial strains exceeding that of tricalcium.
2.5 Preparation of Bacterial Inoculum and Bacterization of Grains
The bacterial inoculates were prepared following the methodology described by Chabot et al. [37]. The selected bacteria (SGH1 and SGK2) were cultivated in 250 mL Erlenmeyer flasks containing 50 mL of LB medium on a rotary shaker at 100 rpm min–1 at 30°C for 48 h. Then, the bacterial cells were concentrated through centrifugation at 5000 rpm for 15 min, washed thrice with a sterile phosphate buffer solution, and suspended in 500 mL of sterile physiological water. A total of 780 mL of bacterial suspension was applied to each plot at durum wheat sowing, resulting in a concentration of 108 germs mL−1 of inoculum, equivalent to an optical density (OD) of 0.5, in accordance with Pradhan et al. [38]. Each seed was inoculated with 3 mL of bacterial suspension.
The sample for durum wheat measurements comprised three plants per elementary plot, for a total of nine plants assessed for each parameter. Sampling was performed at various growth stages of plant development. The following parameters were measured: collar diameter, number of short roots (roots < 1 cm in length, which play a crucial role in ectomycorrhizal symbiosis), and mass of adhering soil (rhizosphere forming a matrix of soil adhering to roots), SA/RA ratio (weight of rhizospheric soil/dry weight of roots), number of tillers plant−1, number ears plant−1, number of grains ear−1, mean 1000 seed weight. Crop harvest was carried out on 01 August 2020 and 28 August 2022 for season I and season II, respectively. The grain yield was quantified as the weight of grains harvested within the plot.
2.7 Starch Analysis of Durum Wheat Grain
Starch analysis was carried out on 100 g of dry plant material corresponding to 100 g of grain powder (semolina). The starch is dosed calorimetrically in accordance with the methodology proposed by McCready et al. [39]:
Reagent: Anthrone or “9-oxo-10-dihydroanthracene” is the tautomeric form of anthranol. Anthrone appears light yellow if dissolved in a concentrated sulfuric medium and blue-colored with carbohydrate solutions, with a fairly luminous range from green to blue-green depending on the concentration of these solutions.
Preparation: 1 g of anthrone is dissolved in 500 mL of 96% sulfuric acid, prepared by adding 1 L of pure sulfuric acid and 40 mL of distilled water.
Procedure: 1 mL of wheat grains extract is placed in a test tube, covered with 2 mL of the sulfuric reagent anthrone, shacked for homogenization, and put for at least 10 min in a rack at 100°C. Then, the optical density of the solution is measured with a spectrophotometer at 630 nm. The calibration curve is derived from a diluted solution of glucose. The result is expressed in g of starch 100 g−1 dry weight (DW) of wheat seeds.
2.8 Protein Analysis of Durum Wheat Grain
Protein determination was conducted calorimetrically via the Bradford method [40] on 100 g of grain powder (semolina). The Bradford method is a commonly used colorimetric assay for measuring protein concentration, based on the binding of Coomassie Brilliant Blue dye to proteins.
Reagents: Bradford Reagent purchased from Sigma-Aldrich (Algeria).
BSA Standard Curve: Prepare a series of BSA dilutions to create a standard curve for protein concentration.
Procedure: 100 mg of grain semolina was homogenized in 5 mL of 0.1 M phosphate buffer (pH 7.0) to extract the protein. They were homogenized thoroughly to ensure an efficient extraction. Then, the mixture was centrifugated at 12,000× g for 12 min and the supernatant containing the extracted proteins was collected. For the Bradford assay, 100 µL of the supernatant was diluted with 10 mL of distilled water, followed by the addition of 5 mL of Bradford reagent and a gentle mixing at room temperature for 5 min. Absorbance was measured at 595 nm using a spectrophotometer. Protein concentration was quantified against a BSA standard and expressed as mg 100 g−1 DW.
Data were subjected to a factorial two-way analysis of variance (ANOVA) model, considering the ‘treatment’, the ‘growing season’, and their interactions as fixed factors. Homoscedasticity was verified using Bartlett’s test, and normality was assessed by inspecting the residuals, which showed no significant deviations. Pairwise mean comparisons were conducted using Tukey’s HSD test at α = 0.05. The ANOVA was performed with the CoStat®® ver. 6.003 software (CoHort, Monterey, CA, USA).
3.1 Durum Wheat Morphological Traits
Except for collar diameter, all morphological traits of durum wheat plants were mostly affected by the inoculation treatment. The ‘treatment × growing season’ interaction was significant for tillers plant−1, short roots plant−1, and SA/RA ratio (Table 2). The mean values for collar diameter across the two growing seasons revealed that co-inoculation (T3) and inoculation with the P. polymyxa SGH1 strain (T1) significantly increased this trait by 16.9% and 21.3% compared to T0, respectively (Table 3). Additionally, collar diameter was higher in season I than in season II (+10.8%), while no significant effects were observed for the other morphological traits across the two growing seasons.
Except for T1 for the mass of adherent soil, all inoculation treatments resulted in enhanced morphological traits (Table 3). Notably, T2 and T3 caused the most pronounced increase of tillers plant−1, short roots plant−1, mass of adherent soil, and SA/RA ratio. Concerning the number of tillers plant−1, T3 and T2 showed the highest increase (+89.8% and +71.5%, respectively, compared to T0) in season I, followed by T1 (+42.8%) (Fig. 3). Although to a lesser extent, season II showed a similar trend to season I, with T3 and T2 that respectively enhanced tillers plant−1 by 41.1% and 39%, compared to T0.
Figure 3: Two-way analysis of variance of (a, b) number of tillers, (c, d) number of short roots, and (e, f) SA/RA ratio (weight of rhizospheric soil/dry weight of roots) of durum wheat var. Waha. Vertical bars are the standard deviation (n = 3). The histograms followed by the same letter are not significantly different at α = 0.05 (Tukey’s HSD test). T0: non-inoculated wheat grains; T1: inoculation with Paenibacillus polymyxa SGH1 strain; T2: inoculation with P. polymyxa SGK2 strain; T3: co-inoculation with the two strains; season I: 2019/2020; season II: 2020/2021
Concerning the short roots plant−1, T2 elicited the most pronounced increases in both season I (+98.2%) and season II (+52.5%), compared to T0 (Fig. 3). Furthermore, T1 and T3 also increased the number of short roots plant−1 in comparison to T0. Similarly to tillers plant−1, T3 and T2 determined the highest increase in the SA/RA ratio in both season I (+35.5% and +35.8%, respectively) and season II (+15.3% and +15.7%, respectively).
3.2 Durum Wheat Yield and Productive Traits
The main factors under study and their interaction significantly affected all durum wheat productive traits (Table 2). The ANOVA revealed that the growing season had the greatest impact on the overall variance for grain yield and ears plant−1. Conversely, the inoculation treatment had the greatest influence on the majority of the variance observed for grains ear−1 and thousand-grain weight. As shown in Table 3, the co-inoculation treatment (T3) induced the highest enhancements for all productive traits. Except for grains ear−1, season I showed higher values than season II for all productive traits.
Fig. 4 shows the two-way interactions of durum wheat productive traits. Concerning grain yield, T3 determined the greatest increase compared to T0, with a rate of +41.1% in season I and +16.6% in season II, followed by T1 and T2. Furthermore, in comparison to the control, T3 caused the highest number of ears plant−1, particularly in season II (+121.0% vs. +59.8% in season I). Conversely, the increase in the number of ears plant−1 caused by T1 and T2 was more pronounced in season I than in season II. Concerning the number of grains ear−1, no significant differences were observed between the inoculation treatments in season I. However, in season II, only T2 did not show any stimulatory effect. The results for thousand-grain weight showed a comparable pattern across both growing seasons, with all the inoculation treatments demonstrating a comparable degree of enhancement for this trait with respect to T0.
Figure 4: Two-way analysis of variance of (a, b) grain yield, (c, d) number of ears, (e, f) number of grains, and (g, h) thousand seed weight of durum wheat var. Waha. Vertical bars are the standard deviation (n = 3). The histograms followed by the same letter are not significantly different at α = 0.05 (Tukey’s HSD test). T0: non-inoculated wheat grains; T1: inoculation with P. polymyxa SGH1 strain; T2: inoculation with P. polymyxa SGK2 strain; T3: co-inoculation with the two strains; season I: 2019/2020; season II: 2020/2021.
3.3 Durum Wheat Technological Quality
The inoculation treatments had a significant influence on both grain starch and protein content, as shown in Table 2. On the average of growing seasons (Table 3), T3 induced the highest increase in grain starch content (+217.8% compared to T0), while T2 was the most effective treatment for grain protein content (+79.1% than T0), even if not statistically different from T3. The growing season did not affect durum wheat technological quality, whereas the ‘treatment × growing season’ interaction was significant for both technological characteristics. T3 caused the highest grain starch content in both seasons, with values of 63 g 100−1 g DW in season I and 80 g 100−1 g DW in season II. T2, on the contrary, did not influence the grain starch content. Concerning the grain protein content, only T1 significantly promoted it in season I (+48.4% compared to T0). In season II, an improvement in the grain protein content was induced by T1 (+124.8%) and T3 (+112.4%) (Fig. 5).
Figure 5: Two-way analysis of (a, b) grain starch content and (c, d) grain protein content of durum wheat var. Waha. Vertical bars are the standard deviation (n = 3). The histograms followed by the same letter are not significantly different at α = 0.05 (Tukey’s HSD test). T0: non-inoculated wheat grains; T1: inoculation with Paenibacillus polymyxa SGH1 strain; T2: inoculation with P. polymyxa SGK2 strain; T3: co-inoculation with the two strains; season I: 2019/2020; season II: 2020/2021
The results of this study indicate that inoculation and particularly the co-inoculation of two P. polymyxa strains selected from Algerian soils had a beneficial impact on the morphology, yield, and technological grain quality of durum wheat cultivated on alkaline and calcareous soils. Concerning the plant growth, co-inoculation of the SGH1 and SGK2 strains (T3) significantly increased the mean collar diameter (+20.3% in season I and +12.1% in season II) and the number of tillers plant−1 (+89% in season 1 and +41% in season 2) compared to the control, denoting the synergistic action of these strains. Co-inoculation, in alignment with previous studies using diverse microbial strains, provides further evidence to support the potential of microbial agents in optimizing plant growth and development [41,42]. SGH1-SGK2 co-inoculation leverages the ability of beneficial rhizobacteria to enhance wheat growth by facilitating nutrient uptake and alleviating the stressors associated with alkaline calcareous soils, including via hormone production [18,23]. For example, some PGPR such as Azospirillum brasilense are able to produce indole-3-acetic acid (IAA), a plant growth hormone that stimulates root and shoot growth [43]. It is reasonable to hypothesize that SGH1-SGK2 co-inoculation may have stimulated IAA production, thus enhancing wheat collar diameter. It has been demonstrated that both P. polymyxa strains and their exopolysaccharides (EPSs) can promote wheat growth by increasing root and shoot length and root and shoot dry weight [44]. Similarly, another study reported significant growth-promoting effects on wheat plants treated with the antagonistic bacterium P. polymyxa ZYPP18 [23]. Jabborova et al. [45] additionally indicated that co-inoculation with multiple strains of microorganisms, such as Pseudomonas putida NUU8, can exert a more pronounced influence on soybean growth than single inoculation.
Strain-specific results have been here observed. For example, the inoculation of P. polymyxa SGK2 had a notable impact on the number of wheat short roots compared to the uninoculated plants. These findings suggest that the SGK2 strain may possess distinctive characteristics that selectively promote short-root development, thereby enhancing ectomycorrhizal associations in the specific context under study. P. polymyxa strains are known for their capacity to solubilize and mobilize soil nutrients, including phosphorus and iron, thus increasing their uptake by plants [22]. The enhanced nutrient availability can stimulate root development, including the formation of short roots, as the plant seeks to maximize nutrient uptake [46]. Furthermore, some P. polymyxa strains show biocontrol properties and can suppress soil-borne pathogens and nematodes [23]. When plants are protected from diseases and pests, their root systems tend to develop more vigorously, potentially leading to an increased number of short roots.
Arbuscular mycorrhizal fungi and bacteria play important roles in enhancing plant growth and nutrient acquisition, but they work through different mechanisms and interactions. Both AMF and bacteria are essential for sustainable agricultural practices thanks to their complementary roles in enhancing plant growth and resilience, as demonstrated for instance by Pandino et al. [47]. While AMF primarily enhances phosphorus and nitrogen uptake through their extensive hyphal networks [48], several bacterial species, including those of the Bacillus genus, promote plant growth by nutrient solubilization, phytohormones production, and pathogens suppression [49]. Moreover, the rhizobacterial community is known to enhance the effectiveness of AMF when co-inoculated [49].
This study also demonstrated that P. polymyxa SGK2 and co-inoculation markedly enhanced the soil mass adhering to the roots. This phenomenon is consistent with previous findings indicating that P. polymyxa, by virtue of enhancing soil mass adhesion to plant roots, leads to a more porous soil structure and therefore to enhanced water retention and nutrient availability [44]. The P. polymyxa SC2 strain showed comparable outcomes [50]. Furthermore, the SA/RA ratio was found influenced by the inoculation and co-inoculation treatments, indicating alterations in the composition of rhizodeposition. It is plausible that this increase can be attributed to the capacity of P. polymyxa to produce biofilms or EPS, which facilitate the aggregation and adherence of soil particles to roots [51,52].
In accordance with the observed results on the morphological characteristics of durum wheat, co-inoculation induced also the highest grain yield, outperforming the inoculation with the P. polymyxa SGH1 strain. Conversely, the SGK2 strain had a negligible effect on grain yield, likely due to the lower average grain weights. A review of the literature revealed that the co-inoculation of P. polymyxa strains with other microbial strains such as A. brasilense, can result in enhanced plant growth-promoting effects and increased grain yields [53]. This indicates that the interactions between different microbial strains may contribute to the optimization of the benefits for the host plant. Moreover, the efficacy of microbial inoculants, including P. polymyxa strains, can vary depending on the specific strain employed [26]. It can therefore be hypothesized that co-inoculation with specific P. polymyxa strains may have a more pronounced effect on grain yield in wheat, in comparison to single-strain inoculation or co-inoculation with other microbial strains. This increase was corroborated by a notable rise in the number of ears plant−1 and grains ear−1, which are indicative of enhanced flower fertility [54]. Furthermore, co-inoculation and SGH1 inoculation determined the highest 1000 seed weights, suggesting that these treatments enhance grain filling and grain weight. These effects can be attributed to the improved nutrient availability, and hormonal signals, as well as to the activation of synthetic enzymes responsible for grain starch and protein production [55].
In fact, we found that microbial inoculation and co-inoculation enhanced the levels of grain starch and protein. As observed for the productive traits, the inoculation with P. polymyxa SGH1 strain induced a considerable increase of the grain starch (+85% compared to control) and protein (+124.65%) contents, whereas the inoculation with P. polymyxa SGK2 strain did not produce a statistically significant difference. This suggests a strain-specific effect of microbial inoculation on durum wheat technological characteristics and emphasizes the importance of strain selection for crop improvement strategies [56]. This increase may be attributed to an improved nitrogen supply to the plants, which may potentially activate synthetic enzymes and subsequently enhance starch accumulation. Moreover, the improved nutrient availability and hormonal signals can trigger the activation of synthetic enzymes responsible for starch and protein production in grains [57]. Furthermore, microbial inoculation can activate specific plant metabolic pathways, thus leading to an increased synthesis of storage compounds such as starch and proteins [58].
The findings of this study demonstrated that the co-inoculation of durum wheat with P. polymyxa SH1 and SGK2 strains had positive effects on the crop’s morphological, productive, and technological attributes. This is consistent with the hypothesis that bacterial inoculants should comprise a mixture of strains, thus ensuring a synergistic activity. Based on these results, it can be concluded that P. polymyxa co-inoculation represents a promising approach for durum wheat cultivation in alkaline and calcareous soils, thereby facilitating its cultivation in deprived areas and supporting food security. However, further research is needed to investigate other rhizobacterial strains across different pedo-climatic conditions.
Acknowledgement: The authors acknowledge Researchers Supporting Project number (RSP-2024R375), King Saud University, Saudi Arabia.
Funding Statement: QK22020008 (National Agency of Agricultural Research of the Czech Republic), TQ03000234 (Technological Agency of the Czech Republic), and MZE-RO0123 (Ministry of Agriculture, CR).
Author Contributions: The authors confirm their contribution to the paper as follows: study conception and design: Bouazza Chami, Souad Guemouri-Athmani and Djamel Baali-Cherif; data collection: Bouazza Chami and Aftab Jamal; analysis and interpretation of results: Bouazza Chami, Jakub Černý, Souad Guemouri-Athmani, Yaser Hassan Dewir and Aurelio Scavo; draft manuscript preparation: Bouazza Chami, Jakub Černý, Nabil Touzout, Adil Mihoub, Aftab Jamal, Muhammad Farhan Saeed, Hayi Mohamed Yassine and Aurelio Scavo. All authors reviewed the results and approved the final version of the manuscript.
Availability of Data and Materials: The data and material for the current study are included in this published article. There is no other data generated or used. The datasets used and/or analyzed during the present experiments are available from the corresponding author upon reasonable request.
Ethics Approval: Not applicable.
Conflicts of Interest: The authors declare no conflicts of interest to report regarding the present study.
References
1. Scavo A, Pandino G, Restuccia A, Caruso P, Lombardo S, Mauromicale G. Allelopathy in durum wheat landraces as affected by genotype and plant part. Plants. 2022;11(8):1021. doi:10.3390/plants11081021. [Google Scholar] [PubMed] [CrossRef]
2. Mancinelli R, Allam M, Petroselli V, Atait M, Jasarevic M, Catalani A, et al. Durum wheat production as affected by soil tillage and fertilization management in a mediterranean environment. Agriculture. 2023;13(2):433. doi:10.3390/agriculture13020433. [Google Scholar] [CrossRef]
3. Eurostat database. Available from: https://ec.europa.eu/eurostat/data/database. [Accessed 2023]. [Google Scholar]
4. Ul Haq I, Khan A, Saeed MF, Mihoub A, Jamal A, Fawad M, et al. Timing and splitting of nitrogen compensated for the loss in grain yield of dual-purpose wheat under varied cutting heights. Gesunde Pflanz. 2023;75(2):237–52. doi:10.1007/s10343-022-00695-2. [Google Scholar] [CrossRef]
5. Jamal A, Fawad M. Effectiveness of phosphorous fertilizers in wheat crop production in Pakistan. J Hort Plant Res. 2019;5:25–9. doi:10.18052/www.scipress.com/JHPR.5.25. [Google Scholar] [CrossRef]
6. Saeed MF, Jamal A, Muhammad D, Shah GM, Bakhat HF, Ahmad I, et al. Optimizing phosphorus levels in wheat grown in a calcareous soil with the use of adsorption isotherm models. J Soil Sci Plant Nutr. 2021;21(1):81–94. doi:10.1007/s42729-020-00344-5. [Google Scholar] [CrossRef]
7. Boukhalfa-Deraoui N, Hanifi-Mekliche L, Mihoub A. Effect of incubation period of phosphorus fertilizer on some properties of sandy soil with low calcareous content, southern Algeria. Asian J Agric Res. 2015;9(3):123–31. [Google Scholar]
8. Taalab A, Ageeb G, Siam HS, Mahmoud SA. Some characteristics of calcareous soils. A review. Middle East J. 2019;8(1):96–105. [Google Scholar]
9. Jamal A, Saeed MF, Mihoub A, Hopkins BG, Ahmad I, Naeem A. Integrated use of phosphorus fertilizer and farmyard manure improves wheat productivity by improving soil quality and P availability in calcareous soil under subhumid conditions. Front Plant Sci. 2023;14:1034421. doi:10.3389/fpls.2023.1034421. [Google Scholar] [PubMed] [CrossRef]
10. Lombardo S, Abbate C, Pandino G, Parisi B, Scavo A, Mauromicale G. Productive and physiological response of organic potato grown under highly calcareous soils to fertilization and mycorrhization management. Agronomy. 2020;10(8):1200. doi:10.3390/agronomy10081200. [Google Scholar] [CrossRef]
11. Duan H-X, Luo C-L, Zhu Y, Zhao L, Wang J, Wang W, et al. Arbuscular mycorrhizal fungus activates wheat physiology for higher reproductive allocation under drought stress in primitive and modern wheat. Eur J Agron. 2024;161(2):127376. doi:10.1016/j.eja.2024.127376. [Google Scholar] [CrossRef]
12. Duan H-X, Luo C-L, Zhou R, Zhao L, Zhu S-G, Chen Y, et al. AM fungus promotes wheat grain filling via improving rhizospheric water & nutrient availability under drought and low density. Appl Soil Ecol. 2024;193:105159. [Google Scholar]
13. Adnan M, Fahad S, Saleem MH, Ali B, Mussart M, Ullah R, et al. Comparative efficacy of phosphorous supplements with phosphate solubilizing bacteria for optimizing wheat yield in calcareous soils. Sci Rep. 2022;12(1):11997. [Google Scholar] [PubMed]
14. Lopes MJS, Dias-Filho MB, Gurgel ESC. Successful plant growth-promoting microbes: inoculation methods and abiotic factors. Front Sustain Food Syst. 2021;5:606454. [Google Scholar]
15. Bhat MA, Mishra AK, Jan S, Bhat MA, Kamal MA, Rahman S, et al. Plant growth promoting rhizobacteria in plant health: a perspective study of the underground interaction. Plants. 2023;12(3):629. [Google Scholar] [PubMed]
16. Bargaz A, Lyamlouli K, Chtouki M, Zeroual Y, Dhiba D. Soil microbial resources for improving fertilizers efficiency in an integrated plant nutrient management system. Front Microbiol. 2018;9:1606. [Google Scholar] [PubMed]
17. Wang Z, Chen Z, Fu X. Integrated effects of co-inoculation with phosphate-solubilizing bacteria and N2-fixing bacteria on microbial population and soil amendment under C deficiency. Int J Environ Res Public Health. 2019;16(13):2442. doi:10.3390/ijerph16132442. [Google Scholar] [PubMed] [CrossRef]
18. Babar S, Jilani G, Mihoub A, Jamal A, Ahmad I, Chaudhary AN, et al. Bacterial redox cycling of manganese in calcareous soil enhances the nutrients bioavailability to wheat. J Soil Sci Plant Nutr. 2022;22(2):1215–23. doi:10.1007/s42729-021-00725-4. [Google Scholar] [CrossRef]
19. Von Bredemann G. Untersuchungen uber die variation und das Stickstoffbindungsvermogen des Bacillus asterosporus AM, ausgefuhrt an 27 Stammen verschiedener Herkunft. Zbl Bakteriol Parasitenkd Infektionskr Hyg. 1908;2:44–89. [Google Scholar]
20. Hino S, Wilson P. Nitrogen fixation by a facultative Bacillus. J Bacteriol. 1958;75(4):403–8. doi:10.1128/jb.75.4.403-408.1958. [Google Scholar] [PubMed] [CrossRef]
21. Negi R, Sharma B, Kumar S, Chaubey KK, Kaur T, Devi R, et al. Plant endophytes: unveiling hidden applications toward agro-environment sustainability. Folia Microbiol. 2024;2023(1):1–26. doi:10.1007/s12223-023-01092-6. [Google Scholar] [PubMed] [CrossRef]
22. Devi R, Kaur T, Kour D, Yadav A, Yadav AN, Suman A, et al. Minerals solubilizing and mobilizing microbiomes: a sustainable approach for managing minerals’ deficiency in agricultural soil. J Appl Microbiol. 2022;133(3):1245–72. [Google Scholar] [PubMed]
23. Yi W, Chen C, Gan X. Polymyxin B1 and E2 From Paenibacillus polymyxa Y-1 for controlling rice bacterial disease. Front Cell Infect Microbiol. 2022;12:283. [Google Scholar]
24. Li X, Ma S, Meng Y, Wei W, Peng C, Ling C, et al. Characterization of antagonistic bacteria Paenibacillus polymyxa ZYPP18 and the effects on plant growth. Plants. 2023;12(13):2504. [Google Scholar] [PubMed]
25. Mahmud K, Missaoui A, Lee K, Ghimire B, Presley HW, Makaju S. Rhizosphere microbiome manipulation for sustainable crop production. Curr Plant Biol. 2021;27:100210. [Google Scholar]
26. Hussain A, Ahmad M, Nafees M, Iqbal Z, Luqman M, Jamil M, et al. Plant-growth-promoting Bacillus and Paenibacillus species improve the nutritional status of triticum aestivum L. PLoS One. 2020;15(12):e0241130. [Google Scholar] [PubMed]
27. He X, Li Q, Wang N, Chen S. Effects of an EPS biosynthesis gene cluster of Paenibacillus polymyxa WLY78 on biofilm formation and nitrogen fixation under aerobic conditions. Microorganisms. 2021;9(2):289. [Google Scholar] [PubMed]
28. Mirskaya GV, Khomyakov YV, Rushina NA, Vertebny VE, Chizhevskaya EP, Chebotar VK, et al. Plant development of early-maturing spring wheat (Triticum aestivum L.) under inoculation with Bacillus sp. V2026. Plants. 2022;11(14):1817. [Google Scholar] [PubMed]
29. Rahnama S, Ardestani EG, Ebrahimi A, Nikookhah F. Seed priming with plant growth-promoting bacteria (PGPB) improves growth and water stress tolerance of Secale montanum. Heliyon. 2023;9(4):e15498. [Google Scholar] [PubMed]
30. Zia R, Nawaz MS, Yousaf S, Amin I, Hakim S, Mirza MS, et al. Seed inoculation of desert-plant growth-promoting rhizobacteria induce biochemical alterations and develop resistance against water stress in wheat. Physiol Plant. 2021;172(2):990–1006. [Google Scholar] [PubMed]
31. Kaziūnienė J, Mažylytė R, Krasauskas A, Toleikienė M, Gegeckas A. Optimizing the growth conditions of the selected plant-growth-promoting Rhizobacteria Paenibacillus sp. MVY-024 for industrial scale production. Biology. 2022;11(5):745. [Google Scholar] [PubMed]
32. Afzal M, Sielaff M, Distler U, Schuppan D, Tenzer S, Longin CFH. Reference proteomes of five wheat species as starting point for future design of cultivars with lower allergenic potential. npj Sci Food. 2023;7(1):9. [Google Scholar] [PubMed]
33. Jones JB Jr. Laboratory guide for conducting soil tests and plant analysis. CRC; 2001. [Google Scholar]
34. Guemouri-Athmani S, Berge O, Bourrain M, Mavingui P, Thiéry JM, Bhatnagar T, et al. Diversity of Paenibacillus polymyxa populations in the rhizosphere of wheat (Triticum durum) in Algerian soils. Eur J Soil Biol. 2000;36(3–4):149–59. [Google Scholar]
35. Guemouri S. Isolement et caractérisation des souches de Bacillus polymyxa isolées de différents sols algériens (Thèse Magister). USTHB: Alger; 1992. [Google Scholar]
36. Guemouri S. Adaptation des populations de Paenibacillus polymyxa aux racines de blé dur cultivé sur des sols Algériens; 2006. [Google Scholar]
37. Chabot R, Antoun H, Cescas MP. Stimulation de la croissance du maïs et de la laitue romaine par des microorganismes dissolvant le phosphore inorganique. Can J Microbiol. 1993;39(10):941–7. [Google Scholar]
38. Pradhan N, Sukla L. Solubilization of inorganic phosphates by fungi isolated from agriculture soil. Afr J Biotechnol. 2006;5(10):850–4. [Google Scholar]
39. McCready RM. Determination of starch and amylose in vegetables. Anal Chem. 1950;22:1156–9. [Google Scholar]
40. Bradford MM. A rapid and sensitive method for the quantitation of microgram quantities of protein utilizing the principle of protein-dye binding. Anal Biochem. 1976;72(1–2):248–54. doi:10.1016/0003-2697(76)90527-3. [Google Scholar] [PubMed] [CrossRef]
41. Benmrid B, Ghoulam C, Zeroual Y. Bioinoculants as a means of increasing crop tolerance to drought and phosphorus deficiency in legume-cereal intercropping systems. Commun Biol. 2023;6(1):1016. doi:10.1038/s42003-023-05399-5. [Google Scholar] [PubMed] [CrossRef]
42. Nadarajah K, Abdul Rahman NSN. The microbial connection to sustainable agriculture. Plants. 2023;12(12):2307. doi:10.3390/plants12122307. [Google Scholar] [PubMed] [CrossRef]
43. Lebrazi S, Niehaus K, Bednarz H, Fadil M, Chraibi M, Fikri-Benbrahim K. Screening and optimization of indole-3-acetic acid production and phosphate solubilization by rhizobacterial strains isolated from acacia cyanophylla root nodules and their effects on its plant growth. J Genet Eng Biotechnol. 2020;18(1):1–12. doi:10.1186/s43141-020-00090-2. [Google Scholar] [PubMed] [CrossRef]
44. Yegorenkova IV, Tregubova KV, Krasov AI, Evseeva NV, Matora LY. Effect of exopolysaccharides of Paenibacillus polymyxa rhizobacteria on physiological and morphological variables of wheat seedlings. J Microbiol. 2021;59(8):729–35. doi:10.1007/s12275-021-0623-9. [Google Scholar] [PubMed] [CrossRef]
45. Jabborova D, Kannepalli A, Davranov K, Narimanov A, Enakiev Y, Syed A, et al. Co-inoculation of rhizobacteria promotes growth, yield, and nutrient contents in soybean and improves soil enzymes and nutrients under drought conditions. Sci Rep. 2021;11:22081. [Google Scholar] [PubMed]
46. López-Bucio J, Cruz-Ramırez A, Herrera-Estrella L. The role of nutrient availability in regulating root architecture. Curr Opin Plant Biol. 2003;6(3):280–7. [Google Scholar]
47. Pandino G, Abbate C, Scavo A, Di Benedetto D, Mauromicale G, Lombardo S. Co-inoculation of arbuscular mycorrhizal fungi and plant growth promoting bacteria improves plant growth and yield of globe artichoke (Cynara cardunculus scolymus). Sci Hortic. 2024;335:113365. [Google Scholar]
48. Emmett BD, Lévesque-Tremblay V, Harrison MJ. Conserved and reproducible bacterial communities associate with extraradical hyphae of arbuscular mycorrhizal fungi. ISME J. 2021;15(8):2276–88. [Google Scholar] [PubMed]
49. Nanjundappa A, Bagyaraj DJ, Saxena AK, Kumar M, Chakdar H. Interaction between arbuscular mycorrhizal fungi and Bacillus spp. in soil enhancing growth of crop plants. Fungal Biol Biotechnol. 2019;6(1):23. [Google Scholar] [PubMed]
50. Liu H, Li Y, Ge K, Du B, Liu K, Wang C, et al. Interactional mechanisms of Paenibacillus polymyxa SC2 and pepper (Capsicum annuum L.) suggested by transcriptomics. BMC Microbiol. 2021;21:1–16. [Google Scholar]
51. Costa OY, Raaijmakers JM, Kuramae EE. Microbial extracellular polymeric substances: ecological function and impact on soil aggregation. Front Microbiol. 2018;9:1636. [Google Scholar] [PubMed]
52. Timmusk S, Copolovici D, Copolovici L, Teder T, Nevo E, Behers L. Paenibacillus polymyxa biofilm polysaccharides antagonise Fusarium graminearum. Sci Rep. 2019;9(1):662. [Google Scholar] [PubMed]
53. Yegorenkova IV, Tregubova KV, Burygin GL, Matora LY, Ignatov VV. Assessing the efficacy of co-inoculation of wheat seedlings with the associative bacteria Paenibacillus polymyxa 1465 and Azospirillum brasilense Sp245. Can J Microbiol. 2016;62(3):279–85. [Google Scholar] [PubMed]
54. Hafez M, Popov AI, Rashad M. Integrated use of bio-organic fertilizers for enhancing soil fertility-plant nutrition, germination status and initial growth of corn (Zea mays L.). Environ Technol Innov. 2021;21:101329. [Google Scholar]
55. Lamichhane JR, Corrales DC, Soltani E. Biological seed treatments promote crop establishment and yield: a global meta-analysis. Agron Sustain Dev. 2022;42(3):45. [Google Scholar]
56. Shahwar D, Mushtaq Z, Mushtaq H, Alqarawi AA, Park Y, Alshahrani TS, et al. Role of microbial inoculants as bio fertilizers for improving crop productivity: a review. Heliyon. 2023;9(6):e16134. [Google Scholar] [PubMed]
57. Zhang D, Zhang M, Liang J. RGB1 regulates grain development and starch accumulation through its effect on OsYUC11-mediated auxin biosynthesis in rice endosperm cells. Front Plant Sci. 2021;12:585174. [Google Scholar] [PubMed]
58. Mason-Jones K, Breidenbach A, Dyckmans J, Banfield CC, Dippold MA. Intracellular carbon storage by microorganisms is an overlooked pathway of biomass growth. Nat Commun. 2023;14(1):2240. [Google Scholar] [PubMed]
Cite This Article
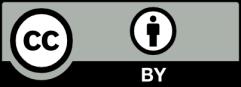
This work is licensed under a Creative Commons Attribution 4.0 International License , which permits unrestricted use, distribution, and reproduction in any medium, provided the original work is properly cited.