Open Access
REVIEW
Effects of Nanofertilizers on the Mechanism of Photosynthetic Efficiency in Plants: A Review
1 College of Resources and Environmental Sciences, China Agricultural University, Beijing, 100193, China
2 China Agricultural University, Professor Workstation in Tangshan Jinhai New Material Co., Tangshan, 063000, China
3 China Agricultural University, Shanghe County Baiqiao Town Science and Technology Courtyard, Jinan, 251600, China
4 China Agricultural University, Hebei Wuqiang County Professor Workstation and Science and Technology Small Courtyard, Hengshui, 053300, China
* Corresponding Author: Yukui Rui. Email:
Phyton-International Journal of Experimental Botany 2024, 93(12), 3197-3216. https://doi.org/10.32604/phyton.2024.059281
Received 02 October 2024; Accepted 14 November 2024; Issue published 31 December 2024
Abstract
Compared with conventional fertilizers, nano fertilizer has many advantages such as controlled release, controlled or slow release of nutrients, high efficiency of nutrition use, cheap, and little polluting of the environment. The use of fertilizers with nanotechnology is a new field in agriculture, and it is a promising and cost-effective substitute for conventional fertilizers to improve the productivity of the world’s food supply. Photosynthesis is an essential biochemical reaction on Earth. Improving photosynthesis, the basic process for light’s transformation into chemical energy is one of the most important areas of research for improving agricultural output and tackling world food security. Nano fertilizers can promote plant photosynthesis, improve crop photosynthetic efficiency, increase plant biomass, improve plant stress resistance, improve nutrient uptake efficiency, and encourage plant growth and development due to their tunable surface properties, special electronic, magnetic, and optical properties, and other characteristics. It can be seen that nano fertilizers and improving photosynthetic efficiency in plants are a hot topic of concern. Therefore, an overview of the effects of nano fertilizers on plant photosynthesis is given in this paper. These effects include the ability to increase biomass, pigment and gas openness, photosynthetic efficiency, and plant resistance to stress. On the other hand, improper use of nano fertilizers can have the opposite effect, inhibiting plant photosynthesis.Graphic Abstract
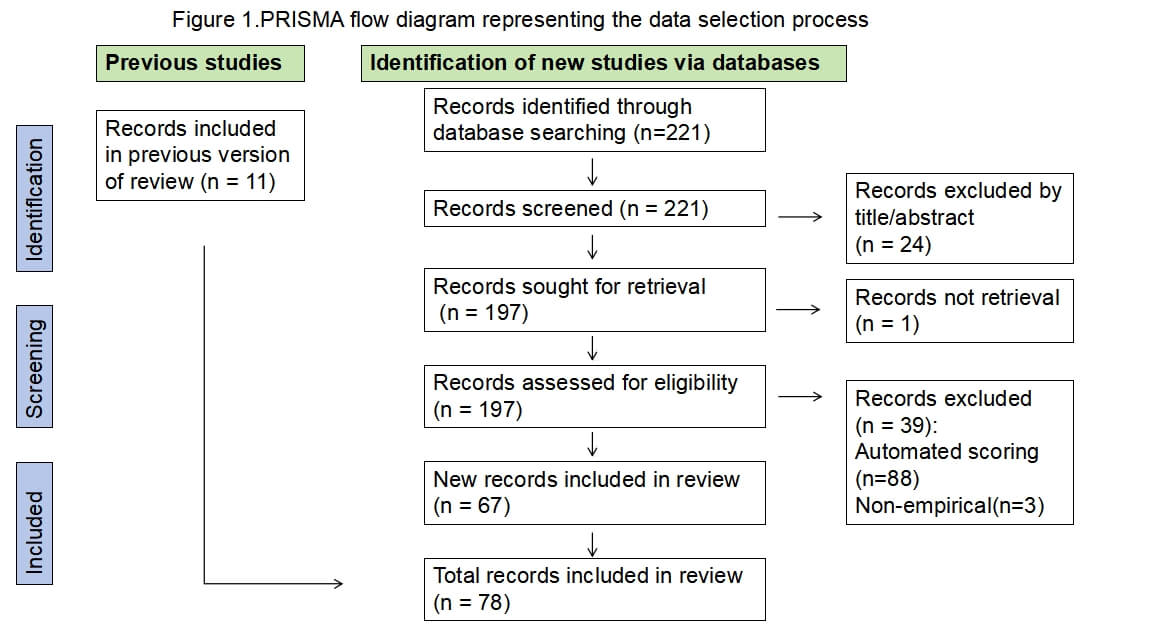
Keywords
An essential biological process for all life on Earth, photosynthesis is the way that plants transform light energy into chemical energy and form the base of the food chain [1]. The chloroplasts located in the cytoplasm of the leaf pulp cells are the primary site of photosynthesis. The light reaction and the dark reaction are the two main phases of the photosynthetic process [2]. Primary reaction, electron transport, and photophosphorylation are all included in the light reaction stage; the carbon assimilation process is what is referred to as the dark reaction stage. The entire process of photosynthetic reactions begins with the light reaction. The protein complex and the chlorophyll molecules implanted in the cyst-like body’s membrane are essential to the process of light capture, which is the initial step of the light reaction. The second stage of the light response is electron transport, which occurs throughout the plant body in a variety of ways. Electron transfer is the second stage of the light reaction. To fuel the ensuing dark reaction, plants manufacture adenosine triphosphate (ATP) and nicotinamide adenine dinucleotide phosphate (NADPH) through the light reaction. In essence, carbon assimilation—the dark reaction stage of photosynthesis—fixes and transforms inorganic carbon into organic carbon. Many mechanisms are used by autotrophs to fix carbon, although most of them involve the Calvin cycle [3]. Most organisms get the materials and energy they need to maintain their normal growth from plant photosynthesis, which is regulated by several factors such as light, CO2 concentration, temperature, water, and so forth. Numerous artificial and semi-artificial solutions have been shown to boost photosynthetic efficiency in direct or indirect ways, which is necessary to effectively improve photosynthesis and raise agricultural yields. Because photosynthesis is the main source of organic matter and oxygen, it is crucial for preserving ecological balance and sustaining human civilization [4]. It is imperative to increase agricultural output to fulfill the demands of a growing global population while simultaneously addressing concerns about environmental sustainability, as the world’s population is expected to reach 9.7 billion by 2050 [5].
Traditional/conventional fertilizers have been widely used to boost crop yield [6], but they often come with drawbacks such as excessive nutrient runoff, soil degradation, and negative impact on beneficial organisms [7]. These issues have led researchers to explore alternative approaches to fertilization, with nanofertilizers (NMs) emerging as a promising innovation in modern agriculture [8]. Nanofertilizers are designed to deliver nutrients at the molecular level, potentially offering improved efficiency, reduced waste, and better environment compatibility than conventional fertilizers [9]. Using the special qualities of nanoparticles—their small size, wide surface area, and capacity to focus on particular biological processes—nanofertilizers provide a fresh means of promoting photosynthesis and plant growth [10]. Nano-fertilizers significantly improve the efficiency of nutrient utilization, high crop yields, increase plant quality and, as a result, make plants more resilient to environmental pressures and a friendly plant-growing environment [11]. Nano-fertilizers’ slow-release method can greatly increase nutrient uptake, drastically lower nutrient seepage into groundwater, and safeguard the environment when compared to conventional fertilizers [7]. Kottegoda et al. infiltrated urea molecules under pressure into hydroxyapatite NMS, which has good biocompatibility, to achieve rapid release of nitrogen nutrients in the early stages and slow release in the later stages [12]. Field trials showed that this material reduced on-farm urea use by 50% and increased rice yields by 0.6 tons per hectare [12]. Nano-fertilizers effectively improve plant nitrogen use efficiency through controlled release patterns, resulting in significant increases in plant yield [9] (Fig. 1).
Figure 1: The nano-fertilizer is superior to the traditional fertilizer in plant photosynthesis
However, the effects of nano fertilizers on plant physiology, particularly their impact on photosynthetic efficiency, need to be understood [13]. While some studies suggest potential benefits, others raise concerns about unintended consequences [14]. Understanding the mechanism of action of nano fertilizers and their long-term effects on plant performance and ecosystem health is crucial to maximizing their potential in agriculture [15]. This review aims to synthesize the current knowledge on the effect of nano fertilizer on photosynthetic efficiency in plants, examining both the potential benefits and limitations of this emerging technology. By exploring the scientific literature, in this review, we seek to contribute to going debate about the future of fertilization in agriculture and suggest nanotechnology for sustainable crop production in the face of global challenges.
Photosynthesis is an essential biochemical reaction on Earth [16]. The foundation of crop biomass buildup and yield creation is photosynthesis [17]. Chloroplasts, which function as micro-green energy producers through the process of photosynthesis, convert solar energy into commercially useful biomass products including minerals, sugars, and carbohydrates [18]. Enhancing photosynthesis is a crucial field of study for raising sustainable agricultural productivity and solving issues with global food security [19]. By photosynthesis, plants change carbon dioxide and water into carbohydrates and oxygen [20]. The antennas of the chloroplasts’ photosystems PSI and PSII—special membrane systems—capture light energy during the first light phase and transfer it to the reaction centers where photochemical processes take place. In this process, electrons are transferred from water to NADP+ by linear electron transfer, which also results in the synthesis of significant NADPH and ATP as well as cyclic and pseudocyclic electron transfer. The Calvin-Benson-Basel cycle (CBB) in the chloroplast stroma, which is the main element of the entire carbon metabolism, is the second stage of C3 species’ oxygen-expelling photosynthesis. It works by using the energy of ATP and NADPH reduction ability created by the light reaction [21] (Fig. 2).
Figure 2: Mechanism and effect of nano-fertilizer on photosynthesis
Improving photosynthesis is crucial for our nation’s food security since it can reduce the strain on arable land and raise demand for agricultural products [21]. Increasing photosynthesis is a good technique to increase crop yields. Several studies have demonstrated that using nano fertilizer improves plants’ ability to absorb solar energy, encourages the synthesis of chlorophyll, ferritin (Fd), ribulose bisphosphate carboxylase (Rubisco), promotes the exchange of gases in the leaves, and improves the efficiency of photosynthesis in plants, which, in turn, significantly improves the photosynthesis of plants [6]. For example, Wang et al. applied foliar sprays to Brassica napus using biomass-derived carbon points and showed that they may encourage the synthesis of chlorophyll, Fd, and Rubisco synthesis, further boosting the genes’ expression to raise the net photosynthetic rate, dry biomass, and fresh biomass [16]. Xing et al. found that manganese ferrite nanomaterials (MnFe2O4 NMs) treated tomato plants had a 20% increase in leaf chlorophyll content and significantly increased the expression of transmitter toxins, PsaA and PsbA in the leaves, increasing photosynthetic efficiency by a noteworthy 13.3% [22]. A research area with great potential for enhancing photosynthesis, which is necessary to address concerns about global food security and increase agricultural output in a sustainable manner [13].
3 Relationship between Nano-Fertilizers and Plant Photosynthesis 1
Conventional fertilizers are not able to maintain a single supply of fertilizer due to their high dosage, low nutritional concentration, and other shortcomings. Traditional fertilizers cannot compare to the advantages of nano fertilizer. It reduces loss and waste and achieves directed and quantitative nutrient transportation in plants by delivering nutrients in the form of nanoparticles. In addition, nano-fertilizers can boost crop tolerance to stress, boost photosynthesis, stimulate hormones regulate enzymes, and raise crop yields in comparison to conventional fertilizers. Compared to conventional fertilizers, which have particle sizes ranging from millimeters to microns, nano fertilizers typically have particle sizes between one and one hundred nanometers. This makes it possible for nano fertilizers to directly enter plant cell walls and increase the efficiency of nutrient uptake, which drastically lowers the quantity of fertilizer used and lessens damage to the environment. Studies have shown that nano nitrogen fertilizers can significantly improve plant nitrogen uptake efficiency when compared to conventional nitrogen fertilizers. The effectiveness of nano nitrogen fertilizers, which can supply a plant with more nitrogen in a smaller amount and increase its biomass, was found to be unaffected by the application method in a comparison experiment between surface irrigation and drip irrigation [23]. Because of their unique, ultra-fine particle size and hydrophobic properties, which prevent them from easily being washed or leached by rainfall, nano-fertilizers can be suspended and distributed in the soil with more effectiveness, hence reducing nutrient losses. According to Liu et al., when compared to soybeans treated with conventional phosphorus fertilizers (Ca(H2PO4)2), synthetic apatite nanoparticles were able to increase soybean growth and seed yield, decrease the loss of phosphorus nutrients, and improve the nutrient quality of crops by increasing phosphorus uptake and utilization. With the use of nano-fertilizers, soybean biomass rose above ground and below ground by 18.2% and 41.2%, respectively [24]. Furthermore, the soil readily immobilizes the components in conventional fertilizers when they are applied; but, nanoparticles can combine with soil particles to create a complex that extends the time that nutrients release into the soil, resulting in a continual supply of fertilizers. The effectiveness of nutrient uptake is increased by the high specific surface area of nano-fertilizers, which facilitates wide interaction between the fertilizer and the soil’s organic matter or plant roots. Additionally, less waste and nutrient loss occur due to the gradual release of nano fertilizers. By using nano fertilizers, the risk of contaminating soil and water resources is decreased, as well as nutrient loss and waste. Additionally, because of increased crop growth, fewer pesticides are used, further safeguarding the natural environment [23].
Nanomaterials have tunable surface properties, and special electronic, magnetic, and optical properties. There are several potential applications for nanotechnology in agricultural productivity [25]. Photosynthetic mechanisms in plants can be modified by interventions such as nanomaterial-mediated delivery processes [26]. On the one hand, by increasing the amount of chlorophyll [27], converting light into energy, and speeding up the exchange of electrons during photosynthesis, nano-fertilizers can increase the efficiency of light utilization by plants [28] (Fig. 3). By improving crop tolerance to environmental challenges and nutrient utilization efficiency, nano-fertilizers can guarantee the correct operation of photosynthesis. Numerous studies have shown that NMs containing essential trace elements for plants can promote crop germination, nutrient growth, and yield, and increase a plant’s resistance to external stressors [29]. Rui et al. used silica nanomaterials (nSiO2) and silicon carbide nanomaterials (nSiC) applied to rice, and demonstrated that rice treated with nano-fertilizers had higher levels of aboveground peroxidase activity, more trace elements like magnesium, copper, and zinc, and enhanced photosynthesis compared to rice treated with regular bulk silica (bSiO2) and sodium silicate (Na2SiO3) [30]. Lu et al. used Mn3O4NPs for foliar spraying of cucumber seedlings, and the results showed that Mn3O4NPs significantly increased the quantity of biomass, net photosynthesis, and photosynthetic pigment in leaves, thus promoting the growth of cucumber. Nano photosynthesis enhancers can increase crop electron transfer rate and plant utilization of light, thus promoting photosynthesis [31]. When compared to traditional fertilizers, Rui et al. demonstrated that applying low-dose molybdenum dioxide nanoparticles (MoS2 NPs) to soybeans increased food yield by 30%. In their studies, Kumar et al. discovered that 150 ppm of Zinc oxide nanoparticles (ZnO NPs) enhanced the vigor index by 51% and the germination rate of pearl barley by 20% [32]. Prakash et al. unequivocally showed that applying ZnONPs significantly increased rice seedling development and tolerance to stress brought on by Cr exposure. The alleviation of Cr stress by ZnONPs was linked to enhanced photosynthetic efficiency, decreased oxidative indicators, positive regulation of important antioxidant genes, and optimized AsA-GSH cycle functioning [15]. In comparison to the control group, the application of titanium dioxide nanoparticles (TiO2NPs) to the leaf surface of brassica napus seedlings resulted in a considerable increase in the plants’ biomass, total phosphorus, and catalase activity, as reported by Hong et al. TiO2 NPs can boost brassica napus’s chlorophyll content, which will aid in the plant’s photosynthesis [33]. The mechanism by which TiO2 NPs can promote the growth of rice increases the accumulation of energy during photosynthesis and reduces energy consumption during metabolism, which in turn promotes the growth of rice, was discovered by Zhang et al. to be true. Applying different concentrations of TiO2 NPs to rice increased biomass by 32.8%, 35.7%, 38.9%, and 37.7%, respectively [34]. The aforementioned studies demonstrate that using nanotechnology to boost crop yields under abiotic stressors and alleviate global food poverty can be a sustainable and successful strategy [35].
Figure 3: Application of nano-fertilizer
In addition to positive effects, NMs may also have a variety of toxic effects on soybeans due to differences in species, size, indicated properties, concentration, and mode of application [36]. The mechanisms of phytotoxic effects of NMs mainly include NMs-induced reactive oxygen species (ROS) production, physical damage to plants by NMs, phytotoxicity due to chemical transformation of NMs, and phytotoxicity due to interaction of NMs with biomolecules such as genes, proteins, and metabolites in the plant [37]. The mechanism of toxic effects of NMs on plants causes oxidative stress, photodamage, and metabolic disorders in plant cells, affecting nutrient uptake and inhibiting photosynthesis, ultimately leading to plant growth inhibition [36]. The agricultural application of NMs is aimed at crop yield and reducing environmental impacts, while toxic effects limit the safe agricultural use of NMs, therefore minimizing negative effects is the primary prerequisite for the agricultural application of NMs [38].
An examination of over forty reports from the previous five years is covered in the paper’s next section, which concluded that nano-fertilizers can promote plant photosynthesis, improve crop photosynthetic efficiency, increase plant biomass, leaf length and width, and nutrient uptake efficiency. At the same time, improper use of nano fertilizers can inhibit plant photosynthesis and inhibit plant growth and development.
3.1 NMs’ Beneficial Impact on Plants
Plant growth the amount of chlorophyll in their leaves, and the efficiency of photosynthetic processes can all be increased by using nano fertilizer [39]. Nutrients required by plants regulate photosynthesis in many different ways. For example, copper can be involved in the electron transfer of photosynthesis, and zinc has a role in the synthesis of several proteins as well as plant chlorophyll. Micronutrient nanofertilizers supply plants with micronutrients like molybdenum, iron, manganese, zinc, copper, and manganese. Nanoscale forms of nutrients can increase the bioavailability of these important elements [40] (Table 1). For instance, iron is an essential trace component for crops and is involved in numerous physiological metabolic processes, including protein synthesis, fixing nitrogen, photosynthesis, and others [41]. Xiong treated maize seeds using four concentrations of zero-valent iron nanoparticles (nZVI). Through the stimulation of Rubisco and RCA activities, it was demonstrated that suitable dosages of nZVI considerably enhanced the photosynthesis potential and photosynthetic carbon accumulation of maize seedlings, hence promoting the formation and growth of maize plants [42]. The effects of co-exposing rice seedlings to Cd (100 μM) and 50~200 mg/L of hydroxyapatite nanoparticles (nHA), iron oxide nanoparticles (nFe2O3), or nZVI were examined by Rui et al. It was demonstrated that all three kinds of nanoparticles greatly decreased the aboveground Cd bio-enrichment in rice, encouraged plant development, and raised the height, aboveground biomass, and root biomass of rice plants to varying degrees [43]. The chapter describes the application of nano-fertilizers that can improve photosynthetic efficiency, biomass, nutrient uptake efficiency, chlorophyll, and light in plants where current research is more concerned (Fig. 4).
Figure 4: NMs’ beneficial impact on plants
3.1.1 Improvement of Photosynthetic Efficiency
Photosynthesis is widely recognized as the key to increasing crop yields and satisfying the expanding global food demand [44]. Plant growth and development, resistance-building, photosynthetic regulation, and fertilizer consumption efficiency are all areas in which nano-fertilizers can be highly effective. Carbon dots (CDs) are a significant class of nanomaterials that are usually round particles that have a diameter of fewer than ten nanometers they are well adapted to be used in the biological field because of their good water solubility, photoluminescence, ease of regulation, low toxicity, and biocompatibility [45]. Several studies have demonstrated the CDs’ amazing capacity to promote plant development and development, regulate plant photosynthesis, and improve plant stress tolerance [46]. Intrinsic mechanism of CDs in regulating plant photosynthetic efficiency N-CDs can significantly promote photosynthetic efficiency and fruit soluble solids accumulation in apple trees. Wang et al. found that N-CDs are capable of giving electrons, transferring electrons generated under light excitation to plastoquinone in the photosynthetic electron transport chain, and improve photosynthesis by accelerating the redox cycle of plastoquinone, which can significantly improve the photosynthetic utilization efficiency of apples and promote the great potential in the quality of fruits [47]. In addition, Liu et al. applied foliar sprays of Mg, and N-CDs to rice plants and showed that N-CDs enhanced the efficiency of light energy use by preventing the loss of excitation energy absorbed by photosynthetic system II to thermal energy [48]. The Rubisco enzyme, a crucial component of the Calvin cycle, was intrinsically stimulated by the carbon sites on N-CDs, which sped up the uptake of CO2 during photosynthesis. Final growth acceleration of Mg, N-CDs-treated rice plants [48].
Numerous studies have demonstrated that the application of various nanofertilizers, in addition to CDs, can enhance plants’ photosynthetic efficiency. At low concentrations, TiO2NPs can help plants absorb more light energy, speed up the electron transfer process in the photoreaction centers, and encourage photolysis of water and NADPH reduction. All of these effects can enhance photosynthetic efficiency and encourage the growth of tomato plants [49]. When compared to traditional Fe sources, it was discovered that FeNPs greatly improved wheat growth, photosynthetic efficiency, and uptake of critical nutrients in both normal and saline soils. The study’s findings showed that adding different Fe sources significantly improved wheat plants’ capacity for photosynthetic activity and gas exchange [50]. Numerous investigations have exhibited the advantageous impacts of nano fertilizers on the growth and photosynthesis of maize. Corn growth, including plant height, dry matter biomass, root biomass, and nutrient uptake such as nitrogen, phosphorus, and potassium, can be greatly enhanced with nano fertilizers. Two popular application techniques are foliar spraying and soil watering, with foliar spraying proving especially successful. These results give nano-fertilizers a scientific foundation for use in agricultural production, potentially increasing corn output and quality. In addition to promoting the uptake and use of mineral nutrients, carbon nanoparticles (CNPs) treatment resulted in a considerable increase in maize plant height, aboveground dry matter biomass, and root dry matter biomass. It also changed the chemical and biochemical properties of the soil. Depending on the concentration and particle size of the nano fertilizers, using CNPs can benefit plants and soil in a number of ways. On the other hand, they can greatly enhance soil quality and encourage plant development [51]. Multiple ZnONP treatments increased the accumulation of H2O2 and ABA in the barley root system and reduced root elongation and leaf growth, as demonstrated by Guo et al. [52]. Although they offer potential for use in agricultural production, nano fertilizers must be used cautiously to prevent harm to plants. When using nano-fertilizers on barley, much thought must be given to how they may affect photosynthesis and other physiological functions. From the foregoing, it is evident that nano fertilizer can enhance plant photosynthetic efficiency, enhance fertilizer use efficiency, and encourage plant growth and development.
3.1.2 Increasing Plant Biomass
One of the main factors influencing crop output, along with biomass and seed yield, is photosynthetic efficiency [53]. Plant growth and development are significantly influenced by macro- and micro-elements, particularly in photosynthesis. Iron is a necessary component of photosynthesis since it is an enzyme cofactor, and iron deficiency will lead to yellowing and necrosis of plants, while the slow release of Fe from Fe-containing nanoparticles improves the utilization rate of Fe in plants and makes it easier to be absorbed by plants [10]. Tombuloglu et al. demonstrated that 20–40 nm Fe-containing NMS exponentially increased root length and root dry and fresh weight and leaf dry and fresh weight in wheat, with the highest growth transporter efficiency, the strongest promotion, and the highest plant chlorophyll content and biomass [54]. Two iron-based (Fe and Fe3O4) nanoparticles were sprayed onto the leaves of maize plants by Li et al. The findings showed that the iron-based nanomaterials significantly increased the chlorophyll content, net photosynthetic rate, and leaf biomass of maize plants, which positively affected the plants’ growth [10]. Rui et al. treated cotton with Fe2O3 NPs and showed that Fe2O3 NPs promoted root hairs and biomass in conventional cotton [55].
In a similar vein, numerous studies demonstrate that applying additional nano-fertilizers can additionally stimulate plant growth. Mn is a necessary component for several enzymes involved in photosynthesis to function. According to Ye et al., MnNPs considerably boosted the Capsicum annuum L. root length and biomass when compared to Mnso4, the traditional manganese fertilizer, improving the production of the crop [56]. Thus, it can be concluded that, at certain concentrations, nano-fertilizers improve plant development and chlorophyll content. However, particular attention must be given to the concentration and particle size of the fertilizer during application.
3.1.3 Effects on Pigmentation and Gas Exchange
In the plant photosynthetic system, chlorophyll, photosynthetic proteins, and enzymes play crucial roles in the absorption, conversion, and transport of light energy [48]. By foliar spraying Mg, N-CDs on rice seedlings, Liu et al. demonstrated how Mg, N-CDs can enhance chlorophyll metabolism by controlling the expression of genes linked to chlorophyll metabolism, so as to keep its content and activity high in the plant, and thus promote its role in photosynthesis [48]. Crop photosynthesis can be aided by photocatalysts such as TiO2NPs. According to Wang et al. ’s application of varying concentrations of TiO2NPs to tomatoes, low concentrations of TiO2NPs considerably enhanced the content of chlorophyll a and chlorophyll b, hence promoting photosynthesis in tomato plants [57]. Chlorophyll content (SPDA value) and gas exchange parameters (Pn, Tr, Ci, Gs) are important indicators of plant photosynthesis in response to NMs in the environment. Stomatal value is the main site of gas exchange between plants and the outside world, and gas exchange parameters (Pn, Tr, Ci, Gs) are key factors affecting plant photosynthesis [58]. In Rui’s study, it was found that 10 mg/kg MoS2NPs increased the Gs and SPAD values of soybeans, and the increase in Gs could promote the raw material for photosynthesis, CO2, to enter the plant, and the plant absorbed the light energy through the photosynthetic pigments, and converted the CO2 into carbohydrates necessary for the plant’s growth, which promoted photosynthesis of soybeans, and thus increased the plant’s biomass and yield [59]. It was demonstrated that moderate doses of nZVI stimulated the overexpression of the AHA2 gene and increased plasma membrane (PM) H+ -ATPase activity in Arabidopsis thaliana [40]. Plant nutrient absorption, cell division, and stomatal regulation are just a few of the physiological activities that depend on plasma membrane H+ -ATPase, or PM H+ -ATPase. Thus, increasing stomatal openness and facilitating CO2 uptake through overexpression of PM H+ -ATPase improves photosynthesis [40]. It is evident that nano-fertilizers can boost the amount of chlorophyll in crops and encourage the flow of leaf gases; nevertheless, it should be noted that various plants may require varying concentrations of fertilizer.
The Earth’s oxygen-rich atmosphere has dictated the evolution of multicellular life forms, and numerous creatures rely on oxygen to survive, physiological processes involving oxygen molecules (O2) happen constantly in cells [60]. These processes result in the production of ROS since O2 changes both with energy and electrons [61]. In plant cells, the plasmodesmata and different organelles like mitochondria, chloroplasts, and peroxisomes are the primary locations where ROS are produced [62]. These areas essentially contain antioxidant systems to buffer the local redox environment. Chloroplasts are the site of photosynthesis. Under light conditions, photosystems I and II produce ROS, and the main types of ROS are O2−, H2O2, and 1O2 [62]. For each of these types of ROS, chloroplasts have corresponding systems for their removal. However, plant injury is primarily caused by the buildup of reactive oxygen species (ROS) under stress, and excessive ROS can cause plant cell damage or even death [63]. ROS damage plant organelles inhibit photosynthesis and are the primary source of plant oxidative stress. High levels of ROS production in chloroplasts impede photosynthesis and reduce crop yields [64]. Nanomaterials protect plants from oxidative damage by scavenging reactive oxygen species, promoting the uptake and distribution of stress hormones, boosting antioxidant enzymes in plants, and reducing damage to cell membranes, DNA, proteins, and other biological components. Numerous studies have demonstrated that because of their special chemical and physical characteristics, nano fertilizers have enzymatic activities that scavenge ROS and prevent oxidative damage to proteins, including organelles and nitrogen-fixing enzymes [65]. For example, cerium oxide nanoparticles (CeO2NPs) have superoxide dismutase (SOD) activity, which can catalyze the decomposition of oxygen radicals and hydrogen peroxide can catalyze the decomposition of ROS through the redox reaction between the oxidized state of Ce3+/4+ and the reduced state of Ce [66]. Wu et al. applied CeO2NPs to Arabidopsis thaliana plants and showed that negatively charged CeO2NPs could effectively enhance plant photosynthesis by trapping and quenching ROS in chloroplasts [67]. MnsO4NPs also have excellent antioxidant-like enzyme functions, the coexistence of reduced and oxidized Mn2+ in MnsO4NPs, and the switching of II and T valence analogous to a redox reaction endowed MnsO4 NPS with the ability to scavenge ROS [68]. ZnO NPs dramatically reduced the toxicity of chromium (Cr) stress on rice seedlings, as demonstrated by Prakash et al. This primarily has to do with how ZnO NPs influence the functional relationships between antioxidant enzyme activities of ascorbate peroxidase (APX), glutathione dehydrogenase (ascorbate) (DHAR), reductase (MDHAR), and glutathione reductase (GR). Thus, the amount of antioxidant enzymes in seedlings exposed to Cr through ZnO NPs treatment was considerably higher than the amount in vivo in rice plants that were not treated [69]. In the meantime, by enhancing their physiological and molecular mechanisms, nanofertilizers can improve plant resistance to salinity and drought stress. Stress hormones have a significant role in how plants respond to salt and drought, and nanofertilizers can enhance plant tolerance to stress by promoting the uptake and dispersion of stress hormones. For instance, Sun et al. demonstrated that Fe3O4 nanoparticles can increase the concentration of stress hormones like abscisic acid (ABA) in plants, hence improving their tolerance to stress [70].
However, under other conditions, NMs may also induce ROS accumulation and toxicity to crops, which is also affected by a variety of factors, including plant species, growing environments, exposure duration, and concentration [71]. For example, Nair et al. showed that ZnO-NPs-treated seedlings resulted in more buildup of zinc in the tissue, more formation of ROS, decreased viability of root cells, more MDA in the leaves, less chlorophyll and carotenoid content, and inhibition of plant growth and development [72]. The above studies have shown that nano-fertilizers are capable of antioxidant-like enzyme activities that can scavenge ROS to reduce oxidative damage to proteins such as nitrogen-fixing enzymes as well as organelles and to improve plant photosynthesis. However, NMs can also produce stress, generate excessive ROS, and inhibit plant growth. Therefore, considerations like the type of fertilizer used, the growth environment, and the type of plant should all be taken into account while administering NMs, and the concentration and duration of exposure.
3.1.5 Improving Plant Stress Resistance
Reduced plant photosynthesis leads to decreased agricultural output and quality. Abiotic stressors, biocompression, inefficient use of fertilizers and pesticides, and soil degradation all contribute to this. Herbivore eating and pathogen infestation are two major biological stressors that have an impact on crop production. Conventional pesticides pose harm to human health and the environment even when they boost crop yields. Ag, Cu, and Al nanoparticles are examples of nanoparticles with antibacterial and pest-controlling characteristics. In a field trial, Cromwell discovered that 150 mg/mL of silver-based nanoparticles decreased nematode populations by 82% and 92% at d 2 and d 4 post-treatment, respectively [73]. The primary factors reducing agricultural yields globally include abiotic stresses, such as drought, high temperatures, high salinities, cold, nutrient shortages, chemical toxicity (such as heavy metals), and oxidative stresses. For example, drought stress causes damage to soybeans by reducing photosynthesis and inhibiting the synthesis of photosynthetic pigments. Cold stress reduces the rate of biochemical reactions in soybeans and affects gene expression in the plant, thereby triggering a decline in plant functions including water and nutrient uptake, cell membrane fluidity, photosynthesis, protein synthesis, and cellular metabolism [74]. According to Hanif et al., ZnO NPs, proline, and betaine safeguard photosynthetic machinery and improve membrane stability, nutrient and water uptake, and plant resistance to drought stress [75]. By balancing plant oxidation levels and controlling phytohormone levels, the use of nanomaterials in agriculture can preserve normal plant growth even in the face of drought and high temperatures. Plants that are exposed to abiotic adversity can withstand it thanks to the ability of nanomaterials to activate and control genes linked to adversity. Heat shock and water channel proteins become more active as a result. Nanomaterials have targeted transportation and delayed release compared to organic compounds. These advantages help plants resist heat stress and drought thanks to nanomaterials [76]. Stress from low temperatures and salt greatly affects photosynthesis. Osmotic stress from salt and low-temperature stress reduces photosynthesis through stomatal and non-stomatal processes. Lower CO2 concentration in the leaves is primarily caused by the former, which is primarily caused by a decreased stomatal aperture, an uneven stomatal spatial distribution, or a stomatal density. Salt and low temperature stress are two examples of non-stomatal factors that cause excessive production of ROS in photosynthetic tissues. In photosynthetic tissues, increased ROS generation is a result of low-temperature stress and salt. Plant yields drop as a result [77].
Because of their unique physicochemical characteristics, NMs can help reduce stress and promote plant development [78]. Abiotic stress on plant growth is decreased by the easily absorbed, physiologically active nano-sized silicon particles (SiO2-NPs) [77]. In the end, it enhanced crop growth and photosynthesis. According to Naidu et al., low-temperature doubly stressed cotton seedlings may withstand some degree of growth damage from SiO2-NPs applied at a certain concentration SiO2-NPs [79]. This was observed under salt-low-temperature doubly stressed conditions. The stomatal state was regulated, PSII’s light energy utilization efficiency and electron transfer activity were improved, an increase in Rubisco activity was induced, and the carbon assimilation capacity under low temperature and double salt stress was increased, all of which contributed to the enhancement of cotton seedling photosynthesis, minimizing harm to cotton seedling development when exposed to both low temperature and salt stress [77]. One of the factors contributing to the decline in crop yield is the growing severity of heavy metal pollution in agricultural soils, both in terms of contamination level and area. Because of their special physicochemical characteristics, nanofertilizers have been shown to have extraordinary effects on soil remediation, crop tolerance, and crop uptake of heavy metals. According to Shen et al., calcium hexacyanoferrate nanoparticles (CahCF NPs) can lessen the uptake of cadmium ions by ion exchange and mimic plants’ detoxifying mechanisms for heavy metals like cadmium, hence reducing the stressors caused by heavy metals on plant growth [80].
It is evident that using nano fertilizers can help plants withstand stress better and sustain less damage when under stress. However, the majority of research on the regulatory action of single salt or low-temperature stress on photosynthetic processes has not been done, which will be a new route. As a result, the influence of nms regulatory action on photosynthetic processes is mostly focused on these two types of stresses. In conclusion, spraying nano fertilizer is one of the trends to improve photosynthesis and alleviate various plant stresses.
3.2 Negative Effects of NMs on Plants
In addition to the positive effects, NMs may also have various toxic effects on plants due to differences in species, size, indication properties, concentration, application methods, etc. The mechanisms of NMs’ phytotoxic effects mainly include NMs-induced ROS production, physical damage to plants by NMs, phytotoxicity caused by the chemical transformation of NMs, and phytotoxicity caused by interaction with biomolecules, such as genes, proteins, and metabolites, in the plant [37]. Damage to DNA and proteins, lipid peroxidation, and oxidative stress can all result from high ROS levels, metabolic disorders, and impaired photosynthesis, ultimately leading to growth inhibition [36].
Metal-based NMs such as AIg NPS, CeO2NPS, CuONPs, and ZnONPS have been extensively researched for their potential use in resisting crop pests and diseases and improving crop nutrition, and their toxic effects on plants have also attracted much attention [81] (Table 2). Yuan et al. showed that Ag NPs disrupted mitosis by reducing the number of chromosomal aberrations and the number of micronuclei in plant cells, thereby inhibiting plant germination [82]. Size determined the toxicity of CuO NPs to soybeans; compared to bigger particles, small-sized (25 nm) CuO NPS considerably increased the amount of oxidative stress in soybeans [83]. High doses of nZVI reduced the stomatal conductance and photoreduction capacity of leaves compared to low concentrations, which in turn inhibited electron transfer, foliar gas exchange, and photosynthetic capacity. High doses of nZVI were also susceptible to peroxidation and osmoregulation of the plasma membrane, aggravating the burden on the antioxidant enzyme system [42]. Lopez-Moreno et al. showed that CeO2NPs do not affect soybean germination, but rather enter the soybean root and are genotoxic to soybeans [84]. The results of the above studies indicate that the type, exposure dose, size, surface properties, and harmful effects of NMs on plants are influenced by both plant species and NM variations.
The mechanism of toxic effects of NMs on plants cause oxidative stress, photodamage, and metabolic disorders in plant cells, affect the uptake of nutrients in the plant, and inhibit photosynthesis, which ultimately results in the suppression of plant development. The agricultural application of NMs is aimed at crop yield and reduction of environmental impacts, while the toxic effects limit the safe use of NMs in agriculture, so minimizing the negative effects is the first prerequisite for the agricultural applications of NMs. In the meantime, nanoparticles are frequently employed in emerging industries like personal care goods, industrial products, pharmaceuticals, and biomedicine, making them inevitably enter soil and water bodies, damaging plants and having an impact on human health via the food chain. Since photosynthesis is the foundation of plant growth and metabolic activity and one of the physiological processes most vulnerable to abiotic stresses, it is critical to evaluate NMs’ phytotoxicity systematically in order to advance contemporary agriculture’s ability to produce food security [85].
4 Conclusions and Future Perspectives
From the literature in recent years, it is evident that the use of nano fertilizers has garnered a lot of interest in how they may impact plant photosynthesis and can improve plant photosynthesis, crop yield and plant quality. Compared with traditional fertilizers, nano fertilizers can achieve precise delivery of nutrients through controlled release and targeting of the target site, improve the bioavailability of nutrients, significantly boost the amount of chlorophyll in plant leaves, enhance how well plants use light, and hence enhance photosynthesis (Fig. 5). Nano fertilizers are used in smaller quantities, making them more economical, by controlling or slowly releasing nutrients, which improves the efficiency of nutrient use and minimizes environmental impact.
Figure 5: The positive effect of nano-fertilizer on plant photosynthesis
Overall, nano-fertilizers can significantly promote plant photosynthesis and are an effective way to increase crop yields, providing a fantastic choice for sustainable agriculture with enormous application potential. However, regarding the toxicity and environmental residue of nanoparticles, there are still unresolved problems, particularly the potential harm that a broad release of NMs into the environment could do to human health (Fig. 6). There is still much to be done before significant amounts of nanofertilizers can be used in practical applications, including determining the ideal action concentration of various nanofertilizers on various plants. The issues of the present and the expectations for the future are discussed below:
1) The ideal application concentration varies depending on the crop, and in order to prevent plant growth suppression, extensive tests are required to find the ideal application concentration, application particle size, and application period.
2) A more thorough investigation of the mechanism of nanofertilizer-induced plant photosynthesis is required.
3) To identify the best kinds of nanofertilizers for various situations, comparative research on various nanofertilizers, such as ZnO-NPs, Fe2O3NPs, CDs, etc., is required.
4) Since there is still a long way to go before widespread adoption, concerns about toxicity, implications for human health, and financial gains should receive more attention.
Figure 6: Negative effects of nano-fertilizers on plant photosynthesis
Acknowledgement: We are grateful for the financial support from China Agricultural University, Professor Workstation in Tangshan Jinhai New Material Co., Shanghe County Baiqiao Town Science and Technology Courtyard, Hebei Wuqiang County Professor Workstation and Science and Technology Small Courtyard.
Funding Statement: This study was funded by the National Key R&D Program of China (2017YFD0801300; 2017YFD0801103) and the National Natural Science Foundation of China (32001014).
Author Contributions: Xinyi Liu: Writing—Original Draft, Visualization, and Conceptualization. Muhammed Nadeem: Writing—Review and Editing. Yukui Rui: Writing—Review and Editing, Supervision. All authors reviewed the results and approved the final version of the manuscript.
Availability of Data and Materials: The datasets analyzed during the current study are available from the corresponding author on reasonable request.
Ethics Approval: Not applicable.
Conflicts of Interest: The authors declare no conflicts of interest to report regarding the present study.
References
1. Qu YC, Sakoda K, Fukayama H, Kondo E, Suzuki Y, Makino A, et al. Overexpression of both Rubisco and Rubisco activase rescues rice photosynthesis and biomass under heat stress. Plant Cell Environ. 2021;44(7):2308–20. doi:10.1111/pce.14051. [Google Scholar] [PubMed] [CrossRef]
2. Johnson MP. Correction: photosynthesis. Essays Biochem. 2017;61(4):429–9. doi:10.1042/EBC20160016_COR. [Google Scholar] [PubMed] [CrossRef]
3. Schlüter U, Weber APM. The road to C4 photosynthesis: evolution of a complex trait via intermediary states. Plant Cell Physiol. 2016;57(5):881–9. doi:10.1093/pcp/pcw009. [Google Scholar] [PubMed] [CrossRef]
4. Cutolo EA, Guardini Z, Dall’Osto L, Bassi R. A paler shade of green: engineering cellular chlorophyll content to enhance photosynthesis in crowded environments. New Phytol. 2023;239(5):1567–83. doi:10.1111/nph.19064. [Google Scholar] [PubMed] [CrossRef]
5. Wijerathna-Yapa A, Pathirana R. Sustainable agro-food systems for addressing climate change and food security. Agriculture. 2022;12(10):1554. doi:10.3390/agriculture12101554. [Google Scholar] [CrossRef]
6. Singh H, Sharma A, Bhardwaj SK, Arya SK, Bhardwaj N, Khatri M. Recent advances in the applications of nano-agrochemicals for sustainable agricultural development. Environ Sci: Proc Imp. 2021;23(2):213–39. doi:10.1039/d0em00404a. [Google Scholar] [PubMed] [CrossRef]
7. Zulfiqar F, Navarro M, Ashraf M, Akram NA, Munné-Bosch S. Nanofertilizer use for sustainable agriculture: advantages and limitations. Plant Sci. 2019;289:110270. doi:10.1016/j.plantsci.2019.110270. [Google Scholar] [PubMed] [CrossRef]
8. Morales-Díaz AB, Ortega-Ortíz H, Juárez-Maldonado A, Cadenas-Pliego G, González-Morales S, Benavides-Mendoza A. Application of nanoelements in plant nutrition and its impact in ecosystems. Adv Nat Sci: Nanosci Nanotechnol. 2017;8(1):013001. doi:10.1088/2043-6254/8/1/013001. [Google Scholar] [CrossRef]
9. Verma KK, Song XP, Joshi A, Tian DD, Rajput VD, Singh M, et al. Recent trends in nano-fertilizers for sustainable agriculture under climate change for global food security. Nanomaterials. 2022;12(1):173. doi:10.3390/nano12010173. [Google Scholar] [PubMed] [CrossRef]
10. Li P, Wang A, Du W, Mao L, Wei ZB, Wang SF, et al. Insight into the interaction between Fe-based nanomaterials and maize (Zea mays) plants at metabolic level. Sci Total Environ. 2020;738(78):139795. doi:10.1016/j.scitotenv.2020.139795. [Google Scholar] [PubMed] [CrossRef]
11. Hofmann T, Lowry GV, Ghoshal S, Tufenkji N, Brambilla D, Dutcher JR, et al. Technology readiness and overcoming barriers to sustainably implement nanotechnology-enabled plant agriculture. Nat Food. 2020;1(7):416–25. doi:10.1038/s43016-020-0110-1. [Google Scholar] [CrossRef]
12. Raguraj S, Wijayathunga WMS, Gunaratne GP, Amali RKA, Priyadarshana G, Sandaruwan C, et al. Urea-hydroxyapatite nanohybrid as an efficient nutrient source in Camellia sinensis (L.) Kuntze (tea). J Plant Nutr. 2020;43(15):2383–94. doi:10.1080/01904167.2020.1771576. [Google Scholar] [CrossRef]
13. Matthews ML, Burgess SJ. How much could improving photosynthesis increase crop yields? A call for systems-level perspectives to guide engineering strategies. Curr Opin Biotechnol. 2024;88:103144. doi:10.1016/j.copbio.2024.103144. [Google Scholar] [PubMed] [CrossRef]
14. Kah M, Kookana RS, Gogos A, Bucheli TD. A critical evaluation of nanopesticides and nanofertilizers against their conventional analogues. Nat Nanotechnol. 2018;13(8):677–84. doi:10.1038/s41565-018-0131-1. [Google Scholar] [PubMed] [CrossRef]
15. Prakash V, Rai P, Sharma NC, Singh VP, Tripathi DK, Sharma S, et al. Application of zinc oxide nanoparticles as fertilizer boosts growth in rice plant and alleviates chromium stress by regulating genes involved in oxidative stress. Chemosphere. 2022;303(1):134554. doi:10.1016/j.chemosphere.2022.134554. [Google Scholar] [PubMed] [CrossRef]
16. Cheng B, Yang Z, Chen F, Yue L, Cao X, Li J, et al. Biomass-derived carbon dots with light conversion and nutrient provisioning capabilities facilitate plant photosynthesis. Sci Total Environ. 2023;901(9):165973. doi:10.1016/j.scitotenv.2023.165973. [Google Scholar] [PubMed] [CrossRef]
17. Yang Y, Chen M, Tian J, Xiao F, Xu S, Zuo W, et al. Improved photosynthetic capacity during the mid- and late reproductive stages contributed to increased cotton yield across four breeding eras in Xinjiang, China. Field Crops Res. 2019;240:177–84. doi:10.1016/j.fcr.2018.11.003. [Google Scholar] [CrossRef]
18. Sørensen M, Andersen-Ranberg J, Hankamer B, Møller BL. Circular biomanufacturing through harvesting solar energy and CO2. Trends Plant Sci. 2022;27(7):655–73. doi:10.1016/j.tplants.2022.03.001. [Google Scholar] [PubMed] [CrossRef]
19. Smith EN, van Aalst M, Tosens T, Niinemets Ü, Stich B, Morosinotto T, et al. Improving photosynthetic efficiency toward food security: strategies, advances, and perspectives. Mol Plant. 2023;16(10):1547–63. doi:10.1016/j.molp.2023.08.017. [Google Scholar] [PubMed] [CrossRef]
20. Guirguis A, Yang W, Conlan XA, Kong L, Cahill DM, Wang Y. Boosting plant photosynthesis with carbon dots: a critical review of performance and prospects. Small. 2023;19(43):2300671. doi:10.1002/smll.202300671. [Google Scholar] [PubMed] [CrossRef]
21. Schansker G. Determining photosynthetic control, a probe for the balance between electron transport and Calvin-Benson cycle activity, with the DUAL-KLAS-NIR. Photosynth Res. 2022;153(3):191–204. doi:10.1007/s11120-022-00934-7. [Google Scholar] [PubMed] [CrossRef]
22. Yue L, Feng Y, Ma C, Wang C, Chen F, Cao X, et al. Molecular mechanisms of early flowering in tomatoes induced by manganese ferrite (MnFe2O4) nanomaterials. ACS Nano. 2022;16(4):5636–46. doi:10.1021/acsnano.1c10602. [Google Scholar] [PubMed] [CrossRef]
23. Sun Y, Zhu G, Zhao W, Jiang Y, Wang Q, Wang Q, et al. Engineered nanomaterials for improving the nutritional quality of agricultural products: a review. Nanomaterials. 2022;12(23):4219. doi:10.3390/nano12234219. [Google Scholar] [PubMed] [CrossRef]
24. Liu R, Lal R. Synthetic apatite nanoparticles as a phosphorus fertilizer for soybean (Glycine max). Sci Rep. 2014;4(1):5686. doi:10.1038/srep05686. [Google Scholar] [PubMed] [CrossRef]
25. Vaidya S, Deng C, Wang Y, Zuverza-Mena N, Dimkpa V. White J Nanotechnology in agriculture: a solution to global food insecurity in a changing climate? NanoImpact. 2024;34:100502. doi:10.1016/j.impact.2024.100502. [Google Scholar] [PubMed] [CrossRef]
26. Wang Z, Zhang Y, Zhang S, Ge M, Zhang H, Wang S, et al. Natural xylose-derived carbon dots towards efficient semi-artificial photosynthesis. J Colloid Interface Sci. 2023;629(6):12–21. doi:10.1016/j.jcis.2022.09.044. [Google Scholar] [PubMed] [CrossRef]
27. Li M, Zhang P, Adeel M, Guo Z, Chetwynd A, Ma C, et al. Physiological impacts of zero valent iron, Fe3O4 and Fe2O3 nanoparticles in rice plants and their potential as Fe fertilizers. Environ Pollut. 2021;269:116134. doi:10.1016/j.envpol.2020.116134. [Google Scholar] [PubMed] [CrossRef]
28. Hu J, Jia W, Wu X, Zhang H, Wang Y, Liu J, et al. Carbon dots can strongly promote photosynthesis in lettuce (Lactuca sativa L.). Environ Sci: Nano. 2022;9(4):1530–40. doi:10.1039/d1en00948f. [Google Scholar] [CrossRef]
29. Nguyễn CT, Gammatantrawet N, Susawaengsup C, Tandee K, Ramli ANM, Tongkoom K, et al. Drought-ready plant resilience: harnessing nano-biotechnology techniques for swift screening and selection of organic crop varieties. S Afr J Bot. 2024;169(1):553–66. doi:10.1016/j.sajb.2024.05.011. [Google Scholar] [CrossRef]
30. Jiang Y, Yang J, Li M, Li Y, Zhou P, Wang Q, et al. Effect of silica-based nanomaterials on seed germination and seedling growth of rice (Oryza sativa L.). Nanomaterials. 2022;12(23):4160. doi:10.3390/nano12234160. [Google Scholar] [PubMed] [CrossRef]
31. Lu L, Huang M, Huang Y, Corvini P, Ji R, Zhao L. Mn3O4 nanozymes boost endogenous antioxidant metabolites in cucumber (Cucumis sativus) plant and enhance resistance to salinity stress. Environ Sci: Nano. 2020;7(6):1692–1703. doi:10.1039/D0EN00214C. [Google Scholar] [CrossRef]
32. Kumar R, Dadhich A, Dhiman M, Sharma L, Sharma MM. Stimulatory effect of ZnO nanoparticles as a nanofertilizer in seed priming of pearl millet (Pennisetum glaucum) and their bioactivity studies. S Afr J Bot. 2024;165(3):30–8. doi:10.1016/j.sajb.2023.12.001. [Google Scholar] [CrossRef]
33. Wei L, Ji L, Rico C, He C, Shakoor L, Fakunle M, et al. Transcriptomics reveals the pathway for increasing Brassica chinensis L. Yield under foliar application of titanium oxide nanoparticles. J Agric Food Chem. 2024;72(34):18957–70. doi:10.1021/acs.jafc.4c04075. [Google Scholar] [PubMed] [CrossRef]
34. Zhang Y, Liu N, Wang W, Sun J, Zhu L. Photosynthesis and related metabolic mechanism of promoted rice (Oryza sativa L.) growth by TiO2 nanoparticles. Front Environ Sci Eng. 2020;14(6):103. doi:10.1007/s11783-020-1282-5. [Google Scholar] [CrossRef]
35. Li M, Zhang P, Guo Z, Cao W, Gao L, Li Y, et al. Molybdenum nanofertilizer boosts biological nitrogen fixation and yield of soybean through delaying nodule senescence and nutrition enhancement. ACS Nano. 2023;17(15):14761–74. doi:10.1021/acsnano.3c02783. [Google Scholar] [PubMed] [CrossRef]
36. Jogaiah S, Paidi MK, Venugopal K, Geetha N, Mujtaba M, Udikeri S, et al. Phytotoxicological effects of engineered nanoparticles: an emerging nanotoxicology. Sci Total Environ. 2021;801(1):149809. doi:10.1016/j.scitotenv.2021.149809. [Google Scholar] [PubMed] [CrossRef]
37. Sun W, Dou F, Li C, Ma X, Ma L. Impacts of metallic nanoparticles and transformed products on soil health. Crit Rev Environ Sci Technol. 2021;51(10):973–1002. doi:10.1080/10643389.2020.1740546. [Google Scholar] [CrossRef]
38. Ray MK, Mishra AK, Mohanta YK, Mahanta S, Chakrabartty I, Kungwani N, et al. Nanotechnology as a promising tool against phytopathogens: a futuristic approach to agriculture. Agriculture. 2023;13(9):1856. doi:10.3390/agriculture13091856. [Google Scholar] [CrossRef]
39. Gao X, Kundu A, Persson DP, Szameitat A, Minutello F, Husted S, et al. Application of ZnO nanoparticles encapsulated in mesoporous silica on the abaxial side of a Solanum lycopersicum leaf enhances Zn uptake and translocation via the phloem. Environ Sci Technol. 2023;57(51):21704–14. doi:10.1021/acs.est.3c06424. [Google Scholar] [PubMed] [CrossRef]
40. Yoon H, Kang YG, Chang YS, Kim J. Effects of zerovalent iron nanoparticles on photosynthesis and biochemical adaptation of soil-grown Arabidopsis thaliana. Nanomaterials. 2019;9(11):1543. doi:10.3390/nano9111543. [Google Scholar] [PubMed] [CrossRef]
41. Shakoor N, Adeel M, Zain M, Zhang P, Ahmad MA, Farooq T, et al. Exposure of cherry radish (Raphanus sativus L. var. Radculus Pers) to iron-based nanoparticles enhances its nutritional quality by trigging the essential elements. NanoImpact. 2022;25:100388. doi:10.1016/j.impact.2022.100388. [Google Scholar] [PubMed] [CrossRef]
42. Yang Y, Zhu Y, Naseer M, Wang Q, Li G, Tao H, et al. Rhizosphere effect of nanoscale zero-valent iron on mycorrhiza-dependent maize assimilation. Plant, Cell Environ. 2023;46(1):251–67. doi:10.1111/pce.14478. [Google Scholar] [PubMed] [CrossRef]
43. Zhou P, Zhang P, He M, Cao Y, Adeel M, Shakoor N, et al. Iron-based nanomaterials reduce cadmium toxicity in rice (Oryza sativa L.) by modulating phytohormones, phytochelatin, cadmium transport genes and iron plaque formation. Environ Pollut. 2023;320:121063. doi:10.1016/j.envpol.2023.121063. [Google Scholar] [PubMed] [CrossRef]
44. Rana RA, Siddiqui N, Skalicky M, Brestic M, Hossain A, Kayesh E, et al. Prospects of nanotechnology in improving the productivity and quality of horticultural crops. Horticulturae. 2021;7:332. doi:10.3390/horticulturae7100332. [Google Scholar] [CrossRef]
45. Tan J, Zhao S, Chen J, Pan X, Li C, Liu Y, et al. Preparation of nitrogen-doped carbon dots and their enhancement on lettuce yield and quality. J Mater Chem B. 2023;11(14):3113–23. doi:10.1039/d2tb02817d. [Google Scholar] [PubMed] [CrossRef]
46. Maholiya A, Ranjan P, Khan R, Murali S, Nainwal R, Chauhan P, et al. An insight into the role of carbon dots in the agriculture system: a review. Environ Sci: Nano. 2023;10(4):959–95. doi:10.1039/d2en00954d. [Google Scholar] [CrossRef]
47. Jing X, Liu Y, Liu X, Zhang Y, Wang G, Yang F, et al. Enhanced photosynthetic efficiency by nitrogen-doped carbon dots via plastoquinone-involved electron transfer in apple. Hortic Res. 2024;11(3):11–3. doi:10.1093/hr/uhae016. [Google Scholar] [PubMed] [CrossRef]
48. Li Y, Xu X, Lei B, Zhuang J, Zhang X, Hu C, et al. Magnesium-nitrogen co-doped carbon dots enhance plant growth through multifunctional regulation in photosynthesis. Chem Eng J. 2021;422(1):130114. doi:10.1016/j.cej.2021.130114. [Google Scholar] [CrossRef]
49. Pan X, Nie D, Guo X, Xu S, Zhang D, Cao F, et al. Effective control of the tomato wilt pathogen using TiO2 nanoparticles as a green nanopesticide. Environ Sci: Nano. 2023;10(5):1441–52. doi:10.1039/d3en00059a. [Google Scholar] [CrossRef]
50. Zia-ur-Rehman M, Mfarrej MFB, Usman M, Anayatullah S, Rizwan M, Alharby H, et al. Effect of iron nanoparticles and conventional sources of Fe on growth, physiology and nutrient accumulation in wheat plants grown on normal and salt-affected soils. J Hazard Mater. 2023;458(3–4):131861. doi:10.1016/j.jhazmat.2023.131861. [Google Scholar] [PubMed] [CrossRef]
51. Xin X, Nepal J, Wright AL, Yang X, He Z. Carbon nanoparticles improve corn (Zea mays L.) growth and soil quality: comparison of foliar spray and soil drench application. J Clean Prod. 2022;363(1):132630. doi:10.1016/j.jclepro.2022.132630. [Google Scholar] [CrossRef]
52. Guo J, Li S, Brestic M, Li N, Zhang P, Liu L, et al. Modulations in protein phosphorylation explain the physiological responses of barley (Hordeum vulgare) to nanoplastics and ZnO nanoparticles. J Hazard Mater. 2023;443(1):130196. doi:10.1016/j.jhazmat.2022.130196. [Google Scholar] [PubMed] [CrossRef]
53. Wei Z, Zhang H, Fang M, Lin S, Zhu M, Li Y, et al. The Dof transcription factor COG1 acts as a key regulator of plant biomass by promoting photosynthesis and starch accumulation. Mol Plant. 2023;16(11):1759–72. doi:10.1016/j.molp.2023.09.011. [Google Scholar] [PubMed] [CrossRef]
54. Al-Amri N, Tombuloglu H, Slimani Y, Akhtar S, Barghouthi M, Almessiere M, et al. Size effect of iron (III) oxide nanomaterials on the growth, and their uptake and translocation in common wheat (Triticum aestivum L.). Ecotoxicol Environ Saf. 2020;194(8):110377. doi:10.1016/j.ecoenv.2020.110377. [Google Scholar] [PubMed] [CrossRef]
55. Van Nhan L, Ma C, Rui Y, Cao W, Deng Y, Liu L, et al. The effects of Fe2O3 nanoparticles on physiology and insecticide activity in non-transgenic and Bt-transgenic cotton. Front Plant Sci. 2016;6(13):1263. doi:10.3389/fpls.2015.01263. [Google Scholar] [PubMed] [CrossRef]
56. Ye Y, Cota-Ruiz K, Hernández-Viezcas JA, Valdés V, Medina-Velo I, Turley R, et al. Manganese nanoparticles control salinity-modulated molecular responses in Capsicum annuum L. through priming: a sustainable approach for agriculture. ACS Sustain Chem Eng. 2020;8(3):1427–36. doi:10.1021/acssuschemeng.9b05615. [Google Scholar] [CrossRef]
57. Li X, Ghanizadeh H, Han Z, Wang Q, Li F, Qiu Y, et al. Metabolic profile and physiological mechanisms underlying the promoting effects of TiO2NPs on the photosynthesis and growth of tomato. Sci Hortic. 2023;322:112394. doi:10.1016/j.scienta.2023.112394. [Google Scholar] [CrossRef]
58. Zhang M. Plasma membrane H+-ATPase overexpression increases rice yield via simultaneous enhancement of nutrient uptake and photosynthesis. Nat Nanotechnol. 2023;18(1):688–91. doi:10.1038/s41467-021-20964-4. [Google Scholar] [PubMed] [CrossRef]
59. Li M. Nano-enabled strategies to enhance biological nitrogen fixation. Nat Nanotechnol. 2023;18(7):688–91. doi:10.1038/s41565-023-01392-5. [Google Scholar] [PubMed] [CrossRef]
60. Zhou H, Huang J, Willems P, Van Breusegem F, Xie Y. Cysteine thiol-based post-translational modification: what do we know about transcription factors? Trends Plant Sci. 2023;28(4):415–28. doi:10.1016/j.tplants.2022.11.007. [Google Scholar] [PubMed] [CrossRef]
61. Berrios L, Rentsch JD. Linking Reactive Oxygen Species (ROS) to abiotic and biotic feedbacks in plant microbiomes: the dose makes the poison. Int J Mol Sci. 2022;23(8):4402. doi:10.3390/ijms23084402. [Google Scholar] [PubMed] [CrossRef]
62. Castro B, Citterico M, Kimura S, Stevens D, Wrzaczek M, Coaker G, et al. Stress-induced reactive oxygen species compartmentalization, perception and signalling. Nat Plants. 2021;7(4):403–12. doi:10.1038/s41477-021-00887-0. [Google Scholar] [PubMed] [CrossRef]
63. Zhang L, Wang L, Fang Y, Gao Y, Yang S, Su J, et al. Phosphorylated transcription factor PuHB40 mediates ROS-dependent anthocyanin biosynthesis in pear exposed to high light. Plant Cell. 2024;36(9):3562–83. doi:10.1093/plcell/koae167. [Google Scholar] [PubMed] [CrossRef]
64. Zhang Y, Fu L, Jeon SJ, Yan J, Giraldo JP, Matyjaszewski K, et al. Star polymers with designed reactive oxygen species scavenging and agent delivery functionality promote plant stress tolerance. ACS Nano. 2022;16(3):4467–78. doi:10.1021/acsnano.1c10828. [Google Scholar] [PubMed] [CrossRef]
65. Zhang P, Jiang Y, Schwab F, Monikh FA, Grillo R, White J, et al. Strategies for enhancing plant immunity and resilience using nanomaterials for sustainable agriculture. Environ Sci Technol. 2024;58(21):9051–60. doi:10.1021/acs.est.4c03522. [Google Scholar] [PubMed] [CrossRef]
66. Korsvik C, Patil S, Seal S, Self WT. Superoxide dismutase mimetic properties exhibited by vacancy engineered ceria nanoparticles. Chem Commun. 2007;303(10):1056. doi:10.1039/b615134e. [Google Scholar] [PubMed] [CrossRef]
67. Wu H, Tito N, Giraldo JP. Anionic cerium oxide nanoparticles protect plant photosynthesis from abiotic stress by scavenging reactive oxygen species. ACS Nano. 2017;11(11):11283–97. doi:10.1021/acsnano.7b05723. [Google Scholar] [PubMed] [CrossRef]
68. Zhao L, Bai T, Wei H, Gardea-Torresdey J, Keller A, White J, et al. Nanobiotechnology-based strategies for enhanced crop stress resilience. Nat Food. 2022;3(10):829–36. doi:10.1038/s43016-022-00596-7. [Google Scholar] [PubMed] [CrossRef]
69. Waqas Mazhar M, Ishtiaq M, Hussain I, Parveen A, Hayat Bhatti K, Azeem M, et al. Seed nano-priming with Zinc Oxide nanoparticles in rice mitigates drought and enhances agronomic profile. PLoS One. 2022;17(3):e0264967. doi:10.1371/journal.pone.0264967. [Google Scholar] [PubMed] [CrossRef]
70. Sun XD, Ma JY, Feng LJ, Duan J, Xie XM, Zhang XH, et al. Magnetite nanoparticle coating chemistry regulates root uptake pathways and iron chlorosis in plants. Proc Natl Acad Sci U S A. 2023;120(27):e2304306120. doi:10.1073/pnas.2304306120. [Google Scholar] [PubMed] [CrossRef]
71. Guo S, Hu X, Yu F, Mu L. Heat waves coupled with nanoparticles induce yield and nutritional losses in rice by regulating stomatal closure. ACS Nano. 2024;18(22):14276–89. doi:10.1021/acsnano.3c13165. [Google Scholar] [PubMed] [CrossRef]
72. Nair PMG, Chung IM. Regulation of morphological, molecular and nutrient status in Arabidopsis thaliana seedlings in response to ZnO nanoparticles and Zn ion exposure. Sci Total Environ. 2017;575(514):187–98. doi:10.1016/j.scitotenv.2016.10.017. [Google Scholar] [PubMed] [CrossRef]
73. Cromwell WA, Yang J, Starr JL, Jo Y-K. Nematicidal effects of silver nanoparticles on root-knot nematode in bermudagrass. J Nematol. 2014;46(3):261–6. [Google Scholar] [PubMed]
74. Tian X, Liu Y, Huang Z, Duan H, Tong J, He X, et al. Comparative proteomic analysis of seedling leaves of cold-tolerant and -sensitive spring soybean cultivars. Mol Biol Rep. 2015;42(3):581–601. doi:10.1007/s11033-014-3803-4. [Google Scholar] [PubMed] [CrossRef]
75. Hanif S, Farooq S, Kiani MZ, Zia M. Surface modified ZnO NPs by betaine and proline build up tomato plants against drought stress and increase fruit nutritional quality. Chemosphere. 2024;362(5):142671. doi:10.1016/j.chemosphere.2024.142671. [Google Scholar] [PubMed] [CrossRef]
76. Zhao W, Wu Z, Amde M, Zhu G, Wei Y, Zhou P, et al. Nanoenabled enhancement of plant tolerance to heat and drought stress on molecular response. J Agric Food Chem. 2023;71(51):20405–18. doi:10.1021/acs.jafc.3c04838. [Google Scholar] [PubMed] [CrossRef]
77. Liang YP, Liu H, Fu YY, Li PH, Li S, Gao Y, et al. Regulatory effects of silicon nanoparticles on the growth and photosynthesis of cotton seedlings under salt and low-temperature dual stress. BMC Plant Biol. 2023;23(1):504. doi:10.1186/s12870-023-04509-z. [Google Scholar] [PubMed] [CrossRef]
78. Zhou P, Adeel M, Shakoor N, Guo M, Hao Y, Azeem I, et al. Application of nanoparticles alleviates heavy metals stress and promotes plant growth: an overview. Nanomaterials. 2021;11(1):26. doi:10.3390/nano11010026. [Google Scholar] [PubMed] [CrossRef]
79. Naidu S, Pandey J, Mishra LC, Chakraborty A, Roy A, Singh I, et al. Silicon nanoparticles: synthesis, uptake and their role in mitigation of biotic stress. Ecotoxicol Environ Saf. 2023;255(1):114783. doi:10.1016/j.ecoenv.2023.114783. [Google Scholar] [PubMed] [CrossRef]
80. Shen X, Yang Z, Dai X, Feng W, Li P, Chen Y, et al. Calcium hexacyanoferrate nanozyme enhances plant stress resistance by oxidative stress alleviation and heavy metal removal. Adv Mater Deerfield. 2024;36(30):2402745. doi:10.1002/adma.202402745. [Google Scholar] [PubMed] [CrossRef]
81. Jiao C, Dong C, Xie C, Luo W, Zhang J, Fan S, et al. Dissolution and retention process of CeO2 nanoparticles in soil with dynamic redox conditions. Environ Sci Technol. 2021;55(21):14649–57. doi:10.1021/acs.est.1c04660. [Google Scholar] [PubMed] [CrossRef]
82. Yuan L, Richardson CJ, Ho M, Willis CW, Colman BP, Wiesner MR. Stress responses of aquatic plants to silver nanoparticles. Environ Sci Technol. 2018;52(5):2558–65. doi:10.1021/acs.est.7b05837. [Google Scholar] [PubMed] [CrossRef]
83. Yusefi-Tanha E, Fallah S, Rostamnejadi A, Pokhrel L. Particle size and concentration dependent toxicity of copper oxide nanoparticles (CuONPs) on seed yield and antioxidant defense system in soil grown soybean (Glycine max cv. Kowsar). Sci Total Environ. 2020;715(7):136994. doi:10.1016/j.scitotenv.2020.136994. [Google Scholar] [PubMed] [CrossRef]
84. López-Moreno ML, De La Rosa G, Hernández-Viezcas JÁ, Castillo-Michel H, Botez C, Peralta-Videa JR, et al. Evidence of the differential biotransformation and genotoxicity of ZnO and CeO2 nanoparticles on soybean (Glycine max) plants. Environ Sci Technol. 2010;44(19):7315–20. doi:10.1021/es903891g. [Google Scholar] [PubMed] [CrossRef]
85. Ding Y, Zhao W, Zhu G, Wang Q, Zhang P, Rui Y. Recent trends in foliar nanofertilizers: a review. Nanomaterials. 2023;13(21):2906. doi:10.3390/nano13212906. [Google Scholar] [PubMed] [CrossRef]
Cite This Article
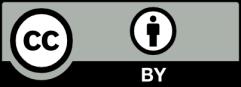
This work is licensed under a Creative Commons Attribution 4.0 International License , which permits unrestricted use, distribution, and reproduction in any medium, provided the original work is properly cited.