Open Access
ARTICLE
Elicitation of PVY Resistance by Coniothyrium aleuritis
1 Agricultural Botany Department, Faculty of Agriculture, Kafrelsheikh University, Kafr Elsheikh, 33516, Egypt
2 Department of Biology, College of Science, Jazan University, Jazan, 45142, Saudi Arabia
3 Department of Chemistry, College of Sciences and Arts, King Abdulaziz University, Rabigh, 21911, Saudi Arabia
* Corresponding Author: Mohsen Mohamed Elsharkawy. Email:
(This article belongs to the Special Issue: Microbe-Mediated Regulation of Plant Growth and Stress Biology)
Phyton-International Journal of Experimental Botany 2024, 93(12), 3373-3385. https://doi.org/10.32604/phyton.2024.058875
Received 23 September 2024; Accepted 21 November 2024; Issue published 31 December 2024
Abstract
Endophytes associated with plants are recognized as bio-reservoirs of natural products and denote a significant symbiotic interaction in nature. Endophytes penetrate the plant’s interior tissues without showing any indications of disease or obvious alterations. In this study, the potential of a novel and new isolated plant growth-stimulating fungus, Coniothyrium aleuritis, was evaluated against PVY (the pathogen potato virus Y) on potato plants. Many parameters, including disease severity, PVY titer, enzymatic profiling, defense-related biochemical marker (carotenoid), phenolic compounds, proline content, as well as growth and yield parameters, have been investigated to clarify the role of C. aleuritis isolate in mitigating PVY-induced damage due to virus infection. Potato and tobacco plants treated with C. aleuritis grew faster, showed fewer symptoms of disease, and had lower levels of PVY accumulation than plants grown without the treatment. Antioxidant enzymes polyphenol oxidase, catalase, and superoxide dismutase were increased in treated potatoes. A notable upsurge in the transcription levels of defensive genes (PR1b, and PAL1 in potato and PR1 and Coi1 in tobacco), phenolic compounds, carotenoid, and proline contents was observed in treated plants after inoculation. All the experimental and analytical data show that C. aleuritis is effective in supporting potato yield and preventing PVY infection. These findings suggest that C. aleuritis is a promising and eco-friendly treatment for controlling PVY infections.Graphic Abstract

Keywords
Solanum tuberosum (potato) is a common and cost-effective food source. A great variety of applications have been launched to enhance agricultural production. Microorganisms such as oomycetes, bacteria, viruses, nematodes, parasitic plants, and fungi may cause severe harm to plants via disease infections [1]. Plant viral infections seriously threaten plant biosecurity and are the primary cause of significant global food shortages [2]. Potato viruses also frequently have a negative economic impact because they lower seed quality and limit trade [3]. Potato virus Y, or PVY, is an epidemic viral disease of potato spread by aphids locally. Production of certified seeds and plants grown for fresh or processed markets may be impacted [4]. About 30 distinct plant families are impacted by PVY [5]. Recombinant strains of PVY are the most prevalent and important, and their pathogenicity can vary from moderate to severe based on the strain [6]. The most obvious symptoms of PVY are leaf necrosis, yellowing, and possibly even complete leaf death [7].
The interior tissues of all plant species are hosts to plant-associated microbes, which are becoming more and more important bio-prospecting targets in the search for new natural growth promoters [8]. Regarding induced systemic resistance against plant diseases, endophytic fungi are among the most promising possibilities. Even though plant-associated endophytes can function as inducers of systemic resistance, there are still issues due to our incomplete understanding of endophyte biology and the mechanism of disease resistance. Endophyte asymptomatic colonization is mediated by metabolite synthesis, which uses multipartite symbiosis to undermine host resistance [9]. The development of defense mechanisms in plant hosts, such as the generation of secondary metabolites toward infections, is a crucial host mechanism. Research priorities should focus on endophytic fungus and their metabolic processes.
Conventional methods of reducing the spread of infectious diseases include controlling vectors with insecticides, using natural predators, and creating physical barriers such as reflecting mulches [10]. Plant diseases have been successfully managed by the removal of weeds, the use of virus-free materials, early seeding, the establishment of rest periods for crops, and the elimination of contaminated harvests [11]. A realistic and long-term approach to agricultural sustainability is the development of disease-resistant crop cultivars [12]. However, cultivars with the appropriate range of resistance could require an extensive expenditure of time and resources to produce [13]. Creating more virus-resistant cultivars can help decrease the degree of agricultural damage that viruses cause [14]. A growing number of people are interested in biocontrol agents as environmentally friendly substitutes for the harmful pesticides now used in plant pest management techniques to preserve sustainable practices in agriculture and the environment [15,16]. Beneficial microorganisms known as “plant growth-promoting microorganisms,” or PGPMs, reside naturally in the rhizosphere and promote plant development while boosting resistance to various diseases [16]. Several investigations have demonstrated the direct impact of endophytes on plant development and viral defenses [16,17]. Endophytes promote plant development by increasing nutrient absorption and producing biomolecules essential for stress tolerance. These acts can either directly target pathogens by producing antibiotics or by out-competing them for vital nutrients, or they can lessen the plant’s susceptibility to infections indirectly by upregulating the plant’s immune system [18].
There are two main mechanisms by which plants might generate induced resistance. The first, known as SAR (systemic-acquired resistance), is caused by pathogens or elicitor chemicals coming in touch with plant leaves. Salicylic acid is essential in controlling this reaction [19]. Induced-systemic resistance, or ISR, is the second type of resistance that is triggered when endophytes and other beneficial microbes come into contact with plant roots [16]. Plants need each of these mechanisms to remain resistant to viruses [16,19]. The activation of SAR and ISR causes several physiological responses that partially overlap, including the production of antioxidant enzymes and defense genes [18]. The purpose of this research was to ascertain whether Coniothyrium aleuritis could be utilized to promote plant development and protection against PVY infection.
2.1 Isolation and Identification of Endophytic Fungus
Samples of roots were taken from the rhizosphere of robust potato plants. The gathered root samples were passed through а rigorous cleaning procedure. They were first washed with running tap water to get rid of debris, followed by washing three times with a sterile solution of distilled water. The root segments (less than 2 cm) were treated with 70% ethanol for 45 s to guarantee surface sterilization. After that, they were washed three times with a sterile solution of distilled water, dried on sterilized filter paper and inoculated on PDA. The plates were then exposed to incubation in the dark (25°C). Following incubation for seven days, fungal growth was noted, and separate colonies with various morphologies were chosen with the use of a sterile loop. After being carefully handled to avoid overgrowth, the colonies were grown on new PDA plates to produce pure cultures [16]. Phenotypic analysis of the isolate was done in the Plant Pathology Lab., Kafrelsheikh University.
An in-house fungal DNA isolation kit was used to extract genomic DNA from a 12-day-old culture, and a Denovix DS-11 spectrophotometer was used to assess the genomic DNA’s concentration and purity [20]. The procedures for polymerase chain reaction (PCR) and sequencing were followed [21]. Using BioEdit, the raw sequences used in this work were manually adapted and proofread. ClustalX was used to align the adjusted sequences [22]. Consensus sequences were established from the obtained sequences and added to Genbank after alignment. Molecular phylogenetic analysis was done using ver. 11.0.2 of MEGA-11 software [22]. Neighbor-joining analysis and information from whole nucleotide sequences were used to create a phylogenetic tree [22].
Barley kernel inoculum (BKI) from the endophytic fungus isolate (laboratory code ME23) was prepared as previously described by Elsharkawy et al. [16]. Colonized barley kernels (2 weeks after inoculation) were air dried and ground to 2 mm size then stored at 4°C until use. The Spunta potato cultivar, which has been verified free of viral infection using ELISA test, was gathered from the Agricultural Research Center’s brown rot research project (ARC, http://www.arc.sci.eg/default.aspx?lang=en) (accessed on 20 November 2024). The potato tubers were placed in 30 cm-wide pots that were filled with a specially made soil combination (1:2 w/w, sand: clay ratio, mixed with barley kernels colonized with the endophytic fungus at a final concentration 0.7% w/w). The potato virus Y subtype NTN and tobacco (Nicotiana tabacum) seeds were obtained from Plant Pathology Lab., Kafrelsheikh University, and the seeds were planted in 15-cm pots [23]. Smashed and extracted (in 0.1 M phosphate buffer solution, pH 7) tobacco leaves were used to produce viral inoculum for the following infection trials. The initial sap extract that had been purified was first filtered through cheesecloth. Potato leaves in both the main and tertiary stages were infected with a 10−1 dilution of the infectious tobacco sap. In a greenhouse at 24°C with a 16-h photoperiod, inoculated tobacco and potato plants for PVY trials were maintained under insect-proof conditions.
2.3 Assessment of the Disease Severity Rating and AUDPC
Periodically, the foliage of infected potato plants was visually inspected to measure the evolution of the symptoms of the PVY disease over time. A consistent 0–4 grading system was used to grade the infection severity of each PVY-inoculated plant separately. The described symptom difference ranged from 0 = absence of obvious symptoms, 1 = moderate mottling of leaf mosaic (<20% infection area), 2 = noticeable mottling (20%–50% infected area), 3 = severe malformation, stunting, and mottling (>50% infected area), to the maximum score of 4 = severe mosaic, deformity, necrosis, and death of the plant. The following formula was used to determine the disease severity (DS) values at 7, 14, and 21 days post-viral infection (DPVI) [23]. The computation of the AUDPC (area under the disease progress curve) was done [24].
2.4 Evaluation of Total Phenols, Proline, Carotenoid and Defense Enzymes
The analysis of proline content [25], carotenoids [26], and phenolic compounds were conducted [27,28]. For enzyme estimation in this experiment, the apical buds of the treated potato plants were the source. An enzyme estimation was performed using the clear supernatant obtained by centrifuging the sample (2 g) for 20 min at 2000 rpm and 2°C after it had been homogenized with 10 mL of phosphate buffer at pH 6.8. A UV spectrophotometer (Spectronic 20D, Thermo Electron) was used to detect the activity of the PPO (poly phenoloxidase), SOD (superoxide dismutase) and CAT (catalase) enzymes [23].
2.5 Colonization of Potato and Tobacco Roots with Coniothyrium aleuritis
Seven weeks following the application treatment, the colonization of C. aleuritis in tobacco and potato roots was assessed. Ten randomly chosen plants’ roots were removed, and after being rinsed three times with sterile distilled water to get rid of any remaining soil, the roots were blotted dry and plated on PDA [16]. Colonies were enumerated from 100 root segments based on the isolates’ basic morphology.
Twelve weeks after planting, the treated potato plants’ yield characteristics were evaluated by harvesting. Each treatment group’s parameters, including the weight and number of tubers as well as the fresh and dry weights, were measured.
2.7 Transcription of Defense Genes in Potato and Tobacco
Leaves of potato and tobacco (treated and non-treated) plants were collected at 0, 2, 4 DAVI (days after virus inoculation) for tobacco and at 2 and 4 DAVI for potato. The methods of Elsharkawy et al. [23] and Livak and Schmittgen [29] were followed to carry out quantitative RT-PCR analysis for potato and RT-PCR for tobacco. The specific primers for tobacco and potato are listed in Tables 1 and 2 [30–33].
Four separate duplicates of each experiment were conducted. Post-hoc analysis of ANOVA results was applied. The means for each group of duplicates were used to illustrate the results. Using the Excel Tokei statistical software, the Least Significant Difference (LSD) at p < 0.05 was used to evaluate the statistical significance of differences between means.
3.1 Endophytic Fungi Identification
PCR amplicons were utilized to identify the isolate. The studied strain has been preliminary identified as Coniothyrium aleuritis, but the exact taxonomic name will need to be classified through additional genetic studies. Through investigation, a 98.3% sequence identity match to C. aleuritis was found using BLAST comparisons and ITS gene sequencing. GenBank received the obtained ITS sequence and accepted it under accession OP862862.
3.2 Impact of C. aleuritis on the Severity of PVY Disease
Both tobacco and potato plants treated with C. aleuritis had considerably fewer disease symptoms than the untreated PVY-infected control group (Fig. 1). There was a noticeable and statistically significant decrease in PVY disease severity. The administration of C. aleuritis before PVY infection resulted in a significant decrease in disease severity. The relevant disease indices are presented in Fig. 2, which highlights the efficiency of C. aleuritis in reducing the severity of PVY infection in both potato and tobacco plants, confirming its potential as a protective strategy against the disease.
Figure 1: Photographs of tobacco plants treated with Coniothyrium aleuritis and non-treated control at 2 weeks after PVY inoculation
Figure 2: Effects of Coniothyrium aleuritis treatments on PVYNTN AUDPC (area under the disease progress curve) in tobacco and potato. Within potato or tobacco, different lowercase letters above the histograms indicate significant differences at p < 0.05
3.3 Impact of C. aleuritis on the PVY Titer
The ELISA findings showed that C. aleuritis plants had considerably less viral accumulation, whereas the non-treated group had the highest amount of viral accumulation (Fig. 3). Potato plants that were not treated had the highest degree of viral accumulation (0.43), whereas the C. aleuritis plants displayed much lower levels of viral accumulation (0.23). Interestingly, PVY concentration was significantly affected by applying C. aleuritis, falling from 0.49 to 0.18 in tobacco (Fig. 3). These findings highlight the multitude of C. aleuritis to mitigate the virus’s effects.
Figure 3: The impact of Coniothyrium aleuritis on the levels of PVYNTN in tobacco and potato plant leaves using DAS-ELISA (enzyme-linked immunosorbent assay) three weeks after virus inoculation. The values denoted by the different letters differ significantly. Within potato or tobacco, different lowercase letters above the histograms indicate significant differences at p < 0.05
3.4 Assessment of the Impact of Treatment with C. aleuritis on Potato Growth and Yield Parameters
Treatments with C. aleuritis produced substantial impacts on fresh and dry weights of tobacco plants (Table 3). The comparison in tobacco plants demonstrated that the C. aleuritis-PVY treatment resulted in the maximum dry and fresh weights (1.1 and 10.1 g/plant, respectively). Table 4 shows the influence of C. aleuritis on major growth and yield measurements of potato. Based on the findings of the data analysis, it is apparent that all indicators have significantly improved. The C. aleuritis treatment produced the greatest fresh weight for PVY-infected potato (8.8 g/plant). C. aleuritis had a greater dry weight (0.91 g/plant) compared to PVY-infected group (0.42 g/plant). C. aleuritis-PVY infection treatment resulted in a modestly decreased fresh weight (8.8 g/plant) compared with the healthy group (10.8 g/plant). The C. aleuritis-PVY infection treatment had the greatest tuber weight (158 g/plant) among infected treatments. Similarly, the C. aleuritis-PVY infection treatment demonstrated the greatest tuber number (5.8) among infected treatments. Overall, the C. aleuritis-PVY treatment regularly displayed favorable impacts on growth indices, highlighting its ability to improve plant performance.
3.5 Assessment of Enzymes and Biochemical Markers Associated with Defense Mechanisms
The treatment of potato plants with C. aleuritis before viral inoculation improves their PPO, CAT, and SOD activities (Table 5). Results indicate that C. aleuritis treatments increased accumulated PPO, CAT, and SOD by 0.14, 0.42 and 0.43 mol/gFW relative to the control group (0.06, 0.12, and 0.14 mol/gFW). Total phenols and proline contents in potato plants treated with C. aleuritis were 1.3 and 0.83 mg/g FW, respectively, compared with 0.98 and 0.64 mg/g FW in the control group. C. aleuritis-PVY infection treatment showed a greater amount of carotenoid (0.94 mg/g FW) than the infected control (0.63 mg/g FW) (Fig. 4).
Figure 4: Impact of Coniothyrium aleuritis on the total phenols and proline contents and carotenoids in potato plants. Different lowercase letters above the histograms on each panel indicate significant differences at p < 0.05
3.6 Re-isolation Frequency of C. aleuritis
According to our findings, 7 weeks after planting, potato and tobacco plants produced significant frequencies of C. aleuritis recording 92% and 95% for potato and tobacco plants, respectively (Fig. 5).
Figure 5: Re-isolation frequency of Coniothyrium aleuritis from potato and tobacco roots at 7 weeks after treatment
3.7 Impact of C. aleuritis on Potato PR-1b Gene Expression
PR-1b and PAL1 genes were shown to express differently in C. aleuritis potato plants compared to healthy and infected control (Fig. 6). In comparison to the control, the potato plants treated with C. aleuritis had relative expression levels of PR-1b that were 3 and 5-folds higher at 2 and 4 days after PVY inoculation (DAVI), respectively. PAL1 showed upregulation of expression in all treatments, especially treatment with C. aleuritis (2.1-fold) and (3.5-fold) at 2 and 4 (DAVI) compared to the control expression levels (Fig. 6).
Figure 6: Expression of the PR1-b and PAL1 genes in potato plants following the application of Coniothyrium aleuritis. Within PR1-b or PAL1, different lowercase letters above the histograms at each time (days) indicate significant differences at p < 0.05
3.8 Impact of C. aleuritis on the Expression of Tobacco Genes
Transcription levels of PR1 and Coi1 genes associated with pathogenesis in tobacco are shown in Fig. 7. In C. aleuritis, the transcription of the PR1 gene in tobacco plants started to be induced one day after the induction treatments and continued at high levels for four days after the inoculation. Coi1 transcription initially appeared in the C. aleuritis treatment at 1 day after infection and remained elevated 4 days later.
Figure 7: Expression of the defense genes (PR1 and Coi1) in tobacco plants following the application of Coniothyrium aleuritis and PVY infection
Plant viruses are important causes of plant diseases since more than half of all new epidemics have a viral cause [3,16]. One of the most significant viruses that seriously reduces the value of potato grown is PVY [34]. In the current investigation, we used Coniothyrium aleuritis, a biotic viricide, in order to attempt elicitation of ISR in potato and tobacco plants against PVY infection. The current study’s findings provide insight into the complicated effects of C. aleuritis on PVY-infected potato plants. It is essential to comprehend the complex relationships that exist between the PVY, the host plants, and C. aleuritis to clarify possible uses in controlling viral infections and boosting plant resistance. We explore the implications of the results regarding the literature that has already been published in this subject, highlighting the importance of C. aleuritis-mediated biocontrol and its possible incorporation into sustainable agriculture methods.
In the process of developing mutualistic relationships, fungal endophytes and plants may provide each other with a wide variety of benefits [16]. Simultaneously, many kinds of endophytic microorganisms might facilitate plant absorption and use of soil nutrients by increasing their biosimulation and availability of various substances. These nutrients are correlated with enhanced plant development and growth, and consequently, higher plant yields [35]. Additionally, various kinds of endophytic fungi could improve plants’ defences against pest and disease invasions [16,17]. Plant growth and systemic resistance responses to biotic stressors can both be induced by the application of fungal endophytes [16]. Endophytic fungi can create metabolites and signals that are important in these beneficial relationships, and their interactions with plants can also have a significant impact on the sustainability and quality of whole agroecosystems [36].
Plants that are exposed to specific helpful microbes have a phenomenon known as ISR, which causes plants to boost their defensive systems and become more resistant to future pathogen attacks. It is known that certain fungal endophytic species cause ISR in plants, preparing them for improved defense against a variety of diseases, including viruses [16]. Applying C. aleuritis proactively before PVY infection during the plant development stage results in a significant reduction in disease symptoms and viral infection, which is consistent with the idea of ISR. Its effectiveness as a preventative and response intervention against PVY is suggested by the constant reduction in disease severity in pre-PVY infection treatments with C. aleuritis. This dual functioning highlights C. aleuritis adaptability and is especially significant for real applications in controlling PVY disease at different growth stages of potato. C. aleuritis potential for biocontrol is further supported by the quantitative measurement of PVY concentration using DAS-ELISA. The capacity of the C. aleuritis to restrict viral growth within the plant is demonstrated by the significant decrease in PVY concentration in protected treatments. These results highlight the efficacy of C. aleuritis in reducing PVY concentration, demonstrating its potential as a beneficial intervention in controlling PVY-infected potato cultivars. This is consistent with research showing endophytic fungi antiviral properties [16]. Understanding the exact processes underlying its antiviral activity might help design focused and optimal biocontrol techniques.
Understanding the physiological reactions of potato plants to PVY infection and C. aleuritis treatment is possible by evaluating the effects of C. aleuritis on defense-associated biochemical indicators. Carotenoid showed a significant recovery with application of the C. aleuritis leading to a possible reduction in virus-induced damage. Enzymatic antioxidant activities also showed similar patterns, with C. aleuritis treatment improving the activities of PPO, CAT and SOD.
The elevation of proline, and total phenols found after treatment with C. aleuritis highlights the activation of defense-related biochemical pathways. It is well recognized that these substances are essential for plants to defend themselves against a variety of threats, such as viral infections. The concentration of these biochemical components that C. aleuritis induces is consistent with the idea that C. aleuritis-mediated biocontrol entails triggering plant systemic defensive responses, which enhances plant resistance to infections. Similarly, defense genes transcriptions in tobacco and potato were elevated in pre-PVY infection treatment with C. aleuritis in comparison with PVY infected control.
Increased fresh weight, tuber weight, and tuber number show that C. aleuritis has a favorable influence on potato plant development and yield indices. These findings are consistent with research showing the growth-promoting properties of beneficial soil fungi [16].
Plant disease losses, particularly those caused by viral infections, are a serious global issue that affects food security. The results of this investigation support C. aleuritis potential as an ISR-inducing agent against PVY by reducing PVY severity and titer. This aligns with integrated pest management’s (IPM) objectives, which aim to maximize the application of several control techniques to accomplish efficient and long-lasting pest management. The transcription of genes associated with plant defense in the signaling pathways (both JA and SA) was elevated by C. aleuritis. The outcomes also demonstrated C. aleuritis beneficial effects on potato development and growth. This is the first investigation of the effectiveness of C. aleuritis in treating PVY disease.
Acknowledgement: The authors would like to thank Agricultural Botany Department, Faculty of Agriculture, Kafrelsheikh University for the facilities granted during performance of the experiments.
Funding Statement: The authors received no specific funding for this study.
Author Contributions: Conceptualization, Mohsen Mohamed Elsharkawy; methodology, Mohsen Mohamed Elsharkawy; software, Mohsen Mohamed Elsharkawy; validation, Mohsen Mohamed Elsharkawy, Mari Sumayli and Faisal Ay Alzahrani; formal analysis, Mohsen Mohamed Elsharkawy, Mari Sumayli and Faisal Ay Alzahrani; investigation, Mohsen Mohamed Elsharkawy; resources, Mohsen Mohamed Elsharkawy, Mari Sumayli and Faisal Ay Alzahrani; data curation, Mohsen Mohamed Elsharkawy; writing—original draft preparation, Mohsen Mohamed Elsharkawy; writing—review and editing, Mohsen Mohamed Elsharkawy, Mari Sumayli and Faisal Ay Alzahrani; visualization, Mohsen Mohamed Elsharkawy, Mari Sumayli and Faisal Ay Alzahrani; supervision, Mohsen Mohamed Elsharkawy; project administration, Mohsen Mohamed Elsharkawy; funding acquisition, Mohsen Mohamed Elsharkawy, Mari Sumayli and Faisal Ay Alzahrani. All authors reviewed the results and approved the final version of the manuscript.
Availability of Data and Materials: The datasets generated during and/or analyzed during the current study are available from the corresponding authors upon reasonable request.
Ethics Approval: Not applicable.
Conflicts of Interest: The authors declare no conflicts of interest to report regarding the present study.
References
1. Abdelkhalek A, Hafez E. Plant viral diseases in egypt and their control. In: Cottage industry of biocontrol agents and their applications. Berlin/Heidelberg, Germany: Springer; 2020. p. 403–21. [Google Scholar]
2. Mumford RA, Macarthur R, Boonham N. The role and challenges of new diagnostic technology in plant biosecurity. Food Secur. 2016;8:103–9. [Google Scholar]
3. Abdelkhalek A, Al-Askar AA, Behiry SI. Bacillus licheniformis strain POT1 mediated polyphenol biosynthetic pathways genes activation and systemic resistance in potato plants against Alfalfa mosaic virus. Sci Rep. 2020;10:16. [Google Scholar]
4. Rykbost KA, Hane DC, Hamm PB, Voss R, Kirby D. Effects of seedborne potato virus Y on Russet Norkotah performance. Am J Potato Res. 1999;76:91–6. [Google Scholar]
5. Claude M, Fauquet M. Virus taxonomy: eighth report of the International Committee on taxonomy of viruses. Amsterdam, The Netherlands: Elsevier Science & Technology; 2004. [Google Scholar]
6. Visser JC, Bellstedt DU, Pirie MD. The recent recombinant evolution of a major crop pathogen, Potato virus Y. PLoS One. 2012;7:e50631. doi:10.1371/journal.pone.0050631. [Google Scholar] [PubMed] [CrossRef]
7. Eraky MA, Rashed SM, Nasr ME-S, El-Hamshary AMS, Salah El-Ghannam A. Parasitic contamination of commonly consumed fresh leafy vegetables in Benha. Egypt J Parasitol Res. 2014;2014:122. [Google Scholar]
8. Tiwari P, Srivastava Y, Bae H. Endophytes: trend of pharmaceutical design of Endophytes as anti-infective. Curr Top Med Chem. 2021;21:1572–86. [Google Scholar] [PubMed]
9. Schulz B, Haas S, Junker C, Andrée N, Schobert M. Fungal endophytes are involved in multiple balanced antagonisms. Curr Sci. 2015;109:39–45. [Google Scholar]
10. Legg JP, Shirima R, Tajebe LS, Guastella D, Boniface S, Jeremiah S, et al. Biology and management of Bemisia whitefly vectors of cassava virus pandemics in Africa. Pest Manage Sci. 2014;70:1446–53. [Google Scholar] [PubMed]
11. Abd El-Rahim WM, Moawad H, Hashem MM, Gebreil GMM, Zakaria M. Highly efficient fungal pectinase and laccase producers among isolates from flax retting liquor. Biocatal Agric Biotechnol. 2020;25:101570. [Google Scholar]
12. Aktar W, Sengupta D, Chowdhury A. Impact of pesticides use in agriculture: their benefits and hazards. Interdiscip Toxicol. 2009;2:1–12. [Google Scholar] [PubMed]
13. Kang B-C, Yeam I, Jahn MM. Genetics of plant virus resistance. Annu Rev Phytopathol. 2005;43:581–621. [Google Scholar] [PubMed]
14. Ma G, Chen P, Buss GR, Tolin SA. Genetics of resistance to two strains of Soybean mosaic virus in differential soybean genotypes. J Hered. 2004;95:322–6. [Google Scholar] [PubMed]
15. Sharma A, Kumar V, Shahzad B, Tanveer M, Sidhu GPS, Handa N, et al. Worldwide pesticide usage and its impacts on ecosystem. SN Appl Sci. 2019;1:1446. [Google Scholar]
16. Elsharkawy MM, Shimizu M, Takahashi H, Hyakumachi M. Induction of systemic resistance against Cucumber mosaic virus by Penicillium simplicissimum GP17-2 in Arabidopsis and tobacco. Plant Pathol. 2012;61:964–76. [Google Scholar]
17. Elsharkawy MM, Mousa KM. Induction of systemic resistance against Papaya ring spot virus (PRSV) and its vector Myzus persicae by Penicillium simplicissimum GP17-2 and silica (Sio2) nanopowder. Int J Pest Manage. 2015;61:353–8. doi:10.1080/09670874.2015.1070930. [Google Scholar] [CrossRef]
18. Esmail SM, Draz IS, Saleem MH, Mumtaz S, Elsharkawy MM. Penicillium simplicissimum and Trichoderma asperellum counteract the challenge of Puccinia striiformis f. sp. tritici in wheat plants. Egypt J Biol Pest Control. 2022;32:116. doi:10.1186/s41938-022-00614-7. [Google Scholar] [CrossRef]
19. Vlot AC, Sales JH, Lenk M, Bauer K, Brambilla A, Sommer A, et al. Systemic propagation of immunity in plants. New Phytol. 2021;229:1234–50. [Google Scholar] [PubMed]
20. Kharkwal AC, Joshi H, Shandilya C, Dabral S, Kumar N, Varma A. Isolation and characterization of a newly discovered plant growth-promoting endophytic fungal strain from the genus Talaromyces. Sci Rep. 2024 Mar 12;14(1):6022. [Google Scholar]
21. Fernandes EKK, Keyser CA, Rangel DEN, Foster RN, Roberts DW. CTC medium: a novel dodine-free selective medium for isolating entomopathogenic fungi, especially Metarhizium acridum, from soil. Biol Control. 2010;54:197–205. [Google Scholar]
22. Tamura K, Peterson D, Peterson N, Stecher G, Nei M, Kumar S. MEGA5: molecular evolutionary genetics analysis using maximum likelihood, evolutionary distance, and maximum parsimony methods. Mol Biol Evol. 2011;28:2731–9. [Google Scholar] [PubMed]
23. Elsharkawy MM, Alotibi FO, Al-Askar AA, Adnan M, Kamran M, Abdelkhalek A, et al. Systemic resistance induction of potato and tobacco plants against Potato Virus Y by Klebsiella oxytoca. Life. 2022;12(10):1521. doi:10.3390/life12101521. [Google Scholar] [PubMed] [CrossRef]
24. Nasr-Eldin M, Messiha N, Othman B, Megahed A, Elhalag K. Induction of potato systemic resistance against the potato virus Y (PVYNTNusing crude filtrates of Streptomyces spp. under greenhouse conditions. Egypt J Biol Pest Control. 2019;29:62. [Google Scholar]
25. Bates L, Waldren R, Teare I. Rapid determination of free proline for water-stress studies. Plant Soil. 1973;39(1):205–7. [Google Scholar]
26. Mibei EK, Ambuko J, Giovannoni JJ, Onyango AN, Owino WO. Carotenoid profiling of the leaves of selected African eggplant accessions subjected to drought stress. Food Sci Nutr. 2016;18:113–22. [Google Scholar]
27. Dai J, Mumper RJ. Plant phenolics: extraction, analysis and their antioxidant and anticancer properties. Molecules. 2010;15(10):7313–52. [Google Scholar] [PubMed]
28. Reda F, Abdelhamid MT, El-Lethy SR. The role of Zn and B for improving Vicia faba L. tolerance to salinity stress. Middle East J. 2014;3(4):707–14. [Google Scholar]
29. Livak KJ, Schmittgen TD. Analysis of relative gene expression data using real-time quantitative PCR and the 2−ΔΔCT method. Methods. 2001;25:402–8. doi:10.1006/meth.2001.1262. [Google Scholar] [PubMed] [CrossRef]
30. Kuchitsu K, Ohashi Y. Pathogen-induced calmodulin isoforms in basal resistance against bacterial and fungal pathogens in tobacco. Plant Cell Physiol. 2007;48:414–23. doi:10.1093/pcp/pcm011. [Google Scholar] [PubMed] [CrossRef]
31. Wilmer C, Gaudin A, Solórzano D, Casas A, Luis N, Chudalayandi P, et al. Self-excision of the antibiotic resistance gene nptII using a heat inducible Cre-loxP system from transgenic potato. Plant Mol Biol. 2006;62:71–82. doi:10.1007/s11103-006-9004-3. [Google Scholar] [PubMed] [CrossRef]
32. Baebler Š, Krečič-Stres H, Rotter A, Kogovšek P, Cankar K, Kok EJ, et al. PVYNTN elicits a diverse gene expression response in different potato genotypes in the first 12 h after inoculation. Mol Plant Pathol. 2009;10:263–75. doi:10.1111/j.1364-3703.2008.00530.x. [Google Scholar] [PubMed] [CrossRef]
33. Wang S, Wu H, Qiao J, Ma L, Liu J, Xia F, et al. Molecular mechanism of plant growth promotion and induced systemic resistance to Tobacco mosaic virus by Bacillus spp. J Microbiol Biotechnol. 2009;19:1250–8. doi:10.4014/jmb.0901.008. [Google Scholar] [CrossRef]
34. Chen H, Han Y, Liu Y, Liu D, Jiang L, Huang K, et al. Classification models for Tobacco Mosaic Virus and Potato Virus Y using hyperspectral and machine learning techniques. Front Plant Sci. 2023;16(14):1211617. doi:10.3389/fpls.2023.1211617. [Google Scholar] [PubMed] [CrossRef]
35. Kawaguchi M, Minamisawa K. Plant-microbe communications for symbiosis. Plant Cell Physiol. 2010;51:1377–80. [Google Scholar] [PubMed]
36. Pérez LI, Gundel PE, Zabalgogeazcoa I, Omacini M. An ecological framework for understanding the roles of Epichloë endophytes on plant defenses against fungal diseases. Fungal Biol Rev. 2020;34:115–25. [Google Scholar]
Cite This Article
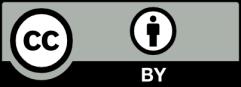
This work is licensed under a Creative Commons Attribution 4.0 International License , which permits unrestricted use, distribution, and reproduction in any medium, provided the original work is properly cited.