Open Access
ARTICLE
Phenotyping of Quinoa (Chenopodium quinoa Willd.) Genotypes for Morphological, Yield and Nutritional Quality Traits
1 Department of Genetics & Plant Breeding, Bangladesh Agricultural University, Mymensingh, 2202, Bangladesh
2 Department of Plant Science, University of Manitoba, Winnipeg, MB R3T 2N2, Canada
3 Department of Horticulture, Bangladesh Agricultural University, Mymensingh, 2202, Bangladesh
4 Grain Quality & Nutrition Division, Bangladesh Rice Research Institute, Gazipur, 1701, Bangladesh
5 Graduate School of Bioresources, Mie University, 1577 Kurimamachiya, Tsu, 514-8507, Japan
* Corresponding Author: Mohammad Anwar Hossain. Email:
(This article belongs to the Special Issue: Recent Research Trends in Genetics, Genomics, and Physiology of Crop Plants)
Phyton-International Journal of Experimental Botany 2024, 93(12), 3443-3463. https://doi.org/10.32604/phyton.2024.058786
Received 21 September 2024; Accepted 26 November 2024; Issue published 31 December 2024
Abstract
Quinoa (Chenopodium quinoa Willd.) is regarded as a superfood due to its exceptionally high nutritional value and ability to withstand stress. Six quinoa genotypes (viz., SAU Quinoa-1, Regalona, GPBQ-1, GPBQ-2, GPBQ-3, and GPBQ-4) were characterized for morphological, yield, and nutritional quality attributes while being grown under the agro-climatic conditions of Bangladesh. The field experiment was carried out in the winter season (November 2022–March 2023) at the Agronomy Field Laboratory of the Department of Agronomy, Bangladesh Agricultural University, Mymensingh, using a randomized complete block design with three replications. Data on various qualitative and quantitative traits related to growth and yield-attributes were recorded. A large variability was observed among the genotypes for growth habit, stem color, panicle shape, and panicle color at maturity. The whitish color of the seed was found in GPBQ-3 which indicates less saponin content as confirmed by saponin test. Yield attributing quantitative traits (viz., days to first flowering, days to maturity, plant height, stem diameter, panicle weight, 1000-seed weight, yield plant−1, above-ground biomass, and harvest index) also showed significant variation among the genotypes studied. Genotypes SAU Quinoa-1 and GPBQ-2 were identified as early maturing genotypes. The higher yield plant−1 was recorded in GPBQ-1 and GPBQ-3 genotypes. The phenotypic coefficient of variation for the majority of the traits evaluated was slightly higher than the corresponding genotypic coefficient of variation. For stem diameter, panicle weight, 1000-seed weight, yields plant−1, and above-ground biomass, high heritability and high genetic advancement were seen as percentages of the mean. Yield plant−1 showed significant positive correlation with days to first flowering, days to maturity, plant height, stem diameter, panicle weight, and above-ground biomass (0.568*, 0.812***, 0.744***, 0.895***, 0.993***, and 0.985***, respectively). The first two components accounted for 85.5% of the overall variation among the genotypes, according to principal component analysis. Significant variability was also found for seed mineral contents (viz., calcium, copper, iron, potassium, magnesium, manganese, and zinc) in the studied genotypes. The SAU Quinoa-1 genotype contained the highest amount of calcium and zinc, whereas, the highest amount of potassium was recorded in the GPBQ-1 genotype. We found a non-significant variability in carbohydrate, protein, fat, fiber, and vitamins (viz., thiamine, riboflavin, niacin, and folic acid) contents in the studied genotypes. Considering all the yield and nutritional quality traits under study, the genotypes GPBQ-1, GPBQ-3, and GPBQ-4 were selected for future variety development program.Keywords
Food systems are under intense pressure from climate change, which highlights the need for stable crops and varieties that can withstand abrupt soil and environmental changes. It is also important to diversify crops to address increasing yield losses that threaten food security, especially with the growing global population [1]. In addition to concerns about undernourishment, many people are not getting enough micronutrients, which is referred to as “hidden hunger” [2]. In Bangladesh, undernutrition continues to be a serious public health problem. Diversifying the food system is crucial, as climate change poses a threat to human nutrition and food security. One such strategy is the use of quinoa (Chenopodium quinoa Willd.) in our food system which is a highly nutritious and climate-resilient crop species.
Quinoa is known as a miracle crop for its great adaptation in diverse edaphic conditions. A dicotyledonous plant (C3), quinoa is a member of the Amaranthaceae family. It yields achene fruits, often known as chenopod grains, which have spherical seeds measuring 1.5–4 mm [3]. With ecotypes growing well at high elevations and in poor soils where other cereal crops, like wheat, rice, and maize, do not grow well, the quinoa plant’s extensive genetic diversity enables it to be highly resistant to cold, salt, and drought conditions [4]. While quinoa is becoming more and more popular as a food source globally, Andean indigenous communities have been consuming it for thousands of years [5]. Quinoa is frequently called a “superfood” because of its high nutritional content and resilience to stress. Because of its characteristics that resemble those of cereal grains like corn, wheat, and rice, quinoa is wrongly thought to be pseudo-cereal; in reality, it is categorized as a dicot [6]. The quinoa seed has been eaten in a variety of forms, including flour in baked and toasted foods (bread, noodles, biscuits, cookies, flakes, pancakes, and tortillas) and in breakfast cereals. Furthermore, quinoa is a very nutrient-dense food that includes a range of minerals (like calcium, iron, potassium, potassium, copper, magnesium, and zinc), vitamins (like A, B1, B2, B3, B5, B6, B9, C, and E), dietary fiber, natural antioxidants like polyphenols, and high-quality protein with a high concentration of essential amino acids (reviewed in Ain et al. [7]). Quinoa is processed using particular technologies to address specific health issues in people, like improving fitness, losing weight, celiac disease, and other metabolic issues like diabetes, obesity, hypertension, and hyperlipidemia [8]. After being rediscovered in the latter part of the 20th Century, quinoa has been studied and distributed to more than 120 nations worldwide [5]. Due to its exceptional nutritional value and balance, as well as its remarkable adaptability to a variety of unfavorable conditions like salt and drought, it is anticipated to become a key player in the global solution to food and nutrition issues.
Bangladesh is one of the countries that would be most affected by climate change. Climate change has increased the frequency of certain problems such as drought, unpredictable rainfall, high temperatures, sea level rise, and soil salinity that are both directly and indirectly related to agricultural production. Ensuring food security for all is one of the primary concerns that Bangladesh is now confronting. Salinity is a major threat to crop productivity in the southwestern part of Bangladesh affecting 30% of the arable land of the coastal areas [9]. Bangladesh’s rice yield is expected to drop by 33% by 2100 according to the climate change model, but we need to raise rice production by 50% to feed the country’s growing population. Importantly, rice production may fall by 10% due to salinity only by 2050 [10]. Therefore, it is high time to introduce crops like quinoa in Bangladesh to include the non-cultivated land of the southern part of the country under cultivation and increase cropping intensity, which in turn will boost the gross domestic product. Despite being the primary grain in Bangladesh, rice lacks essential nutrients, leading to high rates of malnutrition and micronutrient deficits in women and children [11]. As a result, quinoa can be a helpful addition to rice or other cereals in addressing malnutrition. The goal of quinoa breeding is to create cultivars that can thrive in a variety of agroclimatic zones and have higher grain yields and high-quality components. Nevertheless, not much has been done in Bangladesh to alter its genetic makeup or create a new crop variety. Although a quinoa variety called SAU Quinoa-1 has recently been released, there is currently little information available in Bangladesh regarding the characterization of quinoa for productivity, nutritional quality, and adaptive potential. To develop a variety, breeders need to evaluate a range of cultivars and genotypes to understand the phenotypic and genotypic variability present in the germplasm. In addition, understanding the genetic parameters such as genotypic co-efficient of variation, heritability and genetic advance and inter-relationship among the traits is quinoa essential for its genetic improvement through a reliable selection program. Consequently, an in-depth investigation of quinoa variability in terms of morpho-agronomic and qualitative characteristics is required. Development of improved quinoa variety through appropriate breeding techniques might have a significant impact on nutritional food security and sustainable agricultural production systems as well as to achieve the SDGs. We hypothesize that significant variation is present in the studied quinoa genotypes in terms of morphological, yield, and nutritional quality traits that might be helpful in boosting genetic gain through additional selective breeding. Considering the above issues in mind, the present investigation was thus aimed to (i) characterize exotic quinoa genotypes based on morphological, yield, and nutritional quality traits; and (ii) phenotypic and genotypic dissection of the traits for effective selection to develop new variety for maximizing genetic gain.
2.1 Experimental Site, Soils and Season
The field experiment was conducted during the winter season (November 2022–March 2023) at the Agronomy Field Laboratory of the Department of Agronomy, Bangladesh Agricultural University, Mymensingh. A map of the study site, along with its coordinates, is presented in Fig. 1. The trial site was categorized as medium high land in Agro-Ecological Zone-9 (Old Brahmaputra Flood Plain), with a sandy loam texture and a pH range of 6.5 to 6.7 in the soil. The location’s climate was typified by low temperatures and little rainfall during the winter or Rabi season (November to March), and comparatively high temperatures and considerable rainfall during the Kharif or summer season (April to October).
Figure 1: Bangladesh Agricultural University map along with the study site
The experiment was conducted using six quinoa genotypes out of which four genotypes (viz., GPBQ-1, GPBQ-2, GPBQ-3, and GPBQ-4) were collected from the University of Tasmania, Australia and the variety Regalona was collected from Uzbekistan, and SAU Quinoa-1 was collected from Sher-e-Bangla Agricultural University (SAU), Dhaka, Bangladesh.
2.3 Design and Layout of the Experiment
Using three replications, the experiment was carried out using a randomized complete block design. There were six experimental units in per block. The unit plot size was four square meters (2 m × 2 m). Each plot contained six rows, with a 30 cm gap between rows and a 5 cm gap between each plant in a row. Using a table of random numbers, the genotypes were put in each experimental unit in an arbitrary order.
2.4 Land Preparation, Fertilization and Seed Sowing
Using a power tiller, the experimental field was prepared by plowing and cross-ploughing. When the land was being prepared, the weeds and debris were pulled out. To tilt and level the soil properly, the right laddering was done. Full amount of all fertilizers was applied at the time of final land preparation except Urea. Fertilizer such as Urea-TSP-MoP-Gypsum-Zinc-Boron was applied 70 kg acre−1, 100 kg acre−1, 40 kg acre−1, 40 kg acre−1, 2 kg acre−1, and 2 kg acre−1, respectively. In the last stage of land preparation, the soil was combined with half of the urea and all of the other fertilizers. Twenty-five to thirty days after seeding, half of the urea was applied. Seeds of the quinoa genotypes were sown on 14 November 2022 following line sowing methods.
A light irrigation was applied for the quinoa cultivation after 30 days after seed sowing. Weeding and thinning were done one time just before the application of irrigation at 25 days after sowing to maintain a proper plant-to-plant distance of 5 cm.
2.6 Harvest and Post-Harvest Operations
Harvesting took place in February 2023 when 90% of the grain turned yellow to deep brown. Five mature plants were randomly collected from each plot, sundried, threshed, and placed in net bags for further drying. The seeds were cleaned and sun-dried for 3 to 5 days to achieve safe moisture level. The above-ground biomass was cut into pieces and also sun-dried for 3 to 5 days.
Data on ten qualitative traits such as growth habit (GH), stem shape (SS), plant stem color (PSC), pigmented axil (PA), leaf shape (LS), leaf margin (LM), panicle shape (PS), panicle color at flowering (PCF), panicle color at maturity (PCM), and seed color (SC) were taken from randomly selected plants. These traits were measured according to descriptors for quinoa as described by Stanschewski et al. [12].
Data on nine quantitative traits such as days to first flowering (DFF), days to maturity (DM), plant height (PH) (cm), stem diameter (SD) (mm), panicle weight (PW) (g), 1000-seed weight (1000-SW) (g), yield plant−1 (YPP) (g), above-ground biomass (AGB) (g), harvest index percentage (HI%) were taken from five plants chosen at random from each genotype’s replication.
2.7.3 Nutritional Quality Traits
The seeds were analyzed using the “Official Methods of Analysis of AOAC International” [13] to gather information related to nutritional quality attributes such as fiber, protein, fat, and carbohydrates. We used a Shimadzu ICP-OES 9820 to measure calcium (Ca), magnesium (Mg), iron (Fe), zinc (Zn), and potassium (K) in mgKg−1 (ppm). Utilizing Ultra-Pressure Liquid Chromatography (UPLC), the amount of water-soluble B vitamins (B1, thiamine; B2, riboflavin; B3, niacin; and B9, folic acid) was measured from the quinoa samples. Vitamin contents were expressed as mg/100 g, while all other micronutrients were expressed as mg kg−1.
The following technique, as outlined by Stanschewski et al. [12], was used to measure the seed saponin content. Simply, five loosely-hulled quinoa seeds were taken in a 1.5 mL microcentrifuge tube and then 500 μL of double-distilled water was added. The tubes were then violently shaken for thirty seconds by hand until foam with a consistent height appeared. The intensity of the saponin component was shown by foam height.
2.8 Estimation of Genetic Parameters
Genetic parameters, including genetic variance, heritability in the broad sense (h2b), phenotypic co-efficient of variation (PCV) and genotypic co-efficient of variation (GCV), genetic advance (GA) and genetic advance as percentage of mean (GA%) for the quantitative traits under study, were calculated using equations put forth by biometricians such as Allard [14] and Johnson et al. [15]. The PCV and GCV estimates were categorized as low, <10%, moderate, 10%–20%, high, >20% according to Deshmukh et al. [16]. The heritability in the broad sense (h2b) was calculated and classified as low, 0%–30%, medium, 31%–60%, high, >60%, and the GA% as low <10%, moderate, 10%–20%, and high, >20% according to Johnson et al. [15].
SPSS was utilized to do a one-way analysis of variance (ANOVA) for every trait in order to investigate the variations among the genotypes. To differentiate the means, the Tukey’s test was used at a 5% probability level. In order to identify quantitative variation patterns with the eigen vectors and eigen values, Minitab 19 statistical software was used for principal component analysis (PCA). We used the formula proposed by Miller et al. [17] to estimate the phenotypic correlations.
For the examined growth-related qualitative features, a significant amount of variation was found between the genotypes (Table 1). Most of the genotypes (i.e., SAU Quinoa-1, Regalona, GPBQ-2, and GPBQ-4) showed simple growth habit but two genotypes (GPBQ-1 and GPBQ-3) showed branching from the base with more significant panicle type growth habit (Fig. 2). Green color stem was noted in the genotypes Regalona, GPBQ-1, GPBQ-3, and GPBQ-4, whereas yellow color stem was found in SAU Quinoa-1 and GPBQ-2. The stem shape of all the studied six genotypes was angular and also had pigmented axile. Three types of leaf shapes (viz., rhomboidal, triangular, and linear) were present in all the studied quinoa genotypes and rhomboidal and triangular types leaf contain dentate-type leaf margins and linear-type leaves contain entire leaf margins in all the studied genotypes (Fig. 3). Four quinoa genotypes such as SAU Quinoa-1, Regalona, GPBQ-2 and GPBQ-4 had intermediate-type panicle shapes while the genotypes GPBQ-1 and GPBQ-3 had glomerulate-type panicles. At the flowering stage, all of the genotypes showed green color panicle, however, three genotypes such as SAU Quinoa-1, GPBQ-2 and GPBQ-4 showed orange color, two genotypes (GPBQ-1 and GPBQ-3) showed pink color, and one genotype (Regalona) showed yellow color panicle at physiological maturity stage (Fig. 4). Whitish color seed was found in the genotype GPBQ-3, light brown in Regalona, light yellow in GPBQ-1 and deep brown was seen for the genotypes SAU Quinoa-1, GPBQ-2, and GPBQ-4 (Fig. 5).
Figure 2: Variation in growth habit of the studied quinoa genotypes grown at BAU farm during the winter season of 2022–2023
Figure 3: Variation in leaf shape and leaf margin of the studied quinoa genotypes grown at BAU farm during the winter season of 2022–2023
Figure 4: Variation in panicle color at physiological maturity of the studied quinoa genotypes grown at BAU farm during the winter season of 2022–2023
Figure 5: Variation in seed color of the studied quinoa genotypes grown at BAU farm during the winter season of 2022–2023
For all of the quantitative characters examined, the analysis of variance results revealed highly significant (p < 0.001) genotypic variation (Table A1). The mean performance of nine quantitative traits across six quinoa genotypes is presented in Table 2. Among the studied genotypes, the genotypes GPBQ-1 and GPBQ-3 required maximum days to first flowering (27.33 days), whereas SAU Quinoa-1 and GPBQ-2 required minimum days (22.33 days) for flowering and they were significantly different from the rest of the genotypes (Table 2). GPBQ-2 and SAU Quinoa-1 were the early maturing genotypes as these genotypes required minimum days (74.67 and 75.00 days, respectively), to mature. In contrast, GPBQ-1 and GPBQ-3 required maximum days to mature (84.67 and 85.00 days, respectively). Maximum PH (101.45 cm) was recorded in the genotype GPBQ-1 followed by GPBQ-3, Regalona, GPBQ-4, and SAU Quinoa-1 (100.33, 95.20, 90.68 and 86.20 cm, respectively), whereas minimum plant height (81.07 cm) was recorded in GPBQ-2 (Table 2). GPBQ-1 showed the maximum stem diameter (10.84 mm), however, the minimum was found in Regalona (6.54 mm). Among the studied genotypes, GPBQ-1 showed the highest panicle weight (30.69 g) followed by GPBQ-3, GPBQ-4, SAU Quinoa-1, and Regalona (20.58, 15.51, 12.54, and 11.37 g, respectively) whereas the lowest panicle weight was produced by GPBQ-2 (10.87 g). Maximum of 1000-SW (4.61 g) was recorded in GPBQ-4, followed by SAU Quinoa-1, Regalona, GPBQ-2, and GPBQ-3 (3.74, 3.63, 3.47, and 3.24 g, respectively). In contrast, the minimum of 1000-SW was found in GPBQ-1 (2.29 g). Importantly, the highest yield plant−1 (22.40 g) was recorded in GPBQ-1 followed by GPBQ-3, GPBQ-4, SAU Quinoa-1, and Regalona (14.80, 10.48, 9.46, and 7.97 g, respectively), however, the minimum (7.80 g) YPP was recorded in GPBQ-2. In case of above ground biomass, maximum was found in GPBQ-1 (61.74 g) and the minimum amount was found in GPBQ-2 (19.66 g). SAU Quinoa-1 showed maximum harvest index (54.32%) (Table 2).
3.3 Genetic Variability Analysis
Variances in phenotype and genotype, PCV, GCV, heritability, GA, and GA% of the traits under study are displayed in (Table 3). The characters that showed high phenotypic and genotypic variance were AGB (236.62 and 234.63, respectively), PH (65.47 and 63.99, respectively), PW (59.59 and 57.68, respectively), and YPP (31.92 and 31.58, respectively), whereas, the lowest phenotypic and genotypic variances were recorded in 1000-SW (0.61 and 0.55, respectively). Co-efficient of variation studies indicated that the values of PCV were higher than those of the GCV for all the traits. In contrast, the characteristics of DM (5.86 and 5.66, respectively) and HI (4.68 and 4.08, respectively) had the lowest PCV and GCV values, while the trait of YPP had the highest values (46.49 and 46.24, respectively). Overall, all of the examined traits showed strong broad-sense heritability (>60%). While HI had the lowest heritability (75.61%), AGB had the highest heritability (99.16%). The highest genetic advance was found in ABG (31.42). Genetic advance as a percentage of the mean (GA%) ranged from 7.29% to 94.74%. High GA% (>20%) was found in YPP (94.74) followed by AGB, PW, SD, and 1000-SW, (94.13%, 90.95%, 43.53%, and 41.63%, respectively). Medium GA% (20%–10%) was recorded in PH, DFF, and DM 17.61%, 16.64%, and 11.26%, respectively, on the other hand, low GA% (<10%) was found in HI (7.29) (Table 3).
3.4 Correlation Co-efficient Analysis
The association between yield and yield-contributing features was evaluated using the simple Pearson’s correlation coefficient, and the findings are shown in Table 4. Days to first flowering showed significant positive correlation with DM (0.735***), PH (0.831***), SD (0.63**), PW (0.611**), YPP (0.568*) and AGB (0.617**), whereas, it showed a non-significant negative correlation with 1000-SW and HI. Days to maturity had a positive significant correlation with PH (0.908***), SD (0.846***), PW (0.817***), YPP (0.812***), and AGB (0.792***). On the other hand, it demonstrated a noteworthy inverse relationship with 1000-SW (−0.574*). Plant height had a positive significant correlation with SD (0.738***), PW (0.745***), YPP (0.744***) and AGB (0.768***) but it showed a significant negative correlation with 1000-SW (0.517*). In contrast to its strong negative connection with 1000-SW (−0.628*), stem diameter exhibited a significant positive correlation with PW (0.901***), YPP (0.895***), and AGB (0.851***). Panicle weight showed significant positive correlation with YPP (0.993***) and AGB (0.98***). On the contrary, it showed a significant negative correlation with 1000-SW (−0.664**). 1000-seed weight showed significant negative correlation with YPP (−0.715***), and AGB (−0.671**), however, it showed a non-significant positive correlation with HI. Importantly, yield plant−1 showed significant positive correlation with DFF (0.568*), DM (0.812***), PH (0.744***), SD (0.895***), PW (0.993***), and AGB (0.985***).
3.5 Principal Component Analysis (PCA)
Table 5 shows the PCA that was performed taking into account every quantitative trait. There was a cumulative 85.5% variation in the quinoa genotypes for the nine quantitative variables that were investigated, explained by two components that had Eigen values larger than one. PCA revealed that the first component accounted for 69.5% of the total variation where PW (0.383) and YPP (0.383) had the highest positive loadings followed by AGB (0.380), SD (0.370), DM (0.368), PH (0.354) and DFF (0.293), respectively, whereas, the characters 1000-SW (−0.278) and HI (−0.010) showed the negative loadings. The second component accounted for 16% of the total variation. The highest positive loadings were found for the character DFF (0.458) followed by 1000-SW (0.416), PH (0.190), and DM (0.118), respectively, whereas, the highest negative loadings were found for the trait HI (−0.724) followed by YPP (−0.159), AGB (−0.089), and SD (−0.013), respectively.
Significant differences in mineral concentrations were found between the genotypes under study (Table 6 and A2). The highest amount of Ca2+ was found in SAU Quinoa-1 (630.2 mg kg−1) on the other hand, the lowest amount of Ca2+ was found in GPBQ-1 (433.5 mg kg−1). The higher Cu2+ content was recorded in Regalona (6.36 mg kg−1) whereas SAU Quinoa-1 contained low amount of Cu2+ (4.74 mg kg−1) as compared to others. GPBQ-4 showed the highest Fe2+ content (44.3 mg kg−1) whereas GPBQ-2 had the lowest amount of Fe2+ content (42.7 mg kg−1). Maximum K+ content was found in GPBQ-1 (6157.9 mg kg−1) and the minimum amount was found in Regalona (5603.4 mg kg−1). Regalona contained higher amount of Mg2+ (1196 mg kg−1) on the contrary, GPBQ-1 (1098.8 mg kg−1) contained lower amounts of Mg. GPBQ-2 was highly enriched with Mn2+ (13.69 mg kg−1) but less amount was recorded in Regalona (12.30 mg kg−1). SAU Quinoa-1 had the highest amount of Zn2+ content (29.4 mg kg−1), whereas, the lowest amount was recorded in GPBQ-1 (22.1 mg kg−1).
Table 7 displays the vitamin content data for the genotypes under study. The genotypes did not differ significantly in terms of specific vitamin content, according to the results. Vitamin B1 content ranged from 1.43 to 1.31 mg/100 g dry matter whereas B2 ranged from 2.34–2.85 mg/100 g. Almost every genotype had nearly 1 mg of B3 content per 100 g dry matter. Among the vitamin contents studied, B9 content was higher in all of the quinoa genotypes, and the value ranged from 6.45–6.77 mg/100 g.
3.8 Proximate Composition of Quinoa Seeds
The nutritional composition of different quinoa genotypes is presented in Fig. 6. The main constituents of quinoa seeds were the available carbohydrate ranged from 68.21%–68.83%, and followed by protein (14.12%–14.87%), fat (6.37%–6.61%) and fibre (3.15%–3.48%). Importantly, proximate composition of the studied quinoa genotypes showed a non-significant difference.
Figure 6: Proximate composition of the seeds of the studied quinoa genotypes grown at BAU farm during the winter season of 2022–2023
Saponin is an anti-nutritional component that causes bitterness in quinoa. In our study, GPBQ-3 had the lowest amount of saponin followed by GPBQ-4, Regalona, GPBQ-2 and GPBQ-1, respectively. The highest amount of saponin content was found in SAU Quinoa-1 (Fig. 7).
Figure 7: Saponin content in the seeds of the studied quinoa genotypes grown at BAU farm during the winter season of 2022–2023
Continuous collection, characterization, and evaluation of unknown quinoa genotypes are urgently needed in developing nations like Bangladesh due to the need for high-quality quinoa products and its exceptional tolerance to harsh climates. Characterization and documentation of quinoa genotypes are therefore imperative for current and future quinoa breeders to ensure global food and nutritional security. In the current study, we have studied the adaptive potential of six quinoa genotypes and also characterized these genotypes based on morphological, yield, and nutritional quality traits. Although these physical characteristics are typically impacted by the environment, measurements of morphological attributes offer a straightforward method of evaluating genotype performance under certain growth conditions while also analyzing genetic variation [18]. The current research results identified a significant diversity among the quinoa genotypes concerning various qualitative traits (Table 1). The majority of genotypes exhibited a simple growth habit (Fig. 1), and two genotypes displayed branching from the base, indicating potential genetic differences in plant architecture as their panicle shape became glomerular which further provided higher yield. According to Kir and Temel [19], planting density, nutrition, and other environmental elements all affect the branching habit. Nevertheless, there was diversity in stem color, with most showing green stems while a few displayed yellow ones, indicating potential genetic differences in pigment synthesis pathways. A similar type of phenotypic variation was also reported by Tabatabaei et al. [20] and Coronado et al. [21]. Furthermore, the presence of pigmented axils in all genotypes highlights a common genetic determinant for this trait. Leaf morphology (Fig. 3) across all genotypes in terms of shape was rhomboidal, triangular, and linear, whereas, the margin was entire and dentate. Panicle shape and color also showed diversity, with implications for seed yield and quality (Fig. 4). In a study resembling ours, Sarwar et al. [22] also reported that quinoa plants exhibited an angular yellow-green stem and simple, rhomboidal green leaves. They observed that the panicle shape was intermediate, displaying both glomerular and amaranthiform shapes. Additionally, they also noted that the panicle was green during flowering but turned pink, orange, and yellow at the physiological maturity stage. Similar to our results, variability in inflorescence color was also reported by Voronov et al. [23]. Characteristics variation was also observed in the seed color of the genotypes studied (Fig. 5). Variation in seed color in different quinoa genotypes was also reported by others [24].
Variability in that particular attribute among the available germplasm is necessary in order to use it in a quinoa breeding program. Additionally, the adaptive potentiality of a particular genotype is assayed based on yield under particular agroclimatic conditions. Significant variations were found between the genotypes and attributes under investigation in the current experiment’s analysis of variance viz., DFF, DM, PH, SD, PW, 1000-SW, YPP, AGB, and HI. Significant variability in yield and yield-attributing traits in quinoa was also reported by de Santis et al. [24], Pathan et al. [25], and Toderich et al. [26]. In the present study, DFF ranged from 22.33 to 27.33 days and DM ranged from 74.67–85.00 days. Similar to our results, Manjarres-Hernández et al. [27] and Pathan et al. [25] also reported that flowering and maturity in quinoa range from 68–121 days and 80–106 days, respectively. While de Santis et al. [24], Bhargava et al. [28], Shah et al. [29], and Granado-Rodríguez et al. [30] reported a longer mean DM 129 days (range: 109–163 days), 116 days (range: 95–150 days), 131 days (range: 105–148 days) and 119 days (range: 107 to 135 days) respectively. The genotypes used in our experiment were primarily short-duration genotypes. These short-duration genotypes hold significant potential for developing early-maturing quinoa varieties, which could be advantageous for regions with drought, shorter growing seasons, or for achieving multiple cropping cycles within a year. The PH of the genotypes ranged from 81.07 to 101.54 cm. This finding is similar to the observation of Granado-Rodríguez et al. [30] who also found that the PH ranged from 60 to 135 cm. Additionally, the studies by Coronado et al. [21], Bhargava et al. [28], Coronado et al. [31], and Laki et al. [32] also support this result demonstrating similar range and mean of PH. The SD of the current study ranged from 6.54 to 10.84 mm. Similar to our results, Tang et al. [33] also reported that stem thickness ranges from 9.64–10.72 mm in low-, medium-, and high-yielding genotypes. Therefore, the variability in PH and SD further highlighted distinct growth patterns among genotypes, with GPBQ-1 demonstrating the tall stature and thickest stems, and GPBQ-2 showing the short stature and smallest dimensions, indicating potential differences in resource allocation and yield potentiallity. The genotypes differed significantly in PW and AGB. Panicle weight ranged from 10.87 to 30.69 g, while AGB ranged from 19.66 to 61.74 g. Bhargava et al. [28] also found that the AGB ranged from 1.11 to 52.89 g. The thousand seed weight of the genotypes varied from 2.29 to 4.61 g, which was similar with the findings of Coronado et al. [21], Sarwar et al. [22], Bhargava et al. [28], Granado-Rodríguez et al. [30] and Craine et al. [34]. The harvest index (HI) of the genotypes ranged from 48.31% to 50.68%, which was higher than the mean HI reported by Laki et al. [32] and Rojas [35], and they showed that the HI ranged from 34% to 40%, respectively. Quinoa typically exhibits low average HI values, as noted by Bertero et al. [36]. The higher HI observed in the genotypes indicates a greater proportion of biomass is allocated to seed yield, suggesting higher economic efficiency. A significant variation in YPP was found in the genotypes which ranged from 7.80 to 22.40 g which was similar to the results reported by Shah et al. [29] and Manugade et al. [37] where YPP mean were 19.86 and 15.00 g, respectively. In contrast, de Santis et al. [24], Bhargava et al. [28] and Craine et al. [34] reported much lower mean YPP of 4.06, 5.51 and 6.33 g, respectively. These findings suggest the presence of high-yielding genotypes in our study, which could be valuable for selection programs aimed at breeding improvements and the development of high-yielding quinoa varieties.
The existence of genetic diversity and the patterns of inheritance of the desired traits are critical to crop breeding success. Analyzing genetic variation is crucial for breeders as it enables them to identify suitable breeding schemes and selection criteria for improving target traits. Therefore, generally, the phenotypic variance is larger than the genotypic variance. A similar statement was also given by Al-Naggar et al. [38] that PCV was a little higher than GCV for all studied traits. Higher heritability and less environmental effect on the expression of a particular trait were indicated by a low difference between genotypic and phenotypic variance. The PCV was marginally greater than the GCV for the majority of the characteristics examined in this study, indicating that environmental influences have little effect on trait expression. Importantly, SD, PW, 1000-SW, YPP and AGB displayed the highest values for both PCV and GCV, indicating considerable potential for genetic improvement in these traits through selection.
Heritability estimations aid in the selection of plants by breeders according to phenotypic performance. Genetic gains in succeeding generations are generally higher in cases of higher heritability. A high heritability also suggests a high degree of genetic control over the particular trait, which aids in the efficient selection of a particular trait for future development [39,40]. For the majority of the traits in the current study, the estimated heritability ranged from 90 to 100 percent (Table 3). This indicated that the environment had little effect on the traits under study, which are mostly controlled by genetics. The GA, a measure of the potential for genetic improvement, varied across traits, with AGB exhibiting the highest value and 1000-SW the lowest. When expressed as a percentage of the mean, GA ranged from 7.29% to 94.74%, with YPP showing the highest value and HI the lowest. High heritability (>60%) coupled with high genetic advance (>20%) was recorded for YPP, AGB, PW, SD and 1000-SW. In quinoa, other people have experienced similar outcomes [24,28]. Since the expression of these attributes is greatly influenced by genetic constitution, this data implies that genetic improvement of these traits is extremely likely and that selection based on phenotypic expression may be a useful tactic.
The correlation coefficient analysis reveals the intricate relationships among various yield and yield-contributing traits (Table 4). The correlation analysis underscores the significance of YPP to various crucial traits. YPP exhibited significant positive correlations with DFF, DM, PH, SD, PW, and AGB (0.568*, 0.812***, 0.744***, 0.895***, 0.993***, 0.985***, respectively), whereas, it showed significant negative correlation with 1000-SW (−0.715***). Positive associations imply that an increase in YPP corresponds to increases in these traits, suggesting a potentially synergistic relationship that contributes to overall yield enhancement. Similar to our results, de Santis et al. [24], and Bhargava et al. [28] reported a significant positive correlation between YPP, DFF and PH. de Santis et al. [24] and Bhargava et al. [28] shed light on the importance of morphological traits such as DFF, DM, PH, 1000-SW, leaf area, branches plant−1, inflorescence length, inforesence plant−1 and harvest index in influencing grain yield in quinoa genotypes. This suggests that genotypes with taller plants are likely to produce higher grain yields in quinoa cultivation. Pathan et al. [25] reported a similar finding with ours that yield had positive correlation with DM and PH. Furthermore, there was a significant negative correlation observed between YPP and 1000-SW, suggesting that as YPP increases, there is a tendency for 1000-SW to decrease. This negative correlation suggests a trade-off scenario where increasing yield per plant may lead to a reduction in individual seed size, this is the opposite of de Santis et al. [24], studies. Thus, the genotypes with greater PH, SD, and ABG, as well as early flowering and maturity, should be chosen in order to increase yield in quinoa breeding, according to the aforementioned findings.
The PCA conducted on the quantitative traits of six quinoa genotypes yielded insightful findings. The PCA unveiled two components, collectively explaining 85.5% of the total variation among the studied traits. Notably, the first two components accounted for a significant portion of this variability, cumulatively explaining 85.5%. Manjarres-Hernández et al. [27] and Wu et al. [41] found that 59.26% to 64.6% of the observed phenotypic variation is explained by the first two components. The first component, responsible for 69.5% of the variance, underscored the substantial positive influence of traits like PW and YPP, indicating their pivotal roles in shaping overall trait variability. Traits such as 1000-SW and HI exhibited negative loadings on this component. This suggests that as YPP increases, there may be a tendency for 1000-SW and HI to decrease, implying a possible trade-off scenario between YPP and individual seed size. The second component, contributing 16% to the total variation, emphasized the significance of traits such as DFF and 1000-SW in further delineating trait variability among the studied genotypes. These findings offer valuable insights into the underlying relationships among the traits and their contributions to overall trait diversity within quinoa genotypes. By elucidating the role of these specific traits in shaping trait variability, the PCA results provide a deeper understanding of the complex dynamics governing trait expression and variability in quinoa populations.
Mineral contents (viz., Ca2+, Cu2+, Fe2+, K+, Mg2+, Mn2+, and Zn2+) measured in the quinoa genotypes showed significant variations which indicates a substantial diversity in mineral accumulation within the quinoa varieties under evaluation. Consistent with our findings, significant differences in the mineral contents of quinoa grains were also observed by Nowak et al. [42] and de Bock et al. [43]. Moreover, similar variability was also mentioned by others [28,43–45], where they quantified a large variation in micronutrients levels like Ca2+ (275 to 1487 mg kg−1), Cu2+ (2 to 51 mg kg−1), Fe2+ (14 to 168 mg kg−1), Mg2+ (260 to 5020 mg kg−1), K+ (75 to 12000 mg kg−1), and Zn2+ (28 to 48 mg kg−1) in various diverse quinoa genotypes of geographical origin.
The examination of proximate composition (Fig. 6) in the studied quinoa genotypes revealed uniformity across various nutritional components, including carbohydrate (68.21%–68.83%), protein (14.12%–14.87%), fat (6.37%–6.61%), and fiber content (3.15–3.48%). Similar to our results, Pedrali et al. [46], Agarwal et al. [47] and Oustani et al. [48] also reported that carbohydrate content in quinoa genotypes ranged from 55%–77%, protein content ranged from 13%–19%, fat content ranged from 5%–8% and soluble dietary fiber content ranged from 1.3%–4.1%. These nutritional indices did not significantly differ across the cultivars under study, despite phenotypic diversity. This consistency in grain composition aligns with previous studies that have also reported minimal variability in protein and lipid content among different quinoa genotypes by Repo-Carrasco-Valencia et al. [44] and Pedrali et al. [46] however, they have reported significant differences in carbohydrate and fiber content. The insignificant differences in proximate composition among genotypes suggest a high level of stability in the nutritional quality of quinoa grains, which is advantageous for consumers seeking consistent nutritional benefits [49]. However, further research investigating a broader range of genotypes and environmental conditions may provide deeper insights into the factors influencing quinoa grain composition.
The assessment of B-vitamin complexes showed less variability across the studied quinoa genotypes. B1, B2, B3, and B9 content showed non-significant differences among the genotypes. The amount of B3 content ranged from 1.05–1.21 mg/100 g. Similar to our results, Kozioł [50] also reported 1.06 g 100 g−1 of B3 content in quinoa. Importantly, B9 content was higher as compared to the others which ranged from 6.45–6.87 mg 100 g−1. Similar to our results, Agarwal et al. [47] also reported 6.50 mg 100 g−1 of B9 content in quinoa. These findings suggest that quinoa is a rich source of essential B-vitamin complex which is vital for various physiological functions.
A class of natural substances called saponins is generated by certain plants to guard against insects, birds, and hazardous microbes [51]. Researchers reported the presence of saponin, an anti-nutritional substance causing bitterness in quinoa, across different quinoa varieties (reviewed in Suárez-Estrella et al. [52]). In quinoa, these substances are mostly found in the husk. Our study also reported the presence of varying degrees of saponin content among the tested quinoa genotypes, GPBQ-3 contained the lowest amount content of saponin content while SAU Quinoa-1 showed the highest amount of saponin (Fig. 7). Significant variation in saponin content in quinoa genotypes was also reported by others [53]. In another study, Diaz-Valencia et al. [54] reported that quinoa grains contain about 0.00%–4.4% saponins. Although it has been considered as antinutritional fact, recent studies reported that saponin has some positive roles for human health. El Hazzam et al. [55] concluded that quinoa saponins have many important nutritional characteristics such as anticancer, antiobesity, analgesic, hypocholesterolemic, antiallergic, and antioxidants.
Significant variability was observed among the studied qualitative (viz., GH, PSC, PS, PCM, and SC) as well as quantitative traits (viz., DFF, DM, PH, SD, PW, 1000-SW, YPP, AGB, and HI) which offers ample scope of improvement of quinoa for yield and quality. Few of the studied genotypes (viz., GPBQ-1, GPBQ-3, and GPBQ-4) showed better yield performance as compared to the commercial varieties. For the majority of the characteristics under study, PCV was found to be marginally greater than GCV, indicating that environmental influences had little effect on trait expression. The highest values for PCV and GCV were shown by SD, PW, 1000-SW, YPP, and AGB, suggesting significant potential for selection-based genetic improvement in these variables. High h2b coupled with high GA% was recorded for SD, PW, 1000-SW, YPP, and AGB which indicates that improvement could be made in the aforesaid characters. YPP showed a significant positive correlation with DFF, DM, PH, SD, PW, and AGB (0.568*, 0.812***, 0.744***, 0.895***, 0.993***, 0.985***, respectively). This suggests that increased YPP could be achieved by proper selection pressure. According to PCA analysis, the first three PCs accounted for 85.5% of the genotypes’ overall variation. Significant variation was also observed among the genotypes for vitamins and mineral contents, however, a non-significant variation was observed for carbohydrate, protein, fat, and fiber contents. Considering all the traits, the genotypes GPBQ-1, GPBQ-3, and GPBQ-4 can be considered as potential in terms of yield and others. According to the findings of our research, quinoa can be added to the cropping systems as a new winter crop in Bangladesh. However, further research should be conducted under variable agroclimatic conditions in Bangladesh to study their stability and suitability for release as a new variety.
Acknowledgement: The authors gratefully acknowledge Bangladesh Rice Research Institute, Gazipur-1701, Bangladesh for providing the facilities to measure the nutritional quality traits. Special thanks to Professor Dr. Murad Ahmed Farukh, Department of Environmental Science, Bangladesh Agricultural University, Bangladesh, for preparing the the map of the study site.
Funding Statement: The authors received no specific funding for this study.
Author Contributions: Study conception and design: Jannatul Naim, Kristina Toderich, Mohammad Anwar Hossain; data collection: Jannatul Naim, Sheikh Mahfuja Khatun, Biswajit Das, Mumtarin Haque Mim, Sadia Akter; analysis and interpretation of results: Jannatul Naim, Masudur Reza Shakil, Habibul Bari Shozib; draft manuscript preparation: Jannatul Naim, Sheikh Mahfuja Khatun, Biswajit Das; Writing—review & editing: Habibul Bari Shozib, Kristina Toderich, Mohammad Anwar Hossain. All authors reviewed the results and approved the final version of the manuscript.
Availability of Data and Materials: Data available on request from the authors.
Ethics Approval: Not applicable.
Conflicts of Interest: The authors declare no conflicts of interest to report regarding the present study.
References
1. Challinor AJ, Watson J, Lobell D, Howden S, Smith DR, Chhetri N. A meta-analysis of crop yield under climate change and adaptation. Nat Clim Change. 2014;4(4):287–91. doi:10.1038/nclimate2153. [Google Scholar] [CrossRef]
2. Burchi F, Fanzo J, Frison E. The role of food and nutrition system approaches in tackling hidden hunger. Int J Environ Res Public Health. 2011;8(2):358–73. doi:10.3390/ijerph8020358. [Google Scholar] [PubMed] [CrossRef]
3. Garcia M, Condori B, Del Castillo C. Agroecological and agronomic cultural practices of quinoa in South America. In: Murphy K, Matanguihan J, editors. Quinoa: improvement and sustainable production, New Jersey: Wiley-Blackwell; 2015. p. 25–45. doi:10.1002/9781118628041.ch3. [Google Scholar] [CrossRef]
4. Jacobsen SE, Mujica A, Jensen CR. The resistance of quinoa (Chenopodium quinoa Willd.) to adverse abiotic factors. Food Rev Int. 2003;19(1–2):109. doi:10.1081/FRI-120018872. [Google Scholar] [CrossRef]
5. Alandia G, Rodriguez J, Jacobsen SE, Bazile D, Condori B. Global expansion of quinoa and challenges for the Andean region. Glob Food Secur. 2020;26(2):100429. doi:10.1016/j.gfs.2020.100429. [Google Scholar] [CrossRef]
6. Kadereit G, Borsch T, Weising K, Freitag H. Phylogeny of Amaranthaceae and Chenopodiaceae and the evolution of C4 photosynthesis. Int J Plant Sci. 2003;164(6):959–86. doi:10.1086/378649. [Google Scholar] [CrossRef]
7. Ain QT, Siddique K, Bawazeer S, Ali I, Mazhar M, Rasool R, et al. Adaptive mechanisms in quinoa for coping in stressful environments: an update. Peer J. 2023;11(1):e14832. doi:10.7717/peerj.14832. [Google Scholar] [PubMed] [CrossRef]
8. Adetunji CO, Michael OS, Kadiri O, Varma A, Akram M, Oloke JK, et al. Quinoa: from farm to traditional healing, food application, and phytopharmacology. In: Varma A, editor. Biology and biotechnology of quinoa. Singapore: Springer; 2021. p. 439–66. doi:10.1007/978-981-16-3832-9_20. [Google Scholar] [CrossRef]
9. Haque SA. Salinity problems and crop production in coastal regions of Bangladesh. Pak J Bot. 2006;38(5):1359–65. [Google Scholar]
10. IPCC. Fourth assessment report of the intergovernmental panel on climate change: the impacts, adaptation and vulnerability (Working Group III). New York, NY, USA: Cambridge University Press; 2007. [Google Scholar]
11. World Bank. Maintaining Momentum to 2015: an impact evaluation of interventions to Improve maternal and child health and nutrition in Bangladesh; 2015. Available from: https://www.researchgate.net/publication/23742550. [Accessed 2024]. [Google Scholar]
12. Stanschewski CS, Rey E, Fiene G, Craine EB, Wellman G, Melino VJ, et al. Quinoa phenotyping methodologies: an international consensus. Plants. 2021;10(9):1759. doi:10.3390/plants10091759. [Google Scholar] [PubMed] [CrossRef]
13. AOAC International. Official methods of analysis of AOAC International. 18th edGaithersburg, MD, USA: Association of Official Analysis Chemists International; 2019. [Google Scholar]
14. Allard RW. Principles of plant breeding. New York, NY, USA: John Willey and Sons. Inc.; 1960. vol. 485. [Google Scholar]
15. Johnson HW, Robinson HF, Comstock RE. Estimates of genetic and environmental variability in soybeans. Agron J. 1955;47(9):314–8. doi:10.2134/agronj1955.00021962004700070009x. [Google Scholar] [CrossRef]
16. Deshmukh SN, Basu MS, Reddy PS. Genetic variability, character association and path coefficients of quantitative traits in Virginia bunch varieties of groundnut. Indian J Agric Sci. 1986;56(12):816–21. doi:10.13140/RG.2.2.28616.96008. [Google Scholar] [CrossRef]
17. Miller P, Williams J, Robinson H, Comstock R. Estimates of genotypic and environmental variances and covariances in upland cotton and their implications in selection. Agron J. 1958;50:126–31. [Google Scholar]
18. Garcia E, Jamilena M, Alvarez JI, Arnedo T, Oliver JL. Genetic relationships among melon breeding lines revealed by RAPD markers and agronomic traits. Theor Appl Genet. 1998;96(6–7):878–85. doi:10.1007/s001220050815. [Google Scholar] [CrossRef]
19. Kir AE, Temel S. Determination of seed yield and some agronomical characteristics of different quinoa (Chenopodium quinoa Willd.) variety and populations under dry conditions of Iğdır Plain, Iğdır University. J Instit Sci Technol. 2016;4(6):145–54. doi:10.21597/jist.2016624166. [Google Scholar] [CrossRef]
20. Tabatabaei I, Alseekh S, Shahid M. The diversity of quinoa morphological traits and seed metabolic composition. Sci Data. 2022;9(1):323. doi:10.1038/s41597-022-01399-y. [Google Scholar] [PubMed] [CrossRef]
21. Coronado ACM, Hernández EHM, Coronado YM. Phenotypic diversity of agromorphological characteristics of quinoa (Chenopodium quinoa Willd.) germplasm in Colombia. Sci Agrícola. 2022;79(4):1. doi:10.1590/1678-992X-2021-0017. [Google Scholar] [CrossRef]
22. Sarwar AKMG, Khatun MM, Fakir MSA. Quinoa—a functional food crop: morphological descriptors. J Bangladesh Agric Univ. 2023;21(1):12–22. doi:10.5455/JBAU.141111. [Google Scholar] [CrossRef]
23. Voronov S, Pleskachiov Y, Shitikova A, Zargar M, Abdelkader M. Diversity of the biological and proteinogenic characteristics of quinoa genotypes as a multi-purpose crop. Agronomy. 2023;13(2):279. doi:10.3390/agronomy13020279. [Google Scholar] [CrossRef]
24. de Santis G, D.’Ambrosio T, Rinaldi M, Rascio A. Heritabilities of morphological and quality traits and interrelationships with yield in quinoa (Chenopodium quinoa Willd.) genotypes in the Mediterranean environment. J Cereal Sci. 2016;70:177–85. doi:10.1016/j.jcs.2016.06.003. [Google Scholar] [CrossRef]
25. Pathan S, Ndunguru G, Clark K, Ayele AG. Yield and nutritional responses of quinoa (Chenopodium quinoa Willd.) genotypes to irrigated, rainfed, and drought-stress environments. Front Sustain Food Syst. 2023;7. doi:10.3389/fsufs.2023.1242187. [Google Scholar] [CrossRef]
26. Toderich KT, Kravtsova K, Gasimova V, Alizade O, Yakovleva M, Ozturk M, et al. Seed heteromorphism and germination in Chenopodium quinoa Willd. related to crop introduction in marginalized environments. Pak J Bot. 2023;55(4):17. doi:10.30848/PJB2023-4(37). [Google Scholar] [CrossRef]
27. Manjarres-Hernández EH, Morillo-Coronado AC, Ojeda-Pérez ZZ, Cárdenas-Chaparro A, Arias-Moreno DM. Characterization of the yield components and selection of materials for breeding programs of quinoa (Chenopodium quinoa Willd.). Euphytica. 2021;217(6):101. doi:10.1007/s10681-021-02837-5. [Google Scholar] [CrossRef]
28. Bhargava A, Shukla S, Ohri D. Genetic variability and interrelationship among various morphological and quality traits in quinoa (Chenopodium quinoa Willd.). Field Crops Res. 2007;101(1):104–16. doi:10.1016/j.fcr.2006.10.001. [Google Scholar] [CrossRef]
29. Shah SS, Shi L, Li Z, Ren G, Zhou B, Qin P. Yield, agronomic and forage quality traits of different quinoa (Chenopodium quinoa Willd.) genotypes in Northeast China. Agronomy. 2020;10(12):1908. doi:10.3390/agronomy10121908. [Google Scholar] [CrossRef]
30. Granado-Rodríguez S, Vilariño-Rodríguez S, Maestro-Gaitán I, Matías J, Rodríguez MJ, Calvo P. Genotype-dependent variation of nutritional quality-related traits in quinoa seeds. Plants. 2021;10(10):2128. doi:10.3390/plants10102128. [Google Scholar] [PubMed] [CrossRef]
31. Coronado ACM, Castro MA, Hernandez EHM. Interpopulation characterization of quinoa (Chenopodium quinoa Willd.) from different agroecological environments of Colombia. Braz J Biol. 2023;83(3):806. doi:10.1590/1519-6984.271954. [Google Scholar] [PubMed] [CrossRef]
32. Laki ES, Rabiei B, Marashi H, Jokarfard V, Börner A. Association analysis of grain yield and yield components in Quinoa (Chenopodium quinoa Willd.) using SSR markers. Sci Rep. 2024;14:5991. doi:10.1038/s41598-024-56587-0. [Google Scholar] [PubMed] [CrossRef]
33. Tang P, Ren A, Jiang Z, Wang R, Cui K, Wu X, et al. Evaluation of quinoa varieties for adaptability and yield potential in low altitudes and correlation with agronomic traits. Agronomy. 2024;14(4):852. doi:10.3390/agronomy14040852. [Google Scholar] [CrossRef]
34. Craine EB, Davies A, Packer D, Miller ND, Schmöckel SM, Spalding EP, et al. A comprehensive characterization of agronomic and end-use quality phenotypes across a quinoa world core collection. Front Plant Sci. 2023;14:14. doi:10.3389/fpls.2023.1101547. [Google Scholar] [PubMed] [CrossRef]
35. Rojas W. Multivariate analysis of genetic diversity of Bolivian quinoa germplasm. Food Rev Int. 2003;19(1–2):9–23. doi:10.1081/fri-120018864. [Google Scholar] [CrossRef]
36. Bertero H, de La Vega A, Correa G, Jacobsen S, Mujica A. Genotype and genotype-by-environment interaction effects for grain yield and grain size of quinoa (Chenopodium quinoa Willd.) as revealed by pattern analysis of international multi-environment trials. Field Crops Res. 2004;89(2–3):299–318. doi:10.1016/j.fcr.2004.02.006. [Google Scholar] [CrossRef]
37. Manugade RP, Hamane GM, Bhalekar NB. Evaluation of different genotypes of quinoa (Chenopodium quinoa Willd.) for yield and yield contributing parameters. Int J Plant Soil Sci. 2023;35(19):783–7. doi:10.9734/ijpss/2023/v35i193611. [Google Scholar] [CrossRef]
38. Al-Naggar AMM, Abd El-Salam RM, Badran AEE, El-Moghazi Mai MA. Heritability and interrelationships for agronomic, physiological and yield traits of quinoa (Chenopodium quinoa Willd.) under elevated water stress. Arch Curr Res Int. 2017;10(3):1–15. doi:10.9734/ACRI/2017/37215. [Google Scholar] [CrossRef]
39. Dhanwani RK, Sarawgi AK, Solanki A, Tiwari JK. Genetic variability analysis for various yield attributing and quality traits in rice (O. sativa L.). Bioscan Nature Surv. 2013;8(1):1403–7. doi:10.3329/jbau.v15i1.33525. [Google Scholar] [CrossRef]
40. Islam M, Raffi S, Hossain M, Hasan A. Analysis of genetic variability, heritability and genetic advance for yield and yield associated traits in some promising advanced lines of rice. Progress Agric. 2015;26(1):26–31. doi:10.3329/pa.v26i1.24511. [Google Scholar] [CrossRef]
41. Wu G, Ross CF, Morris CF, Murphy KM. Sensory & food quality lexicon development, consumer acceptance, and drivers of liking of quinoa varieties. J Food Sci. 2017;82(4):993–1005. doi:10.1111/1750-3841.13677. [Google Scholar] [PubMed] [CrossRef]
42. Nowak V, Du J, Charrondière RJ. Assessment of the nutritional composition of quinoa (Chenopodium quinoa Willd.). Food Chem. 2016;193(2):47–54. doi:10.1016/j.foodchem.2015.02.111. [Google Scholar] [PubMed] [CrossRef]
43. de Bock P, van Bockstaele F, Muylle H, Quataert P, Vermeir P, Eeckhout M, et al. Yield and nutritional characterization of thirteen quinoa (Chenopodium quinoa Willd.) varieties grown in North-West Europe—part I. Plants. 2021;10. doi:10.3390/plants10122689. [Google Scholar] [PubMed] [CrossRef]
44. Repo-Carrasco-Valencia R, Hellstrom JK, Pihlava JM, Mattila PH. Flavonoids and other phenolic compounds in Andean indigenous grains: quinoa (Chenopodium quinoakiwicha (Amaranthus caudatus) and kañiwa (Chenopodium pallidicaule). Food Chem. 2010;120(1):128–33. doi:10.1016/j.foodchem.2009.09.087. [Google Scholar] [CrossRef]
45. Vega-Galvez A, Miranda M, Vergara J, Uribe E, Puente L, Martínez EA. Nutrition facts and functional potential of quinoa (Chenopodium quinoa Willd.). An ancient Andean grain: a review. J Sci Food Agric. 2010;9(15):2541–7. doi:10.1002/jsfa.4158. [Google Scholar] [PubMed] [CrossRef]
46. Pedrali D, Giupponi L, de la Pena-Armada R, Villanueva-Suarezb MJ, Mateos Aparicio I. The quinoa variety influences the nutritional and antioxidant profile rather than the geographic factors. Food Chem. 2023;402(1):133531. doi:10.1016/j.foodchem.2022.133531. [Google Scholar] [PubMed] [CrossRef]
47. Agarwal A, Rizwana Tripathi AD, Kumar T, Sharma KP, Patel SKS. Nutritional and functional new perspectives and potential health benefits of quinoa and chia seeds. Antioxid. 2023;12(7):1413. doi:10.3390/antiox12071413. [Google Scholar] [PubMed] [CrossRef]
48. Oustani M, Mehda S, Halilat MT, Chenchouni H. Yield, growth development and grain characteristics of seven quinoa (Chenopodium quinoa Willd.) genotypes grown in open-field production systems under hot-arid climatic conditions. Sci Rep. 2023;13(1):1–18. doi:10.1038/s41598-023-29039-4. [Google Scholar] [PubMed] [CrossRef]
49. Abugoch LE. Quinoa (Chenopodium quinoa Willd.composition, chemistry, nutritional and functional properties. Adv Food Nutr Res. 2009;58(1–2):1–31. doi:10.1016/S1043-4526(09)58001-1. [Google Scholar] [PubMed] [CrossRef]
50. Kozioł MJ. Chemical composition and nutritional evaluation of quinoa (Chenopodium quinoa Willd). J Food Compos Anal. 1992;5(1):35–68. doi:10.1016/0889-1575(92)90006-6. [Google Scholar] [CrossRef]
51. Singh B, Kaur A. Control of insect pests in crop plants and stored food grains using plant saponins: a review. J Food Sci Technol. 2018;87(1):93–101. doi:10.1016/j.lwt.2017.08.077. [Google Scholar] [CrossRef]
52. Suárez-Estrella D, Torri L, Pagani MA, Marti A. Quinoa bitterness: causes and solutions for improving product acceptability. J Sci Food Agric. 2018;98(11):4033–41. doi:10.1002/jsfa.8980. [Google Scholar] [PubMed] [CrossRef]
53. Miranda M, Vega-Gálvez A, Quispe-Fuentes I, Rodríguez MJ, Maureira H, Martínez EA. Nutritional aspects of six quinoa (Chenopodium quinoa Willd.) ecotypes from three geographical areas of Chile. Chil J Agric Res. 2012;72(2):175–81. doi:10.4067/S0718-58392012000200002. [Google Scholar] [CrossRef]
54. Diaz-Valencia YK, Alca JJ, Calori-Domingues MA, Zanabria-Galvez SJ, da Cruz SH. Nutritional composition, total phenolic compounds and antioxidant activity of quinoa (Chenopodium quinoa Willd.) of different colours. Nova Biotechnol Et Chim. 2018;17(1):74–85. doi:10.2478/nbec-2018-0008. [Google Scholar] [CrossRef]
55. El Hazzam K, Hafsa J, Sobeh M, Mhada M, Taourirte M, El Kacimi K, et al. An insight into saponins from quinoa (Chenopodium quinoa Willda review. Molecules. 2020;25(5):1059. doi:10.3390/molecules25051059. [Google Scholar] [PubMed] [CrossRef]
Appendix A
Cite This Article
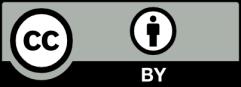
This work is licensed under a Creative Commons Attribution 4.0 International License , which permits unrestricted use, distribution, and reproduction in any medium, provided the original work is properly cited.