Open Access
ARTICLE
Metabolic Characteristics of Taste Differences of Sweet Potato Leaves Grown in Soil and Hydroponic Cultures by Using Non-Targeted Metabolomics
Institute of Crop Sciences, Fujian Academy of Agricultural Sciences, Scientific Observing and Experimental Station of Tuber and Root Crops in South China, Ministry of Agriculture and Rural Affairs, Fuzhou, 350013, China
* Corresponding Author: Sixin Qiu. Email:
Phyton-International Journal of Experimental Botany 2024, 93(12), 3401-3410. https://doi.org/10.32604/phyton.2024.058692
Received 19 September 2024; Accepted 25 November 2024; Issue published 31 December 2024
Abstract
Sweet potato leaves are consumed globally for their nutritional and functional properties, with their taste characteristics significantly influencing their market value and consumer acceptance. However, the metabolic factors determining the taste of sweet potato leaves remain unclear. This study evaluated the taste and metabolic profile of sweet potato leaves cultivated in soil and hydroponic systems, revealing that hydroponic culture improved the taste of the leafy sweet potatoes. Using gas chromatography system (GC)-ToF-MS, 200 metabolites were identified, encompassing most plant metabolic pathways. A comparison of good-tasting vs. poor-tasting sweet potato leaves identified 71 metabolites associated with taste quality formation. Poor-tasting leaves exhibited lower levels of amino acid metabolites and higher levels of carbohydrate and secondary metabolites. This research provides novel insights into enhancing the taste of leafy sweet potatoes.Keywords
Supplementary Material
Supplementary Material FileSweet potato (Ipomoea batatas (Lam.) L.) is a globally significant food crop. Its roots serve as both a food source and raw material for industrial food production, while its leaves and young shoots are consumed as leafy vegetables in numerous tropical and subtropical regions [1]. Leafy sweet potatoes possess excellent taste qualities without bitterness after cooking. Additionally, they exhibit rapid growth and regeneration, allowing for multiple harvests annually with high and consistent yields [2]. Notably, their nutritional and functional values surpass those of the root or other leafy vegetables [3–5]. Furthermore, leafy sweet potatoes demonstrate robust resistance to heat, drought, and water-logging stress [6].
Taste is a crucial quality attribute that significantly influences commodity value and consumer acceptance [7,8]. Research has demonstrated that hydroponic cultivation enhances the vitamin C, flavone, and nitrate content, as well as the yield of sweet potato leaves compared to traditional soil cultivation [9]. In previous studies, hydroponically grown strawberries were found to be preferred over soil-grown strawberries by the majority of participants in sensory evaluations [10]. While hydroponic cultivation can also enhance the taste of leafy sweet potatoes, the underlying metabolic composition and mechanisms responsible for this improved taste quality remain unclear.
Metabolomics is an advanced analytical method characterized by high-throughput, high-resolution, and high-sensitivity capabilities, enabling comprehensive examination of endogenous metabolites in living organisms [11]. This approach has provided valuable insights into various aspects of food science, including taste changes in tomato breeding history [12], sensory quality assessment of garlic [13], exploration of relationships between chemical compounds and white tea flavor [14], and identification of key taste components in loquat [15]. Given its proven utility, this study employs an untargeted metabolomic analysis to investigate the metabolic basis for taste differences in sweet potato leaves cultivated in soil and hydroponic systems.
2.1 Plant Growth and Sample Collection
The experiments were conducted at the Pudang Agricultural Experiment Farm (26°07′59″ N, 119°20′06″ E), part of the Fujian Academy of Agricultural Sciences, in Fuzhou City, China. Eight sweet potato cultivars were utilized in this study: Fucaishu18, Baisheng, Fushu7-6, Tainong71, Fushu24, Guangshu87, Jinshan57, and Fushu604. These cultivars were sourced from the Germplasm Nursery for sweet potato of Fujian Province. The plants were cultivated in both soil and hydroponic cultures within a plastic house, as described below:
Hydroponic culture (H) involved placing eight plants in a container (40 cm × 28 cm × 15 cm) filled with half-strength Hoagland solution and micronutrients [16]. Air was pumped into the nutrient solution for 5 min every 2 h. Three containers were used for each cultivar. Throughout the growth period, the nutrient solution was refreshed every 6 days.
Soil culture (S)—Five plants were positioned in a plastic pot (15 cm in height and 12 cm in diameter) containing 1.5 kg of clay soil with 0.83 g kg−1 total N, 10.72 mg kg−1 available P, and 69.15 mg kg−1 exchangeable K. Prior to planting, basal fertilization was applied at rates of 0.20 g N, 0.24 g P2O5, and 0.9 g K2O per pot. Five pots were allocated for each cultivar. To maintain appropriate soil moisture, the pots were irrigated every 3 days.
Thirty days after planting, the youngest fully expanded leaves were harvested from the plants, immediately frozen in liquid nitrogen, and stored at –80°C until analysis. Each sample consisted of six biological replicates.
Ten trained panelists evaluated the leafy sweet potatoes using modified methods derived from Ona et al. [7] and Ishiguro et al. [17]. The sweet potato leaf samples were cooked in boiling water for 2 min, then immediately assessed for flavor, sweetness, bitterness, and crispiness. The panelists used Fuchaishu18 leaves grown in soil as the standard, assigning it a score of 6. Other samples were scored on a scale from 0 to 9 relative to this standard.
2.3.1 Metabolites Extraction and Gas Chromatography System-Mass Spectrometry (GC-MS) Analysis
Metabolite profiling was conducted by Shanghai Biotree Biotech Co., Ltd. in China, following the methodology described by Wu et al. [18]. In brief, 60 mg of sample was placed in 2 mL EP tubes and extracted with 0.48 mL of extraction liquid (VMethanol:VH2O = 3:1), incorporating 20 μL of adonitol (0.5 mg/mL stock in dH2O) as the internal standard. The mixture underwent vortexing for 30 s, homogenization in a ball mill for 4 min at 45 Hz, and sonication for 5 min in ice water before centrifugation for 15 min at 16,260 g. Subsequently, 0.35 mL of the supernatant was transferred to a fresh 2 mL GC/MS glass vial, and a 40-μL aliquot was extracted from each sample and pooled as a quality control (QC) sample. The supernatant was then dried in a vacuum concentrator without heating. Following this, 80 μL of methoxyamination hydrochloride (20 mg/mL in pyridine) was added and incubated for 30 min at 80°C, after which 100 μL of BSTFA reagent (1% TMCS, v/v) was introduced and incubated for 1.5 h at 70°C. FAMEs (10 μL of a standard mixture of fatty acid methyl esters, 1 mg/mL of C8–C16, and 0.5 mg/mL of C18–C24 in chloroform) were added to the QC sample and cooled to room temperature. All samples were analyzed using a gas chromatography system (GC, Agilent 7890B, Agilent Technologies, Santa Clara, CA, USA) coupled with a time-of-flight mass spectrometer (TOF-MS, Pegasus 4D, LECO Corporation, St. Joseph, MI, USA).
2.3.2 Data Analysis and Annotation
The Chroma TOF 4.3X software and LECO-Fiehn Rtx5 database (LECO Corporation) were utilized for raw peak extraction, data baseline filtering and calibration, peak alignment, deconvolution analysis, peak identification, and peak area integration [19]. Both mass spectrum and retention index matches were considered for metabolite identification. Peaks detected in <50% of QC samples or with RSD >30% in QC samples were eliminated [20]. The internal standard normalization method was applied in the data analysis. The resulting three-dimensional data, comprising peak number, sample name, and normalized peak area, were input into the SIMCA software package (V14.1, MKS Data Analytics Solutions, Umea, Sweden) for principal component analysis (PCA) using the eigenvalue decomposition algorithm and orthogonal projections to latent structures-discriminate analysis (OPLS-DA). Metabolite pathways were identified using public databases, including Kyoto Encyclopedia of Genes and Genomes (KEGG, http://www.genome.jp/kegg/, accessed on 06 January 2021) and MetaboAnalyst (http://www.metaboanalyst.ca/, accessed on 06 January 2021). The heatmap was generated using TBtools-II software (v2.136) with the hierarchical cluster method.
3.1 Taste Differences of Sweet Potato Leaves Grown in Different Cultural Conditions
Leafy sweet potato cultivation traditionally relies on conventional land cultivation methods; however, hydroponic culture has been employed to enhance growth and yield [9]. Hydroponic systems also facilitate pollution-free, standardized, and mechanized production of leafy sweet potatoes. A preliminary study compared the taste characteristics of leaves from eight sweet potato varieties cultivated under hydroponic (H) and soil culture (S) conditions. The results indicated that hydroponic culture improved the taste of sweet potato leaves across all cultivars, with particularly notable enhancements in cultivars that exhibited poor taste under soil culture (Table S1). Among these eight cultivars, the Baisheng variety demonstrated the most significant taste improvement under hydroponic conditions, approaching that of Fucaishu18; Meanwhile The taste quality of Fucaishu18 exhibits minimal variation under both hydroponic and soil cultivation conditions (Fig. 1). Consequently, the Fucaishu18 and Baisheng varieties were selected for metabolomic analysis to identify taste-related metabolites.
Figure 1: Taste values of leaves in Baisheng and Fucaishu18 under different growing conditions
3.2 Metabolite Profiling of Sweet Potato Leaves
The sweet potato leaves cultivated in soil and hydroponic environments underwent GC-TOF-MS analysis, resulting in the detection of 518 peaks. Of these, 200 metabolites were identified through the LECO-Fiehn Rtx5 database (Fig. 2A). To ensure data reliability, five QC samples were employed, demonstrating high repeatability (Figs. S1 and S2) and concordance (Fig. S3). KEGG analysis categorized these metabolites into seven super pathways (Fig. 2B) and 30 sub-pathways (Table S2). The pathways primarily encompassed central and partial secondary metabolism, including 45 amino acids, 94 carbohydrates, 28 lipids, 5 CPGECs (cofactors, prosthetic groups, electron carriers), 5 nucleotides, 22 secondary metabolites, and 1 phytohormone.
Figure 2: GC-TOF-MS analysis and classification of the identified metabolites in sweet potato leaves. (A) Total ions chromatograph (TIC) of four samples with six replicates in sweet potato leaves via GC-TOF-MS. Green and blue graphs represent the samples of Fucaishu18-S (soil culture) and Fucaishu18-H (hydroponic culture), respectively. Red and black graphs represent the samples of Baisheng variety grown in soil (Baisheng-S) (soil culture) and Baisheng-H (hydroponic culture), respectively. (B) Distribution of 200 identified metabolites depicted in a pie chart; sub-classifications of amino acids and carbohydrates are illustrated with histograms
A non-supervised PCA analysis was conducted using all 200 metabolites. Fig. 3 illustrates that the metabolome of the Baisheng variety cultivated in soil (Baisheng-S, poor taste) was distinct from the other three samples. An OPLS-DA analysis generated a loading plot, further demonstrating significant differences between the two growing patterns for each cultivar (Fig. S4). The heat map also revealed a distinct cluster pattern in the relative abundance of Baisheng-S (Fig. 4), which aligns with the observed taste differences between soil and hydroponic cultures (Fig. 1).
Figure 3: Score scatter plot of PCA model for metabolites (peaks) in leaves of Fucaishu18 and Baisheng under different growing patterns. H, hydroponic culture; S, soil culture; QC, quality control
Figure 4: Heatmap visualization of the variation in 200 metabolites in leaves of Baisheng and Fucaishu18 under different cultivation conditions. H, hydroponic culture; S, soil culture. The abundance of each metabolite across four samples was normalized using Z-score analysis. The biochemical names corresponding to each metabolite ID are presented in Table S2
3.3 Metabolites and Their Pathway Related to the Taste of Sweet Potato Leaves
3.3.1 Reduced Amino Acid Abundance in Baisheng-S
Amino acids and their derivatives play a crucial role in imparting flavor to food [21,22]. In this study, eighteen metabolites associated with amino acid metabolism were identified. With the exception of N, N-dimethylarginine (ADMA), 3-cyanoalanine, N-amidino-L-aspartate, and cycloleucine, the Baisheng-S variety, which was characterized by poor taste, exhibited lower concentrations of these amino acids and their derivatives. This was particularly evident in the case of four umami amino acids: Asp, Glu, Gly, and Ala (Fig. 5, Table S2, Fig. S5).
Figure 5: Metabolite abundances in leaves of Baisheng and Fucaishu18 under different growing conditions. The four squares beneath each metabolite name represent abundance changes in Baisheng-Soil culture (S), Baisheng-Hydroponic culture (H), Fuchaishu18-Soil culture (S), and Fuchaishu18-Hydroponic culture (H), respectively. Metabolite abundances of the four samples were normalized using Z-score analysis. Red squares indicate high abundance, while blue squares denote low abundance. Table S2 for detailed information of the changes of these metabolites
3.3.2 Enhanced Lipids Synthesis, but Not for Ester Synthesis
Plant lipids are essential components of cell membranes, serving as signal molecules and stored energy reserves. This investigation identified ten metabolites associated with lipid metabolism, including those derived from acetyl-CoA and 3-PGA. Baisheng-S exhibited an increased abundance of seven of these lipids while demonstrating a lower abundance of stearate, arachidate, and palmitoleate (Fig. 5, Table S2).
3.3.3 Increased Secondary Metabolism and Secondary Metabolites Accumulation
Plant secondary metabolites, also known as natural products or specialized metabolites, represent a diverse array of chemical compounds that contribute significantly to flavor profiles and bioactive properties beneficial for human health. In the Baisheng-S variety, the analysis revealed variations in the content of 13 secondary metabolites, with 9 exhibiting increased levels. Notably, the shikimate metabolic pathway, which is crucial for secondary metabolism, demonstrated enhanced activity (Fig. 5, Table S2, Fig. S5). This enhancement suggests an accumulation of secondary metabolites, potentially contributing to the less desirable taste characteristics observed.
Metabolomics has emerged as a powerful analytical tool for investigating the quality traits of crops [12,13,15]. This approach has been successfully employed to examine various aspects of plant biology, including anthocyanin accumulation in tuberous roots, metabolic mechanisms underlying flesh color differences, metabolic changes during postharvest storage, and metabolic diversity in leaves and roots [23–26]. In the present study, GC–ToF–MS-based untargeted metabolomics was utilized to analyze the taste differences between sweet potato leaves cultivated in soil and hydroponic systems. The analysis identified a total of 200 metabolites, with 71 of these compounds associated with flavor formation in sweet potato leaves. These findings provide a comprehensive metabolic reference for understanding the taste profile of leafy sweet potatoes.
Metabolites are crucial components of plants and can be categorized into carbohydrates, organic and amino acids, vitamins, hormones, flavonoids, phenolics, and glucosinolates [27,28]. Certain metabolites significantly influence the taste profile of leafy vegetables. For instance, glycine and alanine contribute sweetness, valine, tryptophan, and leucine impart bitterness, while aspartic acid and glutamate provide a sour taste [27–30]. Secondary metabolites, including flavonoids, phenolics, and alkaloids, are primarily responsible for bitterness and astringency [28,31,32]. Our findings align with previous research, suggesting that the less desirable taste of sweet potato leaves cultivated in soil is attributed to lower concentrations of amino acid metabolites and higher levels of carbohydrates and secondary metabolites. Future investigations should focus on the dynamic metabolic characteristics of amino acids and secondary metabolites to enhance the flavor profile of leafy sweet potatoes.
Acknowledgement: Not applicable.
Funding Statement: This research was funded by the Natural Science Foundation of China (32101841), the Natural Science Foundation of Fujian Province of China (2021J01493), China Agriculture Research System of MOF and MARA (CARS-10-B14), the Fujian Provincial Public Research Institute of Fundamental Research (2017R1026-5), and the Fujian Academy of Agricultural Sciences Research Project (AGP2018-12, GJYS202201).
Author Contributions: The authors confirm contribution to the paper as follows: study conception and design: Sixin Qiu and Zhaomiao Lin; data collection: Guoliang Li, Hong Zhang, Yongqing Xu, Guochun Xu, Huawei Li and Zhonghua Liu; analysis and interpretation of results: Zhaomiao Lin, Rongchang Ji, Wenbin Luo and Yongxiang Qiu; draft manuscript preparation: Zhaomiao Lin; writing—review and editing: Sixin Qiu and Hao Tang. All authors reviewed the results and approved the final version of the manuscript.
Availability of Data and Materials: The data that support the findings of this study are available from the corresponding author, Sinxi Qiu, upon reasonable request.
Ethics Approval: This article does not contain any studies with animals or humans performed by any of the authors.
Conflicts of Interest: The authors declare no conflicts of interest to report regarding the present study.
Supplementary Materials: The supplementary material is available online at https://doi.org/10.32604/phyton.2024.058692.
References
1. Islam S. Sweetpotato (Ipomoea batatas L.) leaf: its potential effect on human health and nutrition. J Food Sci. 2006;71:R13–21. [Google Scholar]
2. Hong CY, Jo YJ, Kim MY, Chung MN, Choi EK, Kim YB, et al. Biological activities of sweet potato (Ipomoea batatas L.) tips and tubers. Food Sci Nutr. 2022;10(11):4041–8. doi:10.1002/fsn3.v10.11. [Google Scholar] [CrossRef]
3. Tan W, Guo X, Wang Z, Zhang R, Tang C, Jiang B, et al. Metabolic profiles and morphological characteristics of leaf tips among different sweet potato (Ipomoea batatas Lam.) varieties. J Integr Agr. 2024;23(2):494–510. doi:10.1016/j.jia.2023.04.029. [Google Scholar] [CrossRef]
4. Luo D, Mu T, Sun H. Profiling of phenolic acids and flavonoids in sweet potato (Ipomoea batatas L.) leaves and evaluation of their anti-oxidant and hypoglycemic activities. Food Biosci. 2021;39:100801. doi:10.1016/j.fbio.2020.100801. [Google Scholar] [CrossRef]
5. Li G, Lin Z, Zhang H, Liu Z, Xu Y, Xu G, et al. Anthocyanin accumulation in the leaves of the purple sweet potato (Ipomoea batatas L.) cultivars. Molecules. 2019;24(20):3743. doi:10.3390/molecules24203743. [Google Scholar] [PubMed] [CrossRef]
6. Tang CC, Ameen A, Fang BP, Liao MH, Chen JY, Huang LF, et al. Nutritional composition and health benefits of leaf-vegetable sweet potato in South China. J Food Compos Anal. 2021;96:103714. doi:10.1016/j.jfca.2020.103714. [Google Scholar] [CrossRef]
7. Ona CL, Chujoy E. Screening of sweet potato for leaf tips as vegetable (Working Papers). Manila (PhilippinesSoutheast Asia and the Pacific Regional Center of International Potato Center; 1991. p. 194–204. [Google Scholar]
8. Lee JS, Ahn YS, Chung MN, Kim HS, Jeong BC, Bang JK. Varieties for sweetpotato tips production as a vegetable. Korean J Breeding Sci. 2007;39:224–31. [Google Scholar]
9. Chen XY, Zhang ZJ, Zheng JW, Lin YL. Effects of hydroponics on nutrient components and nitrate contents in tips of leaf vegetable sweet potato. Sci Agric Sin. 2013;46(17):3736–42 (In Chinese). [Google Scholar]
10. Treftz C, Zhang F, Omaye ST. Comparison between hydroponic and soil-grown strawberries: sensory attributes and correlations with nutrient content. Food Nutr Sci. 2015;6(15):1371–80. doi:10.4236/fns.2015.615143. [Google Scholar] [CrossRef]
11. van Der Hooft JJ, Mohimani H, Bauermeister A, Dorrestein PC, Duncan KR, Medema MH. Linking genomics and metabolomics to chart specialized metabolic diversity. Chem Soc Rev. 2020;49(11):3297–314. doi:10.1039/D0CS00162G. [Google Scholar] [PubMed] [CrossRef]
12. Zhu G, Wang S, Huang Z, Zhang S, Liao Q, Zhang C, et al. Rewiring of the fruit metabolome in tomato breeding. Cell. 2018;172(1–2):249–61. doi:10.1016/j.cell.2017.12.019. [Google Scholar] [PubMed] [CrossRef]
13. Liu J, Liu LX, Guo W, Fu ML, Yang ML, Huang SX, et al. A new methodology for sensory quality assessment of garlic based on metabolomics and an artificial neural network. RSC Adv. 2019;9(31):17754–65. doi:10.1039/C9RA01978B. [Google Scholar] [PubMed] [CrossRef]
14. Yang C, Hu Z, Lu M, Li P, Tan J, Chen M, et al. Application of metabolomics profiling in the analysis of metabolites and taste quality in different subtypes of white tea. Food Res Int. 2018;106:909–19. doi:10.1016/j.foodres.2018.01.069. [Google Scholar] [PubMed] [CrossRef]
15. Zou S, Wu J, Shahid MQ, He Y, Lin S, Liu Z, et al. Identification of key taste components in loquat using widely targeted metabolomics. Food Chem. 2020;323:126822. doi:10.1016/j.foodchem.2020.126822. [Google Scholar] [PubMed] [CrossRef]
16. Hoagland DR, Arnon DI. The water-culture method for growing plants without soil. Circ Calif Agric Exp Stn. 1950;347:32. [Google Scholar]
17. Ishiguro K, Toyama J, Shahidul Islam MD, Yoshimoto M, Kumagai T, Kai Y, et al. Suioh, a new sweetpotato cultivar for utilization in vegetable greens. Acta Hortic. 2004;637:339–45. doi:10.17660/ActaHortic.2004.637.42. [Google Scholar] [CrossRef]
18. Wu L, Gao X, Xia F, Joshi J, Borza T, Wang-Pruski G. Biostimulant and fungicidal effects of phosphite assessed by GC-TOF-MS analysis of potato leaf metabolome. Physiol Mol Plant Pathol. 2019;106:49–56. doi:10.1016/j.pmpp.2018.12.001. [Google Scholar] [CrossRef]
19. Kind T, Wohlgemuth G, Lee DY, Lu Y, Palazoglu M, Shahbaz S, et al. FiehnLib: mass spectral and retention index libraries for metabolomics based on quadrupole and time-of-flight gas chromatography/mass spectrometry. Anal Chem. 2009;81:10038–48. doi:10.1021/ac9019522. [Google Scholar] [PubMed] [CrossRef]
20. Dunn WB, Broadhurst D, Begley P, Zelena E, Francis-McIntyre S, Anderson N, et al. Procedures for large-scale metabolic profiling of serum and plasma using gas chromatography and liquid chromatography coupled to mass spectrometry. Nat Protoc. 2011;6:1060–83. doi:10.1038/nprot.2011.335. [Google Scholar] [PubMed] [CrossRef]
21. Huang Z, Feng Y, Zeng J, Zhao M. Six categories of amino acid derivatives with potential taste contributions: a review of studies on soy sauce. Crit Rev Food Sci Nutr. 2024;64(22):7981–92. doi:10.1080/10408398.2023.2194422. [Google Scholar] [PubMed] [CrossRef]
22. Lindsay RC. Flavors. In: Damodaran S, Parkin KL, editors. Fennemas food chemistry. 5th ed Boca Raton: CRC Press; 2017. p. 753–802. [Google Scholar]
23. Wang A, Li R, Ren L, Gao X, Zhang Y, Ma Z, et al. A comparative metabolomics study of flavonoids in sweet potato with different flesh colors (Ipomoea batatas (L.) Lam). Food Chem. 2018;260:124–34. doi:10.1016/j.foodchem.2018.03.125. [Google Scholar] [PubMed] [CrossRef]
24. Ren L, Zhang T, Wu H, Ge Y, Zhao X, Shen X, et al. Exploring the metabolic changes in sweet potato during postharvest storage using a widely targeted metabolomics approach. J Food Process Pres. 2021;45:e15118. [Google Scholar]
25. He L, Liu X, Liu S, Zhang J, Zhang Y, Sun Y, et al. Transcriptomic and targeted metabolomic analysis identifies genes and metabolites involved in anthocyanin accumulation in tuberous roots of sweetpotato (Ipomoea batatas L.). Plant Physiol Biochem. 2020;156:323–32. doi:10.1016/j.plaphy.2020.09.021. [Google Scholar] [PubMed] [CrossRef]
26. Drapal M, Rossel G, Heider B, Fraser PD. Metabolic diversity in sweet potato (Ipomoea batatas, Lam.) leaves and storage roots. Hortic Res. 2019;6:2. doi:10.1038/s41438-018-0075-5. [Google Scholar] [PubMed] [CrossRef]
27. Fang C, Luo J, Wang S. The diversity of nutritional metabolites: origin, dissection, and application in crop breeding. Front Plant Sci. 2019;10:1028. doi:10.3389/fpls.2019.01028. [Google Scholar] [PubMed] [CrossRef]
28. Mabuchi R, Tanaka M, Nakanishi C, Takatani N, Tanimoto S. Analysis of primary metabolites in cabbage (Brassica oleracea var. capitata) varieties correlated with antioxidant activity and taste attributes by metabolic profiling. Molecules. 2019;24:4282. doi:10.3390/molecules24234282. [Google Scholar] [PubMed] [CrossRef]
29. Rhyu M-R, Lyall V. Interaction of taste-active nutrients with taste receptors. Curr Opin Physiol. 2021;20:64–9. doi:10.1016/j.cophys.2020.12.008. [Google Scholar] [CrossRef]
30. Zhao CJ, Schieber A, Gänzle MG. Formation of taste-active amino acids, amino acid derivatives and peptides in food fermentations—a review. Food Res Int. 2016;89:39–47. doi:10.1016/j.foodres.2016.08.042. [Google Scholar] [PubMed] [CrossRef]
31. Ye JH, Ye Y, Yin JF, Jin J, Liang YR, Liu Y, et al. Bitterness and astringency of tea leaves and products: formation mechanism and reducing strategies. Trends in Food Sci Tech. 2022;123:130–43. doi:10.1016/j.tifs.2022.02.031. [Google Scholar] [CrossRef]
32. Streit NM, Hecktheuer LHR, do Canto MW, Mallmann CA, Streck L, Parodi TV, et al. Relation among taste-related compounds (phenolics and caffeine) and sensory profile of erva-mate (Ilex paraguariensis). Food Chem. 2007;102:560–4. doi:10.1016/j.foodchem.2006.05.028. [Google Scholar] [CrossRef]
Cite This Article
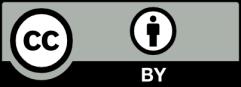
This work is licensed under a Creative Commons Attribution 4.0 International License , which permits unrestricted use, distribution, and reproduction in any medium, provided the original work is properly cited.