Open Access
ARTICLE
Effect of Shading on Nodule Growth at Seedling Stage in Relay Strip Intercropping System
1 Soybean Research Institute, Nanchong Academy of Agricultural Sciences, Nanchong, 637000, China
2 College of Agronomy, Sichuan Agricultural University, Chengdu, 625014, China
3 Sweetpotato and Leguminosae Germplasm Innovation and Utilization Key Laboratory of Sichuan Province, Nanchong, 637000, China
* Corresponding Author: Wenyu Yang. Email:
(This article belongs to the Special Issue: Abiotic Stress Tolerance in Crop Plants: Physio-biochemical and Molecular Mechanisms)
Phyton-International Journal of Experimental Botany 2024, 93(12), 3387-3399. https://doi.org/10.32604/phyton.2024.058494
Received 13 September 2024; Accepted 22 November 2024; Issue published 31 December 2024
Abstract
Relay strip intercropping (RSI) increases soybean nodule number and nitrogen fixation activity at the reproductive stage more than monocropping (M), but the effect of changes in the environment, especially light, on nodules during the coexistence duration and vegetative stage, is unclear. To determine the impact of shading on nodule development at the seedling stage, nodule traits, distribution, and physiological function were compared between M and RSI in a potting experiment in a field environment. Compared with M, nodule number and weight decreased significantly (an average of 81.77% and 93.16%, respectively); thus, the exponential relationship between them changed because of the smaller nodule in RSI. Nodules were clustered on the tap roots, but there was no effect on the distribution of nodule weight on the tap or lateral root. The nodule density of the tap root notably decreased by an average of 56.00%, whereas that of the lateral root showed no difference. Dry weight-specific nodulation (DW-specific), and the ratio of nodule weight to root or whole plant weight decreased (61.33%, 85.46%, and 88.50%, respectively). Nodule leghemoglobin and sucrose content decreased in RSI by 94.29% and 95.17% at the plant level, and nodule nitrogenase activity was suppressed, especially at the plant level. The nodule growth and nitrogen fixation ability of ND showed the strongest adaptability to shading among the varieties.Keywords
Abbreviations
RSI | Relay strip intercropping |
M | Monocropping |
DW-specific | Dry weight specific nodulation |
NTS | Soybean variety nts 1007 |
GX | Soybean variety Guixia3 |
ND | Soybean variety Nandou12 |
P | Planting system, RSI or M |
V | Variety |
RDW | Root dry weight |
SDW | Shoot dry weight |
R/S | Root/shoot value |
N/R | Nodule/root value |
N/W | Nodule/whole plant value |
NAP | Nitrogenase activity per unit |
NAW | Nitrogenase activity whole plant |
NN | Nodule number per plant |
NW | Nodule weight per plant |
PNW | Per nodule weight |
RW | Root weight |
SW | Shoot weight |
LCU | Leghemoglobin content per unit |
SCU | Sucrose content per unit |
With an increasing population and decreasing arable land, increasing the multiple crop index of land is key for the development of grain production [1]. As a widely recognized cropping system, intercropping can obtain a higher output because of the more efficient use of resources and the inhibition of diseases, weeds, and pests [2−4]. Legumes, especially soybeans, play an important role in intercropping and relay cropping systems because of their ability to fix nitrogen [5]. Like other legumes, soybean develops specialized root organs known as nodules, in which host cells establish a symbiotic association with rhizobia. With the differentiated bacteria, nodules reduce atmospheric nitrogen to ammonia and convert it into amides or ureides, which are then exported to the shoots [6]. As the site of nitrogen fixation, nodules are strongly correlated with the amount of N2 symbiotically fixed, nodule number, and nodule biomass [7].
The soybean-nodule association involves extremely complex regulation. Although nodule structures enable the fixation of atmospheric nitrogen, they can be energetically costly to develop while preserving the normal growth of host leguminous plants because the nitrogen fixation process requires a significant amount of photosynthates [8]. In the process of autoregulation of nodulation (AON), which involves long-distance signaling between the root and shoot, the number of nodules is regulated such that nodule overproduction on immature root tissues is prevented by prior nodulation events [9]. Leaves are the most likely source of shoot-derived signals, and several phytohormones have been suggested as candidates for autoregulation signals [10]. Simultaneously, nodules are strong sinks for assimilate partitioning at the plant level [11]. Nodule formation and development interact with root and shoot growth because of competition for photosynthetic products, particularly during the early stages [12]. Moreover, nodules are sensitive to adverse environmental conditions such as drought, waterlogging, etc. [13,14].
Maize-soybean relay strip intercropping has been widely applied to increase the resource use efficiency and grain production per unit land area in China, and it enabled to maintenance of maize yield, while at the same time harvesting an extra soybean crop, about 1.8 tons per hectare [15,16]. Maize is sown in a narrow-wide row pattern, and soybean is sown in a wide row between maize, with a 50–60 days overlap between the sowing of soybean and the harvesting of maize [17]. During this stage, the soybean is shaded by maize and the light environment of the soybean changes due to the selective absorption of maize leaves. Changes in light quantity and quality influence plant morphogenesis, assimilate partitioning, endogenous hormones, and other factors [18]. Thus, nodule development in soybeans is affected by changes in the plant status, which is an important sink of photosynthetic products.
Some reports have confirmed nitrogen transfer from soybean or other legumes to non-legume crops in intercropping systems [19], while others have shown that competition for light has a negative effect on N2-fixation and nodule growth by decreasing plant growth [20]. Previous studies on nodules in different light environments have focused on nodule reductive ability [21,22]; however, there is little information available about the effect of shading on nodule characteristics at the seedling stage. The objective of this study was to investigate the effect of shading on nodule traits, distribution, nitrogen-fixing ability, and their relationships.
2.1 Materials and Environmental Treatments
The experiments were conducted at the farm of Sichuan Agricultural University in Ya’an (29°98′N, 102°99′E, 536 m altitude), Sichuan Province, China. The climate of the experimental site was subtropical and humid. The maize variety was Zhenghong311, a major variety in southwestern, China, sown with a 40 + 160 cm wide-narrow row spacing on 28 March 2020. Three soybean varieties were selected: nts1007 (NTS, hypernodulating mutants from The University of Queensland, Brisbane, QLD, Austrilia), Guixia3 (GX, shade-sensitive local cultivated variety from Guangxi Academy Agricultural Sciences, Nanning, China), and Nandou12 (ND, shade-tolerant leading cultivated variety from Nanchong Academy Agricultural Sciences, Nanchong, China). To avoid mutual interference between soybean and maize roots, eight-ten soybean seeds were sown in plastic pots (35 cm in diameter, 50 cm in height) on 17 June 2020, and thinned to three plants approximately three weeks after sowing. Each pot contained approximately 23 kg of soil with fertilizer consisting of N = 0.602 g, P2O5 = 1.172 g, and K2O = 0.984 g. Half of each soybean variety was placed as pair rows between two wide rows of maize, considered as RSI, and the distance between the centers of the parallel pots was 50 cm. The height of the maize was approximately 2.5 m at the time of soybean sowing. The other half of the pots were exposed to full sunlight, positioned similarly to the RSI pots but without maize, considered as M. Every pot was inoculated with Bradyrhizobium japonicum 3 days after sowing at a concentration of approximately 1 × 109 cells mL−1. Three replicates were used in this study.
2.2 Harvesting and Measurements
For root characteristics and nodule traits, three pots (nine plants) per replicate were tagged for harvesting at the V5 stage. Shoots were cut off at the soil surface, and the soil containing the roots was poured into a nylon bag. The bag was soaked in water to facilitate rinsing the soil. Muddy water was filtered through a filter sieve, and the scattered roots and nodules were collected. The other nodules were detached from the washed roots using forceps as soon as they could be physically separated from the roots without causing damage. This process was conducted in an ice-water mixture. Roots without nodules were scanned using Epsontwain Pro and analyzed with WinRHIO (32-bit, Canada, Regent Instrument-INC), and all detached and scattered nodules from the roots were counted and then preserved at −80°C. The plants were divided into several parts (shoots, roots, and nodules) and weighed separately after drying at 80°C for 48 h.
Photosynthetically active radiation and R: FR ratio
The flux densities of PAR were measured using LI-191SA quantum sensors (LI-COR Inc., Lincoln, NE, USA) with a LI-1400 data logger 10 cm above the soybean canopy. Spectral irradiance at different wavelengths was measured using a fiber-optic spectrometer (AvaSpec-2048; Avantes, Netherlands).
Acetylene reduction activity
A 1 g sample of nodules was placed in a 10 mL glass bottle, and 20% of air was replaced by acetylene. After incubation at 30°C for 30 min, the amount of ethylene produced by the nitrogenase activity was monitored by gas chromatography. Condition: pillar, 60°C; injector, 120°C; FID, 120°C; airspeed: N2, 50 mL min−1; H2, 60 mL min−1; air, 50 L min−1.
Results were analyzed by two-way ANOVA and means were compared using the LSD test at p < 0.05 and p < 0.01, using the SPSS 24 statistical software (SPSS, Chicago, IL, USA).
From 6 am to 6 pm, the tendency of photosynthetically active radiation (PAR) first increased and then decreased, and the R:FR ratio decreased significantly (Fig. 1). Compared with M, PAR decreased by 50.5% in RSI, and R:FR ratio decreased by 0.584, from 1.195. This indicates that the incident solar radiations absorbed and reflected by maize upper leaves decreased the amount of available PAR and the R:FR ratio for soybean canopy at the seedling stage.
Figure 1: Light environment of soybean plants in M and RSI
Note: A, PAR (μmol m−2s−1); B, R: FR ratio. Different lowercase letters denote significant differences among treatments (Tukey, p < 0.05).
3.2 Nodule Number and Nodule Weight
Genotype variation and environmental treatment induced significant differences in nodule number, weight, and per-nodule weight (p < 0.01); however, the amplitude of variation between varieties was different (Table 1). Nodule number and weight per plant of GX showed the most significant decline in RSI (85.88% and 78.02%, respectively), and ND showed the lowest (94.94% and 91.07%, respectively). Moreover, nodule number and weight of NTS were the highest in M and RSI, but per-nodule weight was the lowest in M and was lower than ND in RSI.
Nodule weight was related to the nodule number and weight. There was an exponential relationship between weight and number (Fig. 2), whereas the exponential function was significantly different between M and RSI: yM = 267.25 * (1-exp(−0.0035*x))(R2 = 0.9701), yRSI = 31.5423 * (1-exp(−0.0103*x)) (R2 = 0.9668). The main reason causing this phenomenon was the significant decrease in RSI per nodule weight (Table 1).
Figure 2: The relationship of nodule number and nodule weight in M and RSI
Nodule number and weight per plant formed on the tap or lateral roots of soybean decreased significantly in RSI (p < 0.01), and the difference in nodule number among varieties was significant; however, nodule weight was not significant (Table 2). Nodule numbers formed on the tap or lateral root of NTS were all higher than those of GX and ND in M, and that of GX was higher than ND, but the opposite was true for the lateral roots. Nodule number, weight, and weight per nodule on the taproot of GX showed the most significant decline in RSI (84.09%, 96.48%, and 77.59%, respectively). The number of nodules formed on the taproot of NTS was higher than those of GX and ND in RSI, while those on the lateral roots were not significantly different from those of ND. The difference in lateral nodule weight per plant was significant among the varieties in M, with NTS being the highest and GX being the lowest.
The weight per nodule of tap or lateral roots decreased significantly in RSI, and the difference among the varieties was significant. The interaction between variety and environment treatment was also significant. The nodule weight per nodule of ND was higher than that of NTS and GX on the tap roots, but no significant difference was observed for those on the lateral roots in RSI.
The nodules were clustered on tap roots in RSI, and the distribution of nodule numbers varied significantly among varieties (Table 3). The ratio of tap nodule number of NTS was higher than that of ND and GX in RSI, but the difference was not significant in M.
Genotype variation and environmental treatment did not affect the nodule weight distribution. GX was the highest and NTS was the lowest in M, but there was no significant difference in RSI. Considering the amplitude of variation among different varieties, the ratio of tap nodule weight of NTS increased significantly, and GX decreased significantly in RSI by 15.32% and 13.00%, respectively.
Nodule density can reflect the ability of root nodules to form after rhizobia infection. The nodule density of the tap or lateral roots of NTS was significantly higher than that of GX and ND (Fig. 3). The nodule density of tap roots (NDT) of all varieties decreased significantly in RSI, with the decrease in NTS being the smallest (35.23%) and GX being the largest (71.28%), but there was no significant difference among varieties in nodule density of lateral roots (NDL).
Figure 3: Nodule density on tap and lateral roots of different soybean varieties in M and RSI
Note: * and ** Significantly different at the 0.05 and 0.01 probability levels, respectively.
The NDT of NTS in M was 2.37 and 3.24 times that of GX and ND, respectively. However, it was 4.01 and 4.42 times higher than that of GX and ND in RSI. The results showed that the difference in NDT between NTS and the other varieties was amplified in RSI, which may be associated with differences in nodulation characteristics and sensitivity of tap root growth to environmental changes.
Dry weight-specific nodulation (DW-specific, number of nodules per gram dry weight root) is a more accurate measure of treatment effects on the nodulation process because it is a measure of modulation relative to the amount of root available to modulate. The DW-specific NTS was higher than that of GX and ND, regardless of M or RSI (Fig. 4). The DW-specific decreased significantly in RSI, with the decrease in NTS being the smallest (51.82%) and GX being the largest (67.20%).
Figure 4: DW-specific modulation of different soybean varieties in M and RSI
The DW-specific NTS was 1.27 and 1.24 times that of GX and ND in M, respectively. However, it was 1.55 and 1.69 times that of GX and ND in RSI. This means that the inhibition of nodulation and the formation of supernodulation in NTS in RSI is relatively less than that of common varieties, this difference is likely related to the nodulation characteristics of NTS and the distribution of photosynthetic products within its root system.
3.6 The Relationship between Nodule Dry Weight and Root/Whole Plant Dry Weight
Root dry weight (RDW), shoot dry weight (SDW), and root/shoot value (R/S) decreased significantly in the RSI treatment, with significant differences observed among the varieties (Table 4). The RDW and SDW of ND were higher than those of NTS in M and RSI. The R/S of NTS was higher than that of ND in M, but there was no significant difference in the RSI between NTS and ND because the decrease in the RDW of NTS (55.73%) was significantly higher than that of ND (38.03%). The RDW of GX was lower than that of ND in both M and RSI. There was no significant difference between NTS and ND of SDW in M, but the SDW of GX was lower than ND in RSI. The decrease in RDW was significantly higher than that in SDW in RSI, which may be related to the different shade tolerances.
Nodule/root ratio (N/R) and nodule/whole plant ratio (N/W) both decreased significantly in RSI. There were significant differences among the varieties for N/R or N/W in M; NTS was the highest, and GX was the lowest. NTS was higher than GX and ND in RSI, but the difference between NTS and ND was not significant.
3.7 Nitrogenase Activity, Leghemoglobin, and Sucrose Content of Nodule
Nitrogenase activity, leghemoglobin content, and sucrose levels significantly decreased in RSI (Fig. 5). At the unit level, there were significant differences in nitrogenase activity and leghemoglobin among varieties in M and RSI, with ND exhibiting the highest values and NTS the lowest. Meanwhile, there was no difference in sucrose among the varieties in M, but ND was higher than NTS and GX in RSI.
Figure 5: Nitrogenase activity (A and B), leghemoglobin (C and D), and sucrose (E and F) content of nodules at per-nuit level (A, C, and E) and whole-plant level (B, D and F) of different soybean varieties in M and RSI
Note: A, nitrogenase activity per unit (μmol g−1 h−1); C, leghemoglobin content per unit (mg g−1); E, sucrose content per unit (mg g−1); B, nitrogenase activity per plant (μmol h−1); D, leghemoglobin content per plant (mg); and F, sucrose content per plant (mg). * and ** Significantly different at the 0.05 and 0.01 probability levels, respectively.
At the plant level, nitrogenase activity, leghemoglobin content, and sucrose levels in GX were lower than those in NTS and ND, regardless of M or RSI. Moreover, nitrogenase activity and sucrose levels of NTS were higher than those of ND in M.
A correlation analysis was conducted to determine the relationship between nitrogen fixation ability and root nodule characteristics (Table 5). NAP was significantly positively correlated with NW, RW, SW, and LCU, while NAW was significantly positively correlated with NN, NW, PNW, RW, and SCU.
The below-ground parts of plants play key roles in plant function and performance [23], and plant root plasticity is an important characteristic that confers adaptability across variable environments [24]. As for the location of the nodules, the growth status of the root directly affects nodule formation and development, which exhibit localized sink activity of photosynthates for the energy source as well as the structural materials [25]. As assimilates from photosynthesis provide energy to fuel the symbiosis between legumes and rhizobia, the light conditions under which host plants grow are very important. Our results showed that the accumulation of shoot and root dry weights decreased significantly because of the decrease in PAR and photosynthetic capacity, and nodule number and weight also decreased significantly with shoot shading. Overall, the root/shoot and nodule/plant ratios decreased significantly, indicating that photosynthates were preferentially distributed to the shoots. Furthermore, both root-to-shoot ratio and DW-specific declined, the priority of nodule initiation and formation declined in shade, and photosynthates were preferentially distributed to shoots and roots, but not nodules, to ensure better adaptation of the host plant to changing environments [9], although the developing nodules are strong sinks for assimilates [26]. Comparing varieties, nodule number and weight of NTS were the highest, regardless of M or RSI, which is related to a defective systemic autoregulatory signal that originates in the shoot and arrests nodule development [27]. Shade-sensitive GX had the most significant decline in RSI, and shade-tolerant ND had the least. This demonstrates that adaptability to shading varies among varieties, and directly regulates nodule growth. Thus, nodules can be an important indicator of plant adaptation to adverse conditions [28].
Nodule number and nodule weight were not independent variables, and individual nodule biomass was an important connection factor between them. Nodule weight directly determines nitrogen-fixation ability and consequently affects whole plant growth, which can influence nodule emergence and development [29,30]. Our results indicated a co-regulation between nodule number and nodule weight, following an exponential relationship rising to a maximum, and this relationship was fitted to both M and RSI. However, the increase in nodule weight with nodule number in RSI was less than that in M because of the decrease in the individual nodule biomass. Consequently, the exponential function was significantly different when comparing M and RSI.
Lateral roots can provide more positions for rhizobium infection and nodule occurrence than tap roots owing to their extensive root surface area [23,31], so the lateral nodule number and weight were higher than those of the tap root, regardless of M or RSI. However, the individual lateral nodule weight was less than that of the tap root, which was related to the nodule occurrence time in which most of the lateral nodules were later than that of the tap root [32], and also related to the sucrose transport for nodule development from shoots with phloem and sugar efflux transport proteins [33,34]. In RSI, the ratio of tap root nodule, numbers increased significantly with nodule clustering, but there was no significant effect on the ratio of nodule weight because the individual nodule weight of the tap root declined more than that of the lateral root, which related to excessively crowded nodules and restricted nodule expansion. The lateral nodule number and weight ratio of the NTS showed the greatest decrease, which was attributed to the greatest decrease in the lateral number and total length [35]. The nodule density of the tap root was amplified between NTS and others, but that of the lateral roots showed no difference, which was the result of the combined effect of super-nodulation characteristics and lateral roots being more sensitive to adversity [36].
The N2-fixation is an extremely energy-intensive process. Sucrose provides energy and C skeletons, and the nitrogenase-catalyzed reduction of N2 is carried out in a high-throughput, low-concentration oxygen environment by leghemoglobin [29,37]. ND and NTS had the same leghemoglobin content in M at the plant level, but the nitrogenase activity of NTS was significantly higher than that of ND because of the higher content of sucrose in nodules, and sucrose availability limited the nitrogen-fixing ability [34]. In RSI, decreased leghemoglobin and sucrose content in nodules limited nitrogenase activity, while their decrease at the per-unit level was smaller than that on the whole plant. This means that plant nitrogen-fixing ability was not only influenced by unit nitrogenase activity but also regulated by nodule number and weight.
This study showed that the nodule number, nodule weight, and DW-specific declined at the seedling stage in RSI because the dry matter accumulation capacity decreased and photosynthates were preferentially distributed to the shoot with shoot shading. Moreover, the exponential function relationship between nodule number and nodule weight changed because of the decrease in the individual nodule biomass. The nodules clustered on the taproot, but the nodule density of the taproot remained unchanged. With decreased leghemoglobin and sucrose content in nodules, nitrogenase activity declined significantly, especially at the whole-plant level.
Acknowledgement: We are thankful to all staff in our research group, and Titriku John Kwame for the English language editing of this manuscript.
Funding Statement: This work was supported by the “Challenge Banner and Leadership Board” of Sichuan Science and Technology Project (24JBGOV0007), earmarked fund for CARS-04-CES33, Nanchong Science and Technology Project (23JCYJPT0007), and the Sweetpotato and Leguminosae Germplasm Innovation and Utilization Key Laboratory of Sichuan Province Open Project (2023SLGIU03).
Author Contributions: Wenyu Yang designed the experiments. Jiangang An, Mingrong Zhang and Haiying Wu performed the experiments and analyzed the data. Xiaobo Yu and Taiwen Yong wrote and revised the manuscript. All authors reviewed the results and approved the final version of the manuscript.
Availability of Data and Materials: The data that support the findings of this study are available from the corresponding authors upon reasonable request.
Ethics Approval: Not applicable.
Conflicts of Interest: The authors declare no conflicts of interest to report regarding the present study.
References
1. Blessing DJ, Gu Y, Cao M, Cui Y, Wang X, Asante BB. Overview of the advantages and limitations of maize-soybean intercropping in sustainable agriculture and future prospects: a review. Chil J Agr Res. 2022;82:177–88. doi:10.4067/S0718-58392022000100177. [Google Scholar] [CrossRef]
2. Song C, Wang QL, Zhang XF, Sarpong CK, Wang WJ, Yong TW, et al. Crop productivity an nutrients recovery in maize-soybean additive relay intercropping system under subtropical regions in Southwest China. Int J Plant Prod. 2020;14:373–87. doi:10.1007/s42106-020-00090-9. [Google Scholar] [CrossRef]
3. Gu C, Bastiaans L, Anten NPR, Makowski D, Van DW. Annual intercropping suppresses weeds: a meta-analysis. Agr Ecosyst Environ. 2021;322:107658. doi:10.1016/j.agee.2021.107658. [Google Scholar] [CrossRef]
4. Zhang C, Dong Y, Tang L, Zheng Y, Makowski D, Yu Y, et al. Intercropping cereals with faba bean reduces plant disease incidence regardless of fertilizer input; a meta-analysis. Eur J Plant Pathol. 2019;154:931–42. doi:10.1007/s10658-019-01711-4. [Google Scholar] [CrossRef]
5. Du Q, Zhou L, Chen P, Liu X, Song C, Yang F, et al. Relay-intercropping soybean with maize maintains soil fertility and increases nitrogen recovery efficiency by reducing nitrogen input. Crop J. 2020;8:140–52. doi:10.1016/j.cj.2019.06.010. [Google Scholar] [CrossRef]
6. Perret X, Staehelin C, Broughton WJ. Molecular basis of symbiotic promiscuity. Microbiol Mol Biol R. 2000;64:180–201. [Google Scholar]
7. Voisin AS, Salon C, Jeudy C. Symbiotic N2 fixation activity in relation to C economy of Pisum sativum L. as a function of palnt phenology. J Exp Bot. 2003c;54:2733–44. [Google Scholar] [PubMed]
8. Bethlenfalvay GJ, Phillips DA. Effect of light intensity on efficiency of carbon dioxide and nitrogen reduction in Pisum sativum L. Plant Physiol. 1977;60:868–71. [Google Scholar] [PubMed]
9. Caetano-Anollès G, Gresshoff PM. Plant genetic control of nodulation. Annu Review Microbiol. 1991;45:345–82. [Google Scholar]
10. Mark K, Peter MG. Investigation of downstream signals of the soybean autoregulation of nodulation receptor kinase GmNARK. Mol Plant Microbe In. 2008;10:1337–48. [Google Scholar]
11. Voisin AS, Salon C, Jeudy C, Warembourg FR. Root and nodule growth in Pisum sativum L. in relation to photosynthesis. Analysis using 13C labelling. Ann Bot. 2003b;9:557–63. [Google Scholar]
12. Voisin AS, Salon C, Jeudy C, Warembourg FR. Seasonal patterns of 13C partitionning between shoot and nodulated roots of N2-or nitrate fed-Pisum sativum L. Ann Bot. 2003a;91:539–46. [Google Scholar] [PubMed]
13. Becker JN, Grozinger J, Sarkar A, Reinhold-Hurek B, Eschenbach A. Effects of cowpea (Vigna unguiculata) inoculation on nodule development and rhizosphere carbon and nitrogen content under simulated drought. Plant Soil. 2024;500:33–51. [Google Scholar]
14. Ploschuk PA, Miralles DJ, Striker GG. A quantitative review of soybean responses to waterlogging: agronomical, morpho-physiological and anatomical traits of tolerance. Plant Soil. 2022;475:237–52. [Google Scholar]
15. Fu ZD, Chen P, Zhang XN, Du Q, Zheng BC, Yang H, et al. Maize-legume intercropping achieves yield advantages by improving leaf functions and dry matter partition. BMC Plant Biol. 2023;23:438. doi:10.1186/s12870-2023-04408-3. [Google Scholar] [CrossRef]
16. Wu YS, Wang EL, Gong WZ, Xu L, Zhao ZG, He D, et al. Soybean yield variations and the potential of intercropping to increase production in China. Field Crop Res. 2023;291:108771. [Google Scholar]
17. Zhao XY, Hu Y, Liang B, Chen GP, Feng L, Pu T, et al. Coordination of density and nitrogen fertilization improves stalk lodging resistance of strip-intercropped maize with soybeans by affecting stalk quality traits. Agriculture. 2023;13(5):1009. [Google Scholar]
18. He LX, Xu M, Wang WY, Liu CY, Yu L, Liu WG. The interaction between strigolactone and auxin results in the negative effect of shading on soybean branching development. Agronomy J. 2023;13(9):2383. [Google Scholar]
19. Yang LD, Ren JB, Peng XY, Yang XL, Luo K, Chen P, et al. Crop growth characteristics and its effects on yield formation through nitrogen application and interspecific distance in soybean/maize strip relay intercropping. Acta Agron Sin. 2024;50(1):251–64. [Google Scholar]
20. Carlsson G, Palmborg C, Jumpponen A, Scherer-Lorenzen M, Hogberg P, Huss-Danell K. N2 fixation in three perennial Trifolium species in experimental grasslands of varied plant species richness and composition. Plant Ecol. 2009;205:87–104. [Google Scholar]
21. Rajae V, Alain G, Didier V. Short-term effects of high light intensities on soybean nodule activity and photosynthesis. Environ Exp Botany. 1996;36(3):349–57. doi:10.1016/0098-8472(96)00129-3. [Google Scholar] [CrossRef]
22. Schweitzer LE, Happer JE. Effect of light, dark, and temperature on root nodule activity (acetylene reduction) of soybeans. Plant Physiol. 1985;65:51–6. doi:10.1104/pp.65.1.51. [Google Scholar] [PubMed] [CrossRef]
23. Freschet GT, Pages L, Iversen C, Comas L, Mccormack ML. A starting guide to root ecology: strengthening ecological concepts and standardising root classification, sampling, processing and trait measurements. New Phytol. 2021;232:973–1122. doi:10.1111/nph.17572. [Google Scholar] [PubMed] [CrossRef]
24. Ryser P, Eek I. Consequences of phenotypic plasticity vs. interspecific differences in leaf and root traits for acquisition of aboveground and belowground resources. Am J Bot. 2000;87(3):402–11. doi:10.2307/2656636. [Google Scholar] [CrossRef]
25. Sayuri I, Norikuni O, Kuni S, Takuji O. Allocation of photosynthetic products in soybean during the early stages of nodule formation. Soil Sci Plant Nutr. 2006;52:438–43. doi:10.1111/j.1747-0765.2006.00051.x. [Google Scholar] [CrossRef]
26. Fujikake H, Tamura Y, Ohtake N, Sueyoshi K, Ohyama T. Photoassimilate partitioning in hypernodulation mutant of soybean (Glycine max (L.) Merr) NOD1-3 and its parent Williams in relation to nitrate inhibition of nodule growth. Soil Sci Plant Nutr. 2011;49:583–90. [Google Scholar]
27. Brett JF, Arief I, Satomi H, Lin MH, Lin YH, Dugald ER. Molecular analysis of legume nodule development and autoregulation. J Integr Plant Biol. 2010;52(1):61–76. [Google Scholar]
28. Zari S, Sardor AK, Khabibullo FS. Effect of salinity and drought on symbiotical and biochemical properties of Onobrychis and alfalfa. Agric Sci. 2012;3(3):444–54. [Google Scholar]
29. Voisin AS, Nathalie G, Christophe S. The nodulation process is tightly adjusted to plant growth. An analysis using environmentally and genetically induced variation of nodule number and biomass in pea. Plant Soil. 2010;337:399–412. [Google Scholar]
30. Joel LS, Kenjiro WQ, Camille EW. Legumes versus rhizobia: a model for ongoing conflict in symbiosis. New Phytol. 2018;219:1199–206. [Google Scholar]
31. Timothy RM, Peter HG. Bradyrhizobium japonicum inoculant mobility, nodule occupancy, and acetylene reduction in the soybean root system. Appl Environ Microb. 1989;55(10):2493–8. doi:10.1128/aem.55.10.2493-2498.1989. [Google Scholar] [PubMed] [CrossRef]
32. Liu AL, Contador A, Fan KJ, Larm HM. Interaction and regulation of carbon, nitrogen, and phosphorus metabolisms in root nodules of legumes. Front Plant Sci. 2018;9:1860. doi:10.3389/fpls.2018.01860. [Google Scholar] [PubMed] [CrossRef]
33. Schwember AR, Schulze J, Pozo AD, Cabeza RA. Regulation of symbiotic nitrogen fixation in legume root nodules. Plants. 2019;8(9):333. doi:10.3390/plants8090333. [Google Scholar] [PubMed] [CrossRef]
34. Yu XB, Luo L, Zeng XT, Su BY, Gong WZ, Yong TW, et al. Response of roots morphology and physiology to shading in maize-soybean relay strip intercropping system. Chin J Oil Crop Sci. 2015;37(2):185–93. (In Chinese). [Google Scholar]
35. Callaway RM, Pennings SC, Richards CL. Phenotypic plasticity and interactions among plants. Ecology. 2003;84(5):1115–28. doi:10.1890/0012-9658(2003)084[1115:PPAIAP]2.0.CO;2. [Google Scholar] [CrossRef]
36. Larrainzar E, Villar I, Rubio MC, Maria CPR, Carmen H, Raul S. Hemoglobins in the legume-Rhizobium symbiosis. New Phytol. 2000;228:472–84. doi:10.1111/nph.16673. [Google Scholar] [PubMed] [CrossRef]
37. Lodwig E, Poole P. Metabolism of rhizobium bacteroids. Crit Rev Plant Sci. 2003;22(1):37–78. doi:10.1080/713610850. [Google Scholar] [CrossRef]
Cite This Article
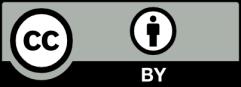
This work is licensed under a Creative Commons Attribution 4.0 International License , which permits unrestricted use, distribution, and reproduction in any medium, provided the original work is properly cited.