Open Access
ARTICLE
Effects of PBO and PP333 on Shoot Growth, Nutrient Accumulation, and Fruit Quality in Carya Illinoinensis cv. ‘Shaoxing’
Guangxi Colleges and Universities Key Laboratory for Cultivation and Utilization of Subtropical Forest Plantation, Guangxi Key Laboratory of Forest Ecology and Conservation, College of Forestry, Guangxi University, Nanning, 530004, China
* Corresponding Authors: Weidong Xie. Email: ; Zailiu Li. Email:
# These authors contributed equally to this work
Phyton-International Journal of Experimental Botany 2024, 93(12), 3293-3312. https://doi.org/10.32604/phyton.2024.058083
Received 04 September 2024; Accepted 28 November 2024; Issue published 31 December 2024
Abstract
To enhance the productivity of Carya illinoinensis cv. ‘Shaoxing’ and mitigate the loss of flowers and fruits, the effects of different concentrations of Piperonyl Butoxide (PBO) wettable powder (2, 5, and 10 g·L–1) and Paclobutrazol (PP333) (150, 300, and 450 mg·L–1, based on active ingredients) on 6-year-old ‘Shaoxing’ plants were investigated with water sprayed as the control. The results showed that: (1) Treatment with 10 g·L–1 PBO and 450 mg·L–1 PP333 significantly inhibited the excessive growth of ‘Shaoxing’ branches. Also, 10 g·L–1 PBO exhibited the best diameter increment effect on fruiting branches, and 150 mg·L–1 PP333 exhibited the best diameter increment effect on vegetative branches. (2) The content of soluble sugar and soluble protein in leaves treated reached the highest level after treatment with 450 mg·L–1 PP333, while the content of starch sugar in leaves reached the highest level after treatment with 300 mg·L–1 PP333. The application of PBO and PP333 mitigated the decline in N, P, K, and other nutrient levels observed in the leaves of ‘Shaoxing’. As the PBO and PP333 concentrations increased, the nutrient elements in the leaves first increased and then decreased. Among them, 300 mg·L–1 PP333 treatment exhibited the best effect on increasing the content of N, P, and K in the leaves at the late stage of fruit development. (3) In terms of fruit setting rate and nutritional quality of ‘Shaoxing’ fruit, 5 g·L–1 PBO treatment showed the most promising effect on improving fruit setting rate, 150 mg·L–1 PP333 exhibited the best effect on improving reducing sugar and decreasing tannin content in the kernel, 10 g·L–1 PBO had the best effect on improving the crude fat content, and 2 g·L–1 PBO had the best effect on improving the cellulose content in the kernel. (4) Principal component analysis showed that 450 mg·L–1 PP333 treatment had the most comprehensive regulatory effect on the growth and development of current-year branches, leaves, and fruits of ‘Shaoxing’. This study provided a theoretical basis and data reference for the growth and development of C. illinoinensis cv. ‘Shaoxing’ fruits from the perspective of the application of plant growth regulators.Keywords
Nomenclature
C.illinoinensis | Carya illinoinensis |
PBO | Piperonyl butoxide |
PP333 | Paclobutrazol |
Carya illinoinensis K. Koch is a member of the walnut family (Juglandaceae), which is native to the United States and Mexico. It was introduced into China during the late 19th and early 20th centuries [1]. The flowering period of C. illinoinensis is from April to May, while the fruiting period extends from May to October. Following planting, the tree begins to bear fruits in its fourth to sixth years and enters the full fruiting period in its seventh to eighth years [2]. Among the numerous varieties introduced into China, ‘Shaoxing’, ‘Pawnee’, ‘Mahan’, and ‘Xibu’ occupy a dominant position. ‘Shaoxing’ is particularly important for its extensive promotion in Huanjiang Maonan Autonomous County, Hechi City, Guangxi Zhuang Autonomous Region [3]. ‘Shaoxing’ exhibits excellent adaptability and high-quality fruits. A 6-year-old plant can enter the initial production phase. The oil content of the fruit kernel can reach 68.1%, with the linoleic acid reaching about 12.56% [1]. However, the introduction of C. illinoinensis has resulted in several undesirable phenomena, including excessive growth of branches and leaves, a low yield of fruits, severe flower and fruit drop, and poor fruit quality. Additionally, reproductive obstacles such as a poor flowering period and poor pollination and fertilization during reproduction contribute to a further reduction in fruit yield [4]. These phenomena have significantly affected the yield and productivity of C. illinoinensis cv. ‘Shaoxing’, consequently affecting the income of local growers. To summarize, inhibiting excessive branch growth, promoting flowering and fruiting, and increasing fruit quality during the introduction and cultivation of ‘Shaoxing’ to improve economic benefits and facilitate the sustainable development of the C. illinoinensis industry in the region is extremely important.
The rational application of plant growth inhibitors and retardants represents a crucial strategy for regulating the vegetative and reproductive growth of plants. It can also be applied to mitigate flower and fruit drop and enhance fruit quality [5,6]. Paclobutrazol (PP333, C15H2N3OCl), also known as chlorobutrazol, is a triazole compound. It can reduce cell division and elongation by inhibiting the synthesis of endogenous gibberellin in plants, thus inhibiting plant growth or the growth of branches. It is a commonly used chemical in agricultural and forestry production because it can efficiently regulate shoot growth, promote fruit development, and inhibit the excessive proliferation of branches and leaves [7]. However, the prolonged application of PP333 results in environmental contamination and the emergence of drug-resistant plants, which can lead to branch death, diseases, and yield reduction. To overcome these limitations, Piperonyl Butoxide (PBO) has emerged as a promising alternative in recent years for treating fruit trees. PBO is a compound preparation comprising a range of growth regulators (e.g., uniconazole, cytokinins, and auxin derivatives), swelling agents, colorants, trace elements, and other components. It has several functions, including the inhibition of excessive shoot growth, an increase in chlorophyll content, the shortening of internodes, the promotion of flowering, an improvement in fruit quality, and an increase in plant resistance [8]. In recent years, PP333 and PBO have been applied extensively in the cultivation of various fruit trees. The application of PP333 can increase the yield and quality of fruit produced by different tree species, including C. illinoinensis cv. ‘Pawnee’ [9], Anacardium occidentale [10], Citrus reticulata [11], and others. Additionally, PP333 can inhibit the longitudinal growth of branches. Treatment with 1500 mg·L–1 PP333 considerably increased the starch content and C/N ratio in Taxus chinensis leaves [12]. The combination of 100 mg·L–1 NAA and 200 mg·L–1 PP333 can inhibit the growth of C. illinoinensis seedlings, stimulate root development, and increase the root-shoot ratio [13]. Compared to treatment with auxin, ABA, and salicylic acid, treatment with PP333 strongly inhibited the vegetative growth of Olea europaea and increased the fruit-setting rate. Foliar spraying is a more effective method than soil treatment [14]. PBO was found to be effective when applied to a range of fruit trees, such as Pyrus Bret Schneider Rehd. [15] and Vitis labrusca × Vitis vinifera ‘Kyoho’ [16], which can control the growth of new shoots and promote the development and maturation of fruits. The application of PBO significantly promoted the growth of Malus Pumila Mill. Shoots, inhibit the growth of new shoots, and increase the chlorophyll content of leaves, fruit weight, and fruit shape index [17]. To summarize, PP333 and PBO can inhibit the growth of branches and internode elongation in various plant species. Moreover, they exert regulatory effects on fruit quality, yield, and plant growth. Additionally, PBO can increase the proportion of carbohydrates in leaves, stimulate the accumulation of photosynthetic products, and promote early fruit maturation. However, further studies are needed to gain a deeper understanding of the effects of PP333 and PBO on C. illinoinensis cv. ‘Shaoxing’ and determine the optimal treatment concentration.
Carya illinoinensis is planted in a large part of Hechi City, Guangxi Zhuang Autonomous Region, China. Some studies have shown that the appropriate application of PP333 and PBO can facilitate the growth and yield of C. illinoinensis. The application of PP333 and PBO during the growth and development period may address the problems of flower and fruit drop, a low fruit setting rate, and reduced yield during the introduction and cultivation of C. illinoinensis cv. ‘Shaoxing’. In this study, 6-year-old C. illinoinensis cv. ‘Shaoxing’ plants were used to conduct experiments. During the flowering and fruiting periods, different concentrations of PBO and PP333 solutions were applied to the leaves by spraying. The effects of treatment with different growth regulators on the regulation of shoots and fruits, nutrient accumulation, and fruit quality of C. illinoinensis cv. ‘Shaoxing’ plants were analyzed. From the perspective of plant growth regulator application, this study provided a theoretical basis for regulating the growth of introduced C. illinoinensis in Huanjiang County.
The experimental site was located in Huanjiang Maonan Autonomous County, Hechi City, Guangxi Zhuang Autonomous Region (108.56°E, 24.51°N, altitude 470.3 m). The area has a subtropical monsoon type of climate. The annual average number of solar irradiation hours is 1396, the annual average temperature is 19.9°C, the frost-free period is 290 days, and the annual average precipitation is 1389.1 mm. Most of the precipitation occurs during the spring and summer months, accounting for about 70% of the annual total. The study was conducted from May to September 2019.
Based on the scheme proposed by Zhang et al., a two-factor randomized block design was used to apply different concentrations of PBO and PP333 plant growth regulators to the leaves of 6-year-old C. illinoinensis cv. ‘Shaoxing’ plants (Fig. A1), with clear water serving as the control (CK) [18] (Table A1). The experiment comprised seven treatments, with 21 plants allocated to each treatment, seven plants allocated to each replicate, and three replicates. During the experiment, the plants that displayed consistent growth and no pests or diseases were selected for further study. Three branches of the ‘Shaoxing’ sample tree were selected from the east, south, west, and north of the middle of the crown for marking and numbering. Foliar sprays of the growth regulators were applied twice: once on May 2 and again on July 15 (an interval of 75 days). Each tree was sprayed with about 700–800 mL of solution until the leaf surfaces were saturated and then dripped (Fig. A1). PBO wettable powder, sourced from Huaye Agricultural Technology Co., Ltd. (Jiangyin, China), was prepared at concentrations of 2, 5, and 10 g·L−1, referring to the total formulation concentration according to the manufacturer’s specifications; PP333 wettable powder (containing 15% active ingredients), obtained from Guoguang Agricultural Chemical Co., Ltd. (Chengdu, China), was prepared at concentrations of 150, 300, and 450 mg·L−1 based on the actual active ingredient content (Fig. A2). Throughout the experiment, maintenance and management conditions, such as irrigation and fertilization, were maintained consistently, and weeds were removed 1–2 times per month.
The growth of the branch internodes was measured on days 19 and 147 after the flowering (an interval of 128 days). Three fruiting branches and three vegetative branches with comparable growth states were randomly selected from the east, south, west, and north of the middle part of the crown of the sample plant for numbering. The diameter of the base of the branch and the length of the third internode were measured using a digital vernier caliper, with an accuracy of 0.01 mm. Each treatment was repeated three times.
2.3.2 Leaf Physiological Indices
As described by Jia et al., the fruit development period of C. illinoinensis can be divided into four distinct phases: the fruit slow growth period, the fruit rapid expansion period, the fruit hardcore period, and the fruit kernel maturity period. The contents of soluble sugar, soluble protein, and starch in the leaves of the ‘Shaoxing’ were determined at 19, 83, 117, and 147 days after flowering [19]. The healthy leaves situated at the periphery of the sample branches in the east, west, south, and north of the plant were mixed and bagged, representing a single plant unit. The samples were quickly transferred to a biological sample refrigerator, transported to the laboratory, and placed in an ultralow temperature refrigerator at −80°C for subsequent analysis. The content of soluble sugar and starch in the leaves was determined by anthrone colorimetry [20], whereas the soluble protein content was determined by the Coomassie brilliant blue G-250 method [21].
The leaves of ‘Shaoxing’ sample branches were collected at 19, 83, 117, and 147 days after flowering, de-enzyme at 105°C for 0.5 h in an oven, and then adjusted to 80°C until a constant weight was reached. The dried materials were pulverized, filtered, and stored in a sealed container at room temperature in a cool environment to determine the total N, P, and K contents in the leaves. The total N content in the leaves was determined by H2SO4-H2O2 digestion using the Kjeldahl method. The total phosphorus content of the leaves was determined using the molybdenum antimony colorimetric method, whereas the total potassium content was determined by flame photometry [22].
The number of female flowers on each branch of each plant was investigated at the late flowering stage (May 2), and the number of fruit set on each branch of each plant was investigated at the fruit maturity stage. The fruit setting rate, defined as the percentage of fruit set to the number of female flowers was calculated.
2.3.5 Nutrient Indices of the Fruit Kernel
At 147 days after the flowering of ‘Shaoxing’, 4–6 fruits were collected from the east, south, west, and north of the branches of each sample plant in each treatment, and 10 fruits were randomly selected after classification and treatment of the harvested fruits. After removing the peel and kernels, the samples were placed in an ultralow temperature refrigerator set at −80°C to determine the nutrients. The tannin content in the kernels was determined by spectrophotometry [23]. The crude fat content of the fruit was determined by the Soxhlet extraction method [24]. The soluble protein content of the kernels was determined by the Coomassie brilliant blue G-250 method [21]. The reducing sugar content in the kernels was determined by the 3,5-dinitrosalicylic acid method [25]. The cellulose content in the kernels was determined by the nitric acid-ethanol method [26].
The data were analyzed using Microsoft Excel 2016 (USA). IBM SPSS 20.0 (USA) was used to conduct multivariate analysis of variance and principal component analysis; the Duncan method (α = 0.05) was used for multiple comparisons. The principal component analysis included a correlation matrix, in which 16 indicators closely related to the shoot control and fruit promotion in ‘Shaoxing’ were selected. The number of principal components was determined according to an eigenvalue greater than 1.0. The function expression of the principal component was listed based on the eigenvector of the correlation matrix of ‘Shaoxing’. Finally, the comprehensive scores of the effects of PBO and PP333 on ‘Shaoxing’ were calculated and compared based on the principal component value.
3.1 Effects of PBO and PP333 on the Growth of Fruiting Branches and Vegetative Branches of ‘Shaoxing’
Different concentrations of PBO and PP333 significantly affected the increase in fruit branch length and vegetative branch length of ‘Shaoxing’ (p < 0.05) (Fig. 1a, Table A2). Among all treatments, the 450 mg·L–1 PP333 (D3) treatment had the most significant inhibitory effect on the growth of fruit branches and vegetative branch length. The increase in the internode lengths of the fruiting branches and vegetative branches were 4.04 and 2.44 mm, respectively, which were 45.26% and 62.46% lower than those of the control group (7.38 and 6.50 mm, respectively). Regarding the application of the two growth regulators, the increase in the internode length of fruiting branches and vegetative branches decreased with an increase in the concentration of the growth regulators. The optimal inhibitory concentrations of the two growth regulators on fruiting branches and vegetative branches were 10 g·L–1 PBO (P3) and 450 mg·L–1 PP333 (D3), respectively.
Figure 1: Effects of different treatments on the increase in internode length and diameter of fruiting branches and vegetative branches of ‘Shaoxing’. (a) Effects of different treatments on the increase in the internode length of fruiting branches and vegetative branches. (b) Effects of different treatments on the increase in the internode diameter of fruiting branches and vegetative branches. Different lowercase letters indicate significant differences in the growth of fruiting branches among the different treatments (p < 0.05), and different uppercase letters indicate significant differences in the growth of vegetative branches among the different treatments (p < 0.05); the error line represents the standard error
Different concentrations of PBO and PP333 significantly affected the increase in vegetative branch diameters in the ‘Shaoxing’ (p < 0.05), whereas their impact on fruiting branch diameter growth was not statistically significant (p > 0.05, Table A2) (Fig. 1b). In all treatments, the increase in branch diameter reached a maximum value of 1.63 mm after treatment with 10 g·L–1 PBO (P3), which was 31.45% greater than that of the control group (1.24 mm). The increase in the internode diameter of vegetative branches reached a maximum value of 1.35 mm after treatment with 150 mg·L–1 PP333 (D1), which was 141.07% greater than that of the control group (0.56 mm). As the PBO and PP333 concentrations increased, the increase in the internode diameter of fruiting branches increased, and the increase in the internode diameter of vegetative branches first increased and then decreased. The optimum concentrations of the two growth regulators to promote an increase in the diameter of fruiting branches were 10 g·L–1 PBO (P3) and 450 mg·L–1 PP333 (D3), and the optimum concentrations to promote an increase in the diameter of vegetative branches were 5 g·L–1 PBO (P2) and 150 mg·L–1 PP333 (D1).
To summarize, both PBO and PP333 significantly inhibited the excessive growth of vegetative branches and fruiting branches. The inhibitory effect of PP333 was stronger than that of PBO, and the inhibitory effect of PP333 on vegetative branches was stronger than that on fruiting branches. Treatment with 450 mg·L–1 PP333 (D3) had the greatest inhibitory effect on the growth of the internode of fruiting branches and vegetative branches. The PBO and PP333 treatments significantly increased the diameter of the vegetative branches of ‘Shaoxing’. Treatment with 10 g·L–1 PBO (P3) had the strongest effect on increasing the diameter of fruiting branches, whereas treatment with 150 mg·L–1 PP333 (D1) had the greatest effect on effect on vegetative branches.
3.2 Effects of PBO and PP333 on the Physiological Indices and Nutritional Status Indices of ‘Shaoxing’ Leaves
3.2.1 Effects of PBO and PP333 on the Physiological Indices of ‘Shaoxing’ Leaves
Different concentrations of PBO and PP333 significantly affected the soluble sugar content in the leaves of ‘Shaoxing’ plants (p < 0.05) (Fig. 2a, Table A3). Following the transition to different stages after anthesis (days 19 to 147), the soluble sugar content of the leaves in each treatment group increased, decreased, and finally increased. The changes in soluble sugar content in the leaves of the ‘Shaoxing’ at different stages of fruit development were as follows. At 19 days after flowering, the soluble sugar content of the leaves treated with 450 mg·L–1 PP333 (D3) reached a maximum value of 22.65 mg·g–1, which was 85.35% greater than that recorded in the control group. At 83 days after flowering, the soluble sugar content of the leaves in all treatment groups was lower than that in the control group. At 117 days after flowering, the soluble sugar content of the leaves treated with 10 g·L–1 PBO (P3) reached a maximum value of 21.31 mg·g–1, which was 31.87% greater than that of the control group. At 147 days after flowering, the soluble sugar content of leaves treated with 450 mg·L–1 PP333 (D3) reached a maximum value of 45.59 mg·g–1, which was 25.45% greater than that of the control group. These results indicated that 10 g·L–1 PBO (P3) and 450 mg·L–1 PP333 (D3) had the strongest effects on the soluble sugar content in the leaves of ‘Shaoxing’ fruits during the fruit development period (19–147 days after flowering) across all treatment groups.
Figure 2: Effects of different treatments on the physiological indices of ‘Shaoxing’ leaves. (a) Effects of different treatments on the soluble sugar content. (b) Effects of different treatments on the starch content. (c) Effects of different treatments on the soluble protein content. (d) Effects of different treatments on the N content in leaves. (e) Effects of different treatments on the P content in leaves. (f) Effects of different treatments on the K content in leaves. Different lowercase letters indicate significant differences (p < 0.05), and the error line represents the standard error
Different concentrations of PBO and PP333 significantly affected the soluble protein content in the leaves of ‘Shaoxing’ plants (p < 0.05) (Fig. 2b, Table A3). Following the transition to different stages after flowering (19–147 days after flowering), the soluble sugar content of the leaves in each treatment group increased, decreased, and finally increased. The changes in the soluble protein content in the leaves of the ‘Shaoxing’ at different stages of fruit development were as follows. At 19 days after flowering, the soluble protein content of the leaves treated with 5 g·L–1 PBO (P2) reached a maximum value of 0.62 mg·g–1, which was 40.91% greater than that of the control group. At 83 days after flowering, the soluble protein content of the leaves treated with 10 g·L–1 PBO (P3) reached a maximum value of 0.79 mg·g–1, which was 11.26% greater than that of the control group. At 117 days after flowering, the soluble protein content in the plants in the 2 g·L–1 PBO (P1) treatment group reached a maximum value of 0.59 mg·g–1, which was significantly greater than that in the control group, indicating an increase of 195.00%. At 147 days after flowering, the soluble protein content in the plants in the 450 mg·L–1 PP333 (D3) treatment group reached a maximum value of 1.07 mg·g–1, but the difference was not significant compared to that in the control group. These results indicated that treatment with PBO and PP333 did not significantly affect the soluble protein content in the leaves of ‘Shaoxing’. However, it partly mitigated the decrease in soluble protein content in leaves from days 83 to 117 after flowering.
Different concentrations of PBO and PP333 significantly affected the starch content in the leaves of ‘Shaoxing’ plants (p < 0.05) (Fig. 2c, Table A3). Following the transition to different stages after flowering (19–147 days after flowering), the starch content of the leaves in each treatment gradually increased. The changes in starch content in the leaves of ‘Shaoxing’ plants at different stages of fruit development were as follows. At 19 days after flowering, the starch content of the leaves treated with 300 mg·L–1 PP333 (D2) reached a maximum value of 14.92 mg·g–1, which was 96.32% greater than that of the control group. At 83 days after flowering, the starch content of the leaves treated with 300 mg·L–1 PP333 (D2) reached a maximum value of 23.22 mg·g–1, which was 91.90% greater than that of the control group. At 117 days after flowering, the starch content of the leaves treated with 300 mg·L–1 PP333 (D2) reached a maximum value of 18.42 mg·g–1, which was 47.48% greater than that of the control group. At 147 days after flowering, the leaf starch content of the leaves treated with 300 mg·L–1 PP333 (D2) reached a maximum value of 40.19 mg·g–1, which was 121.07% greater than that of the control group. To summarize, among the PBO treatments, 5 g·L–1 PBO (P2) had the strongest effect on promoting the starch content of ‘Shaoxing’ leaves during the fruit development period, whereas 300 mg·L–1 PP333 (D2) had the greatest effect among the PP333 treatments.
3.2.2 Effects of PBO and PP333 on the Nutritional Status Indices (Total N, P, and K Content) of ‘Shaoxing’ Leaves
Different concentrations of PBO and PP333 significantly affected the N content in the leaves of ‘Shaoxing’ plants (p < 0.05) (Fig. 2d, Table A3). With the changes in the different stages after flowering (19–147 days after flowering), the N content of the leaves in each treatment gradually decreased. The changes in the N content in the leaves of ‘Shaoxing’ at different stages of fruit development were as follows. At 19 days after flowering, the N content in the leaves treated with 450 mg·L–1 PP333 (D3) reached a maximum value of 26.03 mg·g–1, which was 15.02% greater than that of the control group. At 83 days after flowering, the N content of the leaves treated with 450 mg·L–1 PP333 (D3) reached a maximum value of 20.39 mg·g–1, which was 4.56% greater than that of the control group. At 117 days after flowering, the N content of the leaves treated with 10 g·L–1 PBO (P3) reached a maximum value of 19.61 mg·g–1, which was 8.58% greater than that of the control group. At 147 days after flowering, the N content in the leaves of the control group reached a maximum value of 22.13 mg·g–1, while the N content in the group treated with 300 mg·L–1 PP333 (D2) was the highest, which was 6.28% lower than that of the control group, and this difference was not statistically significant. These findings indicated that the nitrogen content of the leaves decreased during the fruit development period. The PP333 treatment partially increased the nitrogen content of leaves in the late stage of ‘Shaoxing’ fruit development. This resulted in a delay in the leaf yellowing period and an increase in the cycle of photosynthesis.
Different concentrations of PBO and PP333 did not significantly affect the P content in the leaves of ‘Shaoxing’ plants (p < 0.05) (Fig. 2e, Table A3). With the changes in the different stages after flowering (19–147 days after flowering), the P content of the leaves in each treatment decreased, increased, and finally decreased. The changes in leaf P content at different stages of ‘Shaoxing’ fruit development were as follows. At 19 days after flowering, the leaf P content of the leaves treated with 450 mg·L–1 PP333 (D3) reached a maximum value of 1.58 mg·g–1, which was 5.33% greater than that of the control group. At 83 days after flowering, the P content of the leaves treated with 2 g·L–1 PBO (P1) reached a maximum value of 0.78 mg·g–1, which was 69.57% greater than that of the control group. At 117 days after flowering, the P content of the leaves treated with 450 mg·L–1 PP333 (D3) reached a maximum value of 1.34 mg·g–1, which was 8.06% greater than that of the control group. At 147 days after flowering, the P content of the leaves treated with 300 mg·L–1 PP333 (D2) reached a maximum value of 1.10 mg·g–1, which was 34.15% greater than that of the control group. These results indicated that the application of PP333 and PBO at different concentrations did not significantly change the P content of leaves. However, the P content of the leaves treated with PBO and PP333 was greater than that of the control group during the middle and late stages of fruit development (83–147 days after flowering). These findings suggested that spraying PP333 may retard the decline in leaf P content during the late stages of ‘Shaoxing’ fruit development.
Different concentrations of PBO and PP333 significantly affected the K content in the leaves of ‘Shaoxing’ plants (p < 0.05) (Fig. 2f, Table A3). With the changes in the different stages after flowering (19–147 days after flowering), the K content of the leaves in each treatment first decreased and then increased. The changes in the K content in the leaves at different stages of ‘Shaoxing’ fruit development were as follows. At 19 days after flowering, the K content in the leaves treated with 150 mg·L–1 PP333 (D1) reached a maximum value of 7.85 mg·g–1, which was 12.79% greater than that of the control group. At 83 days after flowering, the total K content of the leaves treated with 150 mg·L–1 PP333 (D1) reached a maximum value of 7.20 mg·g–1, which was 26.76% greater than that of the control group. At 117 days after flowering, the K content of the leaves treated with 300 mg·L–1 PP333 (D2) reached a maximum value of 7.40 mg·g–1, which was 11.78% greater than that of the control group. At 147 days after flowering, the K content of the leaves treated with 300 mg·L–1 PP333 (D2) reached a maximum value of 8.83 mg·g–1, which was 20.14% greater than that of the control group. Our results indicated that while the application of PBO and PP333 did not lead to a notable alteration in the K content in leaves, the K content of leaves treated with different concentrations of PP333 during the fruit development period (19–147 days after flowering) was greater than that of the control group. These findings suggested that spraying PP333 can increase the K content of leaves during the fruit development of ‘Shaoxing’ fruits to a certain extent.
3.3 Effects of PBO and PP333 on the Fruit Setting Rate and Nutritional Quality of ‘Shaoxing’ Fruits
3.3.1 Effects of PBO and PP333 on the Fruit Setting Rate of ‘Shaoxing’
Different concentrations of PBO and PP333 had significant effects on the fruit setting rate of ‘Shaoxing’ (p < 0.05) (Fig. 3a, Table A4). In all the treatments, the fruit setting rate reached a maximum of 13.36% under the 5 g·L–1 PBO (P2) treatment, which was 59.81% greater than that of the control group (8.36%). After treatment with the two growth regulators, the fruit setting rate gradually decreased as the PP333 concentration increased but gradually increased as the PBO concentration increased. The fruit-setting rate of ‘Shaoxing’ was significantly different between the P2, P3, and D3 treatment groups and the control group (CK), and the fruit-setting rate of ‘Shaoxing’ branches under the P2 and P3 treatments was significantly greater than that of the CK, indicating that 2–10 g·L–1 PBO treatment can improve the fruit setting rate of ‘Shaoxing’.
Figure 3: Effects of different treatments on the fruit setting rate and fruit quality of ‘Shaoxing’. (a) Effects of different treatments on the fruit setting rate. (b) Effects of different treatments on the tannin content in fruit kernels. (c) Effects of different treatments on the crude fat content of fruit kernels. (d) Effects of different treatments on the soluble protein content of fruit kernels. (e) Effects of different treatments on the reducing sugar content of fruit kernels. (f) Effects of different treatments on the cellulose content of fruit kernels. Different lowercase letters indicate significant differences (p < 0.05), and the error line represents the standard error
3.3.2 Effects of PBO and PP333 on the Nutritional Quality of ‘Shaoxing’ Fruits
Different concentrations of PBO and PP333 significantly affected the tannin content of ‘Shaoxing’ fruits (p < 0.05) (Fig. 3b, Table A4). Among all treatments, the tannin content in the 150 mg·L–1 PP333 (D1) treatment group was the lowest (1.52%), which was 25.49% lower than that in the control group. After applying the two growth regulators, the tannin content gradually increased with an increase in PBO and PP333 concentrations. Except for treatment with 10 g·L–1 PBO (P3) and 450 mg·L–1 PP333 (D3), the tannin content of the remaining treatments was considerably lower than that of the control group. These findings suggested that low concentrations of PBO (2–5 g·L–1) and PP333 (150–300 mg·L–1) effectively decreased the tannin content in ‘Shaoxing’ fruit.
Different concentrations of PBO and PP333 significantly affected the content of crude fat in ‘Shaoxing’ fruit (p < 0.05) (Fig. 3c, Table A4). Among all treatments, the crude fat content of the 10 g·L–1 PBO (P3) treatment group reached a maximum value of 77.38%, which was 7.62% greater than that of the control group. After treatment with the two growth regulators, the content of crude fat in fruits increased gradually as the PBO and PP333 concentrations increased. The crude fat content in the 10 g·L–1 PBO (P3) and 450 mg·L–1 PP333 (D3) treatment groups was significantly greater than that in the control group, indicating that high concentrations of PBO (10 g·L–1) and PP333 (450 mg·L–1) increased the crude fat content in ‘Shaoxing’ fruit and improved fruit quality.
Different concentrations of PBO and PP333 significantly affected the soluble protein content in ‘Shaoxing’ fruit (p < 0.05) (Fig. 3d, Table A4). Among all treatments, the soluble protein content of the fruit treated with 5 g·L–1 PBO (P2) reached a maximum value of 0.69%, which was 6.15% greater than that of the control group. For the two growth regulators, the soluble protein content first increased and then decreased as the PBO concentration increased but gradually increased as the PP333 concentration increased. Compared to the control group, the 10 g·L–1 PBO (P1) and 150 mg·L–1 PP333 (D1) treatment groups presented significantly lower soluble protein contents; however, no significant difference in the soluble protein content was recorded between the other treatment groups and the control group. Additionally, 5 g·L–1 PBO (P2) significantly increased the soluble protein content in fruit compared to the other treatments.
Different concentrations of PBO and PP333 significantly affected the reduced sugar content in ‘Shaoxing’ fruit (p < 0.05) (Fig. 3e, Table A4). Among all treatments, the reduced sugar content of fruits treated with 150 mg·L–1 PP333 (D1) reached a maximum value of 0.91%, which was 102.22% greater than that of the control group. After applying the two growth regulators, the content of reducing sugar gradually increased as the PBO concentration increased but gradually decreased as the PP333 concentration increased. The reduced sugar content of all treatment groups was greater than that of the control group. Additionally, the reducing sugar content in the 10 g·L–1 PBO (P3), 150 mg·L–1 PP333 (D1) and 300 mg·L–1 PP333 (D2) treatment groups was significantly greater than that in the control group, indicating that high concentrations of PBO (10 g·L–1) and low concentrations of PP333 (150–300 mg·L–1) could significantly increase the reducing sugar content in ‘Shaoxing’ fruit.
Different concentrations of PBO and PP333 significantly affected the cellulose content of ‘Shaoxing’ fruit (p < 0.05) (Fig. 3f, Table A4). Among all treatments, the cellulose content of the fruits treated with 10 g·L–1 PBO (P1) reached a maximum value of 2.30%, which was 49.35% greater than that of the control group. After applying the two growth regulators, the cellulose content of the fruit first increased then decreased as the PBO concentration increased but gradually increased as the PP333 concentration increased. The cellulose content of all PBO treatment groups was significantly greater than that of the control group. Additionally, the cellulose content of the fruit increased slightly in the PP333 treatment group, suggesting that the application of PBO may increase the cellulose content of the fruit.
To summarize, the application of PBO and PP333 significantly affected the nutritional quality of C. illinoinensis cv. ‘Shaoxing’ fruits. The 2 g·L–1 PBO (P1) treatment had the strongest effect on increasing the fruit cellulose content. The 5 g·L–1 PBO (P2) treatment had the greatest effect on increasing the soluble protein content of the fruits. The 10 g·L–1 PBO (P3) treatment had the greatest effect on increasing the sugar content of the fruits. The 150 mg·L–1 PP333 (D1) treatment had the greatest effect on reducing the fruit tannin content and increasing the fruit reducing sugar content.
3.4 Principal Component Analysis
The effects of different concentrations of PBO and PP333 on the growth index, leaf index, and fruit quality of ‘Shaoxing’ were determined by analyzing the variance of these indices. The results showed significant differences between the different treatments. A comprehensive evaluation and analysis of the effects of growth regulators on ‘Shaoxing’ were conducted via principal component analysis, and the comprehensive treatment effects of the two growth regulators at different concentrations were evaluated holistically.
3.4.1 Principal Component Extraction
The characteristic value and contribution percentage of principal components serve as the basis for screening principal components. In total, 16 related indices are closely associated with shoot control and fruit promotion in C. illinoinensis cv. ‘Shaoxing’, was selected for analysis. Principal components were extracted using the IBM SPSS software. Four principal components with eigenvalues exceeding 1.0 were selected as comprehensive indices to assess the overall effect of PBO and PP333 on shoot control and fruit promotion in C. illinoinensis cv. ‘Shaoxing’ (Table 1).
3.4.2 Principal Component Score
Among the comprehensive scores of the four principal components with eigenvalues greater than 1.0 (Table 2), the highest score was for the 450 mg·L–1 PP333 (D3) treatment group. The comprehensive scores for the PP333 and PBO treatment groups were higher than those of the CK. Except for the 150 mg·L–1 PP333 treatment, the scores of the PP333 treatments were greater than those of the PBO treatment. These results indicated that treatment with PBO and PP333 positively affected the growth and development of ‘Shaoxing’ fruits. The overall effect of the PP333 treatment was more favorable than that of the PBO treatment group.
4.1 Effects of PBO and PP333 on the Branch Growth of ‘Shaoxing’
The growth of plant branches can be altered by plant growth regulators. PBO and PP333 can regulate the growth of plant branches by inhibiting various processes, including the oxidation of kaurene to kaurene acid [27–29]. The results of this study showed that medium and high concentrations of PBO (5–10 g·L–1) and PP333 (300–450 mg·L–1) inhibited the excessive growth of fruiting branches and vegetative branches of C. illinoinensis cv. ‘Shaoxing’ to a certain extent promoted the periclinal division of branch cells, which was similar to the results of C. illinoinensis cv. ‘Mahan’ [18], Zamioculcasz amiifolia [30], Ficus carica [31], and others. PP333 inhibited plant cell division and the synthesis of gibberellic acid 3, thereby inhibiting the growth of the plant stem’s subapical meristem. However, PP333 did not inhibit the growth of the apical meristem but decelerated cell elongation, thus inhibiting the longitudinal growth of branches. The regulatory effect was closely related to the concentration and the species of the plant [32–34]. The results of this study indicated that an increase in the concentrations of PBO and PP333 was associated with an increase in the inhibitory effect on the elongation of the fruiting and vegetative branches of ‘Shaoxing’. Additionally, a notable increase in the diameter increment of fruiting branches was observed. Similarly, the application of PP333 resulted in a notable inhibition of the growth of Betula alnoides, with a strong correlation between the concentration and degree of inhibition [35]. Compared to the 100 mg·L–1 PP333 treatment, the 200 mg·L–1 PP333 treatment could significantly increase the branch diameter of C. illinoinensis cv. ‘Pawnee’ [9]. To summarize, the optimal concentrations of PBO and PP333 can facilitate the lateral growth of the branches of C. illinoinensis cv. ‘Shaoxing’ while simultaneously inhibiting the elongation of the internodes, thus promoting the formation of short, thick branches.
4.2 Effects of PBO and PP333 on the Physiological Indices and Nutritional Status Indices of ‘Shaoxing’ Leaves
The leaf is essential for photosynthesis, respiration, and transpiration in plants. The physiological indices and elemental composition of leaves strongly influence plant growth and reproduction [36]. The results of this study indicated that foliar application of PBO and PP333 may facilitate the accumulation of carbohydrates in the leaves of ‘Shaoxing’ plants, leading to an increase in the contents of soluble sugar, soluble protein, and starch in leaves. The contents of soluble sugar and soluble protein in leaves treated with 450 mg·L–1 PP333 (D3) were the highest, while the content of starch in leaves treated with 300 mg·L–1 PP333 (D2) was the highest. Similarly, PP333 treatment was found to significantly increase the soluble sugar content in Ligustrum lucidum leaves [37]. Compared to that in the control group, the starch content in the leaves of C. illinoinensis cv. ‘Mahan’ significantly increased in response to 250 mg·L–1 PBO treatment [18]. A high concentration of PP333 was found to significantly increase the soluble sugar content in the leaves of Mangifera indica L. [38] Plant growth regulators such as PBO and PP333 may facilitate the transformation and transfer of organic substances, including carbohydrates, to the leaves, thus increasing the adaptability of plants to environmental stress. Administering plant growth regulators may increase the concentration of nitrogen in the leaves, thereby postponing the onset of leaf yellowing and increasing the duration of the leaf photosynthetic cycle [39–41]. Nitrogen (N), phosphorus (P), and potassium (K) are essential for plant growth and development. N plays a key role in plant biology, serving as a vital component of plant proteins, nucleic acids, and chlorophyll. It is involved in several essential processes, including plant growth, photosynthesis, and metabolic activity. P is a component of ATP, and K can regulate the activity of various enzymes within plant cells, thus increasing the ability of plants to withstand drought [42,43]. Treatment with PBO and PP333 did not significantly alter the contents of N, P, and K in the leaves. However, the PP333 treatment, along with the select PBO treatment groups, delayed the decrease in N, P, and K contents in leaves at the later stage of fruit development. This result was similar to the finding that PP333 treatment can increase the contents of N, P, and K in leaves during the growth and development of Passiflora coerulea. These changes may be attributed to the indirect influence of growth regulators on the accumulation of N, P, and K through effects on plant growth, photosynthesis, and hormone metabolism [44,45]. In this study, PBO treatment promoted the accumulation of N and P in the leaves of ‘Shaoxing’, which did not favor the accumulation of K. PBO treatment may have increased the content of soluble protein in the leaves of ‘Shaoxing’, thus increasing the content of N. This finding was similar to the results reported for Amorpha fruticose and Mytilaria laosensis [46,47]. Moreover, no significant effects were detected on the P content between treatments, which may be attributed to natural biological variation in P uptake and partitioning between plant samples. Factors such as environmental conditions and plant physiological status may also contribute to this variability [48,49]. In conclusion, foliar application of PBO and PP333 can increase carbon and nitrogen nutrient levels in the leaves of C. illinoinensis cv. ‘Shaoxing’ during the middle and late stages of fruit development, and delay the decrease in N, P, and K contents in the leaves.
4.3 Effects of PBO and PP333 on the Fruit Setting Rate and Nutrients in ‘Shaoxing’ Fruit
We found that PBO, at both 200 and 10 g·L–1, significantly improved the fruit-setting rate of C. illinoinensis cv. ‘Shaoxing’. Similarly, another study showed that applying PP333 or PBO can significantly increase the fruit setting rate and fruit yield of Corylus heterophylla × Corylus avellan hybrid hazels [50], Litchi chinensis [51], Dimocarpus longan [52], Pyrus brestschneideri Rehd. [53], and other plants. This may be associated with the high concentration of PBO, which contains relatively high levels of uniconazole, trace elements, and other components that facilitate the accumulation of nutrients in the fruit [54]. The contents of crude fat, protein, reducing sugar, and cellulose are important nutrients in the kernels of C. illinoinensis cv. ‘Shaoxing’ fruits and the tannin content is proportional to the bitterness and astringency of the fruit. A reduction in tannin content results in a corresponding decrease in the perceived bitterness and astringency of the fruit [55–57]. In this study, treatment with PP333 (150–450 mg·L–1) and PBO (2–5 g·L–1) significantly reduced the tannin content of ‘Shaoxing’ kernels, and all treatments increased the crude fat, cellulose, and reducing sugar contents of the kernels. Similarly, spraying 300 mg·L–1 PP333 on leaves during the fruit growth period can significantly reduce the tannin content in the kernels of the fruits of Juglans regia L., thus increasing the overall palatability of the fruit [58]. The foliar application of PBO at the full-bloom stage can substantially increase the soluble sugar content in the fruits of Pyrus spp. [59]. The promotion of plant growth by plant growth regulators may occur due to alterations in nutrient utilization efficiency and the optimization of plant nutrient performance [60,61].
In this study, we investigated the effects of different concentrations of PBO and PP333 on branch growth, leaf physiological indices, and fruit nutritional quality in C. illinoinensis cv. ‘Shaoxing’. The results showed that treatment with 450 mg·L–1 PP333 had the most significant inhibitory effect on the growth of fruiting branches and vegetative branches. Treatment with 10 g·L–1 PBO or 150 mg·L–1 PP333 increased the diameter of branches, and treatment with 5 g·L–1 PBO significantly increased the fruit setting rate. The PBO and PP333 treatments significantly increased the content of carbon and nitrogen nutrients in leaves during the later stage of fruit development, while also delaying the decrease in the levels of N, P, and K. Additionally, PBO and PP333 increased the crude fat and cellulose contents while reducing tannin levels, thereby improving the taste of fruits. Principal component analysis showed that the overall control effect of the PP333 treatment was greater than that of the PBO treatment; applying 450 mg·L–1 PP333 resulted in the highest comprehensive score. To summarize, foliar application of 450 mg·L–1 PP333 to 6-year-old C. illinoinensis cv. ‘Shaoxing’ plants in May (the late flowering stage) and July (the rapid expansion period of the fruit) are recommended for production purposes. Additionally, irrigation and fertilization management should be optimized to facilitate greater accumulation of nutrients by plants, promote the development of short and thick branches, and improve fruit quality. This study focused on the 6-year-old C. illinoinensis cv. ‘Shaoxing’ in Hechi City, Guangxi; however, the different Carya illinoinensis varieties used in agroforestry production need to be further investigated.
Acknowledgement: None.
Funding Statement: This research was funded by the Guangxi Innovation-Driven Development Project (Grant Number AA17204058).
Author Contributions: The authors confirm contribution to the paper as follows: study conception and design: Ying Li, Zailiu Li; data collection: Ying Li, Yashi Deng; analysis and interpretation of results: Yunqi Zhang, Yilin Ou, Dongdong Li; draft manuscript preparation: Yunqi Zhang, Yashi Deng, Daocheng Ma; resources and supervision: Zailiu Li, Weidong Xie. All authors reviewed the results and approved the final version of the manuscript.
Availability of Data and Materials: All of the data developed or examined during this investigation are available in this article and its supplementary materials, or they can be provided at reasonable request.
Ethics Approval: Not applicable.
Conflicts of Interest: The authors declare no conflicts of interest to report regarding the present study.
References
1. Deng L, Pan H, Ma T, Wei Z, Wang F, Qin F, et al. Growing performance and quality characteristics of six thin-shelled pecan varieties planted in Hechi city of Guangxi. Nonwood For Res. 2022;40:241–8. [Google Scholar]
2. Taipina MS, Lamardo LCA, Rodas MAB, del Mastro NL. The effects of gamma irradiation on the vitamin E content and sensory qualities of pecan nuts (Carya illinoensis). Radiat Phys Chem. 2009;78:611–3. doi:10.1016/j.radphyschem.2009.03.019. [Google Scholar] [CrossRef]
3. Chang J, Ren H, Yao X, Yang S, Zhang X, Zhang C, et al. A comparative analysis of nutritional components and fatty acid composition of 41 pecan varieties. J Southwest Univ. 2021;43:20–30. [Google Scholar]
4. Zhang J, Li Y, Zhai M, Wang T, Wang G, Guo Z. Problems in the planting of pecan “Mahan” and its key cultivation techniques. North Hortic. 2021;7:173–4 (In Chinese). [Google Scholar]
5. Islam S, Mohammad F. Triacontanol as a dynamic growth regulator for plants under diverse environmental conditions. Physiol Mol Biol Plants. 2020;26:871–83. doi:10.1007/s12298-020-00815-0. [Google Scholar] [PubMed] [CrossRef]
6. Desta B, Amare G. Paclobutrazol as a plant growth regulator. Chem Biol Technol Agric. 2021;8:1. doi:10.1186/s40538-020-00199-z. [Google Scholar] [CrossRef]
7. Soumya PR, Kumar P, Pal M. Paclobutrazol: a novel plant growth regulator and multi-stress ameliorant. Indian J Plant Physiol. 2017;22:267–78. doi:10.1007/s40502-017-0316-x. [Google Scholar] [CrossRef]
8. Su Y, Li P, Yang J, Wang L, Wang S, Xue H. Study on the bearing tree overgrowth control of Korla pear. North Hortic. 2024;3:17–24. [Google Scholar]
9. Feng G, Li X, Deng Q, Chen W, Peng F. Effects of plant growth regulators on branch growth and accumulation of carbon and nitrogen metabolites in leaves of pecan cultivar “Bonnie”. J Plant Resour Environ. 2018;27:49–55. [Google Scholar]
10. Mog B, Janani P, Nayak MG, Adiga JD, Meena R. Manipulation of vegetative growth and improvement of yield potential of cashew (Anacardium occidentale L.) by Paclobutrazol. Sci Hortic. 2019;257:108748. doi:10.1016/j.scienta.2019.108748. [Google Scholar] [CrossRef]
11. Ju S, Xu D, Zhan C, Ji L, Yin T, Li Z, et al. Influence of paclobutrazol on the growth and photosynthesis of seedlings. J Hortic Res. 2019;27:21–30. doi:10.2478/johr-2019-0003. [Google Scholar] [CrossRef]
12. Yuan Y, Wang L, Yuan X, Lu Y, Zhong Q, Li Y. Response of foliar carbon and nitrogen nutrition of female Taxus chinensis var. mairei to spraying plant growth regulators. J Cent South Univ For Technol. 2020;40:87–93 (In Chinese). [Google Scholar]
13. Qian H, Yang L, Huang C, Wang Z, Yang J, Wu A. Effects of combined administration of naphthalene acetic acid with paclobutrazol on growth and biomass allocation of carya illinoensis ‘Pawnee’ container seedling. J Northeast For Univ. 2023;51:64–9. [Google Scholar]
14. Ajmi A, Larbi A, Morales M, Fenollosa E, Chaari A, Munné-Bosch S. Foliar paclobutrazol application suppresses olive tree growth while promoting fruit set. J Plant Growth Regul. 2020;39:1638–46. doi:10.1007/s00344-020-10188-z. [Google Scholar] [CrossRef]
15. Li S, Wang X, Chen B, Chai Z, Shen X, Ding K, et al. Effects of plant growth regulators on yield and quality of Korla fragrant pear. Res Agric Mod. 2016;37:374–80. [Google Scholar]
16. Zhu X, Zhou Z. Effects of spraying PBO on fruit traits of Kyoho grape. Mod Hortic. 2016;5:20–1. [Google Scholar]
17. Liu L, Gao D, Wei Z, Shi C, Xu Y. Effects of prohexadione calcium on growth and fruit quality of Fuji apple. J Fruit Sci. 2021;38:1084–91. [Google Scholar]
18. Zhang X, Xu Y, Li Y, Xu Y, Zhai M. Effect of foliar application of DA-6, PBO and 6-BA on the growth of Carya illinoensis. Chin Agric Sci Bull. 2015;31:13–7 (In Chinese). [Google Scholar]
19. Jia X, Luo H, Zhai M, Li Y, Guo Z, Qiao Y. Dynamic analysis of pecan (Carya illinoensis ‘Pawnee’) nut development. J Fruit Sci. 2015;32:247–53 (In Chinese). [Google Scholar]
20. Feng Y, Cao D, Guo Y. Determination of polysaccharides in rhizoma Bergeniae scopulosae by anthrone-sulfuric acid colorimetric method. J Northwest Univ Nat Sci Ed. 2013;43:921–3. [Google Scholar]
21. Deng L, Pan X, Sheng J, Shen L. Optimization of experimental conditions for the determination of water soluble protein in apple pulp using coomassie brilliant blue method. Food Sci. 2012;33:185–9. [Google Scholar]
22. Bao S. Soil agrochemical analysis. China: China Agriculture Press; 2000. [Google Scholar]
23. Wang Y, Wang F, Wan T. Determination of persimmon tannin in immature fruit by spectrophotometry. Chin J Anal Lab. 2011;30:16–8. [Google Scholar]
24. Brown RH, Mueller-harvey I. Evaluation of the novel soxflo technique for rapid extraction of crude fat in foods and animal feeds. J AOAC Int. 1999;82:1369–74. [Google Scholar] [PubMed]
25. Yang Q, Zhou Q, Wu S, Wang Y, Zhang M, Hong Y, et al. Comparison of 3, 5-dinitrosalicylic acid method and enzymatic method in the determination of sugar and sucrose content in sweet corn. J Agric Sci Technol. 2017;19:125–31. [Google Scholar]
26. Li C, Yao X, Wang K, Gu X. A study on simplifying the indices of the clone fruit characters of Carya illinoinensis. Acta Agric Univ Jiangxiensis. 2011;33:696–700. [Google Scholar]
27. Echegaray ER, Cloyd RA. Effects of reduced-risk pesticides and plant growth regulators on rove beetle (Coleoptera: Staphylinidae) adults. J Econ Entomol. 2012;105:2097–106. doi:10.1603/EC12244. [Google Scholar] [PubMed] [CrossRef]
28. Shahsavari E, Maheran AA, Akmar ASN, Hanafi MM. The effect of plant growth regulators on optimization of tissue culture system in Malaysian upland rice. Afr J Biotechnol. 2010;9:2089–94. [Google Scholar]
29. Waqas M, Khan AL, Kamran M, Hamayun M, Kang S-M, Kim Y-H, et al. Endophytic fungi produce gibberellins and indoleacetic acid and promotes host-plant growth during stress. Molecules. 2012;17:10754–73. doi:10.3390/molecules170910754. [Google Scholar] [PubMed] [CrossRef]
30. Jiang Y, Peng Y, Li Z, Wu Z, Ren S. Effects of paclobutrazol,uniconazole and chlorcholinchlorid on dwarfing of Zamioculcasz amiifolia. Acta Hortic Sin. 2010;37:823–8. [Google Scholar]
31. Su Y, Yu L, Liu H, Wu C, Ma Q, Wang Z. Effects of plant growth regulators on growth and fruit quality of facility figs. Nonwood For Res. 2024;42:284–93. [Google Scholar]
32. Chan Z. Expression profiling of ABA pathway transcripts indicates crosstalk between abiotic and biotic stress responses in Arabidopsis. Genomics. 2012;100:110–5. doi:10.1016/j.ygeno.2012.06.004. [Google Scholar] [PubMed] [CrossRef]
33. Frost RG, West CA. Properties of kaurene synthetase from Marah macrocarpus. Plant Physiol. 1977;59:22–9. doi:10.1104/pp.59.1.22. [Google Scholar] [PubMed] [CrossRef]
34. Kulkarni MG, Stirk WA, Southway C, Papenfus HB, Swart PA, Lux A, et al. Plant growth regulators enhance gold uptake in Brassica juncea. Int J Phytoremediation. 2013;15:117–26. doi:10.1080/15226514.2012.683207. [Google Scholar] [PubMed] [CrossRef]
35. Wang H, Shen W, Guo J, Wang C, Zhao Z. Regulating growth of Betula alnoides Buch. Ham. ex D. Don seedlings with combined application of paclobutrazol and gibberellin. Forests. 2019;10:378. doi:10.3390/f10050378. [Google Scholar] [CrossRef]
36. Xu W, Wang X, Wang W, Li J. Screening of substrate formulation for floating seedling rearing of tobacco. J Gansu Agric Univ. 2022;57:131–9. [Google Scholar]
37. Zhou G, Liu C, Hu G. The drought resistance of paclobutrazol on Ligustrum lucidum cv. North Hortic. 2010;21:88–90. [Google Scholar]
38. de Souza MA, Mésquita AC, Simões WL, Ferreira KM, Araujo EFJ. Physiological and biochemical characterization of mango tree with paclobutrazol application via irrigation. Pesqui Agropecuária Trop. 2016;46:442–9. doi:10.1590/1983-40632016v4642829. [Google Scholar] [CrossRef]
39. Niinemets Ü. Global-scale climatic controls of leaf dry mass per area, density, and thickness in trees and shrubs. Ecology. 2001;82:453–69. doi:10.1890/0012-9658(2001)082[0453:GSCCOL]2.0.CO;2. [Google Scholar] [CrossRef]
40. Wang J, Jia L, Shao J, Sun J, Peng J. Changes of carbohydrate content and C/N in branches and leaves during flower bud differentiation of Tianhong 2 apple. J Henan Agric Sci. 2024;53:124–32. [Google Scholar]
41. Westoby M, Falster DS, Moles AT, Vesk PA, Wright IJ. Plant ecological strategies: some leading dimensions of variation between species. Annu Rev Ecol Evol Syst. 2002;33:125–59. doi:10.1146/ecolsys.2002.33.issue-1. [Google Scholar] [CrossRef]
42. Huang A, Lu X, Wang Z, Yang S, Bo W, Wu N. Effects of fertilizer application on nutrient utilization and economic traits of Camellia oleifera. J For Environ. 2023;43:642–50. [Google Scholar]
43. Osaki M, Iyoda M, Tadano T. Ontogenetic changes in the contents of ribulose-1,5-bisphosphate carboxylase/oxygenase, phosphoenolpyruvate carboxylase, and chlorophyll in individual leaves of maize. Soil Sci Plant Nutr. 1995;41:285–93. doi:10.1080/00380768.1995.10419585. [Google Scholar] [CrossRef]
44. Du Y, Li J, Li D, Li J, Cun D, Dong J, et al. Effect of different exogenous substances on growth and mineral nutrient content of Passiflora coerulea. Nonwood For Res. 2022;40:196–204. [Google Scholar]
45. Gill K, Kumar P, Negi S, Sharma R, Joshi AK, Suprun II, et al. Physiological perspective of plant growth regulators in flowering, fruit setting and ripening process in citrus. Sci Hortic. 2023;309:111628. doi:10.1016/j.scienta.2022.111628. [Google Scholar] [CrossRef]
46. Liu Y, Li S, Sun H, Song H. Effect of paclobutrazol on growth of Amorpha fruticosa. North Hortic. 2016;13:57–61. [Google Scholar]
47. Zhu M, Yang J, Zou W, Yu N, Dong M, Li R. Effects of plant growth retardants on growth and nutrient elements of Mytilaria laosensis seedlings. J Southwest AF Univ. 2022;50:43–53. [Google Scholar]
48. Pant BD, Burgos A, Pant P, Cuadros-Inostroza A, Willmitzer L, Scheible W-R. The transcription factor PHR1 regulates lipid remodeling and triacylglycerol accumulation in Arabidopsis thaliana during phosphorus starvation. J Exp Bot. 2015;66:1907–18. doi:10.1093/jxb/eru535. [Google Scholar] [PubMed] [CrossRef]
49. Veneklaas EJ, Lambers H, Bragg J, Finnegan PM, Lovelock CE, Plaxton WC, et al. Opportunities for improving phosphorus-use efficiency in crop plants. New Phytol. 2012;195:306–20. doi:10.1111/j.1469-8137.2012.04190.x. [Google Scholar] [PubMed] [CrossRef]
50. Shan X, Song F, Shi Y, Wang H, Guli M, Tao X. Effects of spraying plant growth regulators on photosynthetic characteristics traits and fructify growth of the major hybrid hazel. Xinjiang Agric Sci. 2015;52:1063–70. [Google Scholar]
51. Tang Z, Jiang Y, Gan L, Xiong X. Effects of Ethrel and PP333 on endogenous hormones and flower bud differentiation of Jizui litchi. J Hunan Agric Univ. 2006;32:135–40. [Google Scholar]
52. Tian S, Xue J, Ma X, Wang Y, Chen Q, Li T. Effects of medicament transfusion following trunks on florescence of longan. Guihaia. 2016;36:1353–7. [Google Scholar]
53. Kuerban Y, Muerta Z, Qin W. Effect of compound reagent “M” and PBO on the yield and fruit quality of Korla fragrant pear. Xinjiang Agric Sci. 2017;54:626–33. [Google Scholar]
54. Rehman M, Singh Z, Khurshid T. Pre-harvest spray application of prohexadione-calcium and paclobutrazol improves rind colour and regulates fruit quality in M7 Navel oranges. Sci Hortic. 2018;234:87–94. doi:10.1016/j.scienta.2018.02.018. [Google Scholar] [CrossRef]
55. Luo X, Liu M, Qi X, Xu Q, Chen X. Molecular research progress in fruit astringent. Mol Plant Breed. 2013;11:647–56. [Google Scholar]
56. Robbins KS, Gong Y, Wells ML, Greenspan P, Pegg RB. Investigation of the antioxidant capacity and phenolic constituents of U.S. pecans. J Funct Foods. 2015;15:11–22. doi:10.1016/j.jff.2015.03.006. [Google Scholar] [CrossRef]
57. Zheng L, Nie J, Li M, Kang Y, Kuang L, Ye M. Study on screening of taste evaluation indexes for apple. Sci Agric Sin. 2015;48:2796–805. [Google Scholar]
58. Xu Y, Song Y, Jiang Y, Han X, Zhang R, Zhang S, et al. Effects of DPC and PP333 on walnut growth and quality of nuts. J Henan Agric Sci. 2020;49:108–15. [Google Scholar]
59. Yang X, Li R, Huang D, Yang Z, Hu H. Effects of PBO and Fuxing treatments on calyx shedding and fruit quality of “Xiangnan” pear. South China Fruits. 2014;43:97–9. [Google Scholar]
60. Rajabi Hamedani S, Rouphael Y, Colla G, Colantoni A, Cardarelli M. Biostimulants as a tool for improving environmental sustainability of greenhouse vegetable crops. Sustainability. 2020;12:5101. doi:10.3390/su12125101. [Google Scholar] [CrossRef]
61. Silva TV, Melo HCD, Tarazi MFDA, Junior LCC, Campos LFC, Nascimento ADR. Biostimulants isolated or integrated with paclobutrazol as tomato development triggering factors. Emir J Food Agric. 2020;255. doi:10.9755/ejfa.2020.v32.i4.2091. [Google Scholar] [CrossRef]
Appendix A
Figure A1: Carya illinoinensis cv. ‘Shaoxing’ plant and foliar spray treatment
Note: (a) 6-year-old Carya illinoinensis cv. ‘Shaoxing’ plant. (b) The leaves of ‘Shaoxing’ before foliar spray treatment. (c) The leaves of ‘Shaoxing’ after foliar spray treatment.
Figure A2: PBO and PP333 wettable powder
Note: (a) PBO wettable powder was sourced from Huaye Agricultural Technology Co., Ltd., China (Technical indicators: medium element content (Ca + Mg) ≥ 10.0%). (b) Paclobutrazol (PP333) wettable powder was sourced from Guoguang Agricultural Chemical Co., Ltd., China (containing 15% active ingredients).
Cite This Article
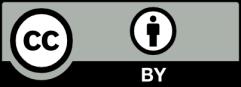
This work is licensed under a Creative Commons Attribution 4.0 International License , which permits unrestricted use, distribution, and reproduction in any medium, provided the original work is properly cited.