Open Access
ARTICLE
Effects of Nitrogen Application Rate on Yield and Quality of Different Genotypes of Foxtail Millet
1 Center for Agricultural Genetic Resources Research, Shanxi Agricultural University, Taiyuan, 030031, China
2 College of Agriculture, Shanxi Agricultural University, Jinzhong, 030801, China
* Corresponding Author: Xiaoning Cao. Email:
(This article belongs to the Special Issue: Integrated Nutrient Management in Cereal Crops)
Phyton-International Journal of Experimental Botany 2024, 93(12), 3487-3507. https://doi.org/10.32604/phyton.2024.057945
Received 31 August 2024; Accepted 18 November 2024; Issue published 31 December 2024
Abstract
In order to elucidate the response mechanism of yield and quality of different genotypes of foxtail millet to nitrogen application. In this study, plant physiology and metabolomics were used to study the effects of different amounts of nitrogen (0, 75, 150, 225 and 300 kg hm−2) on agronomic characters, yield and quality of Jingu 21 and Zhangza 10. The results showed that with the increase of nitrogen application, the plant height of different genotypes of foxtail millet increased gradually, and the content of stem diameter, yield, protein, fat, lysine, phenylalanine, isoleucine, arginine, aspartic, glutamic, glycine, and proline content of different genotypes of foxtail millet showed an increasing and then decreasing trend. The highest yield was recorded in Jingu 21 at 150 kg hm−2, and the highest yield was recorded in Zhangza 10 at 225 kg hm−2 of nitrogen application. Yield of Jingu 21 was positively correlated with protein and tryptophan content (r = 0.91). The yield of Zhangza 10 was positively correlated with fibre content (r = 0.89). The protein content of different genotypes of foxtail millet were negatively correlated with the peak viscosity (PV), trough viscosity (TV) and breakdown value (BD). The results of this study clarify that the optimal nitrogen application of Jinguu 21 was 150 kg hm−2, and that of Zhangza 10 was 225 kg hm−2. The regulation effect of nitrogen on foxtail millet was clarified, which laid the theoretical and technical foundation for foxtail millet cultivation with high yield and high quality.Keywords
Foxtail millet has been cultivated in China for 6000–7000 years and is rich in beneficial vitamins, proteins, fats, and other nutrients, which have a variety of nutritional and medicinal values [1]. China currently preserves 70% of the world’s foxtail millet germplasm resources [2]. Foxtail millet is a drought-resistant and barren-tolerant environmentally friendly crop [3], which has been gradually developed as a new C4 model plant [4]. This is of great significance to dryland ecological agriculture, food production diversity and food security [5].
Nitrogen is a key factor affecting crop growth and development [6]. Zhou et al. showed that nitrogen application could increase the grain yield and the total above-ground nitrogen uptake at the maturity of winter wheat [7]. Reasonable nitrogen application rate can improve the plant height and stem diameter of quinoa [8], as well as the spike length and spike branch of rice plants [7]. After nitrogen application, the number of rice panicles and grains per panicle increased, and the population quality improved significantly [9]. At the same time, it can promote the accumulation of assimilates in early and late rice [10] and the transport of dry matter from vegetative organs to grains [11], thus increasing the yield. However, when nitrogen application exceeds a certain range, crop yield decreases rather than increases [12,13]. The results showed that thousand-grain weight and grain per spike of wheat increased first and then decreased after nitrogen application [14]. Zhang et al. showed that the increase in grain yield was small when nitrogen application was greater than 90 kg hm−2 [15], and others showed that the increase in hybrid foxtail millet yield was not significant when nitrogen application was more than 200 kg hm−2 [16].
Reasonable nitrogen fertilizer regulation can increase crop quality [17]. Proper increase of nitrogen supply can promote nitrogen absorption by plants, improve nitrate reductase activity, and thus promote NO−3 absorption and transformation, provide more amino acid and protein supply for organ building [18], promote wheat starch accumulation and increase protein content [19]. Liu et al. showed that with the increase of nitrogen application, the protein content in rice grain was significantly increased, and the starch content was significantly decreased [20]. Hu et al. showed that with the increase in nitrogen application, the amino acid content of rice generally showed an increasing trend and with glutamic acid content being the highest and methionine content being the lowest [21]. Yang et al. showed that the total amino acid content of summer peanuts increased first and then stabilized gradually after nitrogen application [22]. High nitrogen levels will reduce starch synthesis rate, increase the proportion of amylopectin short branch and amylose long branch [23], reduce amylopectin content and inhibit FV and SB and affect gelatinization quality [17]. The rise in production costs and environmental pollution have a negative impact on the sustainable development of agriculture [24]. Emissions from the production and application of nitrogen fertilizer can have harmful effects on ecosystems [25].
Currently, the application of nitrogen fertiliser to foxtial millet production is mainly focused on agronomic characters [9], quality [19], nitrogen utilisation [17] and yield, photosynthetic characteristics [15,16] and water utilisation efficiency [16]. The effect of nitrogen application on amino acid content and RVA of foxtail millet has been less studied. In this article, different genotypes of foxtail millet were used as materials, and different levels of nitrogen application were set up. Plant physiology and non-targeted metabolomics were used to study the effects of nitrogen application on agronomic characters, quality, amino acid content and RVA of different genotypes of foxtail millet. The regulation effect of nitrogen on the quality formation and nitrogen metabolism of foxtail millet was clarified, and the optimal nitrogen application rate was selected. To establish a theoretical foundation for the cultivation of foxtail millet that achieves both high yield and superior quality.
The experiment was conducted in 2020 and 2021 at Dingxiang Experimental Base, Agricultural Gene Resources Research Center of Shanxi Agricultural University, and the air temperature and precipitation during the reproductive period of the foxtail millet are shown in Fig. 1. The experimental materials were provided by Agricultural Gene Resources Research Center of Shanxi Agricultural University (Taiyuan, China). The test varieties were selected from Jingu 21 and Zhangza 10. Jingu 21 is the most planted area of conventional foxtail millet varieties in Shanxi Province and Zhangza 10 is a high-quality hybrid foxtail millet. Based on the research of Zhang et al. [15] and previous experiments, the following treatments were set up: Jingu 21: 0 kg hm−2 (JGF1), 75 kg hm−2 (JGF2), 150 kg hm−2 (JGF3), 225 kg hm−2 (JGF4), 300 kg hm−2 (JGF5); Zhangza 10: 0 kg hm−2 (ZZF1), 75 kg hm−2 (ZZF2), 150 kg hm−2 (ZZF3), 225 kg hm−2 (ZZF4), 300 kg hm−2 (ZZF5). The experimental plots were organized into randomized block designs with each treatment replicated thrice for accuracy and reliability, plot size of 5 m × 6 m, density of 300,000 plants hm−2, row spacing of 50 cm, and nitrogen fertilizer of urea (N: 46.4%). The seeds were sown on 23th May in 2020 and 25th May in 2021, and the experimental crops were cultivated in strict adherence to the local agricultural technical protocols.
Figure 1: Air temperature and precipitation during foxtail millet growth period from June to October in 2020 and 2021
2.2 Indicators and Methods of Determination
2.2.1 Determination of Agronomic Characters
After maturity, 10 foxtail millet plants were taken from each plot for testing, and agronomic characters such as plant height, stem diameter, pitch number, and spike weight were measured. The measurement method was as follows: the length from the tillering node to the longest leaf tip of the plant was measured by a tape measure to be the plant height (cm); the diameter of the middle stem of the third section of the main stem of the millet was measured by a vernier caliper as the diameter of the millet stem (mm); the number of internodes from the bottom to the top stem of foxtail millet is the number of nodes; the spikes were cut from the stem of the spike and weighed by the analytical balance as the spike weight (g). The investigation standards of agronomic characters refer to foxtail millet Germplasm Resources Description Specification and Data Standard [26].
Harvested by hand at maturity, removing 2 rows of side rows, harvested, threshed, and the seeds weighed for yield after natural air drying.
2.2.3 Determination of Protein, Fat, Starch, and Fiber Content
The protein components in the sample were separated following the method of Wieser et al. [27]. The absorbance of the sample at 595 nm was determined by ultraviolet spectrophotometer. The standard curves of different concentrations were fitted and the content of each protein component was calculated based on the formula: protein content (mg/g) = C × V/M. Here, C is the result calculated based on the standard curve; M is the actual weighed mass; V is the total volume of the extract.
The fat content was determined using the Soxhlet extraction method, in accordance with the national standard GB 5009.5-2016 [28]. A thoroughly mixed sample weighing between 2 to 5 g was placed into a filter paper cartridge. This cartridge was then positioned in the extraction cylinder of the Soxhlet extractor. Anhydrous ether or petroleum ether was added until it filled two-thirds of the volume of the receiving flask, introduced from the upper end of the condenser tube of the extractor. The mixture was heated on a water bath, allowing for extraction at a rate of 6 to 8 cycles per hour for a duration of 6 to 10 h. At the conclusion of the extraction process, a drop of the extraction solution was obtained using a frosted glass rod. When the solvent in the receiving flask remained at approximately 1 to 2 mL, it was evaporated on the water bath and subsequently dried at 100°C ± 5°C for 1 h. The sample was then cooled in a desiccator for 30 min before being weighed. This process was repeated until a constant weight was achieved.
In the formula: X—fat content in the sample (%);
m1—quality of bottles and fats received (g);
m0—quality of the receiving bottle (g);
m2—quality of sample (g).
The starch content was determined by visible spectrophotometry according to Zhuang et al. [29] using the starch content detection kit provided by Solarbio Company. 0.05 g of the sample was pulverized, 1 mL of the reagent was added and transferred to the EP tube. 80°C water bath for 30 min, centrifuge at 3000 g for 5 min, retain precipitate, then add 0.5 mL double distilled water water bath for gelatinization for 15 min, add reagent 2 after cooling, water bath for 15 min, shake 3–5 times, centrifuge at 8000 g for 15 min after cooling, take 0.2 mL of supernatant and 1 mL of working solution to EP tube, water bath at 95°C for 10 min, measure the absorbance value at 620 nm after cooling, and then draw a curve to calculate the starch content.
The fiber content was measured according to the national GB/T 5009.10-2003 [30]. Colorimetry was performed on a spectrophotometer at a wavelength of 620 urn, the extinction value was measured with the sample, and the corresponding cellulose content was found on the curve, and then all in accordance with the following formula calculates the cellulose content in the sample.
In the formula: A—the cellulose content value found on the curve (μg);
B—sample weight (g);
10−6—coefficient for converting μg into g;
C—sample dilution factor;
X—cellulose content in the sample (%).
2.2.4 Determination of Amino Acid Content
After collection, the samples were sent to Sanshu Biotechnology Co, Ltd., Shanghai, China for analysis. Various free amino acids were extracted and measured using the method of Kovacs et al. [31]. The instrument systems used for data acquisition mainly include ultra-high performance liquid chromatographs (Vanquish, UPLC, Thermo, Carlsbad, CA, USA) and high-resolution mass spectrometers (QExactive, Thermo, USA). The parameters of the liquid chromatography were set as follows: The column was Waters BEH C18 (50 * 2.1 mm, 1.7 μm). In the mobile phase, phase A is ultra-pure water containing 0.1% formic acid, and phase B is acetonitrile containing 0.1% formic acid. The flow rate was set to 0.5 mL/min. The column temperature was maintained at 55°C. The sample size was 1 μL.
The quantitative analysis was carried out by external standard method, the fitting curve was constructed by standard substances of different concentrations, and the data provided by the instrument was processed. Amino acid content (ug/g) = (C × V × F × Mw)/M. Molar content (nmol/mg) = (C × V × F)/M. Mw is the molecular weight of the amino acid. C is the concentration of the sample read by the instrument, in nmol/mL. V is the final constant volume of the sample, the unit is mL. F is the dilution ratio of the sample. M is the weighing mass of the sample in mg.
The RVA data were measured according to Qi et al. [32]. RVA data properties of starch were evaluated using (RVA-4) (Perten Instruments Australia, Macquarie Park, NSW, Austrilia). The starch sample was mixed with 25 mL distilled water to bring the total weight to 28 g. The starch was balanced at 50°C for 1 min, then heated at 12 °C/minute to 95°C and kept at 95°C for 2.5 min. It is then cooled to 50°C at a rate of 12 °C/min and held at 50°C for 2 min. Record peak viscosity (PV), trough viscosity (TV), breakdown value (BD), final viscosity (FV), setback (SB), peak time (PT), and pasting temperature (PTM).
SPSS 27.0 and Excel 2022 software were used for statistical analysis of experimental data, including one-way ANOVA, principal component analysis, correlation analysis and cluster analysis. At the level of p < 0.05, the significant difference between the treatment groups was tested by the least significant difference method. In addition, the Origin 2024 software is used for drawing. All indicator data are presented as three repeated mean ± standard errors.
4.1 Effects of Nitrogen Application Rate on Agronomic Characters of Different Foxtail Millet Genotypes
Different nitrogen application rates can have a significant effect on the agronomic characters of foxtail millet (Table 1). With the increase of nitrogen application rate, the plant height of different genotypes of foxtail millet showed a gradual upward trend in the two years and the JGF5 and ZZF5 treatments reached the maximum. In 2020, the stem diameter of Jingu 21 showed a gradual upward trend and the JGF5 treatment was the largest. The stem diameter of Zhangza 10 showed a trend of increasing first and then decreasing in both years and the ZZF4 treatment was the largest. There was no significant difference in the number of foxtail millet nodes between different genotypes in two years. In 2020, the spike weight of Jingu 21 showed a gradual upward trend and the JGF5 was the largest. In 2021, the spike weight of Jingu 21 showed a trend of increasing first and then decreasing and the JGF4 was the largest. The spike weight of Zhangza 10 showed a trend of increasing first and then decreasing in both years and the ZZF4 was the largest.
4.2 Effect of Nitrogen Application Rate on Yield of Different Genotypes of Foxtail Millet
Different nitrogen application rates can have a significant effect on the yield of foxtail millet (Fig. 2). The yield of different genotypes of foxtail millet increased first and then decreased with the increase of nitrogen application rate. In 2020 and 2021, the highest yield of JGF3 treatment was 6005.75 and 6109.8 kg hm−2, respectively, which increased by 13.07% and 11.57% compared with the control. The highest yield of ZZF4 treatment was 7083.5 and 6892.95 kg hm−2, respectively, which increased by 11.46% and 6.82% compared with the control.
Figure 2: Effects of different nitrogen application rates on foxtail millet yield of different genotypes of foxtail millet. (A) Yeld of different genotypes of foxtail millet in 2020 (B) Yield of different genotypes of foxtail millet in 2020
4.3 Effects of Nitrogen Application Rate on Quality of Different Genotypes of Foxtail Millet
Different nitrogen application rates can have a significant effect on the quality of foxtail millet (Table 2). With the increase of nitrogen application rate, the protein and fat content of different genotypes of foxtail millet increased first and then decreased. The protein content of JGF3 treatment was the highest in both years and the protein content of ZZF4 treatment was the highest in both years. The fat content of JGF4 and ZZF4 treatments was the highest in both years. The starch content of JGF5 treatment was the highest in two years and the starch content of ZZF3 treatment was the highest in two years. In 2020, the fiber content of JGF4 treatment was the highest. In 2021, the fiber content of Jingu 21 was the highest without nitrogen application and the fiber content of ZZF4 treatment was the highest in both years.
4.4 Effect of Nitrogen Application Rate on Amino Acid Content of Different Genotypes of Foxtail Millet
Different nitrogen application rates can have a significant effect on the amino acid content of foxtail millet (Tables 3 and 4). With the increase of nitrogen application rate, the lysine, phenylalanine, isoleucine, arginine, aspartic, glutamic, glycine and proline content of different genotypes of millet showed a trend of increasing first and then decreasing in two years. Compared with the control, the contents of essential amino acids (threonine, lysine, valine, tryptophan, isoleucine, phenylalanine, methionine) treated by JGF4 and ZZF4 increased by 3.53% and 3.56% (Jingu 21) and 1.57% and 1.19% (Zhangza 10), respectively. The content of non-essential amino acids (aspartic, glycine, arginine, proline, glutamic, serine, histidine, cysteine) treated by JGF4 increased by 4.02% and 4.05% in two years, respectively. In 2020, the content of non-essential amino acids treated by ZZF4 increased by 1.33% and the content of non-essential amino acids in Zhangza 10 was the highest without nitrogen application in 2021. The contents of 15 amino acids in the grains of different genotypes were different. The highest was glutamic and proline content, followed by aspartic acid and serine content, and the lowest was lysine content.
4.5 Effects of Nitrogen Application Rate on RVA of Different Genotypes of Foxtail Millet
The amount of fertilizer can have a significant effect on the RVA of foxtail millet (Fig. 3). With the increase of nitrogen application rate, the PV, TV, BD and FV of Jingu 21 showed a trend of decreasing first and then increasing and JGF3 treatment was the lowest. The PV, TV, BD and FV of Zhangza 10 decreased gradually with the increase of nitrogen application rate, and ZZF5 treatment was the lowest. There was no significant difference in the SB and PT of different genotypes of foxtail millet. The PTM of Jingu 21 showed a trend of increasing first and then decreasing and the PTM of Zhangza 10 showed a trend of decreasing first and then increasing.
Figure 3: Effect of nitrogen application on RVA of different genotypes of foxtail millet. (A) PV (B) TV (C) BD (D) FV (E) SB (F) PT (G) PTM
4.6 Correlation Analysis and Principal Component Analysis
The correlation analysis of millet quality index and amino acid index (Fig. A1) showed that protein content was significantly positively correlated with most amino acid content (Fig. A1A), among which the positive correlation with glutamic content was the strongest (r = 0.98), and the negative correlation with starch content was the strongest (r = −0.97). Starch content was significantly negatively correlated with other amino acid contents except cysteine content. Starch content had the strongest positive correlation with PV (r = 0.91) and the strongest negative correlation with glutamate content (r = −0.97). The yield had the strongest positive correlation with the protein content and tryptophan content (r = 0.91), and the strongest negative correlation with starch content (r = −0.89).
Protein content was positively correlated with most amino acids (Fig. A1B). Glutamic content had the strongest positive correlation (r = 0.96) and the strongest negative correlation with starch content (r = −0.75). The starch content had the strongest positive correlation with cysteine content (r = 0.59) and the strongest negative correlation with proline content (r = −0.79). The yield had the strongest positive correlation with fiber content (r = 0.89) and the strongest negative correlation with cysteine content (r = −0.75).
Principal component analysis was performed on the quality index and amino acid content of foxtail millet (Fig. 4). The results showed that in 2020, the contribution rates of PC1 and PC2 of Jingu 21 accounted for 57.0% and 20.2%, respectively, and the cumulative contribution rate was 77.2% (Fig. 4A). The contribution rate of Zhangza 10 was 68.2% and 16.9%, respectively, and the cumulative contribution rate was 85.1% (Fig. 4B). The contents of cysteine, tryptophan, methionine, serine, phenylalanine, aspartic acid, threonine and protein in Jingu 21 and Zhangza 10 were significantly positively correlated with PC1 and PC2.
Figure 4: Principal component analysis of grain quality and amino acid content of different genotypes. (A) 2020 Jingu 21 (B) 2020 Zhangza 10 (C) 2021 Jingu 21 (D) 2021 Zhangza 10
In 2021, the contribution rate of PC1 and PC2 of Jingu 21 accounted for 54.9% and 25.9%, respectively, and the cumulative contribution rate was 80.8% (Fig. 4C). The contribution rate of Zhangza 10 was 59.7% and 26.6%, respectively, and the cumulative contribution rate was 86.3% (Fig. 4D). Aspartic, proline, isoleucine, threonine and glutamic content in Jingu 21 and Zhangza 10 were significantly positively correlated with PC1 and PC2.
The agronomic characters, quality, amino acid content and RVA indexes of different genotypes of foxtail millet were analyzed by cluster analysis (Fig. 5). The results show that when the distance between classes is 15. The above indicators are divided into five categories. The first category included aspartic content, isoleucine content, fat content, histidine content, PT, valine content, tryptophan content, proline content, phenylalanine content, glutamic content and protein content. The second category included node number, spike weight, lysine content, glycine content, arginine content, stem diameter, yield and fiber content. The third category includes starch content. The fourth category includes PV, BD and TV. The fifth category included cysteine content, methionine content, serine content, FV, SB, plant height and tryptophan content.
Figure 5: Cluster analysis of agronomic characters, yield, quality, amino acid content and RVA of different genotypes of foxtail millet
5.1 Effects of Nitrogen Fertilizer on Agronomic Traits and Yield
Nitrogen application effectively maintained the number of spikes harvested per unit area of wheat [33]. It increased the spike weight of wheat by 42.6% [34] and increased the plant height and stem diameter of quinoa [8]. In this study, it was found that the plant height of different genotypes of foxtail millet increased in two years after nitrogen application. The stem diameter of different genotypes of foxtail millet was thickest with nitrogen application of 225 kg hm−2 and the spike weight of Zhangza 10 was heaviest with nitrogen application of 225 kg hm−2. The heaviest spike weight of Jingu 21 at 300 kg hm−2 of nitrogen application in 2020 and 225 kg hm−2 of nitrogen application in 2021 may be due to the lower precipitation in 2020, which resulted in damage to the floral organs affecting pollination, degradation of glumes and reduction in the number of grains in the spike and the percentage of fruit set [35].
Increased application of nitrogen fertilizer increased spring maize kernel yield by 6.80% to 24.66% [34]. This study showed that the highest yield (6907.25 kg hm−2) was recorded in Zhangza 10 when nitrogen was applied at a rate of 225 kg hm−2 and Zhangza 10 had the highest yield (6109.8 kg hm−2) when nitrogen was applied at a rate of 150 kg hm−2. Reasonable application of nitrogen fertilizer will have higher root cortical aeration tissues, promote soil excavation and deep soil nitrogen acquisition, thereby increasing the amount of nitrogen absorption and utilization efficiency [36] and increase the re-assimilation ability of leaf NH4+ [37]. It also promotes and assimilate accumulation [10] and dry matter transport from vegetative organs to seeds [11], thereby increasing reservoir capacity [38] and coordinating the source/reservoir ratio [39], promoting the drive to drive nitrogen transport from vegetative organs to seeds [40]. In addition, it can effectively regulate the morphological development and biomass distribution of millet during growth [41]. Nitrate nitrogen can induce the expression of cytokinin synthesis genes IPT3 and CYP735A [42]. This study showed that the yield of different genotypes of foxtail millet was reduced at a nitrogen application rate of 300 kg hm−2. Under the condition of high nitrogen, the root activity and effective root absorption area were improved, decreasing photosynthesis rate, unbalanced carbon and nitrogen metabolism and function of root cells may have changed [43,44]. In addition, the activity of nitrate reductase (NR), a key enzyme in nitrogen metabolism, was reduced and nitrogen uptake was reduced [36]. As a result, the nitrogen retention in leaves and stem sheathing at maturity will increase, leading to the growth of stems and leaves without efficient flow to ear [45] and the proportion of dry matter allocated to economic organs will decrease [46]. This resulted in the decrease of seed setting rate and panicle number [47], which was not conducive to the improvement of cash crop yield [48].
5.2 Effect of Nitrogen Fertilizer on Quality
Reasonable application of nitrogen fertilizer had a significant regulatory effect on grain quality [17]. Sun et al. [49] showed that protein content had the largest loading in the first principal component contribution Nitrogen application could enhance protein content in rice [50] as well as soybean [51]. Nitrogen application in low-fertility wheat fields has a greater effect on the enhancement of protein factors, and nitrogen application in medium-high-fertility wheat fields can increase protein factors [49]. Moderately increasing nitrogen levels could increase the contents of soluble protein and free amino acid in peanut organs, and increase the activities of nitrate reductase, glutamine synthetase and other nitrogen assimilases [52]. It can also increase the storage of nitrogen in the vegetative organs of plants before flowering and delay their senescence [53], which is conducive to the uptake and assimilation of nitrogen and promotes the accumulation of nitrogen in the plant [54], and increase the synthesis of proteins in the seeds [52]. The results of this study showed that the protein content of different genotypes of foxtail millet showed a trend of increasing and then decreasing, and the protein content of different genotypes of foxtail millet decreased at a nitrogen application rate of 300 kg hm−2. This may be because too much nitrogen fertilizer is applied during the vegetative growth stage, resulted in excessive nitrogen absorption and excessive nutrient consumption, which is not conducive to the carbon and nitrogen metabolism of plants in the grouting stage [55]. As a result, more sugars are converted into organic acids by enzymatic conversion, which affects the transfer of photosynthetic products to grains [56], resulting in excessive crop populations [55], intensifying competition for source pools [57] and reducing grain quality [58].
Han et al. showed that the crude fat content of foxtail millet showed an increasing and then decreasing trend with the increase of nitrogen application and the fat content was highest at a nitrogen application rate of 180 kg hm−2 [59]. The starch content of rice was the lowest when the nitrogen rate was 400 kg hm−2 [60]. We found that the starch content of different genotypes of grains decreased at a nitrogen application rate of 225 kg hm−2, which may be due to the fact that starch is affected by the environment, cultivar, and nitrogen application [61] and also because the relative improvement of nitrogen metabolism during the filling process suppresses carbon metabolism [62]. The relative improvement in nitrogen metabolism during ensiling suppresses carbon metabolism [62], nitrate in leaves regulates nitrate reductase activity, which can cause more sugars to undergo enzymatic conversion to organic acids, affecting starch accumulation [56] and higher levels of nitrate also lead to unfavorable starch synthesis due to the inhibition of nitrate reductase on the subunit of ADP-glucose pyrophosphorylase [63].
Bullman et al. showed that the phenylalanine content increased proportionally with the increase in nitrogen fertilizer supply [64]. We found that the phenylalanine content of different genotypes of foxtail millet increased first and then decreased after nitrogen application. It may be due to the fact that the amino acids in roots can be used as precursors for plant hormone synthesis and can regulate nitrogen transport from source to sink [65]. Too little secretion of roots under nitrogen deficiency conditions may change the direction of assimilate transport, and the secretion of basic amino acids will lead to a decrease in grain amino acid content [66]. Wang et al. showed that the contents of aspartic, serine, glutamic, glycine, isoleucine, tryptophan, histidine and arginine were significantly positively correlated with the amount of nitrogen application [67]. Nokerbekova et al. showed that nitrogen application could increase the content of lysine, phenylalanine and valine in sweet sorghum [68]. In this study, it was found that the content of lysine, phenylalanine and valine in sweet sorghum increased when the nitrogen rate was 225 kg hm−2. This may be attributed to the increased application of nitrogen fertilizer, which improved the assimilation indexes of crop nitrogen metabolism, such as nitrogen accumulation in the ground and seeds and the activity of glutamine synthetase in leaves, promoted the absorption and assimilation of nitrogen by plants [69] and enabled the grains to accumulate sufficient NH4+−N [36]. At the same time, increasing the activity of Nitrate Reductase and Glutamine Synthetase [54] promotes nitrogen metabolism [69]. Carbohydrate-derived carbon skeleton and nitrogen contribute to the biosynthesis of amino acids [70]. As the main subunit of all protein molecules, the content of amino acids increases with the increase of protein content [68]. The single form of NO3− −N can also promote the increase of GS activity in leaves, promote the enhancement of nitrogen metabolism, and enhance nitrogen metabolism and amino acid synthesis and transformation [71].
Matsumoto et al. [72] showed that essential amino acids such as isoleucine and valine in millet had a significant enhancement effect on protein synthesis. This study showed that protein content showed a significant positive correlation with the content of most amino acids (Fig. A1). It may be because nitrogen application increased the activity of nitrate reductase (NR) and glutamine synthetase (GS) [52], promoted the absorption and assimilation of nitrogen and increasing protein content [73]. As the main transport form of nitrogen assimilation in plants [74]. amino acid content is the main subunit of all protein molecules [68], which is involved in the absorption and assimilation of nitrogen and is associated with the synthesis and degradation of proteins in organs [39].
5.3 Effect of Nitrogen Fertilizer on RVA
Lei et al. [75] used principal component analysis and cluster analysis to determine the protein content, BD, PV, FV, and SB as the key indicators for evaluating the quality of steamed grain rice. Rice with better eating quality generally showed high PV and BD, while the opposite was true for poor-quality rice [62]. Hu et al. showed that the BD of rice decreased with the increase in nitrogen application [76], and the PV and FV of buckwheat decreased [77]. Excessive nitrogen application inhibited the FV, SB and TV of millet [17]. In this study, we found that the PV, TV and BD of Jingu 21 showed a tendency to decrease and then increase. It may be because nitrogen application reduced the content of amylose [50] and amylopectin [78]. The lower the amylose content, the softer and more sticky after cooking [79] and the higher the PT [58]. Amylopectin has more branch structure, which makes rice have a soft taste [80]. The more the content of short and medium chains of amylopectin, the better the cooking and eating quality [81].
In this study, we found that the PV, FV and BD of Zhangza 10 showed a tendency of increasing and then decreasing. Proteins affect the amount of water absorbed by the rice, thus affecting the pasting behavior of starch [82]. The protein content of grain increased with the increase of nitrogen application [83]. Higher protein content and higher disulfide bonds limit the starch/water interaction, limiting the adhesion and eventually losing the protein's network effect [82] and thus reduce viscosity [17]. The taste quality of rice is closely related to the amino acid content of rice [84], amino acid content is positively correlated with the PV and negatively correlated with the FV [85]. In this study we found that the amino acid content was negatively correlated with the PV, probably due to differences in stratification, and it remains to be investigated whether it is affected by the inherent characteristics of the variety [85].
The plant height of different genotypes of foxtail millet was proportional to the amount of nitrogen application. The contents of protein, fat, isoleucine, arginine, aspartic acid, glutamic acid and glycine in different genotypes of foxtail millet increased first and then decreased under nitrogen application. The PV, TV, BD and FV of Jingu 21 decreased first and then increased, while that of Zhangza 10 decreased gradually. The analysis of correlation results showed that the protein content of different genotypes of millet was significantly positively correlated with most amino acid content. The positive correlation between the yield of Jingu 21 and the content of protein and tryptophan was the strongest (r = 0.91). The positive correlation between the yield of Zhangza 10 and fiber content was the strongest (r = 0.89). Under the experimental conditions, the optimal nitrogen application rates of Jinguu 21 and Zhangza 10 were 150 and 225 kg hm−2, respectively. It provides a theoretical basis for rational fertilization and high-yield and high-quality cultivation techniques of foxtail millet.
Acknowledgement: We thank Ruiyun Wang for her guidance on our manuscript, and the Center for Agricultural Genetic Resources Research, Shanxi Agricultural University, for providing resources and platform.
Funding Statement: This work was funded by the earmarked fund for CARS (CARS-06-14.5-A16), the Shanxi Provincial Key Research and Development Program (Program No. 2022ZDYF110), the earmarked fund for Modern Agro-Industry Technology Research System (2024CYJSTX03-23), the Central Guided Local Science and Technology Development Funds (YDZJSX2022A044, YDZJSX20231A040).
Author Contributions: Original writing, Jiao Mao; contribution experiment and data analysis, Shu Wang, Yuanmeng Xu, Mengyao Wang; experimental design, Yuhan Liu, Jiangling Ren; resource acquisition, supervision, and review, Sichen Liu, Xiaoning Cao, Zhijun Qiao. All authors reviewed the results and approved the final version of the manuscript.
Availability of Data and Materials: All the data supporting the findings of this study are included in this article.
Ethics Approval: Not applicable.
Conflicts of Interest: The authors declare no conflicts of interest to report regarding the present study.
References
1. Diao XM. New progress in research on stress tolerance and cultivation techniques of cereal crops. Sci Agric Sin. 2019;52(22):3943–9. doi:10.3864/j.issn.0578-1752.2019.22.001. [Google Scholar] [CrossRef]
2. Kumar A, Metwal M, Kaur S, Gupta AK, Puranik S, Singh S, et al. Nutraceutical value of finger foxtail millet [Eleusine coracana (L.) Gaertn.], and their improvement using omics approaches. Front Plant Sci. 2016;7(399):934. doi:10.3389/fpls.2016.00934. [Google Scholar] [PubMed] [CrossRef]
3. Jia GQ, Diao XM. Innovation status and future prospects of foxtail millet seed industry in China. Sci Agric Sin. 2022;55(4):653–65. doi:10.3864/j.issn.0578-1752.2022.04.003. [Google Scholar] [CrossRef]
4. Yan JK, Zhang NN, Zhang SQ. Ecophysiological responses of foxtail millet to drought stress. Acta Ecologica Sinica. 2021;41(21):8612–22. doi:10.5846/stxb202008022014. [Google Scholar] [CrossRef]
5. Muthamilarasan M, Khandelwal R, Yadav CB, Bonthala VS, Khan Y, Prasad M. Identification and molecular characterization of MYB transcription factor superfamily in C4 model plant foxtail millet (Setaria italica L.). PLoS One. 2017;9(10):e109920. doi:10.1371/journal.pone.0109920. [Google Scholar] [PubMed] [CrossRef]
6. Pan JW, Li Z, Wang QG, Gang YA, Li XB, Huangfu YG, et al. Phosphoproteomic profiling reveals early salt-responsive mechanisms in two foxtail millet cultivars. Front Plant Sci. 2021;12:712257. doi:10.3389/fpls.2021.712257. [Google Scholar] [PubMed] [CrossRef]
7. Zhou JM, Zhao L, Dong YY, Xu J, Bian WY, Mao YC, et al. Effects of nitrogen fertilizer and planting density on rice yield and nitrogen use efficiency. J Plant Nutr. 2010;16(2):274–81. doi:10.11674/zwyf.2010.0203. [Google Scholar] [CrossRef]
8. Deng Y, Zheng Y, Lu JY, Guo ZY, Sun XM, Zhao L, et al. Effects of different N fertilizer doses on phenology, photosynthetic fluorescence, and yield of quinoa. Agron J. 2024;14(5):914. doi:10.3390/agronomy14050914. [Google Scholar] [CrossRef]
9. Chen LQ, Zhang WZ, Gao JP, Liu YZ, Wang X, Liu YQ, et al. Effects of precise K fertilizr application on the yield and quality of rice under the mode of light, simple, and high-efficiency N fertilizer application during the panicle stage. Agron J. 2022;12(7):1681. doi:10.3390/agronomy12071681. [Google Scholar] [CrossRef]
10. Koutroubas SD, Fotiadis S, Damalas CA. Biomass and nitrogen a cumulation and translocation in spelt (Triticum spelta) grown in a Mediterranean area. Field Crops Res. 2012;127:1–8. doi:10.1016/j.fcr.2011.10.011. [Google Scholar] [CrossRef]
11. Kong LA, Xie Y, Hu L, Feng B, Li SD. Remobilization of vegetative nitrogen to developing grain in wheat (Triticum aestivum L.). Field Crops Res. 2016;196:134–44. doi:10.1016/j.fcr.2016.06.015. [Google Scholar] [CrossRef]
12. Wang YC, Wang EL, Wang DL, Huang SM, Ma YB, Smith CJ, et al. Crop productivity and nutrient use efficiency as affected by long-term fertilisation in North China Plain. Nutr Cycl Agroecosyst. 2010;86(1):105–19. doi:10.1007/s10705-009-9276-5. [Google Scholar] [CrossRef]
13. Tong YA, Zhao Y, Zhao HB, Fan HZ. Effects of nitrogen application rate on nitrogen uptake, translocation and yield of winter wheat. J Plant Nutr. 2007;13(1):64–9. doi:10.3321/j.issn:1008-505X.2007.01.011. [Google Scholar] [CrossRef]
14. Yuan Y, Lin F, Maucieri C, Zhang YJ. Efficient irrigation methods and optimal nitrogen dose to enhance wheat yield, inputs efficiency and economic benefits in the North China Plain. Agronomy. 2022;12(2):273. doi:10.3390/agronomy12020273. [Google Scholar] [CrossRef]
15. Zhang AY, Guo EH, Wang J, Fan HP, Li YH, Wang LX, et al. Effects of nitrogen application rate on agronomic traits, photosynthetic characteristics and yield of spring foxtail millet. Sci Agric Sin. 2015;48(15):2939–51. doi:10.3864/j.issn.0578-1752.2015.15.004. [Google Scholar] [CrossRef]
16. Zhang YQ, Li SW, Fu W, Wen HD. Effects of nitrogen application on yield, photosynthetic characteristics and water use efficiency of hybrid foxtail millet. J Plant Nutr. 2014;20(5):1119–26. doi:10.11674/zwyf.2014.0508. [Google Scholar] [CrossRef]
17. Dong EW, Wang Y, Wang JS, Liu QX, Huang XL, Jiao XY. Effects of nitrogen application rate on foxtail millet yield, nitrogen utilization and foxtail millet quality. Sci Agric Sin. 2024;57(2):306–18. doi:10.3864/j.issn.0578-1752.2024.02.007. [Google Scholar] [CrossRef]
18. Du YC, Wang YB, Fan WT, Gai ZJ, Yu DS, Gu W, et al. Effects of different nitrogen levels on the activities of nitrate reductase and nitrite reductase in sugar beet. J Plant Nutr. 2012;18(3):717–23. doi:10.11674/zwyf.2012.11331. [Google Scholar] [CrossRef]
19. Cai RG, Yin YP, Zhang M, Dai ZM, Yan ML, Fu GZ, et al. Effects of nitrogen level on grain quality of Gaocheng 8901 and Shannong 1391. Acta Agron Sin. 2007;33(2):304–10. doi:10.3321/j.issn:0496-3490.2007.02.020. [Google Scholar] [CrossRef]
20. Liu W, Wang K, Zhao Y, Shen YY, Zhang C, Peng YX, et al. Effects of nitrogen application on physicochemical properties of rice starch under elevated temperature. Food Chem. 2024;433:137303. doi:10.1016/j.foodchem.2023.137303. [Google Scholar] [PubMed] [CrossRef]
21. Hu MM, Lan Y, Peng LG, Li CM, Chen GY, Kuang HD, et al. Effects of nitrogen application rate on yield, quality and γ-aminobutyric acid content of giant embryo rice. J Plant Nutr. 2022;28(11):1947–63. doi:10.11674/zwyf.2022163. [Google Scholar] [CrossRef]
22. Yang QR, Li LT, Zhang X, Miao YH, Sheng K, Zhang D, et al. Effects of nitrogen application on yield, quality, light and temperature physiological characteristics of summer peanut. Chin J Eco-Agric. 2024;32:627–39. doi:10.12357/cjea.20230568. [Google Scholar] [CrossRef]
23. Gous PW, Warren F, Mo OW, Gilbert RG, Fox GP. The effects of variable nitrogen application on barley starch structure under drought stress. J I Brewing. 2015;121(4):502–9. doi:10.1002/jib.260. [Google Scholar] [CrossRef]
24. Karrou M, Nachit M. Durum wheat genotypic variation of yield and nitrogen use efficiency and its components under different water and nitrogen regimes in the Mediterranean region. J Plant Nutr. 2015;38(14):2259–78. doi:10.1080/01904167.2015.1022184. [Google Scholar] [CrossRef]
25. Gu BJ, Ge Y, Ren Y, Bin Xu, Luo WD, Jiang H, et al. Atmospheric reactive nitrogen in China: sources, recent trends, and damage costs. Environ Sci Technol. 2012;46(17):9420–7. doi:10.1021/es301446g. [Google Scholar] [PubMed] [CrossRef]
26. Chen P. Description specification and data standard of foxtail millet germplasm resources. Beijing: China Agriculture Press; 2006. [Google Scholar]
27. Wieser H, Antes S, Seilmeier W. Quantitative determination of gluten protein types in wheat flour by reversed-phase high-performance liquid chromatography. Cereal Chem. 1998;75(5):644–50. doi:10.1094/CCHEM.1998.75.5.644. [Google Scholar] [CrossRef]
28. GB 5009.5–2016. National Standard for Food Safety, Determination of Protein in Food. 2016. Available from: https://kns.cnki.net/kcms2/article/abstract?v=W694F5cljyAoM5tP1_9Zlz95vGd9znZRfkq9bQipvaSLYKjIn2CZVattv23covEtTj9jxV5YA5T2lilz13lLpj7cWXhZ-E5PUi7oJpy4FnQtb1Mdja3G0Zw_2mk5IoT_KRpnuR5ONyRGu2sA_poCvMdmbHmST2dE3TbiMA8urpoG6GprwLL_uA==&uniplatform=NZKPT&language=CHS. [Accessed 2024]. [Google Scholar]
29. Zhuang KY, Kong FY, Zhang S, Meng C, Yang MM, Liu ZB, et al. Whirly1 enhances tolerance to chilling stress in tomato via protection of photosystem II and regulation of starch degradation. New Phytol. 2019;221(4):1998–2012. doi:10.1111/nph.15532. [Google Scholar] [PubMed] [CrossRef]
30. GB/T 5009.10-2003. Determination of crude fiber in plant foods. 2003. Available from: https://kns.cnki.net/kcms2/article/abstract?v=W694F5cljyC3G3-f3hrQctjoAMusWPF8Ky0DoirkhN94U91S8EA5TYEMjnBpjaRKvw8X_hSnZ8brXRkKEgtPGD-cYRCW2Q-tBqFbkcegIOXzQA9JHWWnYSHphRE_mNdEGmuMTppBgGhp6KPw9XDdgUvxoatVWf1mRJf0vHYhBk1Z4KYJTniOMQ==&uniplatform=NZKPT&language=CHS. [Accessed 2024]. [Google Scholar]
31. Kovács Z, Simon-Sarkadi L, Sovány C, Kirsch K, Galiba G, Kocsy G. Differential effects of cold acclimation and abscisic acid on free amino acid composition in wheat. Plant Sci. 2011;180(1):61–8. doi:10.1016/j.plantsci.2010.08.010. [Google Scholar] [PubMed] [CrossRef]
32. Qi YY, Wang N, Yu JL, Wang S, Wang SJ, Copeland L. Insights into structure-function relationships of starch from foxtail millet cultivars grown in China. Int J Biol Macromol. 2020;155(9):1176–83. doi:10.1016/j.ijbiomac.2019.11.085. [Google Scholar] [PubMed] [CrossRef]
33. Liu PZ, Zhou D, Guo XY, Yu Q, Zhang YH, Li HY, et al. Response of water use and yield of winter wheat to nitrogen application rate in dryland of different rainfall years. Sci Agri Sinc. 2021;54(14):3065–76. doi:10.3864/j.issn.0578-1752.2021.14.012. [Google Scholar] [CrossRef]
34. Wang LF, Chen J, Shangguan ZP. Photosynthetic characteristics and nitrogen distribution of large-spike wheat in Northwest China. J Integr Agric. 2016;15(3):545–52. doi:10.1016/S. [Google Scholar] [CrossRef]
35. Zhang WY, Chen YJ, Wang Z, Yang JC. Polyamines and ethylene in rice young panicles in response to soil drought during panicle differentiation. Plant Growth Regul. 2017;82(3):491–503. doi:10.1007/s10725-017-0275-2. [Google Scholar] [CrossRef]
36. Li XL, Wang R, Zhou BY, Wang XB, Wang J, Zhao M, et al. Characterization of root morphology and anatomical structure of spring maize under varying N application rates and their effects on yield. Agron J. 2022;12(11):2671. doi:10.3390/agronomy12112671. [Google Scholar] [CrossRef]
37. Li F, Yang TZ, Zhang XQ, Wu YJ, Li LH, Kang XL, et al. Flue-cured tobacco nitrogen metabolism differences and its influence on aroma precursors in different amount of nitrogen. Acta Agric Boreali Sin. 2014;29(1):170–7. doi:10.7668/hbnxb.2014.01.031. [Google Scholar] [CrossRef]
38. Wang XW, Wang SH, Li GH, Wang QS, Liu ZH, Yu X, et al. Effects of panicle nitrogen f-ertilizer on cytokinin and auxin concentrations in young panicles of rice and their relationship with spikelet development. Acta Agron Sin. 2008;34(12):2184–9. doi:10.3724/SP.J.1006.2008.02184. [Google Scholar] [CrossRef]
39. Gong XW, Han HK, Zhang DZ, Li J, Wang M, Xue ZY, et al. The regulation effect of nitrogen fertilizer management on dry matter accumulation and transport and leaf nitrogen metabolism in the late growth stage of broomcorn millet. Sci Agric Sin. 2018;51(6):1045–56. doi:10.3864/j.issn.0578-1752.2018.06.004. [Google Scholar] [CrossRef]
40. Li GH, Hu QQ, Shi YG, Cui KH, Nie LX, Huang JL, et al. Low nitrogen application enhances starch-metabolizing enzyme activity and improves accumulation and translocation of non-structural carbohydrates in rice stems. Front Plant Sci. 2018;9:1128. doi:10.3389/fpls.2018.01128. [Google Scholar] [PubMed] [CrossRef]
41. Qin N, Zhu CC, Dai ST, Song YH, Wang CY, Li JX, et al. Effects of nitrogen fertilizer application stage on the grain yield and quality and nitrogen use of foxtail millet. J China Agric Univ. 2023;28(1):67–78. doi:10.11841/j.issn.1007-4333.2023.01.06. [Google Scholar] [CrossRef]
42. Takei K, Ueda N, Aoki K, Kuromori T, Hirayama T, Shinozaki K, et al. AtIPT3 is a key determinant of nitrate dependent cytokinin biosynthesis in Arabidopsis. Plant Cell Physid. 2004;45(8):1053–62. doi:10.1093/pcp/pch119. [Google Scholar] [PubMed] [CrossRef]
43. Saiz-Fernández I, De Diego N, Brzobohatý B, Muñoz-Rueda A, Lacuesta M. The imbalance between C and N metabolism during high nitrate supply inhibits photosynthesis and overall growth in maize (Zea mays L.). Plant Physiol Biochem. 2017;120:213–22. doi:10.1016/j.plaphy.2017.10.006. [Google Scholar] [PubMed] [CrossRef]
44. Xu GW, Lu DK, Wang HZ, Li YJ. Morphological and physiological traits of rice roots and their relationships to yield and nitrogen utilization as influenced by irrigation regime and nitrogen rate. Agric Water Manag. 2018;203(4):385–94. doi:10.1016/j.agwat.2018.02.033. [Google Scholar] [CrossRef]
45. Liu F, Shi AL, Zhu HJ, Duan YT, Guan CZ, Luo LX, et al. Effects of nitrogen application and fertilizer ratio on population growth and yield of rice. Hybrid Rice. 2024;39(3):117–26. doi:10.16267/j.cnki.1005-3956.20230707.225. [Google Scholar] [CrossRef]
46. Peng SB, Buresh RJ, Huang JL, Yang JC, Zou YB, Zhong XH, et al. Strategies for overcoming low agronomic nitrogen use efficiency in irrigated rice systems in China. Field Crops Res. 2006;96(1):37–47. doi:10.1016/j.fcr.2005.05.004. [Google Scholar] [CrossRef]
47. Song ZW, Zhao L, Bi JG, Tang QY, Wang GD, Li YX. Effects of nitrogen application rate on dry matter accumulation and nutrient uptake of rice cultivars with different nitrogen use ef-ficiency under drip irrigation. Acta Agron Sin. 2024;50(8):2025–38. doi:10.3724/SP.J.1006.2024.32061. [Google Scholar] [CrossRef]
48. Tekalign T, Hammes PS. Growth and productivity of potato as influenced by cultivar and reproductive growth: I. Stomatal conductance, rate of transpiration, net photosynthesis, and dry matter production and allocation. Sci Hortic. 2005;105(1):13–27. doi:10.1016/j.scienta.2005.01.029. [Google Scholar] [CrossRef]
49. Sun M, Feng HX, Zhang XY, Liu SJ, Han ZD, Han XJ, et al. Effects of nitrogen application rate on wheat yield and quality under different soil fertility. J Triticeae Crops. 2022;42(7):826–34. doi:10.7606/j.issn.1009-1041.2022.07.06. [Google Scholar] [CrossRef]
50. Dong MH, Sang DZ, Wang P, Wang XM, Yang JC. Changes in cooking and nutrition qualities of grains at different positions in a rice panicle under different nitrogen levels. Rice Sci. 2007;14(2):141–8. doi:10.1016/S1672-6308(07)60020-1. [Google Scholar] [CrossRef]
51. Chiluwal A, Haramoto ER, Hildebrang D, Naeve S, Poffenbarger H, Purcell LC, et al. Late-season nitrogen applications increase soybean yield and seed protein concentration. Front Plant Sci. 2021;12:715940. doi:10.3389/fpls.2021.715940. [Google Scholar] [PubMed] [CrossRef]
52. Zhang ZM, Wan SB, Ning TY, Dai LX. Effects of nitrogen levels on nitrogen metabolism and related enzyme activities in peanut. Chin J Plant Ecol. 2008;32(6):1407–16. doi:10.3773/j.issn.1005-264x.2008.06.022. [Google Scholar] [CrossRef]
53. Fang XM, Li Y, Nie J, Wang C, Huang K, Zhang Y, et al. Effects of nitrogen fertilizer an-d planting density on the leaf photosynthetic characteristics, agronomic traits and grain Y-ield in common buckwheat (Fagopyrum esculentum M.). Field Crop Res. 2018;219(2):160–8. doi:10.1016/j.fcr.2018.02.001. [Google Scholar] [CrossRef]
54. Wang YF, Yu ZW, Li SX, Yu SL. Effects of nitrogen nutrition level on the activity of key enzymes of nitrogen metabolism and grain protein content in winter wheat. Acta Agron Sin. 2002;28(6):743–8. doi:10.3321/j.issn:0496-3490.2002.06.005. [Google Scholar] [CrossRef]
55. Osaki M, Iyoda M, Tadano T. Ontogenetic changes in the contents of ribulose-1,5-bisphosphate carboxylase/oxygenase, phosphoeno/pyruvate carboxylase, and chlorophyll in individual leaves of maize. Soil Sci Plant Nutr. 1995;41(2):285–93. doi:10.1080/00380768.1995.10419585. [Google Scholar] [CrossRef]
56. Stitt M, Muller C, Matt P, Gibon Y, Carillo P, Morcuende R, et al. Steps towards an integrated view of nitrogen metabolism. J Exp Bot. 2002;53(370):959–70. doi:10.1093/jexbot/53.370.959. [Google Scholar] [PubMed] [CrossRef]
57. Guo YF, Gan SS. Translational researches on leaf senescence for enhancing plant productivity and quality. J Exp Bot. 2014;65(14):3901–13. doi:10.1093/jxb/eru248. [Google Scholar] [PubMed] [CrossRef]
58. Zhu DW, Zhang HC, Guo BW, Xu K, Dai QG, Wei CX, et al. Effects of nitrogen level on structure and physicochemical properties of rice starch. Food Hydrocoll. 2017;63(5):525–32. doi:10.1016/j.foodhyd.2016.09.042. [Google Scholar] [CrossRef]
59. Han GL, Wang J, Zhao HY, Wang D, Duan Y, Han RH, et al. Response of quality and yield of millet to nitrogen and zinc application. Agriculture. 2023;13(9):1731. doi:10.3390/agriculture13091731. [Google Scholar] [CrossRef]
60. Zhou T, Zhou Q, Li E, Yuan L, Wang W, Zhang H, et al. Effects of nitrogen fertilizer on structure and physicochemical properties of ‘super’ rice starch. Parbohyd Polym. 2020;239(2):116237. doi:10.1016/j.carbpol.2020.116237. [Google Scholar] [PubMed] [CrossRef]
61. Liu Q, Yang HK, Chen YQ, Wu DM, Huang XL, Fan GQ. Effects of nitrogen application rate on grain quality, brewing quality and volatile flavor compounds of waxy and non-waxy wheat. Acta Agron Sin. 2023;49(8):2240–58. doi:10.3724/SP.J.1006.2023.21038. [Google Scholar] [CrossRef]
62. Tang J, Tang C, Guo BW, Zhang CX, Zhang ZZ, Wang K, et al. Effects of nitrogen fertilizer application rate on yield and rice quality of machine-transplanted high-quality late rice. Acta Agron Sin. 2020;46(1):117–30. doi:10.3724/SP.J.1006.2020.92010. [Google Scholar] [CrossRef]
63. Scheible WR, González-Fontes A, Lauerer M, Müller-Röber B, Caboche M, Stitt M. Nitrate acts as a signal to induce organic acid metabolism and repress starch metabolism in tobacco. Plant Cell. 1997;9(5):783–98. doi:10.2307/3870432. [Google Scholar] [CrossRef]
64. Bulman P, Zarkadas CG, Smith DL. Nitrogen fertilizer affects amino acid composition and quality of spring barley grain. Crop Sci. 1994;34(5):1341–6. doi:10.2135/cropsci1994.0011183X003400050037x. [Google Scholar] [CrossRef]
65. Nozu Y, Tsugita A, Kamijo K. Proteomic analysis of rice leaf, stem and root tissues during growth course. Proteomics. 2006;6:3665–70. doi:10.1002/(ISSN)1615-9861. [Google Scholar] [CrossRef]
66. Chang EH, Zhang SF, Wang ZQ, Wang XM, Yang JC. Effects of nitrogen and phosphorus levels on amino acid contents in roots and grains of rice at grain filling stage. Acta Agron Sin. 2008;34(4):612–8. doi:10.1016/S1875-2780(08)60024-3. [Google Scholar] [CrossRef]
67. Wang DR, Lu W, Fang CW. Effect of nitrogen application on protein and amino acid content of rice. J Plant Nutr. 2001;7(3):353. doi:10.3321/j.issn:1008-505X.2001.03.017. [Google Scholar] [CrossRef]
68. Nokerbekova NK, Suleimenov YT, Zhapayev RK. Influence of fertilizing with nitrogen fertilizer on the content of amino acids in sweet sorghum grain. Albanian J Agric Sci. 2018;17(3):166–70. doi:10.20448/journal.512.2018.52.64.67. [Google Scholar] [CrossRef]
69. Wang XC, Wang XH, Xiong SP, Ma XM, Ding SJ, Wu KY, et al. Differences in nitrogen efficiency and nitrogen metabolism characteristics of wheat varieties under different nitrogen levels. Sci Agric Sin. 2015;48(13):2569–79. doi:10.3864/j.issn.0578-1752.2015.13.009. [Google Scholar] [CrossRef]
70. Nikiforova VJ, Bielecka M, Gakière B, Krueger S, Rinder J, Kempa S, et al. Effect of sulfur availability on the integrity of amino acid biosynthesis in plants. Amino Acids. 2006;30(2):173–83. doi:10.1007/s00726-005-0251-4. [Google Scholar] [PubMed] [CrossRef]
71. Jia LJ, Fan XR, Yin XM, Cao Y, Shen QR. Remobilization of nitrate in rice leaf vacuoles measured with double-barrelled nitrate-selective microelectrodes. Sci Agric Sin. 2005;38(7):1379–85. doi:10.3321/j.issn:. [Google Scholar] [CrossRef]
72. Matsumoto K, Koba T, Hamada K, Sakurai M, Higuchi T, Miyata H. Branched-chain amino acid supplementation attenuates muscle soreness, muscle damage and inflammation during an intensive training program. J Sport Med Phys Fit. 2009;49(4):424–31. doi:10.1016/j.humov.2007.07.010. [Google Scholar] [CrossRef]
73. Fan XM, Jiang D, Dai TB, Jin Q, Cao WX. Effects of nitrogen supply on nitrogen metabolism and grain protein accumulation of wheat under different water treatments. J Plant Ecol. 2006;25(2):149–54. doi:10.13292/j/1000-4890.2006.0029. [Google Scholar] [CrossRef]
74. Oliveira IC, Brenner E, Chiu J, Hsieh MH, Kouranoy A, Lam HM, et al. Metabolite and light regulation of metabolism in plants: lessons from the study of a single biochemical pathway. Braz J Med Biol Res. 2001;34(5):567–75. doi:10.1590/s0100-879x2001000500003. [Google Scholar] [PubMed] [CrossRef]
75. Lei Y, Gong YL, Deng RY, Zhang DS, Zhu SS, Tang HH, et al. Comprehensive evaluation of the quality characteristics of steamed rice based on principal component analysis and cluster analysis. Food Sci Technol. 2021;42(7):258–67. doi:10.13386/j.issn1002-0306.2020050209. [Google Scholar] [CrossRef]
76. Hu YJ, Qian HJ, Wu P, Zhu M, Xing ZP, Dai QG, et al. Effects of nitrogen, phosphorus and potassium application rates on yield and quality of soft rice under straw returning. J Plant Nutr. 2018;24(3):817–24. doi:10.11674/zwyf.17347. [Google Scholar] [CrossRef]
77. Gao LC, Xia MJ, Wan CX, Jia YH, Yang LM, Wang M, et al. Analysis of synthesis, accumulation and physicochemical properties of Tartary buckwheat starches affected by nitrogen fertilizer. Carbohyd Polym. 2021;273(2):118570. doi:10.1016/J.CARBPOL.2021.118570. [Google Scholar] [PubMed] [CrossRef]
78. Yang XY, Bi JG, Gilbert RG, Li GH, Liu ZH, Wang SH, et al. Amylopectin chain length distribution in grains of Japonica rice as affected by nitrogen fertilizer and genotype. J Cereal Sci. 2016;71:230–8. doi:10.1016/j.jcs.2016.09.003. [Google Scholar] [CrossRef]
79. Custodio MC, Cuevas RP, Ynion J, Laborte AG, Velasco ML, Demont M. Rice quality: how is it defined by consumers, industry, food scientists and geneticists? Trends Food Sci Tech. 2019;92:122–37. doi:10.1016/j.tifs.2019.07.039. [Google Scholar] [PubMed] [CrossRef]
80. Ramesh M, Zakiuddin AS, Bhattacharya KR. Structure of rice starch and its relation to cooked-rice texture. Carbohydr Polym. 1999;38(4):337–47. doi:10.1016/S0144-8617(98)00125-8. [Google Scholar] [CrossRef]
81. Champagne ET, Bett-Garber KL, Thompson J, Mutters R, Grimm CC, McClung AM. Effects of drain and harvest dates on rice sensory and physicochemical properties. Cereal Chem. 2005;82(4):369–74. doi:10.1094/CC-82-0369. [Google Scholar] [CrossRef]
82. Martin M, Fitzgerald MA. Proteins in rice grains influence cooking properties. J Cereal Sci. 2002;36(3):285–94. doi:10.1006/jcrs.2001.0465. [Google Scholar] [CrossRef]
83. Zhang L, Han ZS, Granato D. Polyphenols in foods: classification, methods of identification, and nutritional aspects in human health. Adv Food Nutr Res. 2021;98:1–33. doi:10.1016/bs.afnr.2021.02.004. [Google Scholar] [PubMed] [CrossRef]
84. Gong R, Huang DQ, Chen YB, Hong L, Wang ZD, Zhou DG, et al. Comparative metabolomics analysis reveals the variations of eating quality among three high-quality rice cultivars. Mol Breeding. 2020;40(12):112. doi:10.1007/s11032-020-01192-y. [Google Scholar] [CrossRef]
85. Ma ZH, Wang YB, Cheng HT, Zhang GC, Lyu WY. Biochemical composition distribution in different grain layers is associated with the edible quality of rice cultivars. Food Chem. 2020;311(11):125896. doi:10.1016/j.foodchem.2019.125896. [Google Scholar] [PubMed] [CrossRef]
Appendix A
Figure A1: Correlation of quality, amino acid content and RVA in different genotypes of foxtail millet. (A) Jingu 21 (B) Zhangza 10. Note: *p <= 0.05, **p <= 0.01. Red indicates a positive correlation. Blue indicates a negative correlation. The lighter the color, the weaker the correlation
Cite This Article
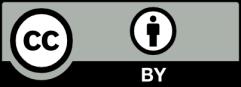
This work is licensed under a Creative Commons Attribution 4.0 International License , which permits unrestricted use, distribution, and reproduction in any medium, provided the original work is properly cited.