Open Access
ARTICLE
Investigating the Mutagenic Impact of Cadmium Nitrate on Cytomorphological and Physiological Attributes in Nigella sativa L. Cultivars
1 Cytogenetics and Plant Breeding Lab, Department of Botany, Aligarh Muslim University, Aligarh, India
2 Department of Biology, Faculty of Science, Umm Al-Qura University, Makkah, Saudi Arabia
3 Department of Biology, College of Science, Princess Nourah bint Abdulrahman University, Riyadh, Saudi Arabia
4 Department of Plant Production (Genetic Branch), Faculty of Environmental Agricultural Sciences, Arish University, El-Arish, Egypt
* Corresponding Authors: Neha Naaz. Email: ; Diaa Abd El Moneim. Email:
Phyton-International Journal of Experimental Botany 2024, 93(12), 3347-3372. https://doi.org/10.32604/phyton.2024.057592
Received 21 August 2024; Accepted 20 November 2024; Issue published 31 December 2024
Abstract
Cadmium (Cd) is a highly toxic heavy metal pollutant primarily introduced into the environment through anthropogenic activities. In plants, the absorption and retention of Cd lead to a cascade of morphological, physiological, and cytological alterations. Nigella sativa L., also known as black cumin, is an annual herb with important commercial and medicinal uses. Thymoquinone, the primary bioactive compound in Nigella sativa L., is renowned for its potential health benefits. The current experiment aimed to ascertain the effects of various concentrations (10, 20, 30, 40 and 50 ppm) of cadmium nitrate (Cd(NO3+)2) on two Nigella sativa cultivars (AN20 and Desi), analyzing their biological, physiological, quantitative attributes and cytological abnormalities in the M1 generation. It adversely effects biological, morpho-physiological, quantitative attributes at higher concentrations (30, 40 and 50 ppm). Morphological variations were observed in terms of cotyledonary leaf (shape and color), vegetative leaves (shape, size and color), plant height (tall and dwarf), growth pattern (bushy and unbranched), flower (no. of petals, androecium and gynoecium), capsules (no. of locules) and seeds (size, color and texture). With increasing cadmium concentration, both physiological and quantitative characteristics declined in a dose-dependent manner, while proline content increased correspondingly with higher concentrations of Cd(NO3)2. The maximum depreciation was found at the highest cadmium nitrate concentration (i.e., 50 ppm). Cadmium nitrate significantly impacts the desi cultivar more than the AN20 cultivar. Scanning electron microscopy (SEM) was used to observe stomatal morphology, which showed changes in stomatal size and structure. The variation in area percentage of compounds such as Thymoquinone; p-cymene; 9-Octadecenoic acid; 9,12-Octadecadienoic acid (Z, Z)-, methyl ester; 1,2,3-propanetriyl was detected by GC MS Analysis. The current study demonstrated that Nigella sativa cultivars AN20 and desi were successfully tested for induced mutagenesis, and it also offered a method that might be applied to future mutational breeding projects.Keywords
In recent years, global interest in the trade of medicinal plants and herbal products has surged, creating a market projected to exceed seven trillion dollars by 2050 [1]. For centuries, medicinal plants have been integral to indigenous healing traditions and folk remedies. They are also used in herbal medicine for their perceived safety compared to modern allopathic medications. Nigella sativa L. (Ranunculaceae), known as black cumin or black seed, is a medicinal herb with 2n = 12 chromosome number. The AN20 cultivar of N. sativa matures in 140–150 days, yielding about 1000–1200 kg per hectare, with 28% total oil content and 0.3% essential oil [2]. The oil, rich in compounds like thymol, thymoquinone (TQ), carvacrol, thymohydroquinone, nigellicine, nigellidine, and α-hederin [3], is responsible for its therapeutic benefits. Thymoquinone (30%–48%), a key phytochemical in N. sativa, is known for its wide range of medicinal properties [4]. Over the last three decades, substantial research has focused on industrial contamination, particularly heavy metals, and organisms’ resistance to their toxic effects [5]. This interest stems from the rapid release of excess metals into soil, water, and the atmosphere [6]. These metals accumulate in plant parts over time, causing morphological, physiological, biochemical, and ultra-structural changes [7,8]. Heavy metals such as cadmium, lead, copper, and zinc have been exposed to hinder plant growth [9]. Cadmium-induced environmental pollution poses a significant challenge. Cadmium (Cd), a nonessential and hazardous pollutant, primarily enters the environment via human caused activities and can severely affect plant growth and development [10]. Due to its high solubility in water, cadmium is highly toxic even at low concentrations [11]. It strongly binds to DNA and RNA, disrupting DNA synthesis and mitotic activity, and causing various types of DNA damage such as strand breaks, aberrant nitrogenous bases, DNA-DNA cross-links, and DNA-protein cross-links [12]. Cadmium (Cd) induces chlorosis and disrupts the absorption of essential nutrients such as potassium, calcium, magnesium, and phosphorus, crucial for plant biological processes, leading to stunted development. Cd inhibits chlorophyll production, affecting photosynthesis and stomatal movements [13]. It causes morphological and physiological abnormalities in various plants, including Trigonella [14], Capsicum annuum [15], and Lens culinaris [12]. Cd exposure demonstrates mutagenic potential, disrupting cell division and causing chromatin bridges, laggards, and multinucleate cells in Nigella sativa [16]. In spring barley seedlings, Cd treatment causes cytogenetic and morphological alterations [17]. In Nigella sativa, Cd treatment reduces carotenoids and chlorophyll levels [18]. As a response to Cd-induced stress, plants increase their proline content, which serves as an adaptive mechanism to mitigate the phytotoxic effects of heavy metals [19]. Proline acts as an osmoprotectant, helping the plant maintain cell stability under stress, and it quenches reactive oxygen species (ROS) to reduce oxidative damage. Moreover, proline functions as a chelator for heavy metals, binding with Cd to reduce its toxicity. Proline also promotes the synthesis of phytochelatins, which further bind to Cd and other heavy metals, effectively mitigating the toxic effects of Cd on plant physiology and development [20]. In conjunction with these natural defenses, seed priming with salicylic acid has been shown to improve germination and resistance to Cd stress in black cumin (Nigella sativa) [21]. However, Cd and lead exposure still significantly reduce germination rates and root length, with the effects becoming more severe at higher concentrations [22]. To combat such heavy metal stress, breeding techniques like induced mutagenesis have been employed to enhance desirable traits, including yield, early maturation, disease tolerance, and reduced plant height [23]. Mutagenesis, whether induced by physical or chemical means, creates novel genetic variations. Heavy metals themselves can act as mutagens, inducing both phenotypic and genotypic changes that may be selected as viable mutants for breeding purposes [12]. The research investigates the impact of cadmium nitrate on biological damage, morpho-physiological traits, and quantitative parameters in the M1 generation of Nigella sativa. The study aims to refine cyto-physiological protocols for Nigella sativa by treating two different cultivars with varying concentrations of cadmium nitrate (Cd(NO3)2). The goal is to identify stably inherited mutations that enhance the plant’s resilience to heavy metal stress. The findings are expected to improve the chemical composition of Nigella sativa, boosting its value for medicinal, aromatic, and agricultural applications.
2.1 Plant Material and Treatment Conditions
Dry and healthy Nigella sativa seeds (var. AN20) were obtained from ICAR NRCSS in Ajmer, Rajasthan, while var. desi seeds were purchased from a local market in Aligarh, Uttar Pradesh. Selecting AN20 and desi varieties offers a great balance for researchers. AN20 can produce higher yields while desi varieties require fewer inputs and thrive in local conditions. Together, they provide both increased production and sustainability, helping producers maximize their profits and efficiency. Cadmium nitrate [Cd(NO3)2] was used as a mutagen. Seeds were soaked in distilled water for 24 h, then treated with five different Cd(NO3)2 doses (10, 20, 30, 40, and 50 ppm) in phosphate buffer (pH 4) for 9 h with continuous shaking at 25°C ± 2°C. Control seeds were not treated. After treatment, seeds were washed to remove any mutagen residues. Fifty seeds from each treatment and control group were sown in five replicates of 30 seeds each in 9-inch pots with a growth medium of farm yard manure, soil, and sand (1:1 ratio). The experiment was conducted in the net house of the Department of Botany, Aligarh Muslim University, Aligarh during the rabi season of 2021–2022 (October–April). The semi-arid, sub-tropical climate of Aligarh features hot summers, cold winters, and an average annual rainfall of 847.30 mm. The experimental site is 187.5 m above sea level with an average temperature of 33°C, relative humidity of 43% or higher, and approximately 11.5 h of natural sunshine in October. The soil type is sandy loam and alkaline. Regular agricultural practices such as fertilization, weeding, and irrigation were performed. Seeds from M1 plants were collected concentration-wise 135–150 days after sowing.
The mutation breeding experiment began with calculating the LD50, the dose at which 50% of the seeds germinate. It was found that doses higher than 50 ppm Cd(NO3)2 reduced seed germination by 50% or more, so these doses were excluded from further experiments. Relevant details on the mutagenic treatment of heavy metals can be found in earlier research [12].
The percentage of seed germination in both the cultivars can be calculated by the following formula:
At maturity, the rate of plant survival in the mutagen-treated plants was measured. The survival percentage was estimated using the following formula:
Fresh young flowers were collected from 20–30 randomly picked plants in each treatment and control group. Pollen from mature, unopened anthers was placed on a slide with 1% acetocarmine solution. Pollen grains that absorbed the stain and maintained a consistent shape were considered viable, while those that did not absorb the stain and exhibited irregular shape and size were classified as sterile.
The photosynthetic pigments were estimated following Mackinney’s method [24]. Fresh leaves (1 g) were ground in a mortar with 20 mL of 80% acetone. The mixture was centrifuged at 5000 rpm for ten minutes. The supernatant was collected in a 100 mL volumetric flask, and the final volume was adjusted with 80% acetone. Absorbance was measured at 645 and 663 nm for chlorophyll and at 480 and 510 nm for carotenoids, using a spectrophotometer (Spectronic 20D, USA). Total chlorophyll and carotenoids were calculated using Arnon’s formula [25].
To estimate proline content, fresh leaves (0.5 g) were ground using a mortar and pestle with 10 mL of 3% sulfosalicylic acid. The resulting homogenate was collected and filtered through the Whatman No. 2 filter paper. From the filtrate, 2 mL was transferred into a test tube, followed by the addition of 2 mL of glacial acetic acid and ninhydrin solution. The mixture was then heated in a water bath for 1 h. To halt the reaction, the test tube was placed in an ice bath. After cooling, 4 mL of toluene was added to each test tube and gently stirred for 30 to 40 s, resulting in a distinct toluene layer forming at the top. The intensity of the resulting red color was measured at 520 nm. A standard series of proline was prepared similarly to create a standard curve, which was then used to quantify the proline content in the samples [26].
For quantitative traits assessment, data were collected from fifteen plants per replication and presented as means. The traits measured included plant height (cm), number of fertile branches per plant, number of capsules per plant, number of seeds per capsule, 1000 seed weight (g), and seed yield (g). Plant height was measured from base to apex at maturity. The number of branches emerging from the main stem and the number of capsules per plant were counted at maturity. Seeds per capsule were counted from randomly selected capsules. Seed weight was determined by weighing 1000 seeds from the selected plants. Total plant yield was measured by weighing all seeds from the fifteen plants.
Morphological screening was conducted periodically after seed germination, and preliminary phenotypic screening was done regularly to monitor mutant characters in plant morphology. Variations were observed in plant height (tall, semi-dwarf, and dwarf), growth habit (few branches, bushy, and unbranched), cotyledonary leaf (shape, size, and color), vegetative leaf (shape, size, and color), flowers (number of petals, color, androecium, and gynoecium), capsules (number of locules), and seeds (size, texture, and color).
For cytological observations, moderately sized flower buds from 110-day-old treated plants and control were collected in the early morning and fixed in Carnoy’s fluid (absolute alcohol, chloroform, and glacial acetic acid 6:3:1 v/v) for 24 h, then stored in 70% alcohol. Anthers from the buds were squashed in 1% propionocarmine, and permanent slides were prepared through the NBA-GAA series, mounted in Canada balsam, and dried at 45°C.
2.10 Scanning Electron Microscopy (SEM) Analysis
The stomatal aperture study was conducted using scanning electron microscope imaging. Fresh leaves from 5-week-old plants were collected and prepared for microscopy. Leaf samples were fixed in 2.5% glutaraldehyde in 0.05 M phosphate buffer (pH 7.1) for four hours, then dehydrated using an alcohol series (30%, 50%, 70%, 90%, 100%) for 15–20 min each. They were then mounted on aluminum stubs, gold-coated for four minutes using a sputter coater, and analyzed with a SEM (JEOL, JSM-6510LV, Japan) at 15 kV at various magnifications at the University Sophisticated Instrumentation Facility (USIF), Aligarh Muslim University, Aligarh, India.
Ten grams of Nigella sativa seeds from both the control and variants were ground into powder using a mortar and pestle. The powdered seeds were soaked in 30 mL of methanol for 24 h. After soaking, the extract was centrifuged at 10,000 rpm for 15 min. The pellet was discarded, and the supernatant was filtered through Whatman filter paper No. 1. The filtered extract was collected and stored in vials for further analysis. Phytochemical components of N. sativa were detected using a GC-MS system (Model: Shimadzu QP 2010 Ultra GC MS) [27].
Data from five replicates of each treatment were presented as mean ± SE. DMRT (Duncan Multiple Range Test) at a 5% significance level (p ≤ 0.05) was used to compare means using RStudio. Graphs were plotted using SigmaPlot to visualize the data. Origin 2024 (10.1) was utilized to generate a Pearson correlation heatmap and a pairwise scatter plot matrix with linear regression lines. These visualizations were created to investigate the connections and correlations between variables within the dataset.
3.1 Seed Germination, Plant Survival and Pollen Fertility
Fig. 1 illustrates the effect of different cadmium nitrate doses on seed germination, plant survival, and pollen fertility in Nigella sativa varieties. The data reveal a negative correlation with increasing mutagen doses over control. Seed germination in var. AN20 and desi were 94.00% and 93.00% in the control but decreased significantly (p < 0.05) with increasing Cd(NO3)2 concentrations, ranging from 85.00% to 67.00% in var. AN20 and 84.00% to 64.00% in var. desi. Plant survival also showed a significant decline with higher mutagen doses. Control plant survival at maturity was 95.74% for var. AN20 and 94.62% for desi, but decreased from 89.29% (10 ppm) to 80.49% (50 ppm) in var. AN20 and from 80.49% (10 ppm) to 64.06% (50 ppm) in var. desi. The highest dose of 50 ppm resulted in the lowest pollen fertility, with 71.82% in var. AN20 and 70.34% in var. desi, compared to the control. Overall, the desi variety exhibited greater sensitivity to mutagenic treatments than var. AN20 (Fig. 1A,B).
Figure 1: Effect of cadmium nitrate on germination, plant survival and pollen fertility of Nigella sativa L. varieties (A) AN20 (B) Desi. The data are expressed as mean ± SE. Error bars annotated with different lowercase letters denote significance at the 5% level, determined by the Duncan multiple range test (DMRT)
3.2 Total Chlorophyll and Carotenoid Contents
The pigment contents (chlorophyll a, chlorophyll b, total chlorophyll, and carotenoids) in the M1 generation decreased with increasing Cd(NO3)2 concentrations in both varieties. The chlorophyll content of control was 1.272 mg g−1 FW and 1.270 mg g−1 FW, and carotenoids were 0.308 mg g−1 FW and 0.302 mg g−1 FW in var. AN20 and desi, respectively. At the highest concentration (50 ppm), var. AN20 showed significant decreases (p < 0.05) in total chlorophyll and carotenoids to 0.925 mg g−1 FW and 0.152 mg g−1 FW, respectively (Fig. 2). In var. desi, total chlorophyll was 0.933 mg g−1 FW, and carotenoids were 0.144 mg g−1 FW (Fig. 2B).
Figure 2: Effect of cadmium nitrate on chlorophyll a, chlorophyll b, total chlorophyll and carotenoid content of Nigella sativa L. varieties (A) AN20 (B) Desi. The data are expressed as mean ± SE. Error bars annotated with different lowercase letters denote significance at the 5% level, determined by the Duncan multiple range test (DMRT)
The effects of cadmium nitrate on the proline content in two varieties of Nigella sativa AN20 and Desi—both exhibited notable variations in proline accumulation compared to control plants. Initially, the proline content in control plants was recorded at 25.02 and 26.24 µg/g FW in var. AN20 and Desi, respectively. Upon treatment with cadmium nitrate, proline levels experienced a significant increase, reaching 34.25 µg/g FW in AN20 and 36.57 µg/g FW in Desi, demonstrating that cadmium stress significantly enhances proline synthesis as a protective response (Fig. 3).
Figure 3: Effect of cadmium nitrate on Proline content of two varieties of Nigella sativa AN20 and Desi. The data are expressed as mean ± SE. Error bars annotated with different lowercase letters denote significance at the 5% level, determined by the Duncan multiple range test (DMRT)
Quantitative traits associated with yield, including plant height, number of fertile branches per plant, number of capsules per plant, number of seeds per capsule, 1000 seed weight, and seed yield, are crucial in Nigella breeding. In this study, the mean values of all quantitative traits decreased with increasing mutagen concentrations (Fig. 4). The control plant heights were 59.22 and 56.12 cm for var. AN20 and desi, respectively, but significantly decreased (p < 0.05) to 55.24–49.85 cm and 47.44–41.32 cm from 30–50 ppm in var. AN20 and desi, respectively (Fig. 4A). The number of fertile branches dropped from 13.22 in control to 11.55 at 50 ppm in var. AN20, and from 10.24 in control to 7.92 at 50 ppm in var. AN20, and from 10.24 in control to 7.92 at 50 ppm in var. desi, with significant decreases observed at the highest concentration (Fig. 4B). Capsules per plant decreased from 16.54 in control to lower numbers in all treatments for both varieties (Fig. 4C). Seeds per capsule were 76.52 and 70.22 in control plants of var. AN20 and desi, respectively, with minimum seeds recorded at 50 ppm (Fig. 4D). The 1000 seed weight significantly decreased at 50 ppm in both varieties (Fig. 4E). Total yield per plant also decreased significantly at 50 ppm (Fig. 4F).
Figure 4: Effect of cadmium nitrate on (A) Plant height, (B) Number of branches per plant, (C) Number of capsules per plant, (D) Number of seeds per capsule, (E) 1000 seed weight and (F) Total yield per plant of Nigella sativa cultivars. The data are expressed as mean ± SE. Error bars annotated with different lowercase letters denote significance at the 5% level, determined by the Duncan multiple range test (DMRT)
3.5.1 Cotyledonary Leaf Variations
Cotyledonary leaf abnormalities were observed in mutagen-treated plants, as depicted in Fig. 5. Control seedlings of var. AN20 and desi exhibited two normal, opposite, green, and equal-sized cotyledonary leaves (Fig. 5A,D). However, plants treated with Cd(NO3)2 showed various cotyledonary leaf variants: inwardly curved cotyledonary leaves at 10 ppm (Fig. 5B), distorted cotyledonary leaves at 30 ppm (Fig. 5C) in var. AN20; and notched cotyledonary leaves at 20 ppm (Fig. 5E), as well as unequal cotyledonary leaves at 40 ppm (Fig. 5F) in var. desi.
Figure 5: (A) Control cotyledonary leaves (AN20), (B) Curved cotyledonary leaves [10 ppm Cd(NO3)2], (C) Deshaped cotyledonary leaves [30 ppm Cd(NO3)2], (D) Control cotyledonary leaves (Desi), (E) Notched cotyledonary leaves [20 ppm Cd(NO3)2] and (F) Unequal cotyledonary leaves [40 ppm Cd(NO3)2]
3.5.2 Vegetative Leaf Variations
Vegetative leaf abnormalities were observed in Cd(NO3)2-treated plants, as depicted in Fig. 6. Control vegetative leaves of var. AN20 and desi exhibited normal lamina with three lobes, each lobe showing an entire margin and acute apex (Fig. 6A,D). However, plants treated with Cd(NO3)2 showed various vegetative leaf variations: deformed lobes at 20 ppm (Fig. 6B), upward folding of lobes at 30 ppm (Fig. 6C) in var. AN20; and fused lobes of lamina at 20 and 40 ppm (Fig. 6E,F), respectively, in var. desi.
Figure 6: (A) Control cotyledonary leaves (AN20), (B) Curved cotyledonary leaves [20 ppm Cd(NO3)2], (C) Deshaped cotyledonary leaves [30 ppm Cd(NO3)2], (D) Control cotyledonary leaves (Desi), (E) Fused cotyledonary leaves [20 ppm Cd(NO3)2] and (F) Fused cotyledonary leaves [40 ppm Cd(NO3)2]
3.5.3 Plant Height and Growth Habit
Several variations in plant height and growth habits due to cadmium nitrate treatments were observed compared to the control, as shown in Fig. 7. The control plants exhibited moderate height and typical branching with an average yield (Figs. 7A and 8A). In var. AN20, variants included plants with decreased height at 20 ppm (Fig. 7B), and decreased number of branches and yield at 40 ppm (Fig. 7C). Meanwhile, var. desi showed plants with less branching and low yield at 30 ppm (Fig. 8B), and no branched plants with low yield at 50 ppm (Fig. 8C).
Figure 7: (A) Control plant [AN20], (B) Normal height with decreased number of capsules [20 ppm Cd(NO3)2], (C) Plant with normal height and yield [40 ppm Cd(NO3)2]
Figure 8: (A) Control plant [Desi], (B) Normal height with decreased number of branches [30 ppm Cd(NO3)2] and (C) No branched dwarf variant [50 ppm Cd(NO3)2]
The plants grown from seeds treated with cadmium nitrate showed significant variations in flowers compared to the control. Control flowers were white with 5 petalloid sepals, broad and ovate, characterized by nectaries, indefinite stamens with yellow anthers, a gynoecium with 5 united follicles, and a long indehiscent style (Fig. 9A,D). The variations observed included flowers with ovate shape and mucronate-like apex petalloid sepals with a hexacarpellary gynoecium at 10 ppm (Fig. 9B), and flowers with yellowish-brown colored anthers, long filament, and tricarpellary gynoecium at 30 ppm (Fig. 9C) in var. AN20. In var. desi, flowers with a tetracarpellary gynoecium at 20 ppm (Fig. 9E) and flowers with normal anthers, long filament, and tricarpellary gynoecium at 40 ppm (Fig. 9F) were observed.
Figure 9: (A) Control flower with pentacarpellary gynoecium [AN20], (B) Flower with hexacarpellary gynoecium [10 ppm Cd(NO3)2] (C) Flower with tricarpellary gynoecium [30 ppm Cd(NO3)2], (D) Control flower [Desi], (E) Flower with tetracarpellary gynoecium [20 ppm Cd(NO3)2] and (F) Flower with tricarpellary gynoecium [40 ppm Cd(NO3)2]
3.5.5 Capsule and Seed Variations
The cadmium nitrate-treated plants also exhibited notable variations in the characteristics of capsules and seeds, as depicted in Figs. 10 and 11. Control plants of var. AN20 and desi had normal-sized capsules with 5 locules (Fig. 10A,D) and black, triquetrous seeds (Fig. 11A and D). In var. AN20, capsules with 4 locules at 20 ppm (Fig. 10B) and 3 locule at 40 ppm (Fig. 10C) were observed, while var. desi showed capsules with 4 locules at 30 ppm (Fig. 10E) and 2 locules at 40 ppm (Fig. 10F). Seed variants included small-sized, white-colored seeds at 30 ppm (Fig. 11B) and bi-colored (black & white) seeds at 40 ppm (Fig. 11C) in var. AN20, and light yellow seeds at 30 ppm (Fig. 11E) and rust-colored seeds at 50 ppm (Fig. 11F) in var. desi.
Figure 10: (A) Pentalocular capsule [Control-AN20], (B) Tetralocular capsule [20 ppm Cd(NO3)2], (C) Trilocular capsule [40 ppm Cd(NO3)2], (D) Pentalocular capsule [Control-Desi], (E) Tetralocular capsule [30 ppm Cd(NO3)2] (F) Bilocular capsule [40 ppm Cd(NO3)2]
Figure 11: (A) Control seeds [AN20], (B) Small and white-coloured seeds [30 ppm Cd(NO3)2], (C) Bicolour (black & white) seeds [40 ppm Cd(NO3)2], (D) Control seeds [Desi], (E) Small and light-yellow seeds [30 ppm Cd(NO3)2] and (F) Small dark brown seeds [50 ppm Cd(NO3)2]
The current study investigated various types of chromosomal behaviors, abnormalities, and their frequencies at different meiotic stages (Fig. 12). In control plants, Nigella sativa L. exhibited normal meiotic division with 6 bivalents (2n = 12) (Fig. 12A–D). Selected variants from mutagenized plants showed abnormalities such as chromatin bridges at anaphase I (Fig. 12E), stickiness at anaphase I (Fig. 12F), laggards at telophase I (Fig. 12G), trinucleate conditions (Fig. 12H), and disturbed polarity at telophase II (Fig. 12I) in both varieties. The frequency of these chromosomal abnormalities varied with the concentration of the mutagen, as detailed in Table 1.
Figure 12: Chromosomal abnormalities induced by cadmium nitrate in Nigella sativa L. (A) Metaphase I (control), (B) Anaphase I (control), (C) Telophase I (control), (D) Telophase II (control), (E) Chromatin bridge at anaphase I, (F) Stickiness at anaphase I, (G) Laggard at telophase I, (H) Trinucleate condition and (I) Disturbed polarity at telophase II
The SEM microphotographs depicted variations in stomatal length and width of Nigella sativa leaves (Fig. 13). In control plants, the stomatal aperture measured 24.63 μm length and 5.82 μm width in var. AN20, and 20.21 μm length and 4.07 μm width in var. desi. Under cadmium nitrate treatment, these dimensions decreased significantly: in var. AN20, to 16.98 μm length and 2.67 μm width at 50 ppm Cd(NO3)2, and in var. desi, to 17.94 μm length and 1.96 μm width at 30 ppm Cd(NO3)2. Cadmium nitrate exhibited more evident lethal causes on the stomata of var. desi compared to var. AN20.
Figure 13: SEM photomicrographs of stomatal aperture in Nigella sativa L. (A) AN20 (control), (B) stomatal aperture under 50 ppm Cd(NO3)2, (C) Desi (control), (D) stomatal aperture under 30 ppm Cd(NO3)2
The analysis of extracts from Nigella sativa seeds revealed a diverse range of phytochemicals. In the GC–MS chromatogram of methanolic extracts, control samples of both var. AN20 and var. desi showed 12–13 peaks, whereas variants showed 30–35 peaks in var. AN20 (Fig. 14) and maintained 12–13 peaks in var. desi (Fig. 15). These peaks were identified as bioactive compounds. The peak area percent in the GC-MS chromatogram indicates the relative abundance or concentration of these compounds within the samples.
Figure 14: GC-MS chromatogram of the methanolic extract of Nigella sativa L. seeds (A) AN20 (control) (B) 30 ppm Cd(NO3)2
Figure 15: GC-MS chromatogram of the methanolic extract of Nigella sativa L. seeds (A) Desi (control) (B) 30 ppm Cd(NO3)2
In var. AN20, compounds such as p-Cymene, t-Butylhydroquinone, 1,2-Benzenedicarboxylic acid, bis(2-methylpropyl ester), 9,12-Octadecadienoic acid (Z,Z)-, methyl ester, and n-Hexadecanoic acid were detected, with some compounds appearing exclusively in the variants (Table 2).
In var. desi, compounds detected included p-Cymene, Apocynin, 3-Butyn-1-ol, Thymoquinone, Tetradecanoic acid, 9,12-Octadecadienoic acid (Z,Z)-, methyl ester, Methyl stearate, 9-Tetradecenal (Z)-, among others (Table 2). The area percentages of these compounds varied with different concentrations of Cd(NO3)2, notably changing at 30 ppm.
3.9 Pearson Correlation Heatmap and Pairwise Scatter Plot Matrix
The Pearson correlation coefficients were computed to evaluate the relationships among the parameters of both varieties, and the findings were depicted as a heatmap in Fig. 16. In the heatmap, yellow shades represented strong positive correlations among the parameters, while blue indicated negative correlations. To delve deeper into the linear relationships between specific continuous variables, a pairwise scatter plot matrix with linear regression lines was generated, as shown in Fig. 17. These linear regression lines were fitted to best approximate the data points, clearly illustrating the relationships between the selected variables. This method is commonly used to assess linear associations among continuous variables and provides insights into how traits may correlate with one another.
Figure 16: Pairwise scatter plot matrix with linear regression lines between the two variables
Figure 17: The heat map showing Pearson’s correlation for the quantitative and physiological traits of Nigella sativa L. cultivars AN20 and Desi
Mutation breeding involves deliberately inducing mutations using physical or chemical mutagens to enhance agricultural crops. Achieving a high frequency of desired mutations in mutation breeding programs depends on selecting optimal mutagen concentrations and growth conditions [28]. This approach plays a vital role in increasing genetic diversity within existing germplasm, thereby expanding the genetic base of crops and enabling more effective plant breeding. Cadmium, for instance, can bind to DNA and RNA, disrupting their synthesis and causing mutations. However, by the M6 and M7 generations, plants are no longer exposed to cadmium, allowing natural selection to eliminate harmful traits and ensure safety and stability for consumer use.
In this study, increasing doses of mutagenic treatment inhibited seed germination, plant survival, and pollen fertility, which aligns with findings in other crops like Nigella sativa [29], Ocimum [30], Capsicum annuum [15], and Lens culinaris [31]. The reduction in seed germination and plant growth can be attributed to cadmium’s interference with enzymatic and photosynthetic functions, as well as its impact on membrane integrity [32]. Decreased plant survival may result from cytogenetic damage, physiological disturbances induced by mutagenic treatments, disruptions in growth regulator balance, and chromosomal impairment directing to mitotic arrest [33]. Pollen fertility decline is linked to abnormal meiosis, which generates abnormal gametes due to structural and physiological changes in pollen grains. Such irregular meiosis, including spindle-induced abnormalities like tripolarity and non-orientation, can produce unbalanced and sterile gametes, ultimately affecting pollen fertility [34].
The mutagen-treated plants revealed substantial variations in chlorophyll and carotenoid concentrations, affecting photosynthetic activity, which is consistent with research on B. juncea [35]. Cadmium (Cd) stress typically reduces photosynthetic pigments and PSII efficiency, hindering energy transfer from chlorophyll a antennae to the reaction center [36]. This impact on energy transfer efficiency suggests a sensitivity of chlorophyll enzymes to Cd, leading to reduced photosynthesis [37]. Cd stress disrupts biosynthetic pathways of photosynthetic pigments, affecting plastid development, photosynthetic efficiency, and overall metabolism, often causing chlorosis and slowed plant growth in contaminated environments [38]. Metals like Cu2+, Zn2+, Cd2+, Hg2+, Pb2+, or Ni2+ can substitute Mg2+ in chlorophyll, disrupting pigmentation and affecting chlorophyll content [39]. Carotenoids act as non-enzymatic antioxidants, protecting chlorophyll, membranes, and cellular genetic material from reactive oxygen species (ROS) during metal stress [40]. Reduced carotenoid levels under metal stress increase plant susceptibility to environmental challenges, while their increase reflects their role in ROS detoxification [41,42].
Heavy metal stress disrupts water balance in plants, leading to a notable increase in proline, which aids in the osmotic regulation of cells [43]. In this study, the rise in proline content in the leaves of Nigella sativa with increasing cadmium (Cd) concentrations suggests a biochemical response to Cd stress. The accumulation of proline acts as a defense mechanism, helping plants cope with adverse environmental conditions. Similarly, under Cd stress in pea plants, proline levels increase while chlorophyll content decreases [44].
In mutation breeding, morphological variations serve as indicators of enhanced traits in crops like Nigella sativa. Researchers have documented a diverse range of morphological mutations, including changes in cotyledonary and vegetative leaves, observed in various crops such as sesame [45], chickpea [46], lablab [47], lentil [12,48] and others. Leaf morphology variations are often attributed to spontaneous gene mutations and DNA recombination [47]. Mutations affecting growth habits, such as dwarf mutants observed in our study and in Cynodon dactylon [49], are linked to chromosome breakage during meiosis, inhibition of DNA synthesis, disruptions in physiological processes, and damage to meristematic cells [49,50]. Floral characteristics, including changes in color, shape, anthers, and carpels, have also been observed in various plants due to induced mutations [51,52]. These variations can arise from genetic changes affecting flowering pathways or mutations induced by mutagens in genes related to floral development [53]. Capsule and seed variations, considered macro mutations, exhibit distinct phenotypic traits influenced by oligogenic traits and are qualitatively inherited. Examples include large capsules and seeds with varying seed colors observed in mutants induced in Linum usitatissimum L. [54], and changes in locular numbers in Nigella sativa [55].
The quantitative attributes such as plant height (cm), fertile branches per plant, capsules per plant, seeds per capsule, 1000 seed weight (g), and seed yield per plant (g) show a consistent decrease with increasing concentrations of Cd(NO3)2 [15]. Similar reductions in plant height under Cd stress have been studied in Brassica spp. [56], indicating the detrimental consequences of heavy metals like Cd, Pb, and Hg on plant growth and yield [57]. Both chromosomal breakage and physiological disruptions contribute to the decrease in quantitative traits [58]. Reductions in the fertile branches lead to fewer capsules per plant [59]. There is a direct association between the average number of seeds per capsule and total seed production per plant, both of which decrease due to mutagenic inhibition. Seed weight, critical for predicting crop yield, also decreases under mutagenic treatments, consistent with findings in Vigna mungo [58]. The decrease in yield is attributed to abnormal meiotic cycles induced by mutagens, which affect spore production and fruit set. Similar effects have been documented in Capsicum annuum [15,51] and Trigonella [14].
The Fig. 12 illustrates various chromosomal alterations induced by genotoxic chemicals, including stickiness, laggards, trinucleate condition, disturbed polarity, and chromatin bridges. Heavy metals such as cadmium exert toxic effects on cell division, leading to chromosomal aberrations [60]. Chromosome bridges and spindles often result from the formation of dicentric chromosomes through breakage-fusion-bridge cycles [61]. Anaphase bridging can be induced by chromosome adhesion, dicentric chromosome formation, exchange-type aberrations, and translocation of chromosomal segments [62]. Laggards observed during anaphase and telophase in cadmium-treated plants occur when chromosomes fail to attach to spindle fibers properly, potentially migrating incorrectly [63]. Spindle disturbances contribute to disrupted polarity during anaphase and telophase, as noted in Nigella sativa [64] and Capsicum annuum L. [51]. These findings suggest that heavy metals, used as mutagens, induce genetic and phenotypic diversity in Nigella sativa. Such variants could potentially be selected as beneficial lines for mutation breeding programs in the future.
In this study, cadmium nitrate-treated stomata in Nigella sativa exhibited a noteworthy closure and reduction in size compared to the control, as observed through SEM analysis. These conclusions are consistent with previous researchers, who also observed similar effects of stress on Nigella sativa stomata [65]. The observed abnormalities, including decreased stomatal density, closure, and increased resistance, likely contribute to reduced photosynthesis, stomatal conductance, and transpiration rates. Significant variations in stomatal characteristics is also reported in groundnut plants exposed to heavy metals [66]. Stomatal opening is typically regulated by ions such as K+ and Ca2+ [67], although in some cases, like Vicia faba, potassium ions may not regulate stomatal closure [68]. A decrease in stomatal aperture size with increasing Cd concentrations, attributing this to changes in calcium and potassium ion movement through guard cells due to their high permeability [69]. Cd stress affects guard cells in an auxin-independent manner, further influencing stomatal behavior [70]. Studies on P. vulgaris exposed to Cd have indicated that Cd can inhibit stomatal opening through various mechanisms depending on the dose and duration of exposure [71], highlighting the varying degrees of Cd toxicity experienced by plants.
Natural plants and their components have shown medicinal potential [72]. GC-MS is effective for identifying bioactive compounds like long-chain hydrocarbons, alcohols, esters, acids, steroids, alkaloids, amino acids, and nitro compounds [73]. Growing sweet basil in Cd and Pb-contaminated soils may enhance essential oil production and composition despite affecting seed germination and plant traits [74]. Summer savory (Satureja hortensis L.) produces more phytoconstituents, especially carvacrol, with varying cadmium levels, making it suitable for mildly contaminated soils [75].
The study indicated that varying concentrations of cadmium nitrate reduced the biological, morphological, as well as some physiological traits of Nigella sativa cultivars AN20 and Desi, leading to chromosomal abnormalities. Notably, the Desi cultivar exhibited greater sensitivity to cadmium nitrate compared to AN20. A significant and proportional rise in proline content was observed with increasing concentrations of Cd(NO3)2. This suggests that Cd(NO3)2 can act as a beneficial factor in enhancing enzyme defense and antioxidant activities but kept in mind its potential adverse effects on quantitative growth parameters. SEM revealed changes in stomatal morphology, while GC-MS identified varied phytocompound % areas in treated variants, suggesting potential for enhanced bioactivity. Using Cd(NO3)2 as a mutagen can boost stress resistance, induce genetic variation, improve adaptation to contaminated soils, promote metabolic changes, advance research in plant biotechnology, aid in bioremediation, and assist in selecting hardier plant varieties. Subsequent generations may accumulate mutations that modify the genetics of Nigella sativa, enhance phytocompound levels, and be advantageous for future breeding programs.
Acknowledgement: The authors are grateful to the Department of Botany, Aligarh Muslim University, Aligarh, India for providing required facilities during the study of the research. This research was funded by the Princess Nourah bint Abdulrahman University Researchers Supporting Project number (PNURSP2024R318), Princess Nourah bint Abdulrahman University, Riyadh, Saudi Arabia.
Funding Statement: This research was funded by the Princess Nourah bint Abdulrahman University Researchers Supporting Project number (PNURSP2024R318), Princess Nourah bint Abdulrahman University, Riyadh, Saudi Arabia.
Author Contributions: Parul Verma: Conceptualization, Methodolgy, Writing—original draft formal analysis; Sana Choudhary: Supervision, Writing—review and editing; Neha Naaz: Methodology, Investigation, Writing—review and editing; Nidhi Sharma: Formal analysis, Data quration, Writing—review and editing; Nora M. Al Aboud: Funding acquisition, Writing—review and editing; Fatmah Ahmed Safhi: Funding acquisition, Writing—review and editing; Diaa Abd El Moneim: Review and editing. All authors reviewed the results and approved the final version of the manuscript.
Availability of Data and Materials: All data generated or analyzed during this study are included in this published article.
Ethics Approval: Not applicable.
Conflicts of Interest: The authors declare no conflicts of interest to report regarding the present study.
References
1. Jimoh MA, Jimoh MO, Saheed SA, Bamigboye SO, Laubscher CP, Kambizi L. Commercialization of medicinal plants: opportunities for trade and concerns for biodiversity conservation. Sustainable Uses Prospects Med Plants. 2023 May 5;309–32.
2. Kant K, Anwer MM, Meena SR, Mehta RS. Advance production technology of Nigella. In: ICAR. Ajmer: National Research Centre on Seed Spices Tabiji; 2009.
3. Ahmad MF, Ahmad FA, Ashraf SA, Saad HH, Wahab S, Khan MI, et al. An updated knowledge of Black seed (Nigella sativa Linn.review of phytochemical constituents and pharmacological properties. J Herb Med. 2021 Feb 1;25:100404. doi:10.1016/j.hermed.2020.100404.
4. Tiwari G, Gupta M, Devhare LD, Tiwari R. Therapeutic and phytochemical properties of thymoquinone derived from Nigella sativa. Curr Drug Abuse Rev. 2024 Jul 1;16(2):145– 56. doi:10.2174/2589977515666230811092410.
5. Tran TA, Popova LP. Functions and toxicity of cadmium in plants: recent advances and prospects. Turkish J Bot. 2013;37(1):1–3. doi:10.3906/bot-1112-16.
6. Kanwar VS, Sharma A, Srivastav AL, Rani L. Phytoremediation of toxic metals present in soil and water environment: a critical review. Environ Sci Poll Res. 2020 Dec;27:44835–60. doi:10.1007/s11356-020-10713-3.
7. Jan S, Parray JA, Jan S, Parray JA. Heavy metal stress signalling in plants. Approach Heavy Metal Toler Plants. 2016:33–55. doi:10.1007/978-981-10-1693-6_3.
8. Ekoa Bessa AZ, Ngueutchoua G, Kwewouo Janpou A, El-Amier YA, Njike Njome Mbella Nguetnga OA, Kankeu Kayou UR, et al. Heavy metal contamination and its ecological risks in the beach sediments along the Atlantic Ocean (Limbe coastal fringes, Cameroon). Earth Syst Environ. 2021 Jun;5:433–44. doi:10.1007/s41748-020-00167-5.
9. Shafique F, Ali Q, Malik A. Effects of heavy metal toxicity on maze seedlings growth traits. Biol Clin Sci Res J. 2020;1. doi:10.54112/bcsrj.v2020i1.27.
10. Rai PK, Lee SS, Zhang M, Tsang YF, Kim KH. Heavy metals in food crops: health risks, fate, mechanisms, and management. Environ Int. 2019 Apr 1;125:365–85. doi:10.1016/j.envint.2019.01.067.
11. Lone MI, He ZL, Stoffella PJ, Yang XE. Phytoremediation of heavy metal polluted soils and water: progresses and perspectives. J Zhejiang Univ Sci B. 2008 Mar;9(3):210–20. doi:10.1631/jzus.B0710633.
12. Shahwar D, Ansari MY, Choudhary S. Induction of phenotypic diversity in mutagenized population of lentil (Lens culinaris Medik) by using heavy metal. Heliyon. 2019 May 1;5(5):e01722. doi:10.1016/j.heliyon.2019.e01722.
13. Chandra R, Kang H. Mixed heavy metal stress on photosynthesis, transpiration rate, and chlorophyll content in poplar hybrids. For Sci Technol. 2016 Apr 2;12(2):55–61. doi:10.1080/21580103.2015.1044024.
14. Choudhary S, Ansari MY, Khan Z, Gupta H. Cytotoxic action of lead nitrate on cytomorphology of Trigonella foenum-graecum L. Turkish J Biol. 2012;36(3):267–73.
15. Hasan N, Choudhary S, Laskar RA, Naaz N, Sharma N. Comparative study of cadmium nitrate and lead nitrate [Cd(NO3)2 and Pb(NO3)2] stress in cyto-physiological parameters of Capsicum annuum L. Horticul, Environ, Biotechnol. 2022 Oct;63(5):627–41. doi:10.1007/s13580-021-00417-z.
16. Kumbhakar DV, Datta AK, Das D, Ghosh B, Pramanik A, Gupta S. Assessment of cytotoxicity and cellular apoptosis induced by azo-dyes (methyl orange and malachite green) and heavy metals (cadmium and lead) using Nigella sativa L. (black cumin). Cytologia. 2018 Sep 25;83(3):331–6. doi:10.1508/cytologia.83.331.
17. Vladimirovich DA, Grigorievich DV, Sergeevna DN. The assessment of cadmium nitrate effect on morphological and cytogenetic indices of spring barley (Hordeum vulgare) seedlings. Braz J Bot. 2021 Mar;44(1):43–56. doi:10.1007/s40415-020-00679-4.
18. Sharifi Rad J, Sharifi Rad M, da Silva JA T. Effects of exogenous silicon on cadmium accumulation and biological responses of Nigella sativa L. (Black Cumin). Commun Soil Sci Plant Anal. 2014 Aug 6;45(14):1918–33. doi:10.1080/00103624.2014.909835.
19. Siddique A, Dubey AP. Phyto-toxic effect of heavy metal (CdCl2) on seed germination, seedling growth and antioxidant defence metabolism in wheat (Triticum aestivum L.) variety HUW-234. Int J Bioresour Stress Manage. 2017 Apr 7;8(2):261–7. doi:10.23910/IJBSM.
20. Xu J, Yin H, Li X. Protective effects of proline against cadmium toxicity in micropropagated hyperaccumulator, Solanum nigrum L. Plant Cell Rep. 2009 Feb;28:325–33. doi:10.1007/s00299-008-0643-5.
21. Espanany A, Fallah S, Tadayyon A. Seed priming improves seed germination and reduces oxidative stress in black cumin (Nigella sativa) in presence of cadmium. Ind Crops Prod. 2016 Jan 1;79:195–204. doi:10.1016/j.indcrop.2015.11.016.
22. AL-Sanabani EJ, Alhadi FA, Al-Amary KM, Hussain MA. Effect of Cd and Pb on germination and early seedling growth of black seed (Nigella sativa L.). Univ Aden J Nat Appl Sci. 2018;22(1):87–97. doi:10.47372/uajnas.2018.n1.a07.
23. Bhoi A, Yadu B, Chandra J, Keshavkant S. Mutagenesis: a coherent technique to develop biotic stress resistant plants. Plant Stress. 2022 Jan 1;3:100053. doi:10.1016/j.stress.2021.100053.
24. Mackinney G. Absorption of light by chlorophyll solutions. J Biol Chem. 1941 Aug 1;140(2):315–22. doi:10.1016/S0021-9258(18)51320-X.
25. Arnon DI. Copper enzymes in isolated chloroplasts. Polyphenoloxidase in Beta vulgaris. Plant Physiol. 1949 Jan;24(1):1. doi:10.1104/pp.24.1.1.
26. Bates LS, Waldren RP, Teare ID. Rapid determination of free proline for water-stress studies. Plant Soil. 1973 Aug;39:205–7. doi:10.1007/BF00018060.
27. Nivetha K, Prasanna G. GC-MS and FT-IR analysis of Nigella sativa L. seeds. Int J Adv Res Biol Sci. 2016;3(6):45–54.
28. Arisha MH, Liang BK, Shah SM, Gong ZH, Li DW. Kill curve analysis and response of first generation Capsicum annuum L. B12 cultivar to ethyl methane sulfonate. Genet Mol Res. 2014 Nov 28;13(4):10049–61. doi:10.4238/2014.November.28.9.
29. Tantray AY, Raina A, Khursheed S, Amin RU, Khan SA. Chemical mutagen affects pollination and locule formation in capsules of black cumin (Nigella sativa L.). Int J Agric Sci. 2017;8(1):108–17.
30. Petrescu I, Sarac I, Bonciu E, Madosa E, Rosculete CA, Butnariu M. Study regarding the cytotoxic potential of cadmium and zinc in meristematic tissues of basil (Ocimum basilicum L.). Caryologia. 2020 Mar 13;73(1):75–81.
31. Shahwar D, Khan Z, Ansari MY, Park Y. Relative mutagenic effectiveness and efficiency of chemical mutagens (Caffeine and EMS) and heavy metals [(Pb(NO3)2 and Cd(NO3)2)] in developing chlorophyll and morphological mutants in lentil. Mutat Res/Genetic Toxicol Environ Mutagen. 2023 Aug 1;890:503668. doi:10.1016/j.mrgentox.2023.503668.
32. Zulfiqar U, Ayub A, Hussain S, Waraich EA, El-Esawi MA, Ishfaq M, et al. Cadmium toxicity in plants: recent progress on morpho-physiological effects and remediation strategies. J Soil Sci Plant Nutr. 2022 Mar;22(1):212–69. doi:10.1007/s42729-021-00645-3.
33. Khursheed S, Laskar RA, Raina A, Amin R, Khan S. Comparative analysis of cytological abnormalities induced in Vicia faba L. genotypes using physical and chemical mutagenesis. Chromosome Sci. 2015;18(3–4):47–51.
34. Taziun T, Laskar RA, Amin R, Parveen SK. Effects of dosage and durations of different mutagenic treatment in lentil (Lens culinaris Medik.) cultivars Pant L 406 and DPL 62. Legume Res. 2018;41(4):500–9. doi:10.18805/LR-3757.
35. Kohli SK, Handa N, Sharma A, Kumar V, Kaur P, Bhardwaj R. Synergistic effect of 24-epibrassinolide and salicylic acid on photosynthetic efficiency and gene expression in Brassica juncea L. under Pb stress. Turkish J Biol. 2017;41(6):943–53. doi:10.3906/biy-1707-15.
36. Ralph PJ, Burchett MD. Photosynthetic response of Halophila ovalis to heavy metal stress. Environ Pollut. 1998 Oct 1;103(1):91–101. doi:10.1016/S0269-7491(98)00121-3.
37. Aqeel M, Khalid N, Tufail A, Ahmad RZ, Akhter MS, Luqman M, et al. Elucidating the distinct interactive impact of cadmium and nickel on growth, photosynthesis, metal-homeostasis, and yield responses of mung bean (Vigna radiata L.) varieties. Environ Sci Pollut Res. 2021 Jun;28:27376–90. doi:10.1007/s11356-021-12579-5.
38. Rai R, Agrawal M, Agrawal SB. Impact of heavy metals on physiological processes of plants: with special reference to photosynthetic system. Plant Responses Xenobiot. 2016;127–40. doi:10.1007/978-981-10-2860-1_6.
39. Küpper H, Küpper F, Spiller M. Environmental relevance of heavy metal-substituted chlorophylls using the example of water plants. J Exp Bot. 1996 Feb 1;47(2):259–66. doi:10.1093/jxb/47.2.259.
40. Hou W, Chen X, Song G, Wang Q, Chang CC. Effects of copper and cadmium on heavy metal polluted waterbody restoration by duckweed (Lemna minor). Plant Physiol Biochem. 2007 Jan 1;45(1):62–9. doi:10.1016/j.plaphy.2006.12.005.
41. Zhang H, Xu Z, Guo K, Huo Y, He G, Sun H, et al. Toxic effects of heavy metal Cd and Zn on chlorophyll, carotenoid metabolism and photosynthetic function in tobacco leaves revealed by physiological and proteomics analysis. Ecotoxicol Environ Saf. 2020 Oct 1;202:110856. doi:10.1016/j.ecoenv.2020.110856.
42. Cazzaniga S, Li Z, Niyogi KK, Bassi R, Dall’Osto L. The Arabidopsis szl1 mutant reveals a critical role of β-carotene in photosystem I photoprotection. Plant Physiol. 2012 Aug 1;159(4):1745–58. doi:10.1104/pp.112.201137.
43. Rady MM, Hemida KA. Modulation of cadmium toxicity and enhancing cadmium-tolerance in wheat seedlings by exogenous application of polyamines. Ecotoxicol Environ Saf. 2015 Sep 1;119:178–85. doi:10.1016/j.ecoenv.2015.05.008.
44. Zdunek-Zastocka E, Grabowska A, Michniewska B, Orzechowski S. Proline concentration and its metabolism are regulated in a leaf age dependent manner but not by abscisic acid in pea plants exposed to cadmium stress. Cells. 2021 Apr 20;10(4):946. doi:10.3390/cells10040946.
45. Nura S, Adamu AK, Mu’Azu S, Dangora DB, Shehu K, Mahamud FM, et al. Assessment of the Growth Responses of Sesame (Sesamum indicum L.) and False Sesame (Ceratotheca sesamoides Endl.) to Colchicine Treatments. Am J Exp Agric. 2014 Mar 25;4(8):902–12. doi:10.9734/AJEA/2014/6480.
46. Laskar RA, Khan S, Khursheed S, Raina A, Amin R. Quantitative analysis of induced phenotypic diversity in chickpea using physical and chemical mutagenesis. J Agron. 2015 Jul 1;14(3):102. doi:10.3923/ja.2015.102.111.
47. More AD, Jagtap SS. Induction of morphological leaf mutations in Lablab purpureus (L.) sweet through chemical and physical mutagens. Int J Curr Microbiol Appl Sci. 2016;5(10):592–7. doi:10.20546/ijcmas.
48. Yousuf J, Raina A, Rasik S, Reshi ZA, Shahwar D. Comparative effects of caffeine and lead nitrate on the bio-physiological and yield associated traits of lentil (Lens culinaris Medik.). Heliyon. 2023 Jun 1;9(6). doi:10.1016/j.heliyon.2023.e16351.
49. Abdali Dehdezi A, Alaei E, Azadi P, Shavandi M, Mousavi SA. Role of phytoremediation in reducing cadmium and nickle toxicity in soil using species (Cynodon dactylon L.). J Human Environ Health Promot. 2021 Dec 10;7(4):213–20. doi:10.52547/jhehp.7.4.213.
50. Ramya B, Nallathambi G, Ram SG. The effect of mutagens on M1 population of black gram (Vigno mungo L. Hepper). Afr J Biotechnol. 2014 Oct 31;13(8):951–6. doi:10.5897/AJB2013.12785.
51. Aslam R, Bhat TM, Choudhary S, Ansari MY, Shahwar D. Estimation of genetic variability, mutagenic effectiveness and efficiency in M2 flower mutant lines of Capsicum annuum L. treated with caffeine and their analysis through RAPD markers. J King Saud Univ-Sci. 2017 Jul 1;29(3):274–83. doi:10.1016/j.jksus.2016.04.008.
52. Asif A, Ansari K, Hashem MY, Tabassum A, Abd_Allah B, Ahmad EF, et al. Proteome profiling of the mutagen-induced morphological and yield macro-mutant lines of Nigella sativa L. Plants. 2019 Sep 2;8(9):321. doi:10.3390/plants8090321.
53. Devi AS, Mullainathan L. Effect of gamma rays and ethyl methane sulphonate (EMS) in M3 generation of blackgram (Vigna mungo L. Hepper). Afr J Biotechnol. 2012;11(15):3548–52. doi:10.5897/AJB10.1773.
54. Ahmad T, Ansari MY, Asif A. Mutagenic effect of 6-Aminopurine (Base Analogue) on the quantitative and qualitative traits of Linum Usitatissimum L. Int J Sci Res. 2017;6(9):2277–8179.
55. Asif A. Mutagenesis in medicinal plants. In: Omics studies of medicinal plants. Boca Raton, Florida, USA: CRC Press; 2023 Feb 21. p. 211–41.
56. Ahmad P, Sarwat M, Bhat NA, Wani MR, Kazi AG, Tran LS. Alleviation of cadmium toxicity in Brassica juncea L. (Czern. & Coss.) by calcium application involves various physiological and biochemical strategies. PLoS One. 2015 Jan 28;10(1):e0114571. doi:10.1371/journal.pone.0114571.
57. Saleem MH, Fahad S, Khan SU, Din M, Ullah A, Sabagh AE, et al. Copper-induced oxidative stress, initiation of antioxidants and phytoremediation potential of flax (Linum usitatissimum L.) seedlings grown under the mixing of two different soils of China. Environ Sci Pollut Res. 2020 Feb;27:5211–21. doi:10.1007/s11356-019-07264-7.
58. Thilagavathi C, Mullainathan L. Influence of physical and chemical mutagens on quantitative characters of Vigna mungo (L. Hepper). Int Multidiscip Res J. 2011 May 22;1(1):6–8.
59. Panigrahi KK, Ayesha M, Bhabendra B. Mutagenic efficiency and effectiveness of gamma rays, ethyl methane sulphonate (EMSnitrosoguanidine (NG) and their synergistic effect for different polygenic traits in black gram (Vigna mungo (L.) hepper) through induced mutagenesis. Int J Plant, Anim Environ Sci. 2015;5(1):292–9.
60. Pizzaia D, Nogueira ML, Mondin M, Carvalho ME, Piotto FA, Rosario MF, et al. Cadmium toxicity and its relationship with disturbances in the cytoskeleton, cell cycle and chromosome stability. Ecotoxicology. 2019 Nov;28:1046–55. doi:10.1007/s10646-019-02096-0.
61. Murnane JP. Telomere dysfunction and chromosome instability. Mutation Res/Fundam Mol Mech Mutagen. 2012 Feb 1;730(1–2):28–36. doi:10.1016/j.mrfmmm.2011.04.008.
62. Bhat TA, Parveen S, Khan AH. Meiotic studies in two varieties of Vicia faba L. (Fabaceae) after EMS treatment. Asian J Plant Sci. 2007 Feb 28;61(1):51–5.
63. Khanna N, Sharma S. Allium cepa root chromosomal aberration assay: a review. Indian J Pharm Biol Res. 2013 Mar 31;1(3):105–19. doi:10.30750/ijpbr.1.3.15.
64. Amin R, Laskar RA, Khursheed S, Raina A, Khan S. Genetic sensitivity towards MMS mutagenesis assessed through in vitro growth and cytological test in Nigella sativa L. Life Sci Int Res J. 2016;3:2347–8691.
65. Arif Y, Singh P, Siddiqui H, Shiraz M, Mir AR, Alam P, et al. Comparative study of stress generated by osmolytes on the growth, photosynthesis and metabolic responses in Nigella sativa. Biocatal Agric Biotechnol. 2023 Sep 1;52:102818. doi:10.1016/j.bcab.2023.102818.
66. Orcen N. Stomatal parameters and growth responses of nicotiana and atriplex to Cd, Pb and Cd-Pb-contaminated soil. Fresen Environ Bull. 2017 Jan 1;26:6340–5.
67. Osakabe Y, Yamaguchi-Shinozaki K, Shinozaki K, Tran LS. ABA control of plant macroelement membrane transport systems in response to water deficit and high salinity. New Phytol. 2014 Apr;202(1):35–49. doi:10.1111/nph.12613.
68. Rucińska-Sobkowiak R. Water relations in plants subjected to heavy metal stresses. Acta Physiol Plant. 2016 Nov;38:1–3. doi:10.1007/s11738-016-2277-5.
69. Kaznina NM, Titov AF, Batova YV, Laidinen GF. The resistance of plants Setaria veridis (L.) Beauv. to the influence of cadmium. Biol Bull. 2014 Sep;41:428–33. doi:10.1134/S1062359014050045.
70. Perfus-Barbeoch L, Leonhardt N, Vavasseur A, Forestier C. Heavy metal toxicity: cadmium permeates through calcium channels and disturbs the plant water status. Plant J. 2002 Nov;32(4):539–48. doi:10.1046/j.1365-313X.2002.01442.x.
71. Januškaitienė I. The dynamics of photosynthetic parameters of Phaseolus vulgaris and Vicia fabo under strong cadmium stress. Biologija. 2014 Oct 29;60(3). doi:10.6001/biologija.v60i3.2975.
72. Khalaf NA, Shakya AK, Al-Othman A, El-Agbar Z, Farah H. Antioxidant activity of some common plants. Turkish J Biol. 2008;32(1):51–5.
73. Muthulakshmi AR, Mohan VR. GC-MS analysis of bioactive components of Feronia elephantum Correa (Rutaceae). J Appl Pharm Sci. 2012 Feb 27;2:69–74.
74. Fattahi B, Arzani K, Souri MK, Barzegar M. Effects of cadmium and lead on seed germination, morphological traits, and essential oil composition of sweet basil (Ocimum basilicum L.). Ind Crops Prod. 2019 Oct 5;138:111584. doi:10.1016/j.indcrop.2019.111584.
75. Azizollahi Z, Ghaderian SM, Ghotbi-Ravandi AA. Cadmium accumulation and its effects on physiological and biochemical characters of summer savory (Satureja hortensis L.). Int J Phytoremediation. 2019 Oct 15;21(12):1241–53. doi:10.1080/15226514.2019.1619163.
Cite This Article
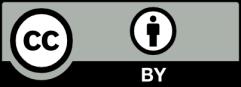
This work is licensed under a Creative Commons Attribution 4.0 International License , which permits unrestricted use, distribution, and reproduction in any medium, provided the original work is properly cited.