Open Access
ARTICLE
Improving Okra Performance: Biostimulants and their Influence on Yield, Quality, Physiology, and Nutrient Composition
1 Department of Soil Science, Faculty of Agricultural Sciences and Technology, Bahauddin Zakariya University, Multan, 60800, Pakistan
2 Department of Biological and Environmental Sciences, Emerson University, Multan, 60000, Pakistan
3 Sichuan Provincial Key Laboratory of Philosophy and Social Sciences for Monitoring and Evaluation of Rural Land Utilization, Chengdu Normal University, Chengdu, 611130, China
4 College of Agronomy and Life Sciences, Zhaotong University, Zhaotong, 657000, China
5 Department of Zoology, College of Science, King Saud University, Riyadh, 11451, Saudi Arabia
* Corresponding Authors: Abdur Rehim. Email: ; Muhammad Amjad Bashir. Email:
Phyton-International Journal of Experimental Botany 2024, 93(12), 3531-3548. https://doi.org/10.32604/phyton.2024.057356
Received 15 August 2024; Accepted 27 November 2024; Issue published 31 December 2024
Abstract
Chemical fertilizers have contributed to improving crop production and coping with food safety challenges, but their excessive application in agriculture has resulted in soil and environmental problems. The present study was performed to assess the potential of biostimulants as an alternative to chemical fertilizers. A pot experiment was conducted to examine the response of okra against different biostimulants, including glycine (G.L.), lysine (L.Y.), aspartic acid (A.A.), vitamin B complex (V.B.), and chemical fertilizers (control; C.K.). The results revealed that G.L. and V.B. significantly improved chlorophyll a (12.51%) and chlorophyll b (7.14%) contents in okra leaves compared to C.K. V.B. (44.54%) and A.A. (43.15%) significantly improved the plant’s fresh weight. Plant dry weight was significantly increased with V.B. (66.80%) followed by A.A. (32.05%) and G.L. (13.24%). Total phenolic compounds were significantly improved in okra pods with V.B. (32.56%), followed by A.A. (14.99%). In addition, G.L. (126.74%) significantly improved the protein content, followed by L.Y. (106.55%), V.B. (82.26%), and A.A. (69.28%). Moreover, L.Y. (54.48%) and G.L. (37.46%) also increased the ascorbic acid content in pods. The highest N concentration in okra shoots was observed with V.B. (87.42%), followed by G.L. (68.55%) and L.Y. (59.75%). In addition, A.A. (67.27%) and V.B. (54.55%) improved the N concentration in okra pods compared to C.K. L.Y. (48.30%) significantly improved the total P concentration in the shoot and G.L. improved the P concentration in the pod (12.64%). Although C.K. produced the maximum okra pod fresh weight, biostimulants (V.B., G.L., A.A.) also performed well with minimal loss in yield. The study concludes that biostimulants application can significantly affect the plant’s physiological parameters, quality of vegetables, and production.Keywords
Chemical fertilizers play an integral role in improving crop productivity, but they also alter the soil’s physicochemical and biological properties. The continuous employment of chemical fertilizers results in soil health and quality degradation. For instance, the overuse of chemical fertilizers diminishes soil nutrients, hardens soils, pollutes soil, air, and water, and even causes greenhouse gas emissions [1]. The use of Nitrogenous fertilizers has rapidly increased globally and are expected to increase four to fivefold by 2050 [2]. Although it significantly contributes to yield enhancement, it also reduces nitrogen use efficiency, increases nitrate contamination, nitrate leaching and contamination of groundwater, eutrophication, and nitrous oxide volatilization, causing global warming and a high risk of environmental pollution [3].
The uncontrolled nitrogen (N) application without understanding the crop demand also increases N concentration in groundwater, thereby increasing microbial activity and promoting the release of arsenic from peat sediments [4]. Moreover, it has also been reported that more than 50% of the N fertilizers remained unused and lost through leeching due to inefficient uptake of nutrients [5]. In this scenario, a suitable alternative must be adopted to reduce the N-associated problems without compromising the crop yield.
Biostimulants are organic/inorganic substances that have the potential to modify plants’ physiological processes in such a way that they benefit the growth or, development or stress response of plants [6]. Moreover, it aims to achieve one or more characteristics of the plants, including nutrient use efficiency (NUE), quality characteristics, nutrient uptake, and/or resistance against environmental stresses [7]. Numerous studies have reported that biostimulants can improve plant growth and help plants survive in reduced fertilizer conditions. A study reported that the application of a biostimulant (Viva®) has the capacity to reduce the chemical fertilization dose; it also stated that biostimulants enable tomato plants to grow well under reduced fertilization (NPK) without compromising fruit quality and yield [6].
Moreover, RootsTM is an organic biostimulant that can stimulate plant growth and provide optimum yield with up to 50% lower chemical fertilization [8]. Biostimulants also have the ability to reduce or completely replace chemical fertilizers, along with a significant increase in radish yield under controlled conditions [9]. Furthermore, the use of biostimulants under normal environmental conditions and reduced/absence of chemical fertilizers has also been reported [10]. The quality and proximal composition of watermelon were also improved with the use of biostimulants, which were identified as a sustainable agricultural practice [11]. In addition, biostimulants induce major physiological changes in plants due to hormones including amino acids, cytokinin, and Indole-3-acetic acid [12]. The improved plant quality can be associated with the better uptake of nutrients that might be influenced by the expression of membrane transporters controlling micro-nutrient uptake and distribution in plants [13].
The application of amino acids can positively influence vegetative, reproductive, and quality traits and improve plant growth [14]. Glycine is proteinogenic amino acid that works as a signal transduction molecule and readily improves nutrient uptake in plants [15,16]. Lysine is also an amino acid that helps plants to build resistance against biotic and abiotic stresses through the saccharopine pathway [17]. Aspartic acid is another amino acid involved in protein synthesis as well as other amino acids, sugars, and hormones, along with nitrogen assimilation and synthesis of other nitrogen-containing molecules [18]. Vitamin B plays an important role in primary metabolism in plants; it develops resistance against abiotic stresses and stimulates root growth [19].
Okra (Abelmoschus esculentus L.), also known as Lady’s finger, belongs to the Malvaceae family and is cultivated in different geographical regions of the globe. The major geographical regions of the okra seed market are Europe, Asia-Pacific, and North America. It was also estimated that by the end of 2023, the okra-based nutraceutical market will reach a worth of 222.9 million USD [20]. In the Asia Pacific region, the dominant producers of okra seeds include Pakistan, Malaysia, India, and the Philippines [20]. Okra crop is grown in an area of 15,081 ha in Pakistan with an annual production of 114,657 tons [21].
Okra has numerous health benefits as it is a rich source of phenols, nutrients, vitamins, and unsaturated fatty acids [22]. It is a multipurpose crop cultivated for garden and commercial farming purposes. Its buds, flowers, leaves, pods, stem, and seeds are in high demand for its food consumption and medicinal benefits [22]. Besides this, it is a hardy crop with an adaptability to grow and survive in tropical and sub-tropical regions, but there is limited literature on this aspect [23]. To the authors’ knowledge, there is no existing literature on prior experiments investigating the role of exogenously applied biostimulants on okra crop physiology, quality, nutrition, and yield in the absence of chemical fertilizers under ambient conditions.
The objective of this study was to: (i) investigate the performance of okra against the exogenous application of biostimulants, (ii) find the potential of biostimulants to replace chemical fertilizers in normal environmental conditions, and (iii) estimate the efficacy of biostimulants as agricultural input in vegetable production.
A pot experiment was conducted to assess the role of biostimulants on yield, quality, and physiology of okra at the research area of the Department of Soil Science, Bahauddin Zakariya University, Multan, Pakistan. Plant biometric, physiological, quality, and nutritional parameters and soil properties were studied. The experiment was performed from May to August 2021. About 20 kg of soil was filled into the pots having the following physicochemical properties: pH 7.8, EC 0.1 dS·m−1, soil organic matter 0.7%, saturation percentage 28%, texture sandy loam, N 450 mg kg−1, P 7.1 mg kg−1, and K 62.34 mg kg−1. The experiment consisted of five treatments, including recommended chemical fertilizer (C.K.; N 86.4 kg ha−1, P 74.1 kg ha−1, K 61.8 kg ha−1), glycine (G.L.; 3.51 g pot−1), lysine (L.Y.; 6.82 g pot−1), aspartic acid (A.A.; 16.18 g pot−1), and vitamin B complex (V.B.; 0.65 g pot−1). Each treatment was replicated thrice, and pots were arranged in a completely randomized design (CRD). The doses of biostimulants were according to the crop’s N requirements (86.4 kg ha−1) following our previous study [19].
Six okra seeds (cultivar; “Hybrid Gala 455-F1”, AgriTech, Multan, Pakistan) were sown in each pot on 25 May 2021. Smaller seedlings were later thinned out, and only two plants per pot were maintained. The foliar application was performed four times (25-day intervals starting from 20 June 2021) in the morning on sunny and windless days. Regular manual weed eradication using the hand weeding method and irrigation practices was maintained during the growth period. Okra is a multi-picker; therefore, fruits were collected thrice from 15 September to 01 November. The crop was harvested on 01 November 2021. Moreover, soil samples from each pot were collected and stored for analysis at 4°C for a week.
2.2.1 Physiological Attributes
Chlorophyll contents were determined in okra leaves. Fresh leaf samples were collected from each pot, cut into fine pieces, dipped into ethanol (96% v/v), and placed in darkness for 24 h for chlorophyll extraction. Afterward, the absorbance was measured at 665 and 649 nm using a spectrophotometer (Hitachi UV-Vis U 3000 spectrophotometer, Tokyo, Japan). The same leaves and extracts were used for both absorbance measurements to minimize error. Leaf relative water content was also determined using the following equation. Fresh leaf samples were collected, and their fresh weight was measured. The same leaf was placed in distilled water for 3 h, and its turgid weight was recorded. Later, the leaf was kept in the oven, and the dry weight of the leaf was recorded as per Eq. (1).
RWC=Leaf fresh weight −leaf dry weightleaf turgid weight −leaf dry weight × 100 RWC=Leaf fresh weight −leaf dry weightleaf turgid weight −leaf dry weight × 100 (1)
Plant fresh weight was recorded immediately after harvesting, whereas plants were kept in an oven at 65°C until constant weight was achieved. Afterward, plant dry weight was recorded using a weighing balance. Similarly, okra fruit samples were collected multiple times, and their fresh and dry weight was also recorded. At harvesting, plant height was measured using a measuring tape, the number of leaves was counted, stem diameter was measured with digital Vernier calipers, and leaf area was estimated using the following Eq. (2) [24].
leaf area=[0.34 (leaf length ×leaf width)]1.12 (2)
Fresh okra samples were collected to determine total phenolic contents, total protein contents, and ascorbic acid contents. Total phenolic compounds (TPC) were assessed in root and shoot. The fresh plant samples (0.5 g) were homogenized using ethanol (10 mL, 80%) and then centrifuged (10 min, 4500 rpm). The supernatant (0.1 mL) was taken, deionized water (2 mL), Folin Ciocalteu (F.C.) reagent (0.2 mL), and the samples were mixed using a vortex mixture. Later, 20% sodium carbonate (1 mL) was added and again mixed. Then, the samples were left in the dark for an hour. The absorbance was measured at 765 by standard curve nm using a spectrophotometer [25].
The total protein content in plant samples was determined by the colorimetric method. The fresh plant sample (0.5 g), both root and shoot, was homogenized with 25 mL distilled water using a mortar and pestle and centrifuged at 4500 rpm for 10 min [26]. The supernatant (0.2 mL) was added to an alkaline copper solution (5 mL), mixed well, and left for 10 min. Further, the F.C. reagent (0.5 mL) was added and incubated at room temperature for 30 min. The absorbance was measured at 660 nm wavelength. Bovine serum albumin was used to prepare a calibration graph was prepared. The protein contents were estimated in mg 100 g−1 fresh weight [27]. Ascorbic acid (vitamin C) was also determined. The fresh plant root and shoot samples (0.5 g) were homogenized using 4% oxalic acid (20 mL) using a mortar and pestle and centrifuged at 4500 rpm for 10 min. The supernatant was titrated against Tillmans’ reagent using a calibrated burette until the pink color was developed and sustained for 30 s [28].
Oven dried plant and fruit samples were ground, digested, and analyzed for nutrient concentration. Plant N concentration was determined by Kjeldahl N determination [29]. Meanwhile, P and K concentrations were measured using the colorimetric method [30] and the photometric method [31].
Soil samples from each pot were analyzed in triplicate to eliminate standard error. Soil pH and E.C. were determined using standard methods. Soil pH and E.C. meter were used. Walkley-Black method [30] and Kjeldahl method [31] were used to determine soil organic matter and total N in soil, respectively. Moreover, soil P was extracted with 0.5 M sodium bicarbonate [32] and estimated using a spectrophotometer. Soil K was extracted with IN ammonium acetate solution [33] and concentrations were determined using a flame photometer (PFP7 Industrial Flame Photometer 110 V, Jenway, Garforth, England). All the instruments were calibrated using standard solutions. A sample of known concentration was also tested to ensure the correctness of the instruments.
To estimate soil urease activity, a 5 g moist soil sample was taken, urea solution was added (2.5 mL), and incubated for 2 h at 37°C. Later, potassium chloride (KCl) solution (50 mL) was poured into it, shaken for 30 min, and then filtered. For the colorimetric determination of released ammonia, filtrate (1 mL), distilled water (9 mL), sodium salicylate/NaOH solution (5 mL), and 0.1% sodium dichloroisocyanurate solution was prepared and allowed to stand for 30 min. A spectrophotometer was used to measure the absorbance at 690 nm [34]. Ammonium standard solutions were prepared using ammonium chloride.
The one-way analysis of variance (ANOVA) was carried out to analyze the significance of data using Statistix version 9. The Least Significant Difference at a 5% probability level was used to identify differences among means. R-studio (2022.12.0) was used for Pearson correlation (corrplot package).
The okra performance improved significantly with the use of biostimulants. In this study, all the treatments were compared with control group plants that received chemical fertilizers.
Foliar application of biostimulants influences the photosynthetic pigments in leaves. For okra, chlorophyll a contents were significantly enhanced with G.L. (12.51%) as compared to C.K. (Fig. 1A). V.B. significantly improved the chlorophyll b contents in okra leaves (7.14%) compared to C.K. Whereas G.L. indicated the lowest values for chlorophyll b contents (Fig. 1B).
Figure 1: Physiological attributes in okra: (A) chlorophyll a (B) chlorophyll b (C) relative water content using chemical fertilizer (C.K.), glycine (G.L.), lysine (L.Y.), aspartic acid (A.A.), and vitamin B complex (V.B.). The values mentioned herein are indicated as mean ± S.D., and lowercase letters indicate statistical differences among means (p ≤ 0.05)
Biostimulants positively influence the relative water contents in leaves. Results indicated the lowest relative water content with A.A. While all other treatments, including V.B. (10.58%), G.L. (9.50%), and L.Y. (7.64%) significantly increased the relative water content as compared to C.K. (Fig. 1C).
3.2.1 Plant Fresh and Dry Weight
Results demonstrated that the application of biostimulants positively influenced plant fresh and dry weight. V.B. and A.A. significantly improved the plant fresh weight reporting by 44.54% and 43.15% increase as compared to C.K. Moreover, L showed a 9.10% increase, and G was insignificant in relation to C.K. (Fig. 2A). Plant dry weight significantly increased with V.B. (66.80%) followed by A.A. (32.05%) and G.L. (13.24%) as compared to C.K. Whereas, L.Y. showed non-significant results (Fig. 2B).
Figure 2: Plant weight in okra: (A) plant fresh weight (B) plant dry weight (C) pod fresh weight (D) pod dry weight using chemical fertilizer (C.K.), glycine (G.L.), lysine (L.Y.), aspartic acid (A.A.), and vitamin B complex (V.B.). The values mentioned herein are indicated as mean ± S.D., and lowercase letters indicate statistical differences among means (p ≤ 0.05)
Chemical fertilization significantly increased the fresh weight of okra pod (fruit; 10.12 g pot−1), followed by G.L. (6.95 g pot−1), L.Y. (6.81 g pot−1), A.A. (6.22 g pot−1), and V.B. (5.64 g pot−1; Fig. 2C). Besides this, C.K. showed the highest dry weight of okra pod, followed by L.Y. (1.10 g pot−1), G.L. (0.99 g pot−1), A.A. (0.88 g pot−1) and V.B. (0.77 g pot−1, Fig. 2D).
Plant morphological attributes were significantly improved with the foliar application of biostimulants. Plant height was significantly increased in A.A. (44.32%) and V.B. (38.87%), followed by G.L. (26.57%) and L.Y. (15.59%) as compared to C.K. (Fig. 3A). Moreover, the average number of leaves (pot−1) was also positively influenced by the application of biostimulants. It significantly improved with V.B. (38.67%), L.Y. (31.28%), G.L. (26.07%), and A.A. (21.72%) as compared to C.K. (Fig. 3B). Stem diameter was significantly improved with the use of biostimulants as compared to C.K. (Fig. 3C). Leaf area was significantly enhanced with V.B. (104.53%) followed by A.A. (74.77%) as compared to C.K. (Fig. 3D).
Figure 3: Plant morphology in okra: (A) plant height (B) average no. of leaves (C) stem diameter (D) leaf area using chemical fertilizer (C.K.), glycine (G.L.), lysine (L.Y.), aspartic acid (A.A.), and vitamin B complex (V.B.). The values mentioned herein are indicated as mean ± S.D., and lowercase letters indicate statistical differences among means (p ≤ 0.05)
Total phenolic compounds were improved in fruit significantly with V.B. (32.56%) followed by A.A. (14.99%), G.L. (5.03%), and L.Y. (3.01%) as compared to C.K. (Fig. 4A). The application of biostimulants contributed to significant changes in the quality. The foliar application of G.L. significantly improved the protein content (126.74%), followed by L.Y. (106.55%), V.B. (82.26%), and A.A. (69.28%) as compared to C.K. (Fig. 4B). Foliar application of biostimulants significantly influenced the ascorbic acid contents in okra pods. The results demonstrated that L.Y. (54.48%) significantly increased the ascorbic acid content, followed by G.L. (37.46%) as compared to C.K. Whereas, A.A. (30.91%) and V.B. (28.29%) were statistically similar (Fig. 4C).
Figure 4: Quality attributes: (A) total phenolic contents (B) total protein contents (C) ascorbic acid using chemical fertilizer (C.K.), glycine (G.L.), lysine (L.Y.), aspartic acid (A.A.), and vitamin B complex (V.B.). The values mentioned herein are indicated as mean ± S.D., and lowercase letters indicate statistical differences among means (p ≤ 0.05)
The application of biostimulants contributed to significant changes in the nutritional content. The results revealed that all the treatments significantly improved the N concentration in shoot and pod compared to C.K. The highest N concentration in okra shoot was observed in plants receiving V.B. treatment (87.42%), followed by G.L. (68.55%) and L.Y. (59.75%) as compared to C.K. In pods, A.A. improved the N concentration (67.27%), followed by V.B. (54.55%) as compared to C.K. L.Y. (29.09%) and G.L. (20.00%) also improved the N concentration in pods. Lowest N concentration was observed in C.K. (Fig. 5A). Phosphorus concentration in shoots and pods was also improved. L.Y. (48.30%) significantly improved the total P concentration in the shoot, followed by G.L. (33.98%) as compared to C.K. Whereas, G.L. also improved P concentration in the pod (12.64%) as compared to C.K. All other treatments were not effective than C.K. (Fig. 5B). In the case of K concentration in plants, all treatments showed non-significant results (Fig. 5C).
Figure 5: Nutrient concentration in okra: (A) nitrogen in shoot and pod (B) phosphorus in shoot and pod (C) potassium in shoot and pod using chemical fertilizer (C.K.), glycine (G.L.), lysine (L.Y.), aspartic acid (A.A.), and vitamin B complex (V.B.). The values mentioned herein are indicated as mean ± S.D., and lower and upper-case letters indicate statistical differences among means (p ≤ 0.05)
Foliar application of biostimulants slightly influenced the soil properties (Table 1). Results revealed that C.K. showed the highest pH value (8.74) while E.C. showed non-significant results. Soil organic matter was significantly improved with V.B. (157.36%) followed by L.Y. (142.03%), A.A. (119.03%), and G.L. (106.98%) as compared to C.K. Soil N was also enhanced with L.Y. (176.32%), V.B. (162.89%) followed by A.A. (138.48%) and G.L. (98.76%) as compared to C.K. Soil P was significantly reduced with C.K. while all other treatments improved the value. In addition, soil K was significantly improved with the L.Y. (29.06%) followed by G.L. (19.08%) as compared to C.K. Other treatments reduced the K concentration in soil. Compared to C.K., soil urease enzyme activity was significantly enhanced with the application of V.B. (21.08%) and L.Y. (18.67%), followed by G.L. (14.73%) and A.A. (6.06%).
Pearson correlation shows the association among different parameters. Blue indicates a positive correlation, red indicates a negative correlation, and color intensity indicates the strength of association among the parameters (Fig. 6). The higher the color intensity, the stronger the correlation will be. Results demonstrated that plant fresh weight has a positive association with biometric and quality parameters, as well as soil parameters; however, it has a negative correlation with fresh and dry weight of pod, P, and K in shoot and pod. A similar trend was observed with plant dry weight. Pod fresh and dry weight has a weak positive correlation with P in shoot and K in shoot and root, showing a negative association with all other parameters. In addition, a positive correlation among the average number of leaves, leaf area, stem diameter, phenol, ascorbic acid, protein in the pod, and N in the shoot and pod was observed (Fig. 6).
Figure 6: Pearson correlation. Chl a is chlorophyll a, chl b is chlorophyll b, RWC is relative water content, PFW is plant fresh weight, PDW is plant dry weight, pFW is pod fresh weight, pDW is pod dry weight, P.H. is plant height, A.L. is the average number of leaves, S.D. is stem diameter, L.A. is leaf area, Phe is phenolic content, Pro is protein contents, Asc is ascorbic acid content, sN is straw nitrogen, pN is pod nitrogen, sP is straw phosphorus, pP is pod phosphorus, sK is straw potassium, pK is pod potassium, spH is soil pH, sEC is soil E.C., sTN is soil total nitrogen, sAP is soil available phosphorus, sAK is soil available potassium, and sUEA is soil urease enzyme activity
Leaf chlorophyll content was significantly improved with the application of amino acid-based biostimulants. Green leaves are an indication of a healthy plant and proper plant functioning [35]. Chlorophyll a contents in okra leaves were improved with G.L., while chlorophyll b contents were improved with V.B. (Fig. 1A,B). It might be due to improved water and ion use efficiency, enhanced photosynthetic activity and stomatal conductance, and better bioactive compounds, i.e., vitamins, amino acids, and mineral nutrients [19]. In addition, chlorophyll a is directly involved in photosynthesis [36]. Chlorophyll b contributes to enhancing cell metabolism and synthesizing secondary metabolites in plants because it absorbs a wider range of wavelengths [37]. In addition, vitamins also play an integral role in the biosynthesis of amino acids, organelle-specific compounds, fatty acids, neurotransmitters, and plant hormones. Concurrently, amino acids act as osmolytes and also contribute to bio-membrane integrity and ion transport. Amino acids are also a source of N and increase the chlorophyll content in plants [38].
The foliar application of biostimulants enhanced the relative water content (Fig. 1C) indicating improved photosynthesis and better plant growth. The leaf relative water content was improved with V.B., G.L., and L.Y. It could be due to the better plant pressure potential, elevation of water absorption in plant cells, and enhanced water uptake. For instance, G.L. has the potential to enhance water uptake in plants and protect plasma membrane [39]. Furthermore, Vitamin B also prevents cell damage due to its antioxidant properties [9].
The plant biomass was also improved with the application of biostimulants (Fig. 2). It might be associated with improved water and nutrient uptake, nutrient assimilation, and plant photosynthesis [40]. Amino acids influence primary and secondary metabolic processes, plant physiological processes, vegetative growth, and fruit maturity [41]. Biostimulants containing amino acids also act as a precursor for endogenous plant hormones. The bioactive compound induces signaling pathways in plants; for instance, amino acids act as signaling molecules and regulate the growth and development of plants [42]. Vitamin B1 is a cofactor that significantly improves primary metabolic processes in plants (glycolysis, tricarboxylic acid cycle, and pentose phosphate pathway), ultimately increasing plant growth performances [43]. Photosynthetic efficiency, chlorophyll formation, cell enlargement, and formation of growth hormones, including auxin, gibberellin, and cytokine, might be the reason behind improved crop production [9]. Pearson correlation indicated that the plant height, plant fresh and dry weight, leaf area, and the average number of leaves clearly showed the role of biostimulants in plant growth and performance (Fig. 6). Literature reported that enhanced crop production including bean (Phaseolus vulgaris L.), soya bean (Glycine max L.), Lavandin (Lavandula × intermedia), potatoes (Solanum tuberosum L.), and lettuce with the application of biostimulants [44–47].
The number of leaves, plant height, stem diameter, and leaf area were improved with biostimulants (Fig. 3). It might be due to multiple reasons such as triggering N metabolism or P release from soil to enhancing root growth and plant development [28]. In addition, huge energy is required to produce amino acids and proteins themselves; hence, the exogenous application of biostimulants promotes plant growth and development [10].
Studied experiments showed improvement in phenolic contents with the application of biostimulants (Fig. 4A). It might be associated with the positive effect of amino acids in plants, which play a vital role in secondary metabolites’ metabolism and are strongly linked with hormones, proteins, and carbohydrate synthesis [48]. The plants promptly absorb amino acids, become a stable source of molecule precursors, and contribute to plant metabolic activities [49]. Phenolic compounds participate as anti-feedants, plant pigmentation, phytoalexins, anti-oxidants, and protectants. Various studies reported that the foliar application of biostimulants improved the total phenolic contents in plants. Capsicum annuum L., when applied with natural biostimulants, improved the plant phenolic contents grown hydroponically [50], tomato plants also improved the phenolic and ascorbic acid concentration with the application of biostimulants under field conditions [51] and foliar application of amino-acids based biostimulants also increased the phenolic contents in lettuce cultivars grown in soilless conditions [48].
Recent findings reported that foliar or soil application of amino acids could improve plant protein content [52]. Moreover, amino acids are building blocks of protein and protein synthesis and act as a precursor for enzymes involved in protein synthesis. Proteins play an integral role in the plant cell in producingmembrane protein and enzymes, and GLY has been reported to stabilize the membrane and protect the denaturation of enzymes [53] The observations suggest that plants stimulate two metabolic pathways when applied with biostimulants, i.e., N primary metabolism produces proteins, and secondary metabolism involves synthesizing phenolic compounds.
The increase in protein content may also be associated with improved carbohydrate and sugar content in leaves. Carbohydrates are essential in incorporating ammonia in amino acids and enhancing protein biosynthesis. At the same time, sugars accelerate N inclusions through the nitrate assimilation pathway. In addition, sugars are also a good energy source and promote N uptake in plants. Improved chlorophyll content and net photosynthesis are critical indicators for enhancing sugar biosynthesis [28]. Ascorbic acid contents were improved with the application of biostimulants (Fig. 4C). It might be associated with the role of biostimulants in plant metabolism, including vitamin C synthesis [54].
The application of biostimulants significantly influenced the nutrient concentration in shoot and pods (Fig. 5). Possibly through, exogenously applied amino acids act as a signal molecule and influence hormones’ action via amino acid conjugation. Literature also reported that plant leaves immediately absorb and translocate amino-acids-based biostimulants and later can perform various functions in plants as modulators for stomatal openings, transport regulators, compatible osmolytes, detoxify heavy metals, and signaling molecules [42]. Glycine has a better potential to release N molecules than urea [55]. Lysine also plays numerous roles in plant growth and development. It has been reported that lysine induces serotonin accumulation and the jasmonate signaling pathway in rice. Moreover, it also has a role in plant growth and development, energy metabolism, and also develops systemic immunity in plants [56].
Mirtaleb et al. indicated that amino acids could induce N enzyme activity in plants leading to improved N concentration in leaves and improved protein synthesis. In addition, amino acids are a source of N and a practical component in plant nutrient use efficiency [57]. These are intermediate compounds in N assimilation and the main form of N translocation from phloem to other parts of plants. Thus, the application of amino acids can reduce nitrate accumulation in plants. Furthermore, biostimulation of amino acids also improves nutrient uptake [52] in plants, as reported through current studies where biostimulants’ application helped plants uptake nutrients from the soil.
The mechanisms of action of biostimulants are very diverse and may include P release from soils, generic stimulation of soil microbial activity, and root growth enhancement. Moreover, amino acids-based biostimulants increase plant productivity—improving ion transport, photosynthesis, assimilation of essential nutrients such as N, sulphur, and carbon [58], and nutrient uptake [59]. The findings of our study are consistent with previous studies where nutrient availability was increased with the application of amino-acids-based biostimulants [58], improving nutrient availability in sweet yellow pepper [50] and N uptake efficiency was improved in baby spinach and lamb’s lettuce [60].
The results of our study revealed that the application of biostimulants did not influence soil pH and E.C. (Table 1). It might be due to the application method, i.e., foliar, used in this experiment. Besides this, soil organic matter was little influenced, and it might be associated with the high rates of N application that activate the soil organism and decomposition that ultimately deplete organic matter and soil P. Moreover, the biostimulants used in these experiments were not a source of P and K. However, they influenced the uptake of nutrients, leading to changes in soil properties. It might be associated with the release of organic compounds produced by the plants that alter the root environment and improve the P availability [61].
Plants play an active role in transforming soil nutrient status through root exudates and root residues [62]. Plants’ roots face various forms of N in soil including organic and inorganic N. More than 90% of organic N (amino acids, amino-sugars, vitamins, amines, proteins) whereas a small amount of mineral N exists in soil. Mineralization of N takes place with the help of urease enzyme [63]. Amino acids are the main component of root exudates and also contribute to influencing N uptake in plants [64]. The presence of amino acids in soil contributes to the activity of microorganisms, which might be a reason for enhancing soil urease enzyme activity, decomposing the organic forms of N to available forms, and improving N uptake in plants [65]. It is reported that the urease enzyme activity has a positive correlation with soil N contents [66].
4.6 Implementation of the Study
The practical implementation of the study is important to minimize the environmental hazards due to the excessive use of chemical fertilizers. The study discusses the potential of biostimulants in improving crop performance and yield. Some published studies also support the idea that using biostimulants as an alternative to chemical fertilizers can reduce environmental pollution without compromising crop yield [67,68]. Although it is a pot experiment, it is still an innovative approach to organic farming. Moreover, small-scale farmers, as well as kitchen gardeners, can prefer biostimulants over chemical fertilizers. It is further recommended to perform short and long-term experiments including field trials, and crop and site-specific studies, different times and methods of application need to be addressed. Moreover, the economic aspects and the impacts of biostimulants on nutrient losses and gas emissions are also a research gap.
Biostimulants are an eco-friendly alternative to chemical fertilizers that improve crop quality and performance. The results of our study demonstrated an improvement in plant physiology, biometrics, quality, and nutritional attributes of the crop. However, a minimal reduction in fruit yield was observed. It elaborated that different combinations of biostimulants, or integration of chemical fertilizers could help enhance crop yield.
Moreover, biostimulants are complex multi-component products, making it difficult to understand their ‘mode of action’ in plants. Due to its tremendous outcomes, it is an excellent challenge for the scientific community to conduct further research using biostimulants and identify their ‘mechanism of action’ using advanced technology, bioinformatics, plant biology, and various other methodologies. In the future, long-term field trials need to be conducted to identify the potential of biostimulants. In addition, it is also important to explore the suitable time and dose of biostimulants, the influence of temperature and climatic conditions on crop performance when applied with biostimulants, the leeching and volatilization losses, and its impact on different crop rotations. Furthermore, the mechanism behind the benefits of biostimulants gained by the plants needs to be identified.
Acknowledgement: We extend our appreciation to the Researchers Supporting Project (no. RSP2025R218), King Saud University, Riyadh, Saudi Arabia. We also acknowledge the Higher Education Commission (HEC) for providing funds to Qurat-Ul-Ain Raza (graduate scholar) to pursue her Ph.D. research.
Funding Statement: The authors received no specific funding for this study.
Author Contributions: The authors confirm their contribution to the paper as follows: study conception and design: Abdur Rehim, Muhammad Amjad Bashir, Mingzheng Duan, Yucong Geng, Abdulwahed Fahad Alrefaei; data collection: Qurat-Ul-Ain Raza, Muhammad Amjad Bashir; analysis and interpretation of results: Qurat-Ul-Ain Raza, Mingzheng Duan; statistical support: Yucong Geng, Abdulwahed Fahad Alrefaei; funding: Mingzheng Duan, Abdulwahed Fahad Alrefaei; supervision: Abdur Rehim, Mingzheng Duan, Abdulwahed Fahad Alrefaei; draft manuscript preparation: Qurat-Ul-Ain Raza, Muhammad Amjad Bashir, Abdur Rehim, Yucong Geng, Mingzheng Duan, Abdulwahed Fahad Alrefaei. All authors reviewed the results and approved the final version of the manuscript.
Availability of Data and Materials: The data that support the findings of this study are available from the corresponding author upon reasonable request.
Ethics Approval: Not applicable.
Conflicts of Interest: The authors declare no conflicts of interest to report regarding the present study.
References
1. Pahalvi HN, Rafiya L, Rashid S, Nisar B, Kamili AN. Chemical fertilizers and their impact on soil health. In: Microbiota and biofertilizers. Cham: Springer; 2021. vol. 2, p. 1–20. doi:10.1007/978-3-030-61010-4_1. [Google Scholar] [CrossRef]
2. Nadarajan S, Sukumaran S. Chemistry and toxicology behind chemical fertilizers. In: Controlled release fertilizers for sustainable agriculture. Cambridge, MA, USA: Academic Press; 2021. p. 195–229. doi:10.1016/b978-0-12-819555-0.00012-1. [Google Scholar] [CrossRef]
3. Yu X, Keitel C, Zhang Y, Wangeci AN, Dijkstra FA. Global meta-analysis of nitrogen fertilizer use efficiency in rice, wheat and maize. Agric Ecosyst Environ. 2022;338:108089. doi:10.1016/j.agee.2022.108089. [Google Scholar] [CrossRef]
4. Ahmed M, Rauf M, Mukhtar Z, Saeed NA. Excessive use of nitrogenous fertilizers: an unawareness causing serious threats to environment and human health. Environ Sci Pollut Res. 2017;24(35):26983–7. doi:10.1007/s11356-017-0589-7. [Google Scholar] [PubMed] [CrossRef]
5. Noor MA. Nitrogen management and regulation for optimum NUE in maize—a mini review. Cogent Food Agric. 2017;3(1):1348214. doi:10.1080/23311932.2017.1348214. [Google Scholar] [CrossRef]
6. Koleška I, Hasanagić D, Todorović V, Murtić S, Klokić I, Paradiković N, et al. Biostimulant prevents yield loss and reduces oxidative damage in tomato plants grown on reduced NPK nutrition. J Plant Interact. 2017;12(1):209–18. doi:10.1080/17429145.2017.1319503. [Google Scholar] [CrossRef]
7. Bashir MA, Rehim A, Raza Q-U-A, Muhammad Ali Raza H, Zhai L, Liu H, et al. Biostimulants as plant growth stimulators in modernized agriculture and environmental sustainability. Technol Agricult. 2021. doi:10.5772/intechopen.98295. [Google Scholar] [CrossRef]
8. Berlyn GP, Sivaramakrishnan S. The use of organic biostimulants to reduce fertilizer use, increase stress resistance, and promote growth. In: Northeastern Forest Nursery Association Conference, 1996; New England, CT, USA. [Google Scholar]
9. Rehim A, Amjad Bashir M, Raza Q-U-A, Gallagher K, Berlyn GP. Yield enhancement of biostimulants, vitamin B12, and CoQ10 compared to inorganic fertilizer in radish. Agronomy. 2021;11(4):697. doi:10.3390/agronomy11040697. [Google Scholar] [CrossRef]
10. Raza Q, Bashir MA, Rehim A, Geng Y, Raza HMA, Hussain S, et al. Identifying the role of biostimulants in turnip (Brassica rapa L.) production compared with chemical fertilization. Sustainability. 2023;15(15):11851. doi:10.3390/su151511851. [Google Scholar] [CrossRef]
11. Fernandes Â., Polyzos N, Mandim F, Pereira C, Petrović J, Soković M, et al. Combined effect of biostimulants and mineral fertilizers on crop performance and fruit quality of watermelon plants. Horticulturae. 2023;9(7):838. doi:10.3390/horticulturae9070838. [Google Scholar] [CrossRef]
12. Johnson R, Joel JM, Puthur JT. Biostimulants: the futuristic sustainable approach for alleviating crop productivity and abiotic stress tolerance. J Plant Growth Regul. 2024;43(3):659–74. doi:10.1007/S00344-023-11144-3. [Google Scholar] [CrossRef]
13. du Jardin P, Xu L, Geelen D. Agricultural functions and action mechanisms of Plant Biostimulants (PBsan introduction. Chem Biol Plant Biostimulants. 2020;8(1):3–30. doi:10.1002/9781119357254.CH1. [Google Scholar] [CrossRef]
14. Mohammadipour N, Souri MK. Beneficial effects of glycine on growth and leaf nutrient concentrations of coriander (Coriandrum sativum) plants. J Plant Nutr. 2019;42(14):1637–44. doi:10.1080/01904167.2019.1628985. [Google Scholar] [CrossRef]
15. Mosa WFA, Salem MZM, Al-Huqail AA, Ali HM. Application of glycine, folic acid, and moringa extract as bio-stimulants for enhancing the production of ‘Flame Seedless’ grape cultivar. Bioresources. 2021;16(2):3391–410. doi:10.15376/BIORES.16.2.3391-3410. [Google Scholar] [CrossRef]
16. Noroozlo YA, Souri MK, Delshad M. Stimulation effects of foliar applied glycine and glutamine amino acids on lettuce growth. Open Agric. 2019;4(1):164–72. doi:10.1515/OPAG-2019-0016/MACHINEREADABLECITATION/RIS. [Google Scholar] [CrossRef]
17. Alfosea-Simón M, Simón-Grao S, Zavala-Gonzalez EA, Cámara-Zapata JM, Simón I, Martínez-Nicolás JJ, et al. Application of biostimulants containing amino acids to tomatoes could favor sustainable cultivation: implications for tyrosine, lysine, and methionine. Sustainability. 2020;12(22):1–19. doi:10.3390/su12229729. [Google Scholar] [CrossRef]
18. Sadak MS, Sekara A, Al-ashkar I, Habib-ur-Rahman M, Skalicky M, Brestic M, et al. Exogenous aspartic acid alleviates salt stress-induced decline in growth by enhancing antioxidants and compatible solutes while reducing reactive oxygen species in wheat. Front Plant Sci. 2022;13:987641. doi:10.3389/FPLS.2022.987641/BIBTEX. [Google Scholar] [CrossRef]
19. Raza QUA, Bashir MA, Rehim A, Ejaz R, Raza HMA, Shahzad U, et al. Biostimulants induce positive changes in the radish morpho-physiology and yield. Front Plant Sci. 2022;13:2475. doi:10.3389/FPLS.2022.950393. [Google Scholar] [PubMed] [CrossRef]
20. Elkhalifa AEO, Alshammari E, Adnan M, Alcantara JC, Awadelkareem AM, Eltoum NE, et al. Okra (Abelmoschus esculentus) as a potential dietary medicine with nutraceutical importance for sustainable health applications. Molecules. 2021;26(3):696. doi:10.3390/MOLECULES26030696. [Google Scholar] [PubMed] [CrossRef]
21. Dantas TL, Alonso Buriti FC, Florentino ER. Okra (Abelmoschus esculentus L.) as a potential functional food source of mucilage and bioactive compounds with technological applications and health benefits. Plants. 2021;10(8):1683. doi:10.3390/plants10081683. [Google Scholar] [PubMed] [CrossRef]
22. Gemede HF. Nutritional quality and health benefits of okra (Abelmoschus esculentusa review. J Food Process Technol. 2015;6(458):2. doi:10.4172/2157-7110.1000458. [Google Scholar] [CrossRef]
23. Mathiba MT, Reddy GVS, Ntushelo K, Kanu SA. The potential of omics technologies as tools to understand the environmental factors influencing okra (Abelmoschus esculentus) growth and adaptation. S Afr J Plant Soil. 2018;35(1):1–8. doi:10.1080/02571862.2017.1335891. [Google Scholar] [CrossRef]
24. He J, Reddy GVP, Liu M, Shi P. A general formula for calculating surface area of the similarly shaped leaves: evidence from six Magnoliaceae species. Glob Ecol Conserv. 2020;23:e01129. doi:10.1016/J.GECCO.2020.E01129. [Google Scholar] [CrossRef]
25. Brighente IMC, Dias M, Verdi LG, Pizzolatti MG. Antioxidant activity and total phenolic content of some Brazilian species. Pharm Biol. 2007;45(2):156–61. doi:10.1080/13880200601113131. [Google Scholar] [CrossRef]
26. Lu Z-L, Liu L-W, Li X-Y, Gong Y-Q, Hou X-L, Zhu X-W, et al. Analysis and evaluation of nutritional quality in Chinese Radish (Raphanus sativus L.). Agric Sci China. 2008;7(7):823–30. doi:10.1016/S1671-2927(08)60119-4. [Google Scholar] [CrossRef]
27. Waterborg JH. The lowry method for protein quantitation. Totowa, NJ, USA: Humana Press; 2009. p. 7–10. doi:10.1007/978-1-59745-198-7_2. [Google Scholar] [CrossRef]
28. Godlewska K, Pacyga P, Michalak I, Biesiada A, Szumny A, Pachura N, et al. Systematic investigation of the effects of seven plant extracts on the physiological parameters, yield, and nutritional quality of radish (Raphanus sativus var. sativus). Front Plant Sci. 2021;12:651152. doi:10.3389/fpls.2021.651152. [Google Scholar] [PubMed] [CrossRef]
29. Kjeldahl J. Neue Methode zur Bestimmung des Stickstoffs in organischen Körpern. Zeitschrift Für Analytische Chemie. 1883;22(1):366–82. doi:10.1007/BF01338151. [Google Scholar] [CrossRef]
30. Walkley A. A critical examination of a rapid method for determining organic carbon in soils—effect of variations in digestion conditions and of inorganic soil constituents. Soil Sci. 1947;63(4):251–64. doi:10.1097/00010694-194704000-00001. [Google Scholar] [CrossRef]
31. Jackson ML. Soil chemical analysis. J Agric Food Chem. 1960;7(2). doi:10.1021/jf60096a605. [Google Scholar] [CrossRef]
32. Olsen SR. Estimation of available phosphorus in soils by extraction with sodium bicarbonate. US Dep Agric Circ. 1954;939:1–19. [Google Scholar]
33. Richards LA. Diagnosis and improving of saline and alkaline soils. Washington, DC, USA: US Department of Agriculture; 1954. [Google Scholar]
34. Kandeler E, Gerber H. Short-term assay of soil urease activity using colorimetric determination of ammonium. Biol Fertil Soils. 1988;6(1):68–72. doi:10.1007/BF00257924. [Google Scholar] [CrossRef]
35. Souri MK, Hatamian M. Aminochelates in plant nutrition: a review. J Plant Nutr. 2019;42(1):67–78. doi:10.1080/01904167.2018.1549671. [Google Scholar] [CrossRef]
36. Yang X, Wang J, Xia X, Zhang Z, He J, Nong B, et al. OsTTG1, a WD40 repeat gene, regulates anthocyanin biosynthesis in rice. Plant J. 2021;107(1):198–214. doi:10.1111/tpj.15285. [Google Scholar] [PubMed] [CrossRef]
37. Toscano S, Romano D, Patanè C. Effect of application of biostimulants on the biomass, nitrate, pigments, and antioxidants content in radish and turnip microgreens. Agronomy. 2023;13(1):145. doi:10.3390/agronomy13010145. [Google Scholar] [CrossRef]
38. Shafie F, Bayat H, Aminifard MH, Daghighi S. Biostimulant effects of seaweed extract and amino acids on growth, antioxidants, and nutrient content of yarrow (Achillea millefolium L.) in the field and greenhouse conditions. Commun Soil Sci Plant Anal. 2021;52(9):964–75. doi:10.1080/00103624.2021.1872596. [Google Scholar] [CrossRef]
39. Miri MR, Ghooshchi F, Tohidi-Moghadam HR, Larijani HR, Kasraie P. Ameliorative effects of foliar spray of glycine betaine and gibberellic acid on cowpea (Vigna unguiculata L. Walp.) yield affected by drought stress. Arab J Geosci. 2021;14(10):1–9. doi:10.1007/s12517-021-07228-7. [Google Scholar] [CrossRef]
40. Haghighi M, Saadat S, Abbey L. Effect of exogenous amino acids application on growth and nutritional value of cabbage under drought stress. Sci Hortic. 2020;272(6):109561. doi:10.1016/j.scienta.2020.109561. [Google Scholar] [CrossRef]
41. Alfosea-Simón M, Simón-Grao S, Zavala-Gonzalez EA, Cámara-Zapata JM, Simón I, Martínez-Nicolás JJ, et al. Physiological, nutritional and metabolomic responses of tomato plants after the foliar application of amino acids aspartic acid, glutamic acid and alanine. Front Plant Sci. 2021;11:2138. doi:10.3389/fpls.2020.581234. [Google Scholar] [PubMed] [CrossRef]
42. Yakhin OI, Lubyanov AA, Yakhin IA, Brown PH. Biostimulants in plant science: a global perspective. Front Plant Sci. 2017;7:2049. doi:10.3389/fpls.2016.02049. [Google Scholar] [PubMed] [CrossRef]
43. Soppelsa S, Kelderer M, Casera C, Bassi M, Robatscher P, Matteazzi A, et al. Foliar applications of biostimulants promote growth, yield and fruit quality of strawberry plants grown under nutrient limitation. Agronomy. 2019;9(9):483. doi:10.3390/agronomy9090483. [Google Scholar] [CrossRef]
44. Szparaga A, Kocira S, Kapusta I, Zaguła G. Prototyping extracts from Artemisia absinthium L. for their biostimulating properties yield-enhancing, and farmer income-increasing properties. Ind Crops Prod. 2021;160(6):113125. doi:10.1016/j.indcrop.2020.113125. [Google Scholar] [CrossRef]
45. Kocira S, Szparaga A, Kocira A, Czerwińska E, Wójtowicz A, Bronowicka-Mielniczuk U, et al. Modeling biometric traits, yield and nutritional and antioxidant properties of seeds of three soybean cultivars through the application of biostimulant containing seaweed and amino acids. Front Plant Sci. 2018;9:343695. doi:10.3389/fpls.2018.00388. [Google Scholar] [PubMed] [CrossRef]
46. Caccialupi G, Caradonia F, Ronga D, Ben Hassine M, Truzzi E, Benvenuti S, et al. Plant biostimulants increase the agronomic performance of lavandin (Lavandula x intermedia) in Northern Apennine range. Agronomy. 2022;12(9):2189. doi:10.3390/agronomy12092189. [Google Scholar] [CrossRef]
47. Di Mola I, Cozzolino E, Ottaiano L, Giordano M, Rouphael Y, Colla G, et al. Effect of vegetal- and seaweed extract-based biostimulants on agronomical and leaf quality traits of plastic tunnel-grown baby lettuce under four regimes of nitrogen fertilization. Agronomy. 2019;9(10):571. doi:10.3390/agronomy9100571. [Google Scholar] [CrossRef]
48. Al-Karaki GN, Othman Y. Effect of foliar application of amino acids biostimulants on growth, macronutrient, total phenols contents and antioxidant activity of soilless grown lettuce cultivars. S Afr J Bot. 2023;154:225–31. doi:10.1016/j.sajb.2023.01.034. [Google Scholar] [CrossRef]
49. Rouphael Y, Colla G. Toward a sustainable agriculture through plant biostimulants: from experimental data to practical applications. Agronomy. 2020;10(10):1461. doi:10.3390/agronomy10101461. [Google Scholar] [CrossRef]
50. Paradiković N, Vinković T, Vinković Vrček I, Žuntar I, Bojić M, Medić-Šarić M. Effect of natural biostimulants on yield and nutritional quality: an example of sweet yellow pepper (Capsicum annuum L.) plants. J Sci Food Agric. 2011;91(12):2146–52. doi:10.1002/jsfa.4431. [Google Scholar] [PubMed] [CrossRef]
51. Caruso G, De Pascale S, Cozzolino E, Cuciniello A, Cenvinzo V, Bonini P, et al. Yield and nutritional quality of Vesuvian piennolo tomato PDO as affected by farming system and biostimulant application. Agronomy. 2019;9(9):1–14. doi:10.3390/agronomy9090505. [Google Scholar] [CrossRef]
52. Noroozlo YA, Souri MK, Delshad M. Effects of foliar application of glycine and glutamine amino acids on growth and quality of sweet basil. Adv Hort Sci. 2019;33(4):495–502. [Google Scholar]
53. Gupta P, Rai R, Vasudev S, Yadava DK, Dash PK. Ex-foliar application of glycine betaine and its impact on protein, carbohydrates and induction of ROS scavenging system during drought stress in flax (Linum usitatissimum). J Biotechnol. 2021;337(10):80–9. doi:10.1016/j.jbiotec.2021.06.012. [Google Scholar] [PubMed] [CrossRef]
54. Drobek M, Frąc M, Cybulska J. Plant biostimulants: importance of the quality and yield of horticultural crops and the improvement of plant tolerance to abiotic stress—a review. Agronomy. 2019;9(6):335. doi:10.3390/agronomy9060335. [Google Scholar] [CrossRef]
55. McCoy RM, Meyer GW, Rhodes D, Murray GC, Sors TG, Widhalm JR. Exploratory study on the foliar incorporation and stability of isotopically labeled amino acids applied to turfgrass. Agronomy. 2020;10(3):358. doi:10.3390/agronomy10030358. [Google Scholar] [CrossRef]
56. Yang Q, Zhao D, Zhang C, Sreenivasulu N, Sun SSM, Liu Q. Lysine biofortification of crops to promote sustained human health in the 21st century. J Exp Bot. 2022;73(5):1258–67. doi:10.1093/jxb/erab482. [Google Scholar] [PubMed] [CrossRef]
57. Mirtaleb SH, Niknejad Y, Fallah H. Foliar spray of amino acids and potassic fertilizer improves the nutritional quality of rice. J Plant Nutr. 2021;44(14):2029–41. doi:10.1080/01904167.2021.1889588. [Google Scholar] [CrossRef]
58. Navarro-León E, López-Moreno FJ, Borda E, Marín C, Sierras N, Blasco B, et al. Effect of l-amino acid-based biostimulants on nitrogen use efficiency (NUE) in lettuce plants. J Sci Food Agric. 2022;102(15):7098–106. doi:10.1002/JSFA.12071. [Google Scholar] [PubMed] [CrossRef]
59. Rouphael Y, Colla G. Synergistic biostimulatory action: designing the next generation of plant biostimulants for sustainable agriculture. Front Plant Sci. 2018;9:1655. doi:10.3389/fpls.2018.01655. [Google Scholar] [PubMed] [CrossRef]
60. Di Mola I, Cozzolino E, Ottaiano L, Nocerino S, Rouphael Y, Colla G, et al. Nitrogen use and uptake efficiency and crop performance of baby spinach (Spinacia oleracea L.) and Lamb’s Lettuce (Valerianella locusta L.) grown under variable sub-optimal N regimes combined with plant-based biostimulant application. Agronomy. 2020;10(2):278. doi:10.3390/agronomy10020278. [Google Scholar] [CrossRef]
61. Lal MK, Vengavasi K, Pandey R. Interactive effects of low phosphorus and elevated CO2 on root exudation and nutrient uptake in wheat is modified under sulphur nutrition. Plant Physiol Rep. 2019;24(1):63–73. doi:10.1007/s40502-019-0433-9. [Google Scholar] [CrossRef]
62. Mergel A, Timchenko A, Kudeyarov V. Role of plant root exudates in soil carbon and nitrogen transformation. In: Root demographics and their efficiencies in sustainable agriculture, grasslands and forest ecosystems. Dordrecht: Springer; 1998. p. 43–54. doi:10.1007/978-94-011-5270-9_3. [Google Scholar] [CrossRef]
63. Ahmad E, Sharma PK, Khan MS. Roles of root exudates in different processes in the nitrogen cycle in the rhizosphere. In Soil Nitrogen Ecology. 2021;62(15):179–200. doi:10.1007/978-3-030-71206-8_8. [Google Scholar] [CrossRef]
64. Gent L, Forde BG. How do plants sense their nitrogen status? J Exp Bot. 2017;68(10):2531–39. doi:10.1093/jxb/erx013. [Google Scholar] [PubMed] [CrossRef]
65. Radkowski A, Radkowska I, Bocianowski J, Cyplik A, Wolski K, Bujak H. Effect of amino acids and effective microorganisms on meadow silage chemical composition. Agronomy. 2021;11(6):1198. doi:10.3390/agronomy11061198. [Google Scholar] [CrossRef]
66. Sun TR, Cang L, Wang QY, Zhou DM, Cheng JM, Xu H. Roles of abiotic losses, microbes, plant roots, and root exudates on phytoremediation of PAHs in a barren soil. J Hazard Mater. 2010;176(1–3):919–25. doi:10.1016/j.jhazmat.2009.11.124. [Google Scholar] [PubMed] [CrossRef]
67. Ban Z, Niu C, Li L, Gao Y, Liu L, Lu J, et al. Exogenous brassinolides and calcium chloride synergically maintain quality attributes of jujube fruit (Ziziphus jujuba Mill.). Postharvest Biol Technol. 2024;216(7):113039. doi:10.1016/J.POSTHARVBIO.2024.113039. [Google Scholar] [CrossRef]
68. Huang J, Ma H, Sedano F, Lewis P, Liang S, Wu Q, et al. Evaluation of regional estimates of winter wheat yield by assimilating three remotely sensed reflectance datasets into the coupled WOFOST-PROSAIL model. Eur J Agron. 2019;102(1):1–13. doi:10.1016/j.eja.2018.10.008. [Google Scholar] [CrossRef]
Cite This Article
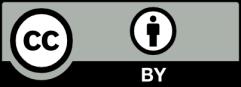
This work is licensed under a Creative Commons Attribution 4.0 International License , which permits unrestricted use, distribution, and reproduction in any medium, provided the original work is properly cited.